Bacterial Pathogenesis: Assessment of Intracellular Positioning of Pathogen-Containing Vacuoles During Infection
Farah Nasser, Farah Nasser, Mosopefoluwa T. Oke, Mosopefoluwa T. Oke, Sara Knezevic, Sara Knezevic, Vanessa M. D'Costa, Vanessa M. D'Costa
bacterial pathogenesis
host-pathogen interactions
intracellular pathogen
intracellular trafficking
Salmonella enterica
Abstract
Intracellular bacterial pathogens implement a diverse array of strategies to target host cells and establish infection. For vacuolar pathogens, the process of pathogen-containing vacuole movement within host cells, termed intracellular trafficking, is central to both pathogen survival and infection progression. Typically a process mediated by secreted virulence factors that manipulate the host cytoskeletal machinery, internalized pathogen-containing vacuoles traffic to the site of replication to establish a unique replicative niche, and if applicable, traffic back toward the host cell periphery for cell-to-cell spread. As such, the intracellular positioning of pathogen-containing vacuoles represents a fundamental measure of infection progression. Here, we describe a fluorescence microscopy-based method to quantitatively assess bacterial intracellular positioning, using Salmonella enterica serovar Typhimurium infection of epithelial cells as a model. This experimental approach can be modified to study infection in diverse host cell types, and with a broad array of pathogens. The system can also be adapted to examine the kinetics of infection, identify secreted virulence factors that mediate host trafficking, investigate host factors that are targeted by the pathogen for trafficking, and assess functional domains within a virulence factor responsible for mediating the phenotype. Collectively, these tools can provide fundamental insight into the pathogenesis of a diverse array of intracellular bacterial pathogens, and new host factors that are hijacked to mediate infection. © 2024 The Authors. Current Protocols published by Wiley Periodicals LLC.
Basic Protocol 1 : Culture and preparation of host cells
Alternate Protocol : Culture and preparation of host cells to assess host factor contribution to bacterial positioning
Basic Protocol 2 : Infection of epithelial cells with S. Typhimurium
Basic Protocol 3 : Fluorescence staining for analysis of bacterial positioning
Basic Protocol 4 : Fluorescence microscopy analysis of bacterial positioning
INTRODUCTION
Intracellular bacterial pathogens have evolved diverse molecular strategies to manipulate host cells to establish a replicative niche (Bhavsar et al., 2007). Often with the use of secreted virulence factors, pathogens can target and evade immune signaling pathways (Bhavsar et al., 2007; Diacovich & Gorvel, 2010) or cellular pathways that function in host response to infection (Jiao & Sun, 2019; Newton & Roy, 2011; Matsuda et al., 2012; Tuli & Sharma, 2019). Similarly, virulence factors can also be used to hijack other host cellular functions for the benefit of the pathogen, such as the host cytoskeletal machinery (Bhavsar et al., 2007; Brumell & Scidmore, 2007; Stevens et al., 2006) or organelle function (Kellermann et al., 2021; Lobet et al., 2015). The host cytoskeleton represents an important target for bacterial pathogens (Brumell & Scidmore, 2007; Stevens et al., 2006). Among the broad array of strategies implemented, bacterial pathogens can modulate the host cytoskeleton to induce bacterial uptake into host cells, direct their intracellular movement inside host cells, and recruit membrane or vesicle cargo to the site of the pathogen (Brumell & Scidmore, 2007; Stevens et al., 2006). Vacuolar pathogens are especially adept at manipulating the host cytoskeleton. Upon internalization into host cells, vacuolar pathogens reside in membrane-bound compartments termed pathogen-containing vacuoles (PCVs), where each pathogen establishes a unique replicative niche (Brumell & Scidmore, 2007; Henry et al., 2005). In order to accomplish this, pathogens must direct the movement of PCVs within host cells to the site of replication, which often occurs in the perinuclear region, by a process termed PCV trafficking (Brumell & Scidmore, 2007; Henry et al., 2005). Intracellular PCV trafficking is typically a pathogen-mediated process and occurs along the host cytoskeleton (Henry et al., 2005). For example, the pathogen Salmonella enterica uses a series of secreted virulence factors that collectively manipulate host microtubule motor proteins to direct the trafficking of Salmonella -containing vacuoles (SCVs) (Jennings et al., 2017; Szeto et al., 2009). Virulence factors mediate SCV trafficking to the perinuclear region, subsequent anchoring of SCVs to the Golgi, and during the later stages of infection, retrograde migration of SCVs toward the cell periphery (Jennings et al., 2017; Szeto et al., 2009).
Given the importance of PCV trafficking to the infection life cycle, the assessment of intracellular bacterial positioning within host cells represents an instrumental tool for pathogenesis researchers. Studies of bacterial intracellular positioning can provide insight into the kinetics of infection and cell-to-cell spread, as well as dissecting both virulence factors important for infection and host factors that contribute to bacterial trafficking (D'Costa et al., 2019; Szeto et al., 2009). If a virulence factor implicated in PCV trafficking is identified, positioning assays can also be used in domain mapping studies or studies with point mutants to assess the contribution of functional domains within the virulence factor (D'Costa et al., 2019).
The following outlines a method to quantitatively assess intracellular bacterial positioning in host cells, using Salmonella enterica serovar Typhimurium infection of epithelial cells as a model (Fig. 1). S. Typhimurium has been shown to use a series of secreted virulence factors to manipulate host trafficking for infection progression using this fluorescence microscopy-based assay (Fig. 2) (D'Costa et al., 2019; Szeto et al., 2009). Protocols discussed include the culturing and preparation of host cells, infection of host cells, fluorescence staining for analysis of intracellular positioning, and fluorescence microscopy-based analysis. This method was used to assess functional domains within a virulence factor of interest (D'Costa et al., 2019), and to establish the involvement of a newly identified host protein target in the phenotype (D'Costa et al., 2019). The methods described can be adapted for the study of diverse pathogens in a broad array of host cell types and highlight important considerations for adapting this assay to the model system of interest.
