Blue Native Polyacrylamide Gel Electrophoresis (BN-PAGE) for the Analysis of Protein Oligomers in Plants
Lei Li, Lei Li, Pratchaya Pramoj Na Ayutthaya, Pratchaya Pramoj Na Ayutthaya, Derek Lundberg, Derek Lundberg, Detlef Weigel, Detlef Weigel
Abstract
Protein-protein interactions, including oligomerization, are involved in regulation of many cellular processes. Unfortunately, many proteins are expressed at a very low level in vivo , making it challenging to observe oligomerization by size-exclusion chromatography, also known as gel filtration. In this protocol, we present detailed steps to perform blue native polyacrylamide gel electrophoresis (BN-PAGE), a method to study protein oligomers in plants. The article describes protein sample preparation from transgenic Arabidopsis thaliana and running a BN-PAGE gel followed by direct western blotting or, alternatively, two-dimensional sodium dodecyl sulfide–polyacrylamide gel electrophoresis (2D SDS-PAGE). This protocol will be helpful for new researchers conducting related experiments to analyze stable protein interactions including homo- or hetero-oligomerization in plants. © 2020 The Authors.
Basic Protocol:
Oligomerization of proteins controls numerous biochemical features, such as the stability of proteins and the activity of enzymes, immune receptors, and ion channels (Gell, Grant, & Mackay, 2012). Gel filtration and blue native polyacrylamide gel electrophoresis (BN-PAGE) are the two principle approaches to studying native protein oligomerization in vitro and in vivo (Fiala, Schamel, & Blumenthal, 2011; see Current Protocols article; Irvine, 2001; Wittig, Braun, & Schägger, 2006). Compared to gel filtration, which requires special equipment and large quantities of protein, BN-PAGE is much more sensitive and uses easily available conventional protein gel electrophoresis equipment, while at the same time requiring lower protein quantities. In addition, BN-PAGE can be employed to detect dynamic protein oligomerization when the protein complexes are relatively stable upon different treatments.
By taking advantage of Coomassie Brilliant Blue R-250 or G-250 dye to render a net negatively charged protein, BN-PAGE gel is able to separate proteins in their native conformations, regardless of the different isoelectric points of different proteins (Crichton, Harding, Ruprecht, Lee, & Kunji, 2013). Based on their sizes, the protein molecules or complexes migrate to a position where the pore sizes of the gradient gel become restrictive. Combined with a downstream immunodetection approach, this enables determination of the molecular weights and oligomeric states of native protein complexes in plants. In this protocol, we begin with a description of procedures for isolating proteins from transgenic Arabidopsis thaliana seedlings and analyzing them by BN-PAGE and direct western blotting. We use an intracellular immune receptor nucleotide-binding domain leucine-rich repeat (NLR) protein, RPP7, as an example to illustrate how one can monitor ligand-triggered protein oligomerization in plants by BN-PAGE (Li, Habring, Wang, & Weigel, 2020). We also describe two dimensional sodium dodecyl sulfide–polyacrylamide gel electrophoresis (2D SDS-PAGE), in which the protein molecules are separated according to molecular mass, as an alternative to direct western blotting for further protein-complex analyses.
Materials
-
A. thaliana seeds (transgenic seeds expressing RPP7b-FLAG and HR4-HA proteins, referred to (Li et al., 2020))
-
70% (v/v) ethanol
-
Deionized, distilled water (ddH2O), with or without autoclaving
-
½ MS medium plates (see recipe)
-
Appropriate chemical for induction of gene expression and attendant protein expression
