Animal Models for Toxoplasma gondii Infection
Carlos S. Subauste, Carlos S. Subauste, Alyssa Hubal, Alyssa Hubal
Abstract
Toxoplasma gondii is an obligate intracellular protozoan parasite that commonly infects mammals and birds throughout the world. This protocol describes murine models of acute T. gondii infection, toxoplasmic encephalitis and toxoplasma retinochoroiditis. T. gondii infection in severe combined immunodeficient (SCID) mice, deficient in T and B cells, has allowed for the study of T cell-independent mechanisms of defense against intracellular organisms, as described here. The uracil auxotroph strain cps1-1 and temperature-sensitive mutant strains of T. gondii induce protection against challenge with virulent strains of the parasite. They have allowed studies of immunization and adoptive-transfer experiments. A protocol is provided for infection with these mutant strains. The EGS strain of T. gondii has the unique feature of spontaneously forming tissue cysts in cell culture. Dual fluorescent reporter stains of this strain have allowed the study of tachyzoite to bradyzoite transitions in vitro and in vivo. A protocol for in vitro and in vivo growth of this strain and tissue cyst isolation is provided. Genetic manipulation of T. gondii and mice has led to the development of parasites that express fluorescent proteins as well as mice with fluorescently labeled leukocytes. This together with the use of T. gondii that express model antigens and transgenic mice that express the appropriate T cell receptor have facilitated the in vivo study of parasite host-interaction. In addition, parasites that express bioluminescent markers have made it possible to study the dynamics of infection in real time using bioluminescence imaging. Support protocols present methodology for evaluation of progression of infection and immune response to the parasite that includes these newer methodologies. In addition, support protocols address the maintenance of T. gondii tissue cysts and tachyzoites, as well as preparation of T. gondii lysate antigens. © 2023 The Authors. Current Protocols published by Wiley Periodicals LLC.
Basic Protocol 1 : Induction of acute T. gondii infection in mice
Basic Protocol 2 : Model of toxoplasmic encephalitis and toxoplasma retinochoroiditis in chronically infected mice
Basic Protocol 3 : Assessment of T. gondii invasion into neural tissue
Basic Protocol 4 : T. gondii infection in scid/scid (SCID) mice
Basic Protocol 5 : Infection with the uracil auxotroph strain CPS1-1 or the temperature-sensitive TS-4 strain of T. gondii
Basic Protocol 6 : In vivo and in vitro maintenance of the EGS strain of T. gondii
Support Protocol 1 : Assessment of progression of infection and immune response to T. gondii
Support Protocol 2 : Maintenance of a bank of T. gondii cysts of the ME49 strain
Support Protocol 3 : Maintenance of T. gondii tachyzoites using human foreskin fibroblasts
Support Protocol 4 : Maintenance of T. gondii tachyzoites in mice
Support Protocol 5 : Preparation of T. gondii lysate antigens
Support Protocol 6 : Isolation of T. gondii tissue cysts from brain
INTRODUCTION
During the acute phase of Toxoplasma gondii infection, the rapidly proliferating tachyzoite form of the parasite (Fig. 1) invades any nucleated cell of multiple tissues in the infected host. The chronic (latent) phase of infection is characterized by disappearance of tachyzoites and formation of bradyzoite-containing tissue cysts (Fig. 2), which appear to persist in the host for life. Whereas acute infection with this organism is usually asymptomatic in healthy humans, systemic illness, severe neurologic sequelae, and even death can occur when infection is acquired congenitally or during reactivation of the chronic (latent) infection in patients with deficiencies in cell-mediated immunity. Toxoplasmic encephalitis is the most common clinical presentation of toxoplasmosis in immunocompromised patients. In addition, immunocompetent and immunosuppressed patients can develop toxoplasma retinochoroiditis resulting in legal blindness in at least one eye in ∼25% of patients (Bosch-Driessen et al., 2022). Murine models of T. gondii infection have demonstrated the importance of cell-mediated immunity for protection against T. gondii and have proven useful for the study of immune mechanisms that control infections with nonviral intracellular organisms.