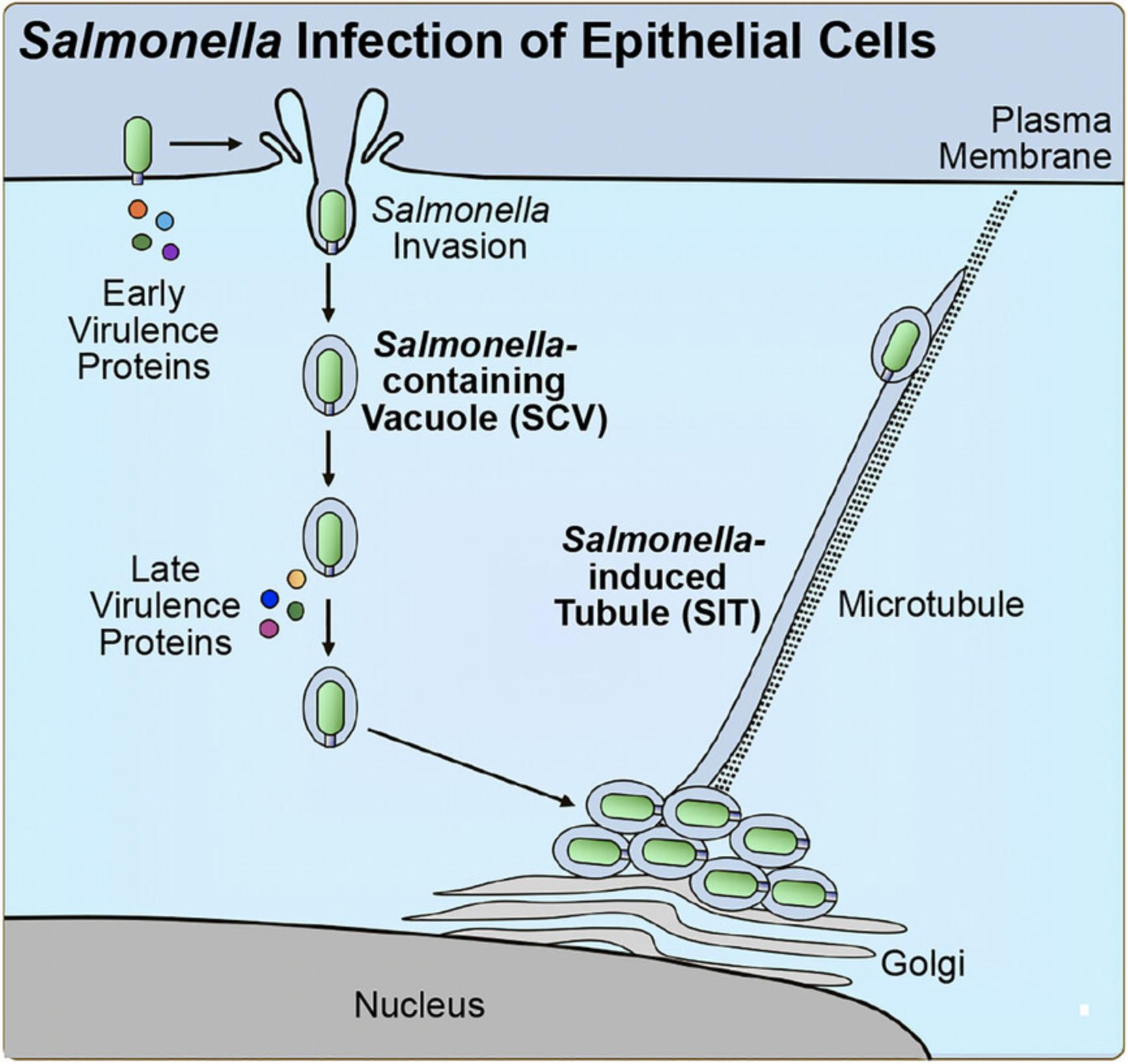
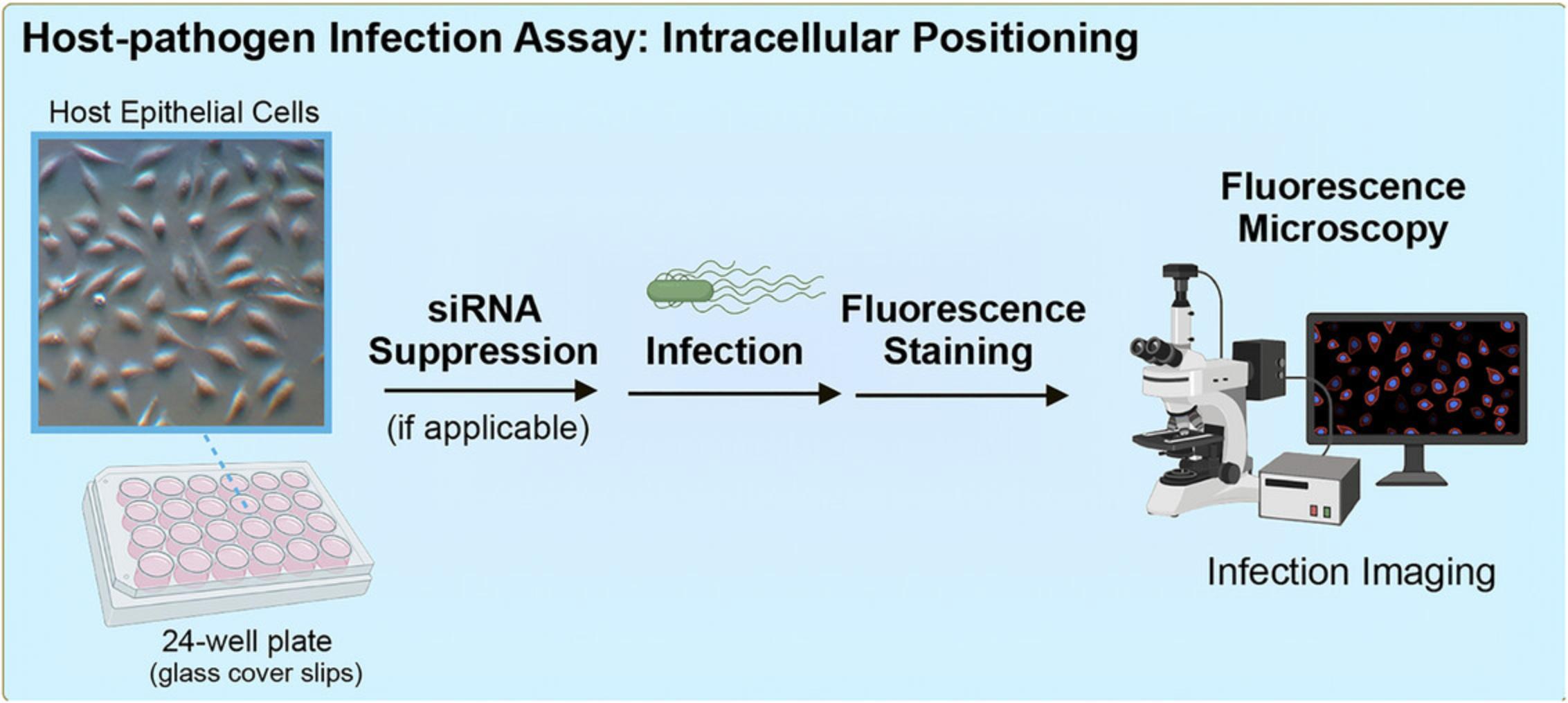
STRATEGIC PLANNING
Bacteria of Interest
This assay can be adapted to study any intracellular vacuolar pathogen with an established tissue culture-based model system for infection. As this assay is fluorescence microscopy-based, methods for detection of each component of importance must be established. From the perspective of the pathogen, a method for microbe visualization must be in place. An antibody-based approach suitable for immunofluorescence microscopy is a common strategy for detection, as described in this method. Alternatively, fluorescent protein-expressing shuttle plasmids or a strain with a chromosomally integrated fluorescent protein encoding gene can be used. Additional information regarding pathogen detection can be found in Basic Protocol 2.
Host Cell of Interest
This assay is adaptable to any host cell type permissive to intracellular replication of the vacuolar pathogen of interest, given that infection conditions have been established. Should this assay be of interest for a poorly understood pathogen, it is recommended that optimal infection conditions be established first. Parameters that are useful to consider in infection model optimization are host cell culture conditions, host cell density at the time of infection, and pathogen growth conditions for inoculum preparation. With respect to pathogen growth conditions, this factor is especially important in situations where the secretion of virulence factors is dependent on culture conditions or growth phase.
As this assay is aimed to study vacuolar pathogens, it is essential to have established a host marker protein on the pathogen-containing vacuole membrane that can be used for fluorescence microscopy detection at the timepoint(s) of interest. Antibodies directed against a host marker protein is a common approach. Alternatively, a more general stain, such as fluorophore-conjugated wheat germ agglutinin, might also be used if needed.
CAUTION : The host cell type (HeLa) and pathogen (Salmonella enterica serovar Typhimurium) used in these methods are classified as Biosafety Level 2 (BSL-2). Follow all appropriate regulations and guidelines for the handling of biohazardous eukaryotic cells and bacteria.
Basic Protocol 1: CULTURE AND PREPARATION OF HOST CELLS
This protocol uses the epithelial cell line HeLa, an established model for the study of Salmonella infection (Castanheira & Garcia-Del Portillo, 2017; Giannella et al., 1973), and describes the culturing of host cells in preparation for infection. This method can be modified to investigate diverse intracellular pathogens and host cell types. Included within the protocol are important considerations for adapting this assay to the model system of interest. The assay can also be adapted to a series of different experimental applications, including bacterial positioning kinetics, virulence factor identification, virulence factor domain mapping, and contribution of a host factor. For additional information regarding experiment-specific considerations, see Table 1.