-
Liquid nitrogen
-
Native protein extraction buffer (see recipe; make fresh), 4°C
-
NativePAGE™ 5% G-250 Sample Additive (Thermo Fisher Scientific, cat. no. BN2004)
-
20× NativePAGE™ Running Buffer (Thermo Fisher Scientific, cat. no. BN2001)
-
20× NativePAGE™ Cathode Buffer Additive (Thermo Fisher Scientific, cat. no. BN2002)
-
NativePAGE™ 3-12% Bis-Tris Protein Gel, 1.0 mm, 10-well (Thermo Fisher Scientific, cat. no. BN2001BOX)
-
NativeMark™ Unstained Protein Standard (Thermo Fisher Scientific, cat. no. LC0725)
-
Coomassie Brilliant Blue R-250 stain solution (see recipe)
-
Coomassie Brilliant Blue R-250 destain solution (see recipe)
-
20× NuPAGE™ Transfer Buffer (Thermo Fisher Scientific, cat. no. NP0006)
-
Methanol
-
Powdered milk (Roth, cat. no. T145)
-
Phosphate-buffered saline with Tween 20 (PBST; see recipe)
-
8% (v/v) acetic acid
-
Anti-FLAG antibody (Sigma, cat. no. F1804)
-
Anti-mouse IgG–peroxidase antibody (Sigma, cat. no. A4416)
-
Western blotting detection reagent (Amersham ECL™ Prime Western Blotting Detection Reagent, GE Healthcare, cat. no. RPN2232, or Amersham ECL™ Select Western Blotting Detection Reagent, GE Healthcare, cat. no. RPN2236)
-
20× NuPAGE™ MOPS SDS Running Buffer (Thermo Fisher Scientific, cat. no. NP0001)
-
4× protein loading buffer (see recipe)
-
100 mM dithiothreitol (DTT)
-
NuPAGE™ 4-12% Bis-Tris Protein Gels, 1.0 mm, 2D-well (Thermo Fisher Scientific, cat. no. NP0326BOX)
-
PageRuler™ Prestained Protein Ladder, 10 to 180 kDa (Thermo Fisher Scientific, cat. no. 26616)
-
Anti-HA antibody (Roche, cat. no. 12013819001)
-
1.5-ml microcentrifuge tubes (Safe-Lock Tubes, Eppendorf, cat. no. 0030120086)
-
Micropestle (Roth, cat. no. CXH7.1), pre-frozen in liquid nitrogen
-
Microcentrifuge (Eppendorf 5417R), 4°C
-
Gel-load filter tips (Greiner, cat. no. 775288)
-
XCell SureLock™ Mini-Cell (Thermo Fisher Scientific, cat. no. EI0001)
-
Electrophoresis power supply (EPS 301, GE Healthcare, cat. no. 18-1130-01, or similar instrument)
-
Gel knife (Thermo Fisher Scientific, cat. no. EI9010)
-
Platform shaker
-
Immun-Blot® PVDF membrane (Bio-Rad, cat. no. 1620177)
-
Mini Trans-Blot Electrophoretic Transfer Cell (Bio-Rad, cat. no. 1703930), including gel holder cassette and foam pads
-
Blotting papers, grade GB003 (GE Healthcare, cat. no. 10426892)
-
Magnetic stir plate and stir bar
-
6 × 10–cm container
-
Film (Hyperfilm MP, GE Healthcare, cat. no. 28906844)
-
Film processor (AGFA CP 1000 LC 50 or similar instrument)
-
Microwave
-
Additional reagents and equipment for immunoblotting following 2D SDS-PAGE (see Current Protocols article; Ni, Xu, Sabanayagam, & Gallagher, 2016)
Plant growth
1.Sterilize ∼50 A. thaliana seeds per sample in 1 ml of 70% ethanol for 2 min and then wash seeds five times with 1 ml autoclaved ddH2O each. Stratify sterile seeds at 4°C for 2 days. Then, distribute seeds across two ½ MS medium plates (one for induced expression, the other for control) and cultivate under long-day conditions (16 hr of light/8 hr of dark) at 23°C for 9 days.
2.Spray one plate with an appropriate chemical for induction of gene expression and attendant protein expression 12 hr before the intended harvest. Harvest eight seedlings from each sample into a 1.5-ml microcentrifuge tube. Freeze samples in liquid nitrogen immediately and store at −80°C until use.
Protein sample preparation from transgenic A. thaliana seedlings
3.Grind frozen samples in each microcentrifuge tube using a clean, pre-cooled micropestle to mechanically homogenize the plant tissue until it becomes a fine powder (Fig. 1).
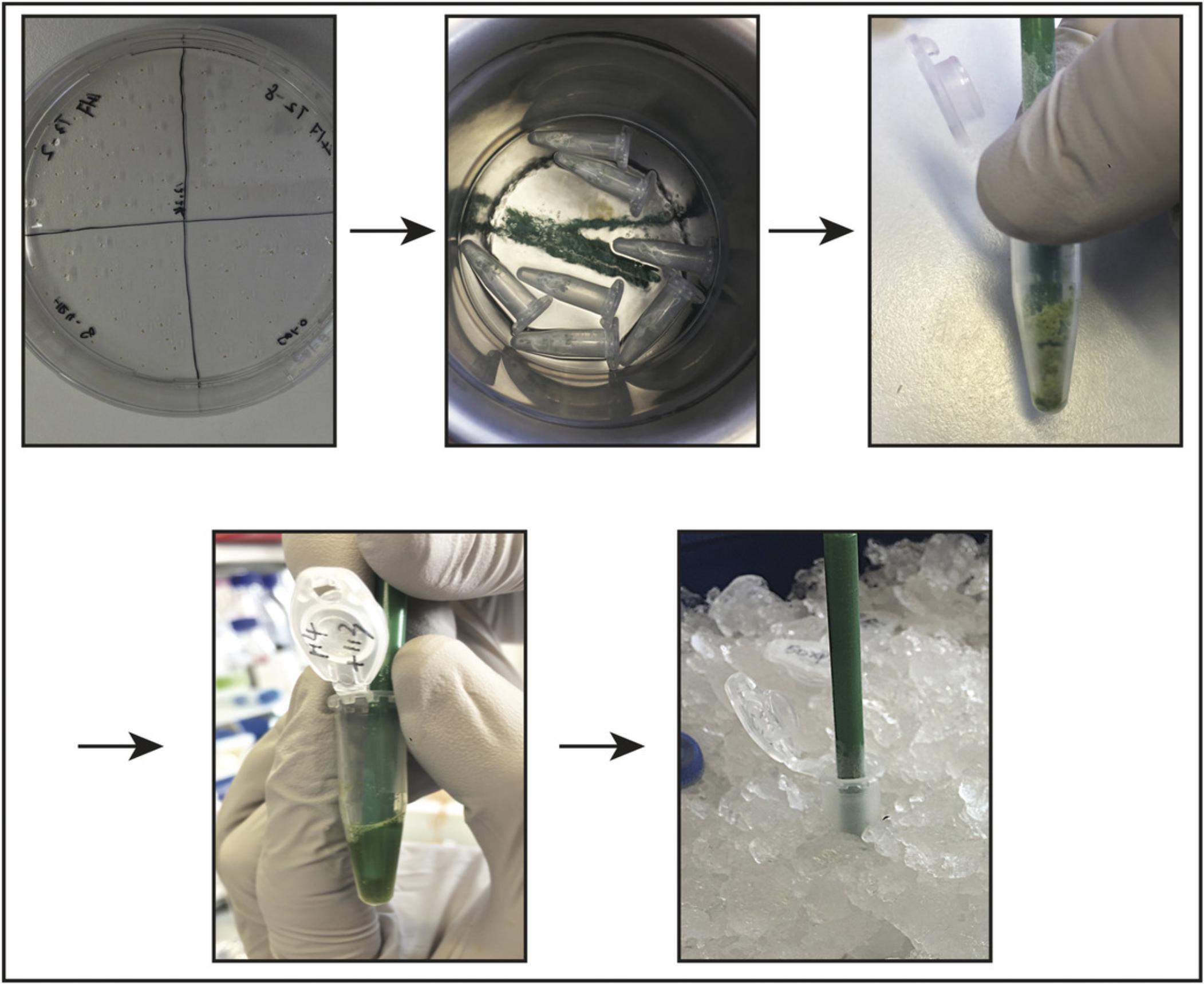
4.Add 100 µl cold native protein extraction buffer to each sample.