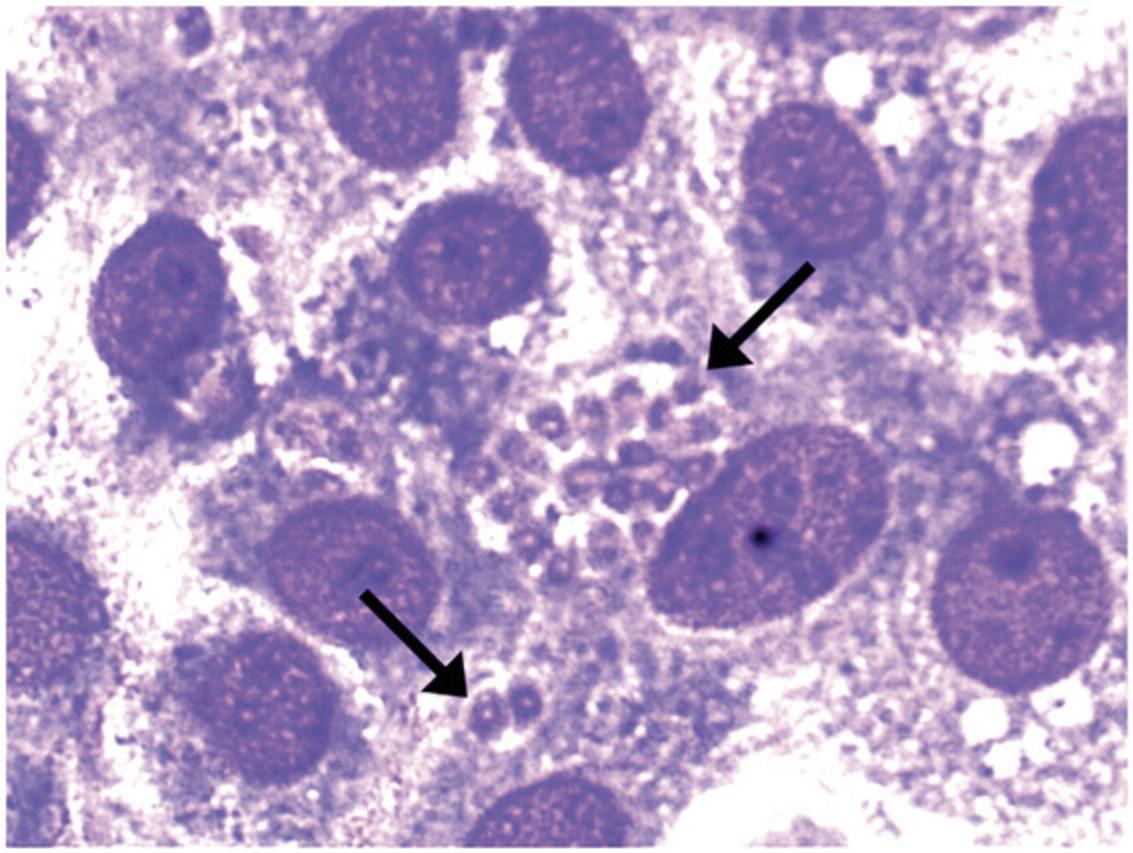
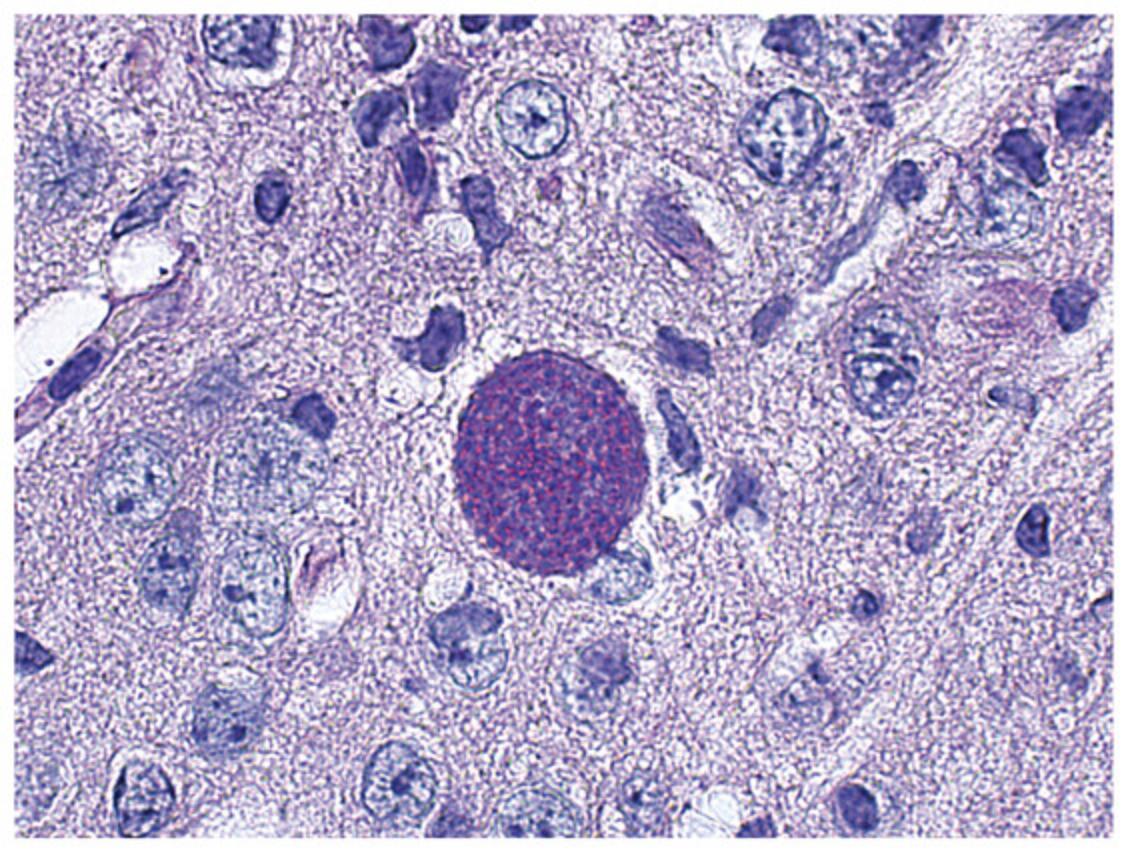
Most strains of T. gondii in North America and Europe fall into 3 genotypes: Type I, II and III (Howe & Sibley, 1995). However, atypical strains with high genetic heterogeneity are common in South America (Dubey et al., 2012). Strains of T. gondii differ in their virulence for mice. The RH strain (Type I) is uniformly virulent (lethal) for naive mice. In contrast, other strains such as ME49 (Type II) are considered relatively avirulent, since they may or may not cause death of naive mice. In addition to the strain of parasite used for infection, the strain of mouse and route of infection are critical for the outcome of infection (Blackwell et al., 1993; Johnson, 1984). The influence of these latter factors is illustrated in Table 1, which lists the susceptibility or resistance of different mouse strains infected with the ME49 strain. We present models that use the ME49 strain of T. gondii since numerous laboratories study immunity to the parasite utilizing tissues cysts of this strain. The use of transgenic parasites that express fluorescent or bioluminescent markers as well as model antigens is also discussed. Strains such as RH have been used to challenge mice after experimental immunization protocols and to test efficacy of antimicrobial regimens against the parasite (Fox & Bzik, 2002).
Acute infection | |||
---|---|---|---|
Strain | Oral | Intraperitoneal | Chronic infection (toxoplasmic encephalitis) |
BALB/c | Resistant | Susceptible | Resistant |
C57BL/6 | Susceptible | Resistant | Susceptible |
CBA/Ca | Unknown | Resistant | Susceptible |
- aStrains of mice are available from The Jackson Laboratory, Charles River Labs, Harlan, and Taconic Farms.