Assay purpose | Bacterial strains required | Additional information and considerations |
---|---|---|
Bacterial positioning kinetics | Wildtype | Host cells: one well needed per timepoint of interest |
Kinetics: kinetics of intracellular positioning may vary by strain and host cell line, and therefore should be determined experimentally if not previously established | ||
Fixation: fixation with paraformaldehyde in a tissue culture plate can impair growth in adjacent wells; for kinetic studies within the same plate, seal wells undergoing fixation with tape | ||
Virulence factor identification | Wildtype, isogenic knockout(s), complementation strain(s) | Complementation: all strains should harbor the complementation plasmid, with or without the gene encoding the virulence factor of interest |
Assay can be scaled down to a smaller experimental scale if needed to perform a screen of knockout strains | ||
Virulence factor domain mapping | Wildtype, isogenic knockout, complementation strains harboring wildtype and truncation mutants | Complementation: all strains should harbor the complementation plasmid, with or without the gene encoding the virulence factor of interest |
For virulence factors with limited information, in silico tools (e.g., Phyre2; Kelley et al., 2015) may provide valuable insight to guide hypotheses) | ||
For effectors with catalytic sites or important functional residues, point mutants can also be included (D'Costa et al., 2019) | ||
Contribution of host factor | Wildtype; if applicable, isogenic knockout, complementation strain | To investigate the candidate role of a host factor, siRNA-mediated knockdown or an equivalent approach can be used |
For knockdown studies, knockdown validation should be performed in parallel, and if possible, confirmation of the phenotype associated with knockdown |
Materials
-
HeLa cells (ATCC, cat. no. CCL-2)
-
Phosphate-buffered saline without calcium and magnesium (PBS-/-) (Wisent, cat. no. 311-425-CL)
-
Trypsin, 0.05%, EDTA, phenol red (Wisent, cat. no. 325-542-CL)
-
Dulbecco's Modified Eagle Medium (DMEM), 4.5 g/L glucose with L-glutamine, sodium pyruvate, and phenol red (Wisent, cat. no. 319-005-CL)
-
Fetal bovine serum (FBS) (Wisent, cat. no. 080-450)
-
T75 tissue culture flask
-
CO2 incubator (Nuaire, cat. no. NU-425-400)
-
24-well tissue culture plate, sterile (Corning, cat. no. C353047)
-
12-mm glass cover slips suitable for microscopy, sterile, 0.16 to 0.19-mm thickness (Fisher, cat. no. ER12CIR15G)
Procedure
Before starting, prewarm host cell culture medium to 37°C.
1.Detach host cells from tissue culture flask. For HeLa cells:
-
Remove cell culture medium and rinse with PBS-/-.
-
Apply trypsin to the cells.
For a standard T75 tissue culture flask, use 2 ml.
-
Incubate in a CO2incubator for several minutes until the cells detach.
-
Resuspend the cells in DMEM supplemented with 10% FBS as a culture medium.
2.Using aseptic technique, transfer a sterile glass cover slip to each well of interest in a 24-well plate.
3.Seed host cells in 24-well plates containing glass cover slips. For HeLa cells, seed at 2.5 × 104 cells/well, using DMEM supplemented with 10% FBS as a culture medium. A volume of 1 ml is suggested.
4.Incubate host cells at 37°C in a CO2 incubator until the optimal confluency for infection of the pathogen of interest. For S. Typhimurium infection of epithelial cells, incubate until HeLa cells are 70% to 80% confluent.
Alternate Protocol: CULTURE AND PREPARATION OF HOST CELLS TO ASSESS HOST FACTOR CONTRIBUTION TO BACTERIAL POSITIONING
This experiment can be adapted to study the contribution of host factors to intracellular PCV trafficking (D'Costa et al., 2019) (Table 1). This can involve either short interfering RNA (siRNA)-mediated knockdown of the host factor of interest, suppression by an equivalent method, or using a knockout model. The following protocol is for siRNA-mediated suppression in HeLa cells, but the approach can be adapted to other cell types. When using this approach for the first time, it is important to be sure that expression of the host factor can be sufficiently suppressed prior to conducting an infection experiment. As such, it is recommended to optimize knockdown conditions first and validate suppression of expression (e.g., immunoblotting, quantitative RT-PCR), and if possible, function, based on previously established assays in the literature.
Additional Materials (also see Basic Protocol 1)
-
Opti-MEM reduced serum medium (Gibco, cat. no. LS31985070)
-
Lipofectamine RNAiMAX transfection reagent (Invitrogen, cat. no. 13778-075)
-
siRNA directed against host gene of interest
-
Control siRNA
-
1.5-ml microcentrifuge tubes, sterile
-
Microcentrifuge
Procedure
Before starting, prewarm host cell culture medium to 37°C.
1.Detach host cells from tissue culture flask. For HeLa cells:
-
Remove cell culture medium and rinse with PBS-/-.
-
Apply trypsin to the cells and incubate in a CO2incubator for several minutes until detachment.
-
Resuspend cells in DMEM supplemented with 10% FBS as a culture medium.
2.Using a sterile tool and aseptic technique, transfer a sterile glass cover slip to each well of interest in a 24-well plate. For siRNA-mediated knockdown studies, two wells should be seeded for infection with each strain. In addition, a set of two wells without cover slips should be seeded for validation of knockdown.
3.Seed host cells in a 24-well plate. For HeLa cells, seed at 2.5 × 104 cells/well and a suggested volume of 1 ml, using DMEM supplemented with 10% FBS as a culture medium. Incubate host cells at 37°C in CO2 incubator overnight.
4.At the appropriate cell density for the cell type, set up transfection reactions for knockdown. For HeLa cells, the next day, prepare the following in sterile microcentrifuge tubes:
-
In one tube, add 50 μl Opti-MEM medium, then 3 μl transfection reagent Lipofectamine RNAiMAX.
-
In another tube, add 50 μl Opti-MEM medium, then dilute siRNA to 100 nM.
It is recommended to set up knockdown reactions as a master mix. This will minimize variations in response between equivalent wells.
5.Add diluted siRNA to the tube containing diluted transfection reagent. Mix by inversion and centrifuge 5 s at 10,000 × g , room temperature.
6.Incubate for 20 min at room temperature.
7.During this incubation, aspirate cell culture medium from each well and add 0.5 ml fresh DMEM supplemented with 10% FBS.
8.Add transfection reaction to each well dropwise.
9.The next day, change culture medium to fresh DMEM supplemented with 10% FBS.
10.Knockdown is often detectable at 48 hr post-transfection. If this has been established, this would serve as the starting point for pathogen infection.
Basic Protocol 2: INFECTION OF EPITHELIAL CELLS WITH S. Typhimurium
This protocol focuses on S. Typhimurium infection of epithelial cells. Salmonella uptake into nonphagocytic epithelial cells is mediated by virulence factors secreted by a Type 3 Secretion System (T3SS) encoded on Salmonella pathogenicity island (SPI)-1 (Brumell & Grinstein, 2004), although other uptake mechanisms have been documented to contribute (Boumart et al., 2014). In culture, SPI-1 T3SS virulence factor secretion is promoted by bacterial growth in the late logarithmic phase (Steele-Mortimer et al., 1999). As such, inocula are prepared from actively growing cultures. Should infection efficiency be observed to be less than optimal, consult Critical Parameters and Troubleshooting sections for additional information.
Common S. Typhimurium strains used in pathogenesis research include the strains S. Typhimurium 14028S and SL1344 (D'Costa et al., 2019; Steele-Mortimer et al., 1999). Regarding strain choice, it is important to note that S. Typhimurium SL1344 is a histidine auxotroph, and under certain circumstances, intracellular growth in host cells can lead to septation defects (Henry et al., 2005). This inability to divide limits the ability to accurately assess intracellular bacterial positioning. If needed, an alternative strain can be chosen, or experimental media can be supplemented with L-histidine (Lathrop et al., 2015).
This approach can be adapted to the study of other intracellular vacuolar pathogens. Bacterial growth conditions and inoculum preparation can be optimized for the pathogen of interest, if not previously established in the literature. Growth conditions can vary from growth on solid agar medium to liquid culture. Growth medium choice, nutrient supplementation, and growth phase of harvesting (e.g., logarithmic, stationary, etc.) can also affect efficiency of infection.
Finally, from the perspective of the bacteria, it is important to consider detection for fluorescence microscopy. The methods described here involve antibody-based detection (performed in Basic Protocol 3). However, should an immunofluorescence-compatible antibody not exist for the pathogen of interest, a strain expressing a fluorescent protein (e.g., RFP) may be suitable. This may be accomplished using a plasmid or chromosomal integration (Hautefort et al., 2003; Valdivia et al., 1996). Should a suitable pathogen-specific plasmid not exist, broad host spectrum plasmids exist that might be valuable to consider (Barbier & Damron, 2016).