5.Incubate samples on ice for 10 min to allow the detergent to increase protein solubilization.
6.Centrifuge samples for 15 min at 20,000 × g , 4°C. Transfer supernatant into a new 1.5-ml microcentrifuge tube.
7.Centrifuge samples again for 15 min at 20,000 × g , 4°C. Transfer supernatant into a new 1.5-ml microcentrifuge tube.
8.Add 0.25% NativePAGE™ G-250 Sample Additive to samples, which contain 1% DDM detergent (see step 4) (Schägger, 2001). Mix immediately by pipetting up and down and then immediately load samples onto a BN-PAGE gel (see step 13).
BN-PAGE
9.Combine 50 ml of 20× NativePAGE™ Running Buffer and 950 ml ddH2O to prepare 1 L of 1× NativePAGE™ Anode Buffer. Mix well.
10.Combine 190 ml buffer from step 9 and 10 ml of 20× NativePAGE™ Cathode Additive to prepare 200 ml NativePAGE™ Dark Blue Cathode Buffer. Mix well.
11.Use 199 ml buffer from step 9 and 1 ml of 20× NativePAGE™ Cathode Additive to prepare 200 ml NativePAGE™ Light Blue Cathode Buffer. Mix well.
12.Rinse wells of a NativePAGE™ 3-12% Bis-Tris Protein Gel with 1 ml NativePAGE™ Dark Blue Cathode Buffer (see step 10) twice. Fill wells with NativePAGE™ Dark Blue Cathode Buffer. Place gel in an XCell SureLock™ Mini-Cell (Fig. 2A, first and second panels).
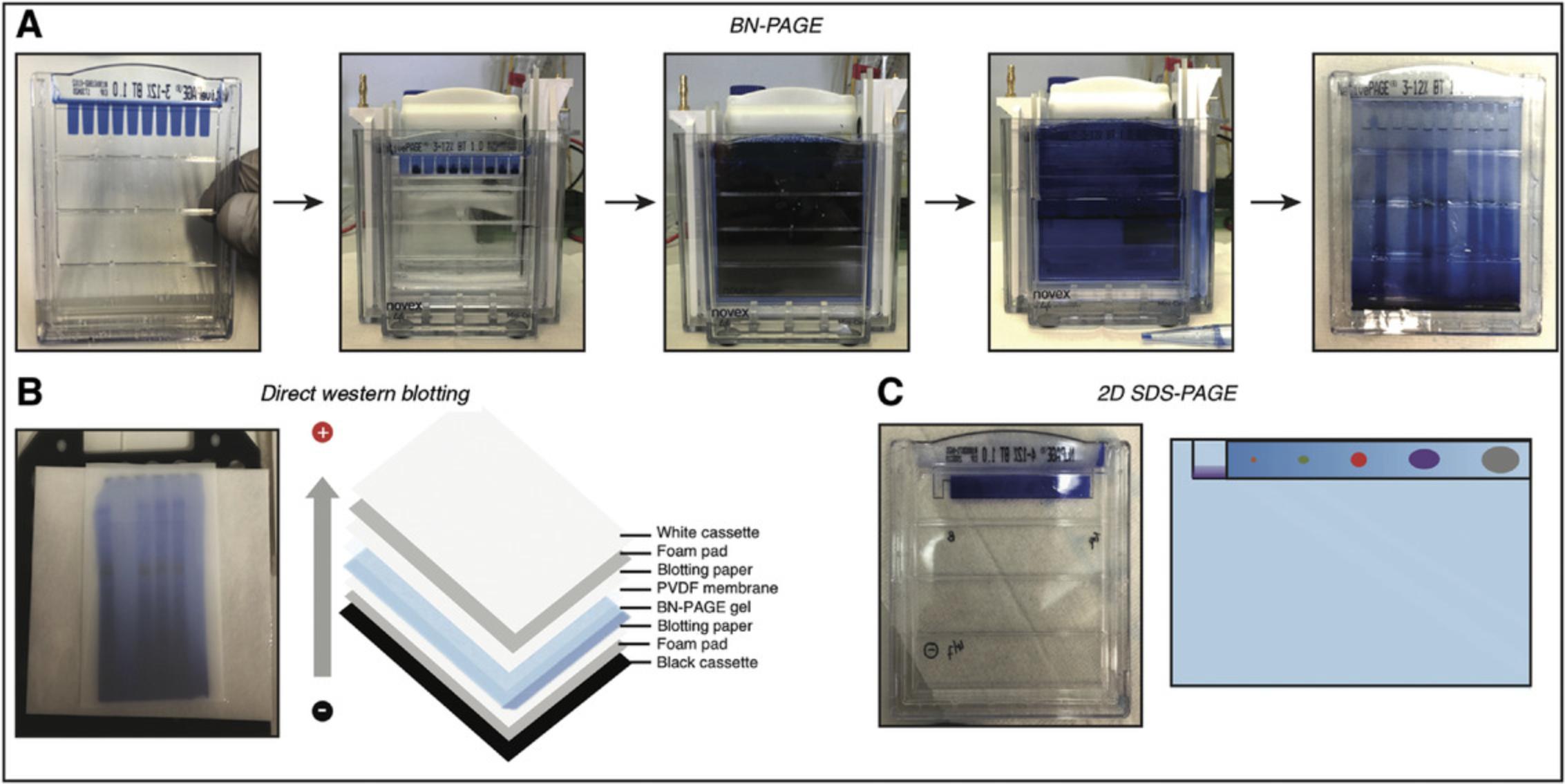
13.Load 10 µl of each sample (see step 8) into each well of the gel using gel-load filter tips. Add 4 µl NativeMark™ Unstained Protein Standard to an empty well.