This protocol describes murine models of acute T. gondii infection (see Basic Protocol 1), models of toxoplasmic encephalitis and toxoplasma retinochoroiditis (see Basic Protocol 2), and models to assess invasion of neural tissue (see Basic Protocol 3). T. gondii infection in SCID mice has allowed for the study of T cell-independent mechanisms of defense against intracellular organisms (see Basic Protocol 4). The establishment of the uracil auxotroph strain cps1-1 enabled vaccination studies without concern for parasite replication. This strain does not divide in the absence of uracil and is avirulent in immunocompetent as well as IFN-γ deficient mice (see Basic Protocol 5). The temperature-sensitive mutant strain of T. gondii has also been used in vaccination studies. While the temperature-sensitive mutant ts-4 strain does not form tissue cysts in tissues of immunocompetent mice, it does replicate in the acute phase of infection and can cause disease in immunodeficient mice (see Basic Protocol 5). The EGS strain of T. gondii has allowed for studies of formation of bradyzoite-containing tissue cysts in vitro (Basic Protocol 6). Support protocols present methodology for evaluation of progression of infection and immune response to T. gondii (see Support Protocol 1), maintenance of T. gondii tissue cysts (see Support Protocol 2) and tachyzoites (see Support Protocols 3 and 4), preparation of T. gondii lysate antigens and isolation of tissue cysts (see Support Protocol 5 and 6).
CAUTION: T. gondii is a biosafety level 2 (BSL-2) pathogen. Appropriate precautions should be followed when handling this organism. Pregnant women should not be exposed to live parasites, even if they are seropositive for IgG antibodies to T. gondii. A baseline T. gondii serology should be obtained prior to working with the organism and the serum stored for future reference.
NOTE : All protocols involving animals must be reviewed and approved by the appropriate Animal Care and Use Committee and must follow regulations for the care and use of laboratory animals.
NOTE : Sterility of all materials and solutions that come into contact with samples intended for mouse injection is essential.
Basic Protocol 1: INDUCTION OF ACUTE T. GONDII INFECTION IN MICE
Mice are infected either orally or intraperitoneally with cysts of the ME49 strain of T. gondii. Although the natural route of infection with T. gondii is not intraperitoneal, this model has the advantage of allowing direct access to the site of primary infection for collection of cells and of samples for measurement of cytokine concentrations (see Support Protocol 1). To track infection and study host-parasite interactions in vivo, mice can be infected intraperitoneally with tachyzoites of transgenic parasites that express fluorescent proteins, bioluminescent markers, or a model antigen.
Materials
- Female mice (C57BL/6, CBA/Ca, or Swiss-Webster) chronically infected (>1 month) with the ME49 strain of T. gondii (see Support Protocol 2).
- 70% ethanol
- Phosphate buffered saline (PBS), pH 7.2 (Current Protocols, 2006)
- Tachyzoites of transgenic expressing fluorescent proteins (such as ME49/PTG-GFP, ATCC 50941, dual fluorescent stage specific reporter strain available through L.M. Weiss, louis.weiss@einsteinmed.edu or other transgenic parasites available through D. Roos, droos@sas.upenn.edu), a bioluminescent marker (such as ME49/PTG-firefly luciferase and RH-firefly luciferase available through A. Barragan, antonio.barragan@ki.se), and a model antigen (such as Prugniaud-OVA, available through D. Roos, droos@sas.upenn.edu) (see Support Protocol 3)
- Specific-pathogen-free female mice (6 to 8 weeks old; see Table 1 for strain susceptibility; for maintenance see Donovan & Brown, 2007)
-
- Small curved scissors
- Small forceps
- Mortar and pestle
- 1- or 3-ml syringes with 22-G needles
- Microscope slides and 22-mm × 22-mm coverslips
- 18-G, 1.5-in. (3.8-cm) gavage needle
-
- Additional materials for euthanasia (Donovan & Brown, 2006b), intraperitoneal injection of mice (Donovan & Brown, 2006c), assessment of T. gondii infection (see Support Protocol 1), in vitro culture of T. gondii tachyzoites (see Support Protocol 3), harvest of tachyzoites (see Support Protocol 3).
1.Obtain tissue cysts of the ME49 strain of T. gondii from brains of chronically infected mice (see Support Protocol 2) as follows. After sacrificing T. gondii -infected mice by inhalation of CO2 (Donovan & Brown, 2006b), clean their heads with 70% ethanol. Incise the skin to expose the skull.
2.With a pair of small curved scissors, open the skull to expose the brain. Remove the brain with forceps and place it in a mortar.