Materials
-
Salmonella enterica serovar Typhimurium 14028S (Miao et al., 1999)
-
LB Miller broth (BioShop, cat. no. LBL407)
-
Agar, bacteriological grade (BioShop, cat. no. AGR001)
-
Phosphate-buffered saline with calcium and magnesium (PBS+/+) (Wisent, cat. no. 311-011-CL)
-
Host cells (see Basic Protocol 1 and Alternate Protocol)
-
Dulbecco's Modified Eagle Medium (DMEM), 4.5 g/L glucose with L-glutamine, sodium pyruvate, and phenol red (Wisent, cat. no. 319-005-CL)
-
Fetal bovine serum (FBS) (Wisent, cat. no. 080-450)
-
Gentamicin sulfate, sterile, 50 mg/ml (BioShop, cat. no. GTA401)
-
Paraformaldehyde (PFA), 16% (Electron Microscopy Sciences, cat. no. 15710)
-
Bacterial culture tubes, sterile (Corning, cat. no. C352059)
-
Incubator shaker (New Brunswick Innova 42)
-
125-ml flask, sterile
-
Microcentrifuge
-
CO2 incubator (Nuaire, cat. no. NU-425-400)
Procedure
Regarding timing of host and pathogen cells, host cell growth should be monitored such that bacterial inocula can be prepared when needed based on host cell considerations (e.g., confluency, timing required for knockdown). It is always recommended to start with a freshly grown agar plate of bacteria, streaked for single colonies. If the strain of interest is resistant to an antibiotic that can be used for selection purposes, it is recommended to supplement the initial growth plate with antibiotic to minimize contamination.
Before starting, prewarm host cell culture medium to 37°C.
1.Prepare the pathogen inoculum such that it is ready for infection of host cells when needed. For S. Typhimurium 14028S, this involves setting up an overnight culture the day before infection. In a sterile bacterial culture tube, inoculate 2 ml LB with several single colonies. Incubate for 16 to 20 hr at 37°C with shaking at 250 rpm.
2.On the day of infection, prepare the pathogen inoculum as required for infection. For S. Typhimurium infection of epithelial cells:
-
Set up a sterile 125-ml flask containing 10 ml LB.
-
Subculture the strain from the overnight culture at a dilution of 1:33 (Steele-Mortimer et al.,1999), and grow at 37°C and 250 rpm for 3 hr.
-
Pellet the culture by centrifuging 2 min at 10,000 ×g, room temperature.
-
Remove spent culture medium and resuspend cells in the equivalent volume of sterile phosphate-buffered saline (PBS+/+).
-
Prepare the inoculum dilution in sterile PBS+/+to the appropriate multiplicity of infection.
For Salmonella, this subculturing step will promote the secretion of SPI-1 T3SS virulence factors needed for optimal invasion (Steele-Mortimer et al.,1999). SPI-1 T3SS virulence factor secretion is correlated with the logarithmic phase of growth (Steele-Mortimer et al.,1999). Should infection efficiency be poor, consult the Critical Parameters and Troubleshooting sections for additional information.
3.Aspirate culture medium from host cells, and wash with PBS+/+. Apply pathogen inoculum to host cells as previously established for the pathogen. For S. Typhimurium infection of epithelial cells, incubate inoculated host cells in a CO2 incubator at 37°C for 10 min.
4.The time period for inoculation of host cells will vary among pathogens. After the appropriate amount of time, aspirate sample from host cells and wash three times with PBS+/+. Apply host cell growth medium and incubate in the CO2 incubator. For S. Typhimurium infection of epithelial cells, use DMEM supplemented with 10% FBS.
5.At the appropriate timepoint previously established for the pathogen, change culture medium to growth medium supplemented with antibiotic for selection of intracellular bacteria. For Salmonella infection of epithelial cells:
-
At 30 min post-infection (p.i.), change medium to DMEM supplemented with 10% FBS and 100 μg/ml gentamicin (D'Costa et al.,2015).
-
At 2 hr p.i., change culture medium to DMEM supplemented with 10% FBS and 10 μg/ml gentamicin. This lower concentration of gentamicin will serve for maintenance purposes.
The time required for PCV formation (completion of internalization into host cells) is an important consideration for this step. Depending on the pathogen, this information may be previously established in the literature or can be assessed experimentally. If the intracellular selection step is carried out too early, the pathogen may be prematurely targeted by the extracellular antibiotic, leading to low levels of pathogen uptake.
6.Prepare the paraformaldehyde (PFA) solution for fixation by diluting the stock solution with PBS+/+ to a concentration of 2.5%.
7.At each timepoint of interest, wash infected cells with PBS+/+ and add 300 μl of 2.5% PFA solution per well. Incubate in CO2 incubator at 37°C for 15 min.
8.Wash cover slips three times with PBS+/+, leaving excess buffer on cover slips.
Basic Protocol 3: FLUORESCENCE STAINING FOR ANALYSIS OF BACTERIAL POSITIONING
To assess intracellular positioning of vacuolar pathogens, the following elements should be visualized by microscopy: the bacteria, the pathogen-containing vacuole membrane, and the host nucleus. This protocol involves the staining of S. Typhimurium-infected human cells, using antibodies for the detection of the bacteria and Salmonella -containing vacuole membrane, which is positive for the host marker LAMP1 (Fig. 3). The host cell nucleus is visualized using the dye 4',6-diamidino-2-phenylindole dihydrochloride (DAPI).
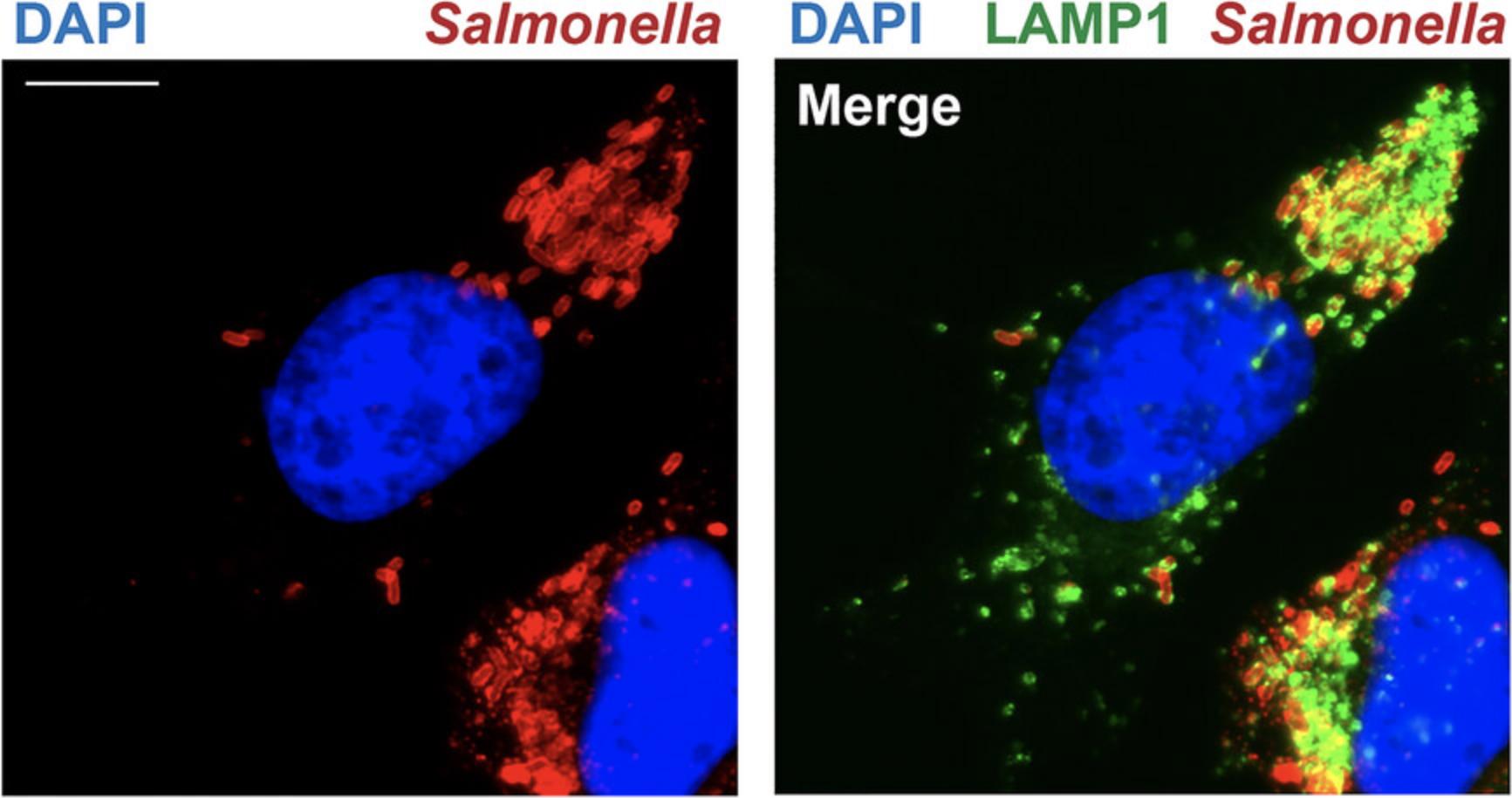
To adapt this approach for the study of other pathogens and hosts, equivalent antibodies can be used. If a fluorescent protein (e.g., RFP)-expressing bacterial strain is used, the staining of the pathogen can be omitted. This method visualizes the PCV membrane in the green fluorescence channel. If GFP is used for bacterial detection, an alternate secondary antibody for the host PCV can be used (e.g., Alexa Fluor 568 conjugated). Conditions for antibody staining (i.e., concentration, incubation time) will depend on the antibodies selected. This information is usually preestablished in the literature, but for a poorly studied pathogen, this can be optimized experimentally.