14.Use ∼600 ml of 1× NativePAGE™ Anode Buffer (see step 9) to fill outer buffer chamber and use 200 ml NativePAGE™ Dark Blue Cathode Buffer (see step 10) to fill inner buffer chamber.
15.To separate native proteins, using an electrophoresis power supply, run gel at 120 V for ∼30 min, or until the dye front reaches one-third the length of the gel. Then, replace NativePAGE™ Dark Blue Cathode Buffer with NativePAGE™ Light Blue Cathode Buffer (see step 11; Fig. 2A, fourth panel).
16.Continue running gel at 120 V for ∼2 hr to achieve separation of proteins.
17.Carefully open gel cassette using a gel knife. To visualize the unstained marker, cut marker lane out as a strip and stain with 30 ml Coomassie Brilliant Blue R-250 stain solution.
18.Remove stain solution and gently shake with 50 ml Coomassie Brilliant Blue R-250 destain solution on a platform shaker until background becomes transparent.
19.For the sample lanes, perform direct western blotting (see steps 20a to 34a) or cut each lane out as a strip and use each strip in perpendicular orientation for 2D SDS-PAGE (see steps 20b to 27b).
Direct western blotting after BN-PAGE
20a. Prepare 1 L of 1× transfer buffer by dilution of 20× NuPAGE™ Transfer Buffer (50 ml of 20× NuPAGE™ Transfer Buffer in 950 ml ddH2O) and cool buffer to 4°C.
21a. Submerge Immun-Blot® PVDF membrane in methanol for 30 s to activate the membrane.
22a. Place materials for assembling the western blotting cassette (gel holder cassette and two foam pads from Mini Trans-Blot Electrophoretic Transfer Cell, two blotting papers, activated PVDF membrane, and gel from step 19) in 1× transfer buffer (Fig. 2B).
23a. Assemble cassette, avoiding air bubbles between the gel and the PVDF membrane. Insert cassette into the Mini Trans-Blot Electrophoretic Transfer Cell.
24a. Perform blotting at 110 V for 2 hr at 4°C.
25a. Prepare blocking solution by completely dissolving 2.5 g powdered milk in 50 ml PBST using magnetic stirring and store at 4°C.
26a. Disassemble cassette after the transfer step and incubate PVDF membrane in 20 ml of 8% acetic acid for 10 min on a platform shaker with gentle shaking to fix proteins.
27a. Wash PVDF membrane in a 6 × 10–cm container for 5 min using 10 ml PBST.
28a. Discard PBST and incubate PVDF membrane in 10 ml blocking solution (see step 25a) for 1 hr at room temperature or overnight at 4°C on a platform shaker with gentle shaking.
29a. Discard blocking solution and wash PVDF membrane again for 5 s using 10 ml PBST.
30a. Discard PBST and incubate PVDF membrane with a 1:5000 dilution of anti-FLAG antibody (2 µl in 10 ml blocking solution) for 1 hr at room temperature on a platform shaker with gentle shaking.
31a. Discard primary antibody solution and wash PVDF membrane for 5 min using 20 ml PBST on a platform shaker with gentle shaking. Repeat wash three times in total.
32a. Incubate PVDF membrane with a 1:8000 dilution of anti-mouse IgG–peroxidase antibody (1.25 µl in 10 ml blocking solution) for 45 min at room temperature on a platform shaker with gentle shaking.
33a. Discard secondary antibody solution and wash PVDF membrane for 5 min using 20 ml PBST on a platform shaker with gentle shaking. Repeat wash three times in total.
34a. Develop film using western blotting detection reagent and a film processor.
2D SDS-PAGE after BN-PAGE
20b. Prepare 1 L of 1× SDS running buffer by dilution of 20× NuPAGE™ MOPS SDS Running Buffer (50 ml of 20× NuPAGE™ MOPS SDS Running Buffer in 950 ml ddH2O) and store at room temperature.
21b. Prepare 1× protein loading buffer by dilution of 4× protein loading buffer and addition of 100 mM dithiothreitol (DTT). Incubate gel strips from Basic Protocol, step 19, in 10 ml of 1× protein loading buffer each for 10 min on a platform shaker with gentle shaking.
22b. Use a microwave to heat gel strips submerged in 1× protein loading buffer at maximum power for 20 s to denature proteins. Then, incubate heated gel strips in the heated buffer for 10 min on a platform shaker with gentle shaking at room temperature.