3.Grind the brain using a pestle, then add 1 ml PBS per brain. Homogenize the brain suspension using repeated aspiration and ejection from a 1- or 3-ml syringe fitted with a 22-G needle.
4.Determine the concentration of tissue cysts in the brain suspension by transferring three 20-µl samples of the suspension onto microscope slides. Place a 22-mm × 22-mm coverslip over each sample and count the number of cysts in the entire sample under 100× magnification. Using the average of these three numbers, calculate the concentration of cysts per ml of brain suspension.
5.Dilute brain suspension with PBS to adjust to desired final concentration of tissue cysts (50 to 500 per ml).
6a. If the intraperitoneal route of infection is chosen : Administer 0.2 ml of the brain suspension (usually 10 to 20 tissue cysts) to specific-pathogen-free female mice intraperitoneally using a 22-G needle (Donovan & Brown, 2006c).
6b. If the oral route of infection is chosen : Use an 18-G, 1.5 in. gavage needle to administer 0.2 ml cyst suspension (usually 10 to 100 cysts) to specific-pathogen-free female mice.
6c. If transgenic parasites that express fluorescent or bioluminescent markers and/or a model antigen are chosen : Maintain tachyzoites by culturing them in human foreskin fibroblasts as described in Support Protocol 3.Release and harvest tachyzoites (see Support Protocol 3). Remove human cells from tachyzoite suspension (see Support Protocol 3). Inject specific-pathogen-free mice intraperitoneally (Donovan & Brown, 2006c) with tachyzoites resuspended in PBS.
7.Assess progression of infection and/or immune response (see Support Protocol 1).
Basic Protocol 2: MODEL OF TOXOPLASMIC ENCEPHALITIS AND TOXOPLASMA RETINOCHOROIDITIS IN CHRONICALLY INFECTED MICE
Mice are infected with cysts of the ME49 strain (either orally or intraperitoneally) or infected intraperitoneally with tachyzoites of tissue cyst forming strains of the parasite (such as PTG/ME49, Prugniaud) that express fluorescent or bioluminescent markers or model antigens. See Basic Protocol 6 for studies with the EGS strain of T. gondii. Animals can be studied for development of toxoplasmic encephalitis and toxoplasma retinochoroiditis at various times post-infection beginning at 2 weeks, but usually at 4 weeks, after infection.
Materials
- Specific-pathogen-free female mice from a susceptible strain (6 to 8 weeks old; see Table 1 for strain susceptibility; for maintenance see Donovan & Brown, 2007)
- Methoxyflurane (Donovan & Brown, 2006b)
- PBS, pH 7.2 (Current Protocols, 2006)
-
- 30-ml syringe equipped with 22-G needle or straight 24-G gavage needle
- Forceps
- Small curved scissors
-
- Additional reagents and equipment for induction of T. gondii infection in mice and removing mouse brain (see Basic Protocol 1), anesthesia of mice (Donovan & Brown, 2006b), and assessment of T. gondii infection (see Support Protocol 1)
1.Infect mice from a susceptible strain with 10 to 20 cysts of ME49 either intraperitoneally or orally (see Basic Protocol 1) or infect intraperitoneally with transgenic tachyzoites (4 × 102 to 1 × 105) of cyst forming strains (ME49/PTG, Prugniaud).
2.Anesthetize mice with methoxyflurane (Donovan & Brown, 2006b) at various times post-infection (see Support Protocol 1 for clinical assessment). Open the thoracic cavity and perfuse the circulatory system by injecting 20 ml PBS through the left ventricle using a 30-ml syringe with a 22-G needle or a straight 24-G gavage needle, to remove blood from the brain.
3.Remove the brain as described in Basic Protocol 1, then assess progression of toxoplasmic encephalitis (see Support Protocol 1).
4.Remove the eyes by gently pressing around the eye socket until the eye globe is displaced. Open the scissors halfway and gently place them under the eye globe without closing the scissors. Rotate the scissors 90⁰ upwards until the eye globe releases from the socket while continuing to hold the mouse still on a flat surface.
5.Assess progression of infection and/or immune response (see Support Protocol 1).