Materials
-
Serum-saponin-PBS solution (SS-PBS) (see recipe)
-
Plates with pathogen-infected host cells (see Basic Protocol 2)
-
Rabbit anti-Salmonella antibody (BD, cat. no. 229481)
-
Mouse anti-LAMP1 antibody (H4A3) (DSHB, cat. no. H4A3-s)
-
Phosphate-buffered saline with calcium and magnesium (PBS+/+) (Wisent, cat. no. 311-011-CL)
-
Goat anti-Mouse IgG antibody, Alexa Fluor 488 conjugate (Invitrogen, cat. no. A-11029)
-
Goat anti-Rabbit IgG antibody, Alexa Fluor 568 conjugate (Invitrogen, cat. no. A-11036)
-
DAPI stock solution (see recipe)
-
ddH2O
-
Dako fluorescence mounting medium (Agilent, cat. no. S3023)
-
Aluminum foil
-
Microscope slide (VWR, cat. no. 16004-368)
-
Fine-tipped tweezers
Procedure
1.Prepare SS-PBS solution (see recipe).
2.Aspirate PBS from wells and add 250 μl per well of SS-PBS. Incubate at room temperature for 30 min.
3.While incubating, prepare the primary antibody solution by diluting the following antibodies 1:100 in SS-PBS: rabbit anti-Salmonella primary antibody, and mouse anti-LAMP1 primary antibody.
4.Aspirate SS-PBS and cover surface of the cover slips with primary antibody solution. A volume of 12 to 14 μl is suggested. Incubate at room temperature for 1 hr.
5.Wash cover slips twice with PBS+/+.
6.Reapply PBS+/+ to cover slips and incubate at room temperature for 10 min.
7.Aspirate liquid and repeat step 6 two more times.
8.Aspirate liquid and add 250 μl per well of SS-PBS. Incubate at room temperature for 30 min.
9.While incubating, prepare the secondary antibody solution by diluting the following antibodies 1:200 in SS-PBS: goat anti-mouse Alexa Fluor 488 antibody, and goat anti-rabbit Alexa Fluor 568 antibody.
10.Aspirate buffer and cover surface of cover slips with secondary antibody solution. A volume of 12 to 14 μl is suggested. Incubate at room temperature for 1 hr.
11.Wash cover slips twice with PBS+/+.
12.Reapply PBS+/+ to cover slips and incubate at room temperature for 10 min.
13.Repeat step 12 two more times.
14.While incubating, prepare DAPI solution (see recipe) by diluting dye in ddH2O to a concentration of 2 μg/ml.
15.Wash cover slips twice with ddH2O.
16.Aspirate liquid and add 250 μl DAPI solution per well. Incubate at room temperature for 5 min.
17.Wash cover slips twice with ddH2O.
18.Reapply ddH2O to cover slips and incubate at room temperature for 10 min.
19.Aspirate liquid and repeat step 18.
20.Apply one drop of Dako fluorescence mounting medium on a microscope slide per cover slip. Using fine-tipped tweezers, invert cover slip onto mounting medium, and remove excess mounting medium by gently blotting.
21.Place microscope slides in a dark location overnight at room temperature to dry.
Basic Protocol 4: FLUORESCENCE MICROSCOPY ANALYSIS OF BACTERIAL POSITIONING
Fluorescence microscopy imaging can be performed with either an epifluorescence or confocal microscope, as long as individual bacteria in the images are clearly defined. It is recommended that a high magnification oil immersion lens (e.g., 63× objective) be used. It must also be ensured that the microscope is calibrated for the objective of choice. The following protocol outlines analysis using the commonly used software Fiji (ImageJ). However equivalent analyses can be performed with any suitable imaging software, such as Volocity.
Materials
- Microscope slide with samples mounted (from Basic Protocol 3)
- Leica DMIRE2 epifluorescence microscope
- Fiji (ImageJ, v1.54f 29, June 2023) analysis software
- Microsoft Excel
Procedure
1.With a fluorescence microscope and 63× oil immersion objective, assess cover slip for infected host cells. The infected host cell should fit within the field of view of the camera.
2.Capture images of infected host cells in all three channels.
3.For analysis, open imaging software Fiji (ImageJ).
4.Open image of interest. It is recommended to use the Composite setting such that all channels can be assessed as an overlay.
5.Because this assay measures PCV positioning, for each infected host cell of interest, it is important to establish whether each intracellular bacterium is in a vacuole. Carefully inspect the infected host cell of interest, assessing each bacterium for the presence of a PCV membrane. For Salmonella , the host marker for the PCV is LAMP, visualized in the green channel (Fig. 4A).
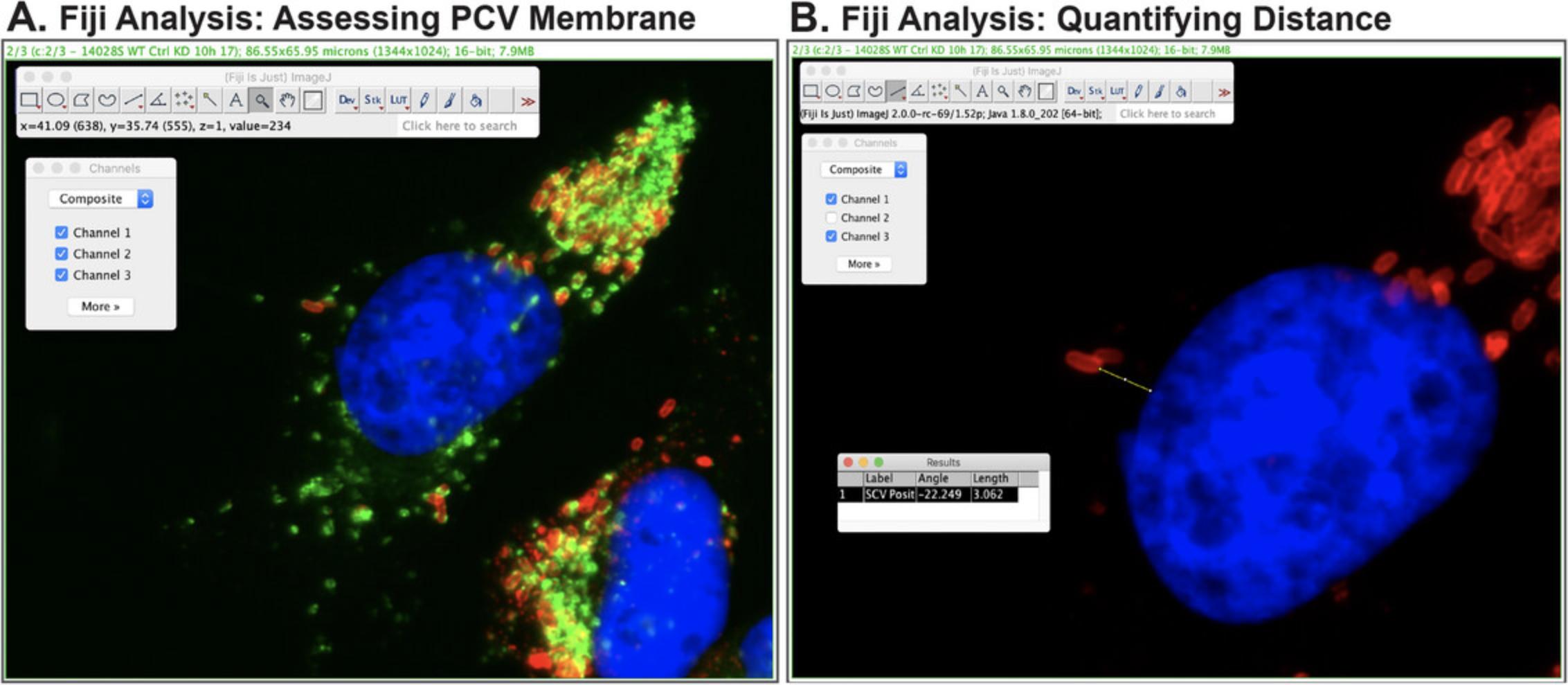
6.Bacterial positioning will be measured as a distance relative to the host nucleus. Select the Line Tool icon from the menu bar. Ensure that the lines to be drawn will be straight lines by right clicking on the icon and selecting Straight Line.