23b. After rinsing once with 1× SDS running buffer (see step 20b), fill 2D well of a NuPAGE™ 4-12% Bis-Tris Protein Gel with 1× SDS running buffer.
24b. Carefully insert a gel strip into empty 2D (wide) well to avoid trapping of air bubbles between the gel strip and the 2D SDS-PAGE gel. Load 5 µl PageRuler™ Prestained Protein Ladder into marker (narrow) well (Fig. 2C).
25b. Add 100 μl of 1× protein loading buffer on top of gel strip. Place gel in the XCell SureLock™ Mini-Cell and fill instrument with 1× SDS running buffer.
26b. Run gel at 120 V for ∼2 hr to separate the denatured proteins by size.
27b. Perform immunoblotting using a 1:5000 dilution of anti-FLAG antibody and a 1:3000 dilution of anti-HA antibody following previously described instructions (see Current Protocols article; Ni et al., 2016).
REAGENTS AND SOLUTIONS
½ MS medium plates
- 0.5× Murashige & Skoog medium including vitamins (Duchefa Biochemie, cat. no. M0255)
- 15 g/L sucrose
- Adjust pH to 5.7 with KOH
- 3 g/L Phytagel (Sigma, cat. no. P8169)
- Autoclave at 121°C for 15 min
- Cool to ∼60°C and pour into plates
- Store ≤6 months at 4°C
Coomassie Brilliant Blue R-250 destain solution
- 5% (v/v) ethanol
- 10% (v/v) acetic acid
- Store ≤1 year at room temperature
Coomassie Brilliant Blue R-250 stain solution
- 0.1% (w/v) Coomassie Brilliant Blue R-250
- 25% (v/v) isopropanol
- 10% (v/v) acetic acid
- Store ≤1 year at room temperature
Native protein extraction buffer
- 1× NativePAGE™ Sample Buffer (Thermo Fisher Scientific, cat. no. BN2008)
- 1× protease inhibitors (Roche, cat. no. 5056489001)
- 1% (w/v) n-dodecyl-β-D-maltoside (DDM; Thermo Fisher Scientific, cat. no. BN2005)
- Prepare fresh immediately before use
Phosphate-buffered saline with Tween 20 (PBST)
- 1.8 mM KH2PO4
- 10 mM Na2HPO4
- 2.7 mM KCl
- 137 mM NaCl
- Adjust pH to 7.2 with 1 M NaOH
- Sterilize by autoclaving
- Store ≤6 months at room temperature
- Add 0.1% (v/v) Tween 20 immediately before use
Protein loading buffer, 4×
- 200 mM Tris·HCl, pH 6.8
- 8% (w/v) SDS
- 40% (v/v) glycerol
- 0.032% (w/v) bromophenol blue
- Store ≤1 year at room temperature
- Dilute to 1× and add 100 mM DTT before use
COMMENTARY
Background Information
Proteins can exist in complexes, forming an in vivo protein-protein interaction network that may be involved in a range of cellular processes. Protein activities occasionally can be regulated by dynamic homo- or hetero-oligomerization. Therefore, it is of importance to investigate protein oligomerization to understand how protein complexes change during physiological responses.
Gel filtration is a robust technique to separate proteins according to molecular size. However, this method can be tedious and requires considerable experience and skill. A large quantity of samples is needed, and specialized equipment must be available. These obstacles make gel filtration less practical for studying in vivo protein oligomerization (Gell et al., 2012; see Current Protocols article; Irvine, 2001), particularly for proteins with low abundances, such as plant NLR proteins. BN-PAGE was first developed to analyze mitochondrial membrane protein complexes (Schägger & von Jagow, 1991). It has been extensively applied to study protein function because of its advantages, including procedures that can be easily learned; simple and readily available protein gel electrophoresis equipment; and the ability to investigate protein in-gel activity, native molecular weight, and oligomeric states (Eubel, Braun, & Millar, 2005; Schägger, 2001; Schägger, Cramer, & von Jagow, 1994). Thus, BN-PAGE protocols have been adapted and optimized for samples from animal and microbial cells (Fiala et al., 2011; Wittig et al., 2006). Because idiosyncrasies of plant samples, such as the high abundance of chloroplast proteins, may affect immunodetection after BN-PAGE, the current protocol was optimized for detection of oligomerization of low-abundance NLR proteins in plants, but it is not limited to NLR proteins.
Critical Parameters and Troubleshooting
Seedling conditions
Seedlings for native protein extraction should not be older than 10 days, unless the protein of interest cannot be expressed at this stage. Older plants will typically produce more chloroplast-related proteins, which may interfere with downstream analyses.
Native protein samples
In principle, native protein samples can be kept at –80°C for longer-term storage; however, we recommend using fresh samples to avoid degrading the protein or destroying its structure. In addition, it is important to keep your samples cold during extraction; otherwise, protein complexes may be destabilized. The protein samples should be loaded into the gel wells before filling the inner chamber with Dark Blue Cathode Buffer. The gel wells can be filled with up to 25 μl, but we recommend never to load that much; a smaller sample volume (∼10 μl) is effective and gives more consistent results.
Western blotting after BN-PAGE
We suggest using the wet transfer method to transfer proteins from the BN-PAGE gel to the PVDF membrane. Methanol is used to activate the PVDF membrane but is not contained in the transfer buffer to preserve proteins in their native states.
Antibodies for immunodetection
Antibodies against either native protein epitopes or fused tags can be used for immunodetection. However, as the proteins are in their native state, some epitopes are hidden inside the folded proteins, thus blocking antibody recognition. Furthermore, it is common to detect some nonspecific proteins in their native state. Therefore, we recommend using antibodies against a flexible tag, such as the FLAG tag, and to always include a control sample (e.g., from a non-transgenic or mutant plant) to help identify the nonspecific bands. Additionally, using different fused tags at different positions in a protein and/or 2D SDS-PAGE can help to troubleshoot failures of immunodetection.
Antibody incubation time
A longer incubation with the primary antibody enhances detection sensitivity, but it also increases the risk of background noise. For most cases, 1 hr of incubation with the primary antibody and 30 to 60 min of incubation with the secondary antibody incubation are sufficient. Weak signals can be compensated for through longer film exposure time or stronger detection reagent (ECL™ Select Western Blotting Detection Reagent).
Understanding Results
Following the protocol described here, dynamic protein oligomerization in plants can be detected by BN-PAGE followed by direct western blotting. We use oligomerization of the plant immune protein RPP7 triggered by the immune protein HR4 as an example. We generated transgenic plants with RPP7 fused to a 3× FLAG tag and driven by an RPP7 native promoter and with HR4 fused to a 3× HA tag and driven by an estradiol-inducible promoter (Li et al., 2020). RPP7 oligomerization could be observed in samples after 12 hr of induction with estradiol (Fig. 3A). Because RPP7 protein has a very low expression level, longer western blotting film exposure time results in strong nonspecific bands, even in the control (non-transgenic) plants (Fig. 3A). However, nonspecific bands are not detected when we investigate oligomerization of the high-abundance HR4 protein, in spite of its presence in complexes of various sizes (Fig. 3B). In addition, the lower expression level of RPP7 makes it largely impossible to perform silver staining and mass spectroscopy after BN-PAGE. For cases with higher protein expression levels, mass spectroscopy can be incorporated into the Basic Protocol to identify the interaction partners in native protein complexes (Elmore & Coaker, 2011). In sum, this protocol can be used to investigate protein oligomerization in a relatively short time.
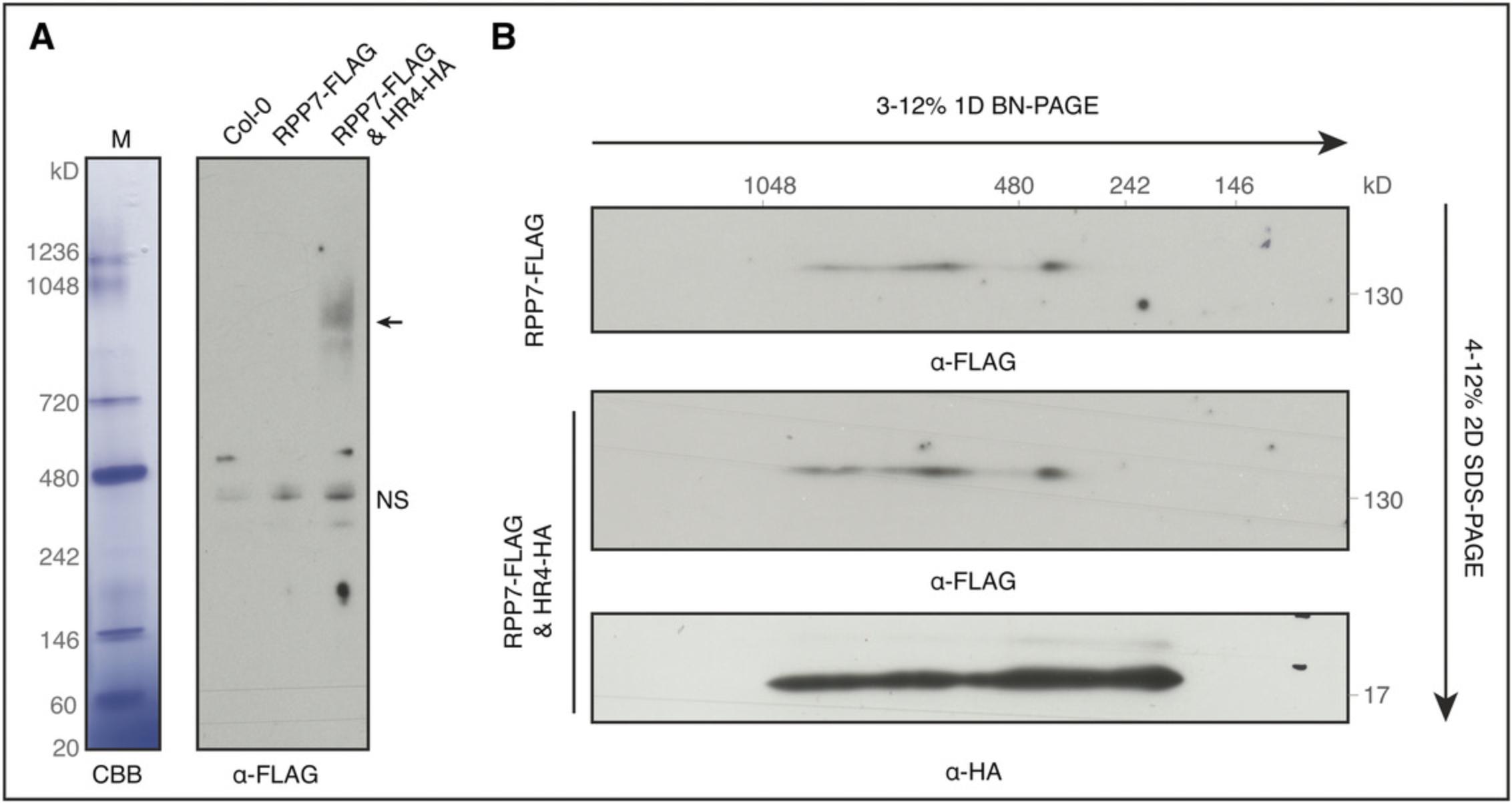
Time Considerations
This protocol can be finished within 12 days after germination of A. thaliana seeds. Seedlings and native protein extracts can be frozen in liquid nitrogen and stored at −80°C for longer-term storage. We normally complete the experiment within 1 to 2 days after sample collection. BN-PAGE can be started in the morning, and the blocking step for western blotting can be performed overnight.