7.Bacterial positioning is measured as the shortest distance between the edge of the bacterium to the nearest edge of the host nucleus. To do this, click on the starting point on the edge of the bacterium and drag the cursor to the end point on the edge of the nucleus.
8.Measure the distance by selecting from the menu Analyze → Measure. A Results window will appear, indicating the calculated length (Fig. 4B). These values can be copied and pasted into Excel for subsequent Analysis.
9.Repeat step 8 for every intracellular bacterium established to be within a vacuole.
10.Continue distance measurements until quantifications are performed on at least 20 infected cells, such that >100 bacteria were assessed per biological replicate experiment. At least three biological replicate experiments should be performed.
11.Using Excel, calculate the mean distance from the nucleus, expressed in μm, for each experimental condition.
REAGENTS AND SOLUTIONS
DAPI stock solution, 5 mg/ml
- For 2 ml:
- 10 mg of 4',6-diamidino-2-phenylindole, dihydrochloride (DAPI) (Invitrogen, cat. no. D1306)
- 2 ml ddH2O
- Dissolve dye in ddH2O
- Aliquot in small volumes (e.g., 50 μl)
- Store protected from light up to 6 months at −20°C
Serum-saponin-PBS (SS-PBS) solution
- For 10 ml:
- 1 ml goat serum (10% final concentration) (Wisent, cat. no. 053-150)
- 0.2 ml of 10% saponin (0.2% final concentration) (Sigma, cat. no. 558255)
- 8.8 ml of PBS+/+ (Wisent, cat. no. 311-011-CL)
- Prepare fresh
COMMENTARY
Background Information
The ability to assess infection progression is a valuable tool in pathogenesis research. Intracellular vacuolar pathogens commonly use secreted virulence factors to hijack the host cytoskeleton to direct PCV trafficking to the site of replication, and if applicable, may subsequently manipulate host trafficking for bacterial movement back toward the host cell periphery for cell-to-cell spread (Brumell & Scidmore, 2007; Stevens et al., 2006). As such, intracellular bacterial positioning represents a valuable measure of infection progression. This approach describes a general method that can be adapted for the study of diverse intracellular pathogens and host cell types and can be used to assess both well established and poorly understood microbes. Once the kinetics of intracellular positioning have been established for the pathogen and host cell of interest, the assay can subsequently be adapted to ask more intricate questions regarding pathogenesis, including the assessment of virulence factors that mediate trafficking and host factors that are targeted, as well as domain mapping studies to investigate functional domains within the virulence factor that mediate the phenotype of interest.
The fluorescence microscopy-based analysis approach used in this protocol allows for the visualization of individual infected host cells, and as such, is amenable to studies of the heterogeneity of infection. Given that broad host spectrum fluorescent protein-expressing plasmids are accessible to researchers and are compatible with this method, this allows for the analysis of poorly understood pathogens in which commercially available antibodies for pathogen visualization may not exist. It is also important to note that while intracellular bacterial positioning is a valuable measure of infection, the intracellular life cycle of each pathogen of interest is unique, and it is possible that the timepoint of interest may not be one in which the bacteria are actively trafficking. If this is the case, an alternative measure of infection progression [e.g., intracellular bacteria count or colony forming unit (CFU) quantification] may be more suitable. Finally, while the analysis described in this approach can be considered low throughput, this approach is likely amenable to quantification by high content methods.
Critical Parameters
Host cell culture conditions
From the perspective of host cell type, host cell density and culture conditions are important considerations for establishing a successful infection assay (see Strategic Planning). Pathogens may demonstrate an optimal infection efficiency within specific ranges of host cell confluency (Gaillard & Finlay, 1996; Mooney et al., 2003). Similarly, certain cell types may require culture supplementation with specific cytokines or differentiation-promoting factors [e.g., macrophage colony stimulating factor (M-CSF) for macrophages] to allow for pathogen uptake. In instances where the pathogen of interest is poorly studied, these factors should be considered in assay development.
Should this assay be needed to assess contribution of a host cell factor of interest, this method describes an approach for siRNA-mediated knockdown (see Alternate Protocol). Should gene knockdown be the system of choice, it is important to ensure that knockdown conditions for the host protein selected for targeting have been established such that the pathway of interest is sufficiently suppressed. Alternatively, knockout cells may be used in comparison to the equivalent wildtype cell line.
Bacterial culture and infection conditions
In the context of Salmonella Typhimurium infection of epithelial cells, bacterial uptake is predominantly mediated by secreted virulence factors from the SPI-1 T3SS (Brumell & Grinstein, 2004). With regards to inoculum preparation, SPI-1 T3SS virulence factor secretion is evident in the late logarithmic phase of growth (Steele-Mortimer et al., 1999). As such, inocula are prepared from actively growing cultures. Should infection efficiency be observed to be less than optimal, it is possible that the growth kinetics of the strain selected could be slightly different such that effector secretion in a 3 hr subculture is less than optimal. If so, it is recommended to conduct a growth curve series of the subculture, plotting OD600 vs time, and assessing CFU counts of each timepoint.
Should the system chosen require the strain of interest to express a shuttle plasmid (e.g., for fluorescent protein expression or for complementation purposes), it is possible that the presence of the additional plasmid may impair pathogen uptake into host cells (Clark et al., 2009; Knodler et al., 2005). Should this be observed, an adjustment in multiplicity of infection can help to compensate.
Another important assay parameter is selection for intracellular bacteria, to ensure that the phenotype of interest is not induced or promoted by extracellular bacteria (Elsinghorst, 1994). This is accomplished by treatment of infected host cells with an antibiotic that demonstrates low levels of penetration into host cells, such as gentamicin (termed gentamicin protection) (Elsinghorst, 1994). It is therefore important to know that the bacterial strain demonstrates sensitivity to the antibiotic of choice. In cases of multidrug resistant pathogens or where the pathogen is resistant to gentamicin and other aminoglycosides, the polymyxin class may be a suitable alternative (Lee et al., 1990).
Troubleshooting
As discussed in the Critical Parameters section, several common challenges can be encountered during assay development. For a summary of common challenges encountered and suggested parameters to optimize, see Table 2.