Acknowledgments
This work was supported by an Human Frontier Science Program (HFSP) Long-Term Fellowship (LT000314/2017-L) to L.L. and by Collaborative Research Center 1101 (DFG/SFB1101) and funding from the Max Planck Society to D.W.
Literature Cited
- Crichton, P. G., Harding, M., Ruprecht, J. J., Lee, Y., & Kunji, E. R. S. (2013). Lipid, detergent, and Coomassie Blue G-250 affect the migration of small membrane proteins in blue native gels: Mitochondrial carriers migrate as monomers not dimers. The Journal of Biological Chemistry , 288, 22163–22173. doi: 10.1074/jbc.M113.484329.
- Elmore, J. M., & Coaker, G. (2011). Biochemical purification of native immune protein complexes. Methods in Molecular Biology , 712, 31–44. doi: 10.1007/978-1-61737-998-7_4.
- Eubel, H., Braun, H.-P., & Millar, A. H. (2005). Blue-native PAGE in plants: A tool in analysis of protein-protein interactions. Plant Methods , 1, 11. doi: 10.1186/1746-4811-1-11.
- Fiala, G. J., Schamel, W. W. A., & Blumenthal, B. (2011). Blue native polyacrylamide gel electrophoresis (BN-PAGE) for analysis of multiprotein complexes from cellular lysates. Journal of Visualized Experiments: JoVE , (48), 2164. doi: 10.3791/2164.
- Gell, D. A., Grant, R. P., & Mackay, J. P. (2012). The detection and quantitation of protein oligomerization. In J. M. Matthews (Ed.), Protein Dimerization and Oligomerization in Biology (pp. 19–41). New York, NY: Springer. doi: 10.1007/978-1-4614-3229-6_2.
- Irvine, G. B. (2001). Determination of molecular size by size-exclusion chromatography (gel filtration). Current Protocols in Cell Biology , 6(1), 5.5.1–5.5.16. doi: 10.1002/0471143030.cb0505s06.
- Li, L., Habring, A., Wang, K., & Weigel, D. (2020). Atypical resistance protein RPW8/HR triggers oligomerization of the NLR immune receptor RPP7 and autoimmunity. Cell Host and Microbe , 27(3), 405–417.e6. doi: 10.1016/j.chom.2020.01.012.
- Ni, D., Xu, P., Sabanayagam, D., & Gallagher, S. R. (2016). Protein blotting: Immunoblotting. Current Protocols Essential Laboratory Techniques , 12(1), 8.3.1–8.3.40. doi: 10.1002/9780470089941.et0803s12.
- Schägger, H. (2001). Blue-native gels to isolate protein complexes from mitochondria. Methods in Cell Biology , 65, 231–244. doi: 10.1016/s0091-679x(01)65014-3.
- Schägger, H., Cramer, W. A., & von Jagow, G. (1994). Analysis of molecular masses and oligomeric states of protein complexes by blue native electrophoresis and isolation of membrane protein complexes by two-dimensional native electrophoresis. Analytical Biochemistry , 217, 220–230. doi: 10.1006/abio.1994.1112.
- Schägger, H., & von Jagow, G. (1991). Blue native electrophoresis for isolation of membrane protein complexes in enzymatically active form. Analytical Biochemistry , 199, 223–231. doi: 10.1016/0003-2697(91)90094-a.
- Wittig, I., Braun, H.-P., & Schägger, H. (2006). Blue native PAGE. Nature Protocols , 1, 418–428. doi: 10.1038/nprot.2006.62.
- Zuo, J., Niu, Q. W., & Chua, N. H. (2000). Technical advance: An estrogen receptor-based transactivator XVE mediates highly inducible gene expression in transgenic plants. The Plant Journal , 24(2), 265–273. doi: 10.1046/j.1365-313x.2000.00868.x.
Citing Literature
Number of times cited according to CrossRef: 14
- Guo-Bang Li, Jie Liu, Jia-Xue He, Gao-Meng Li, Ya-Dan Zhao, Xiao-Ling Liu, Xiao-Hong Hu, Xin Zhang, Jin-Long Wu, Shuai Shen, Xin-Xian Liu, Yong Zhu, Feng He, Han Gao, He Wang, Jing-Hao Zhao, Yan Li, Fu Huang, Yan-Yan Huang, Zhi-Xue Zhao, Ji-Wei Zhang, Shi-Xin Zhou, Yun-Peng Ji, Mei Pu, Min He, Xuewei Chen, Jing Wang, Weitao Li, Xian-Jun Wu, Yuese Ning, Wenxian Sun, Zheng-Jun Xu, Wen-Ming Wang, Jing Fan, Rice false smut virulence protein subverts host chitin perception and signaling at lemma and palea for floral infection, The Plant Cell, 10.1093/plcell/koae027, 36 , 5, (2000-2020), (2024).