Problem | Possible cause | Solution |
---|---|---|
Poor infection efficiency | Suboptimal host cell conditions | Host cells may be at suboptimal confluency at the time of infection; a confluency assessment can be performed if needed |
Host cells may require additional supplementation in cell culture medium to promote uptake; consult literature if needed | ||
Suboptimal inoculum | Perform CFU analysis to ensure that inoculum was viable | |
If virulence factor production is dependent on growth phase, perform growth curve analysis to verify for strain of interest | ||
Poor infection efficiency (plasmid expressing strains) | Presence of additional plasmid | Increase multiplicity of infection as needed |
Poor infection efficiency (Salmonella specific) | Poor SPI-1 virulence factor secretion | Perform growth curve with corresponding CFU assessment to identify late logarithmic phase |
No intracellular bacteria | Intracellular protection assay ineffective | Selection before completion of uptake into intact pathogen-containing vacuole can result in bacterial killing; consider increasing this time period |
Phagocytic host cells may have internalized selective drug, leading to pathogen killing (Hamrick et al., 2003); consider optimization of antibiotic concentration | ||
Extracellular bacteria observed | Intracellular protection assay ineffective | If strain should be sensitive to the antibiotic chosen (e.g., gentamicin), perform a minimum inhibitory concentration assay to determine level of sensitivity; concentration chosen for assay may need slight adjustment |
If strain is resistant to antibiotic chosen, an alternative drug class will be needed (e.g., polymyxin) |
||
Bacteria appear filamentous (Salmonella specific) | Septation defect due to histidine auxotrophy (Henry et al., 2005) | Supplement culture medium with L- histidine (Lathrop et al., 2015); alternatively, a different Salmonella strain can be chosen (e.g., 14028S) |
Understanding Results
Intracellular vacuolar pathogens have evolved diverse strategies to manipulate host cell processes to mediate infection (Brumell & Scidmore, 2007; Henry et al., 2005). While each pathogen of interest is likely to have established a unique arsenal of strategies to hijack host trafficking, an important commonality is that each pathogen must traffic from the site of uptake, namely the host plasma membrane, to the site of replication (Brumell & Scidmore, 2007; Henry et al., 2005). As such, the mean relative distance to the nucleus calculated should decrease during the early stages of infection. In terms of data presentation, the results of this assay are most simply presented as bar graphs measuring mean distance from the nucleus (D'Costa et al., 2019; Szeto et al., 2009).
If intracellular positioning has not been previously established for the strain and host cell of interest, it is strongly recommended to perform a kinetic analysis of the wildtype strain before proceeding to more complex experimental questions (e.g., virulence factor modulation, host target assessment, etc.). This will allow the researcher to establish a baseline measurement to map the intracellular life cycle. This is especially important in cases where the pathogen is capable of mediating retrograde trafficking of PCVs during the later stages of infection. Without a baseline assessment, the consequences of virulence factor modulation or host factor contribution can be difficult to interpret. It is also important to appreciate that the kinetics of intracellular trafficking may vary between strains of the same bacterium, further highlighting the importance of establishing a baseline set of measurements.
It is possible that a series of comparative experiments (e.g., virulence factor modulation or host target assessment) could generate misleading results due to the cooperativity of virulence factor mechanisms of action. Bacterial pathogens often evolve multiple virulence factors that work in concert to manipulate host cellular pathways (Galan, 2009). For example, the contribution of a virulence factor in wildtype vs isogenic knockouts assays could be underappreciated or undetected if multiple virulence factors contribute to the phenotype. As such, a phenotype might not be detected until a multiple gene knockout strain is created.
Another possible unexpected observation is if an isogenic knockout strain or host knockdown condition results in the inability to detect the PCV membrane (Beuzon et al., 2000). While this could represent one of several scenarios, including nonfusogenic vacuoles (Sinai & Joiner, 1997), a likely possibility is that the strain or condition causes a destabilization of the PCV membrane, resulting in cytosolic bacteria (Beuzon et al., 2000). This phenotype is known to result from suppressing virulence factors or host proteins that function via the host cytoskeleton (Boucrot et al., 2005; D'Costa et al., 2019; Guignot et al., 2004). While this observation cannot be corrected by experimental optimization, it represents an important suggestion that the virulence factor or host protein contributes to PCV trafficking.
Time Considerations
Collectively, the described protocols using Salmonella infection of epithelial cells as a model take ∼5 to 7 days to perform. Culturing of host cells (Basic Protocol 1 or Alternate Protocol) usually require 2 to 3 days to complete, and Salmonella infection (Basic Protocol 2) and fluorescence staining (Basic Protocol 3) can each be performed within a day. Fluorescence microscopy (Basic Protocol 4) can take one to several days, depending on the complexity of the experiment and the number of samples to assess. When adapting the assay to different host cells or pathogens, the host culturing and infection procedures may take longer to complete. For example, some host cell types are slow to expand to a cell density that is appropriate for infection or require time for differentiation, while some pathogens are either slow to culture to prepare the inoculum, or slow to replicate within host cells.
Acknowledgments
This protocol was adapted from a previously published study (D'Costa et al., 2019). We thank members of the D'Costa Lab for helpful discussions. This work was funded by Operating Grants from the Natural Sciences and Engineering Research Council of Canada (NSERC) (PJ4-175369 and PJT-178191) to V.M.D.
Author Contributions
Farah Nasser : Data curation; methodology; writing original draft; writing review and editing. Mosopefoluwa Oke : Writing original draft; writing review and editing. Sara Knezevic : Writing original draft; writing review and editing. Vanessa D'Costa : Conceptualization; data curation; methodology; writing original draft; writing review and editing.
Conflict of Interest
The authors declare no conflict of interest.
Open Research
Data Availability Statement
This protocol was adapted from a previously published study (D'Costa et al., 2019). Data sharing not applicable to this article as no datasets were generated for quantification during the current study.
Literature Cited
- Barbier, M., & Damron, F. H. (2016). Rainbow vectors for broad-range bacterial fluorescence labeling. PLoS ONE , 11(3), e0146827. https://doi.org/10.1371/journal.pone.0146827
- Beuzon, C. R., Meresse, S., Unsworth, K. E., Ruiz-Albert, J., Garvis, S., Waterman, S. R., Ryder, T. A., Boucrot, E., & Holden, D. W. (2000). Salmonella maintains the integrity of its intracellular vacuole through the action of SifA. EMBO Journal , 19(13), 3235–3249. https://doi.org/10.1093/emboj/19.13.3235
- Bhavsar, A. P., Guttman, J. A., & Finlay, B. B. (2007). Manipulation of host-cell pathways by bacterial pathogens. Nature , 449(7164), 827–834. https://doi.org/10.1038/nature06247
- Boucrot, E., Henry, T., Borg, J. P., Gorvel, J. P., & Meresse, S. (2005). The intracellular fate of Salmonella depends on the recruitment of kinesin. Science , 308(5725), 1174–1178. https://doi.org/10.1126/science.1110225
- Boumart, Z., Velge, P., & Wiedemann, A. (2014). Multiple invasion mechanisms and different intracellular behaviors: A new vision of Salmonella -host cell interaction. FEMS Microbiology Letters , 361(1), 1–7. https://doi.org/10.1111/1574-6968.12614
- Brumell, J. H., & Grinstein, S. (2004). Salmonella redirects phagosomal maturation. Current Opinion in Microbiology , 7(1), 78–84. https://doi.org/10.1016/j.mib.2003.12.005
- Brumell, J. H., & Scidmore, M. A. (2007). Manipulation of rab GTPase function by intracellular bacterial pathogens. Microbiology and Molecular Biology Reviews , 71(4), 636–652. https://doi.org/10.1128/MMBR.00023-07
- Castanheira, S., & Garcia-Del Portillo, F. (2017). Salmonella populations inside host cells. Frontiers in Cellular and Infection Microbiology , 7, 432. https://doi.org/10.3389/fcimb.2017.00432
- Clark, L., Martinez-Argudo, I., Humphrey, T. J., & Jepson, M. A. (2009). GFP plasmid-induced defects in Salmonella invasion depend on plasmid architecture, not protein expression. Microbiology , 155(Pt 2), 461–467. https://doi.org/10.1099/mic.0.025700-0
- D'Costa, V. M., Braun, V., Landekic, M., Shi, R., Proteau, A., McDonald, L., Cygler, M., Grinstein, S., & Brumell, J. H. (2015). Salmonella disrupts host endocytic trafficking by SopD2-Mediated inhibition of Rab7. Cell Reports , 12(9), 1508–1518. https://doi.org/10.1016/j.celrep.2015.07.063
- D'Costa, V. M., Coyaud, E., Boddy, K. C., Laurent, E. M. N., St-Germain, J., Li, T., Grinstein, S., Raught, B., & Brumell, J. H. (2019). BioID screen of Salmonella type 3 secreted effectors reveals host factors involved in vacuole positioning and stability during infection. Nature Microbiology , 4(12), 2511–2522. https://doi.org/10.1038/s41564-019-0580-9
- Diacovich, L., & Gorvel, J. P. (2010). Bacterial manipulation of innate immunity to promote infection. Nature Reviews Microbiology , 8(2), 117–128. https://doi.org/10.1038/nrmicro2295
- Elsinghorst, E. A. (1994). Measurement of invasion by gentamicin resistance. Methods in Enzymology , 236, 405–420. https://doi.org/10.1016/0076-6879(94)36030-8
- Gaillard, J. L., & Finlay, B. B. (1996). Effect of cell polarization and differentiation on entry of Listeria monocytogenes into the enterocyte-like Caco-2 cell line. Infection and Immunity , 64(4), 1299–1308. https://doi.org/10.1128/iai.64.4.1299-1308.1996
- Galan, J. E. (2009). Common themes in the design and function of bacterial effectors. Cell Host & Microbe, 5(6), 571–579. https://doi.org/10.1016/j.chom.2009.04.008
- Giannella, R. A., Washington, O., Gemski, P., & Formal, S. B. (1973). Invasion of HeLa cells by Salmonella typhimurium : A model for study of invasiveness of Salmonella. Journal of Infectious Diseases , 128(1), 69–75. https://doi.org/10.1093/infdis/128.1.69
- Guignot, J., Caron, E., Beuzon, C., Bucci, C., Kagan, J., Roy, C., & Holden, D. W. (2004). Microtubule motors control membrane dynamics of Salmonella -containing vacuoles. Journal of Cell Science , 117(Pt 7), 1033–1045. https://doi.org/10.1242/jcs.00949
- Hamrick, T. S., Diaz, A. H., Havell, E. A., Horton, J. R., & Orndorff, P. E. (2003). Influence of extracellular bactericidal agents on bacteria within macrophages. Infection and Immunity , 71(2), 1016–1019. https://doi.org/10.1128/IAI.71.2.1016-1019.2003
- Hautefort, I., Proenca, M. J., & Hinton, J. C. (2003). Single-copy green fluorescent protein gene fusions allow accurate measurement of Salmonella gene expression in vitro and during infection of mammalian cells. Applied and Environmental Microbiology , 69(12), 7480–7491. https://doi.org/10.1128/AEM.69.12.7480-7491.2003
- Henry, T., Garcia-Del Portillo, F., & Gorvel, J. P. (2005). Identification of Salmonella functions critical for bacterial cell division within eukaryotic cells. Molecular Microbiology , 56(1), 252–267. https://doi.org/10.1111/j.1365-2958.2005.04540.x
- Jennings, E., Thurston, T. L. M., & Holden, D. W. (2017). Salmonella SPI-2 Type III secretion system effectors: Molecular mechanisms and physiological consequences. Cell Host & Microbe, 22(2), 217–231. https://doi.org/10.1016/j.chom.2017.07.009
- Jiao, Y., & Sun, J. (2019). Bacterial manipulation of autophagic responses in infection and inflammation. Frontiers in Immunology , 10, 2821. https://doi.org/10.3389/fimmu.2019.02821
- Kellermann, M., Scharte, F., & Hensel, M. (2021). Manipulation of host cell organelles by intracellular pathogens. International Journal of Molecular Sciences , 22(12), 6484. https://doi.org/10.3390/ijms22126484
- Kelley, L. A., Mezulis, S., Yates, C. M., Wass, M. N., & Sternberg, M. J. (2015). The Phyre2 web portal for protein modeling, prediction and analysis. Nature Protocols , 10(6), 845–858. https://doi.org/10.1038/nprot.2015.053
- Knodler, L. A., Bestor, A., Ma, C., Hansen-Wester, I., Hensel, M., Vallance, B. A., & Steele-Mortimer, O. (2005). Cloning vectors and fluorescent proteins can significantly inhibit Salmonella enterica virulence in both epithelial cells and macrophages: Implications for bacterial pathogenesis studies. Infection and Immunity , 73(10), 7027–7031. https://doi.org/10.1128/IAI.73.10.7027-7031.2005
- Lathrop, S. K., Binder, K. A., Starr, T., Cooper, K. G., Chong, A., Carmody, A. B., & Steele-Mortimer, O. (2015). Replication of Salmonella enterica Serovar Typhimurium in human monocyte-derived macrophages. Infection and Immunity , 83(7), 2661–2671. https://doi.org/10.1128/IAI.00033-15
- Lee, C. K., Roberts, A. L., Finn, T. M., Knapp, S., & Mekalanos, J. J. (1990). A new assay for invasion of HeLa 229 cells by Bordetella pertussis : Effects of inhibitors, phenotypic modulation, and genetic alterations. Infection and Immunity , 58(8), 2516–2522. https://doi.org/10.1128/iai.58.8.2516-2522.1990
- Lobet, E., Letesson, J. J., & Arnould, T. (2015). Mitochondria: A target for bacteria. Biochemical Pharmacology , 94(3), 173–185. https://doi.org/10.1016/j.bcp.2015.02.007
- Matsuda, S., Okada, N., Kodama, T., Honda, T., & Iida, T. (2012). A cytotoxic type III secretion effector of Vibrio parahaemolyticus targets vacuolar H+-ATPase subunit c and ruptures host cell lysosomes. Plos Pathogens , 8(7), e1002803. https://doi.org/10.1371/journal.ppat.1002803
- Miao, E. A., Scherer, C. A., Tsolis, R. M., Kingsley, R. A., Adams, L. G., Bäumler, A. J., & Miller, S. I. (1999). Salmonella typhimurium leucine-rich repeat proteins are targeted to the SPI1 and SPI2 type III secretion systems. Molecular microbiology , 34(4), 850–864. https://doi.org/10.1046/j.1365-2958.1999.01651.x
- Mooney, A., Byrne, C., Clyne, M., Johnson-Henry, K., Sherman, P., & Bourke, B. (2003). Invasion of human epithelial cells by Campylobacter upsaliensis. Cellular Microbiology , 5(11), 835–847. https://doi.org/10.1046/j.1462-5822.2003.00325.x
- Newton, H. J., & Roy, C. R. (2011). The Coxiella burnetii Dot/Icm system creates a comfortable home through lysosomal renovation. MBio , 2(5). https://doi.org/10.1128/mBio.00226-11
- Sinai, A. P., & Joiner, K. A. (1997). Safe haven: The cell biology of nonfusogenic pathogen vacuoles. Annual Review of Microbiology , 51, 415–462. https://doi.org/10.1146/annurev.micro.51.1.415
- Steele-Mortimer, O., Meresse, S., Gorvel, J. P., Toh, B. H., & Finlay, B. B. (1999). Biogenesis of Salmonella typhimurium -containing vacuoles in epithelial cells involves interactions with the early endocytic pathway. Cellular Microbiology , 1(1), 33–49. https://doi.org/10.1046/j.1462-5822.1999.00003.x
- Stevens, J. M., Galyov, E. E., & Stevens, M. P. (2006). Actin-dependent movement of bacterial pathogens. Nature Reviews Microbiology , 4(2), 91–101. https://doi.org/10.1038/nrmicro1320
- Szeto, J., Namolovan, A., Osborne, S. E., Coombes, B. K., & Brumell, J. H. (2009). Salmonella -containing vacuoles display centrifugal movement associated with cell-to-cell transfer in epithelial cells. Infection and Immunity , 77(3), 996–1007. https://doi.org/10.1128/IAI.01275-08
- Tuli, A., & Sharma, M. (2019). How to do business with lysosomes: Salmonella leads the way. Current Opinion in Microbiology , 47, 1–7. https://doi.org/10.1016/j.mib.2018.10.003
- Valdivia, R. H., Hromockyj, A. E., Monack, D., Ramakrishnan, L., & Falkow, S. (1996). Applications for green fluorescent protein (GFP) in the study of host-pathogen interactions. Gene , 173(1), 47–52. https://doi.org/10.1016/0378-1119(95)00706-7