- Jawad Ali, Fayaz Asad, Muhammad Irshad, Tabassum Yaseen, Imtiaz Ahamd, undefined Ambrin, Screening of selected wheat cultivars for leaf rust resistance genes, Journal of Crop Improvement, 10.1080/15427528.2024.2340505, 38 , 4, (317-328), (2024).
- Hilda Delgado de la Herran, Denis Vecellio Reane, Yiming Cheng, Máté Katona, Fabian Hosp, Elisa Greotti, Jennifer Wettmarshausen, Maria Patron, Hermine Mohr, Natalia Prudente de Mello, Margarita Chudenkova, Matteo Gorza, Safal Walia, Michael Sheng-Fu Feng, Anja Leimpek, Dirk Mielenz, Natalia S Pellegata, Thomas Langer, György Hajnóczky, Matthias Mann, Marta Murgia, Fabiana Perocchi, Systematic mapping of mitochondrial calcium uniporter channel (MCUC)-mediated calcium signaling networks, The EMBO Journal, 10.1038/s44318-024-00219-w, (2024).
- Furong Liu, Zhenlin Yang, Chao Wang, Zhang You, Raoul Martin, Wenjie Qiao, Jian Huang, Pierre Jacob, Jeffery L. Dangl, Jan E. Carette, Sheng Luan, Eva Nogales, Brian J. Staskawicz, Activation of the helper NRC4 immune receptor forms a hexameric resistosome, Cell, 10.1016/j.cell.2024.07.013, 187 , 18, (4877-4889.e15), (2024).
- Qingqing Wei, Baoyin Chen, Junjun Wang, Manna Huang, Yuanye Gui, Aqib Sayyed, Bao-Cai Tan, PHB3 Is Required for the Assembly and Activity of Mitochondrial ATP Synthase in Arabidopsis, International Journal of Molecular Sciences, 10.3390/ijms24108787, 24 , 10, (8787), (2023).
- Hee‐Kyung Ahn, Xiao Lin, Andrea Carolina Olave‐Achury, Lida Derevnina, Mauricio P Contreras, Jiorgos Kourelis, Chih‐Hang Wu, Sophien Kamoun, Jonathan D G Jones, Effector‐dependent activation and oligomerization of plant NRC class helper NLRs by sensor NLR immune receptors Rpi‐amr3 and Rpi‐amr1, The EMBO Journal, 10.15252/embj.2022111484, 42 , 5, (2023).
- Xiaohua Hu, Xiaoping Wu, Kalen Berry, Chuntao Zhao, Dazhuan Xin, Sean Ogurek, Xuezhao Liu, Liguo Zhang, Zaili Luo, Masahide Sakabe, Joanna Trubicka, Maria Łastowska, Frank Szulzewsky, Eric C. Holland, Lindsay Lee, Ming Hu, Mei Xin, Q. Richard Lu, Nuclear condensates of YAP fusion proteins alter transcription to drive ependymoma tumourigenesis, Nature Cell Biology, 10.1038/s41556-022-01069-6, (2023).
- Ming-Yu Wang, Jun-Bin Chen, Rui Wu, Hai-Long Guo, Yan Chen, Zhen-Ju Li, Lu-Yang Wei, Chuang Liu, Sheng-Feng He, Mei-Da Du, Ya-long Guo, You-Liang Peng, Jonathan D.G. Jones, Detlef Weigel, Jian-Hua Huang, Wang-Sheng Zhu, The plant immune receptor SNC1 monitors helper NLRs targeted by a bacterial effector, Cell Host & Microbe, 10.1016/j.chom.2023.10.006, 31 , 11, (1792-1803.e7), (2023).
- Mariam Tahoun, Marianne Engeser, Vigneshwaran Namasivayam, Paul Martin Sander, Christa E. Müller, Chemistry and Analysis of Organic Compounds in Dinosaurs, Biology, 10.3390/biology11050670, 11 , 5, (670), (2022).
- Tapan Kumar Mohanta, Muhammad Shahzad Kamran, Muhammad Omar, Waheed Anwar, Gyu Sang Choi, PlantMWpIDB: a database for the molecular weight and isoelectric points of the plant proteomes, Scientific Reports, 10.1038/s41598-022-11077-z, 12 , 1, (2022).
- Zhuanzhuan Liu, Shihong Xu, Zhiwei Zhang, Hanying Wang, Qiyue Jing, Shenghan Zhang, Mengnan Liu, Jinzhi Han, Yanbo Kou, Yanxia Wei, Lu Wang, Yugang Wang, FAM96A is essential for maintaining organismal energy balance and adipose tissue homeostasis in mice, Free Radical Biology and Medicine, 10.1016/j.freeradbiomed.2022.09.011, 192 , (115-129), (2022).
- Purna Bahadur Chetri, Heena Khan, Timir Tripathi, Methods to determine the oligomeric structure of proteins, Advances in Protein Molecular and Structural Biology Methods, 10.1016/B978-0-323-90264-9.00005-2, (49-76), (2022).
- Javed Ahmad, Arlene Asthana Ali, Muhammed Iqbal, Abrar Ahmad, M. Irfan Qureshi, Proteomics of mercury-induced responses and resilience in plants: a review, Environmental Chemistry Letters, 10.1007/s10311-022-01388-y, 20 , 5, (3335-3355), (2022).
- Shannon L. Thomas, Jonathan B. Thacker, Kevin A. Schug, Katarína Maráková, Sample preparation and fractionation techniques for intact proteins for mass spectrometric analysis, Journal of Separation Science, 10.1002/jssc.202000936, (2020).