Basic Protocol 3: ASSESSMENT OF T. GONDII INVASION INTO NEURAL TISSUE
T. gondii reaches the brain and retina hematogenously either as circulating free tachyzoites or within infected leukocytes (Courret et al., 2006; Konradt et al., 2016; Lambert et al., 2006; Lopez Corcino, Portillo & Subauste, 2019; Portillo et al., 2019). Mice are infected intravenously to simulate this route of dissemination. This model provides a useful technique for the study of invasion of the brain and retina. The use of fluorescence or bioluminescent parasites allows for tracing of parasite.
Materials
- Specific-pathogen-free female mice (see Basic Protocol 1)
- Tachyzoites of transgenic expressing fluorescent proteins (such as ME49/PTG-GFP; see Basic Protocol 1)
- Anesthetic agent (ketamine/xylazine cocktail, isoflurane anesthesia system)
-
- Animal restrainer
- Infrared heat lamp or other heating device
- Topical wintergreen oil
- Gauze sponges
- 1-ml syringe with a 27- to 30-G needle
1.When challenging mice with free tachyzoites, harvest parasites from infected human foreskin fibroblasts (see Support Protocol 3) and prepare a suspension of tachyzoites in PBS (2.5 × 106 to 50 × 106 parasites/ml).
2.When challenging mice with infected leukocytes, purify CD11b+ cells from mouse blood on day 7 post-infection with ME49 tissue cysts (Courret et al., 2006), or collect mouse dendritic cells (DC 2.4 cell line) 24 hr post-infection with Type II strain of T. gondii (PTG) (Lopez Corcino, Portillo & Subauste, 2019; Portillo et al., 2019). Prepare suspension of CD11b+ (0.5 × 106 cells/ml) or DC2.4 (2.5 × 106/ml) in PBS.
3.Anesthetize the mouse and place in a restraint device.
4.Gently mix suspensions of tachyzoites or infected cells.
5.Using the heat lamp, gently warm the tail of the restrained animal by placing the lamp roughly 10 to 12 in. above the mouse for a few seconds.
6.To further encourage vasodilation of the tail vein, apply a wintergreen oil-soaked gauze onto the tail vein.
7.Hold the warmed tail flat and locate either of the two lateral tail veins before picking up the pre-filled needle with the dominant hand.
8.Insert the needle a few millimeters into the skin above the visible tail vein with the bevel facing away from the mouse. Push the needle upwards towards the base of the tail and keep the syringe parallel.
9.Slowly inject 0.2 ml of parasite or cell suspension into the tail vein (Diehl et al., 2001). If there is resistance, slowly remove the needle and attempt again by moving further up the tail.
10.After injection, leave the needle in the tail vein for a few seconds to allow injected volume to clear the vein before removing the needle slowly. Apply pressure with gauze to reduce bleeding.
11.Place the anesthetized mouse on a heating pad and monitor recovery for 5 to 10 min.
12.Return the animal back to the original cage and wait ∼24 to 48 hr before euthanizing the mouse.
13.Evaluate progression of infection (see Support Protocol 1).
Basic Protocol 4: T. GONDII INFECTION IN scid/scid (SCID) MICE
SCID mice infected with the ME49 strain of T. gondii die within 2 to 3 weeks post-infection. Sulfadiazine (200 mg per liter of drinking water) can be administered to establish a “chronic” infection. Reactivation of infection and death will occur after discontinuation of sulfadiazine. This has been used as a model that attempts to mimic the pathogenesis of toxoplasmic encephalitis in immunocompromised individuals. Experiments in which T cells and other immune cells are reconstituted into SCID mice can be used to study the role of these cells in the immune response to T. gondii.
Materials
- Specific-pathogen-free female C.B-17S SCID mice (6 to 8 weeks old; The Jackson Laboratory, Charles River Labs, Harlan, or Taconic Farms)
- Sulfadiazine (Sigma, cat. no. S8626)
- Single-cell suspension of lymphoid cells (Kruisbeek, 2001) from either naive mice, T. gondii -immune animals (e.g., mice infected with the ts-4 strain; see Basic Protocol 4), or BALB/c mice chronically infected (>1 month) with the ME 49 strain
-
- Additional reagents and equipment for housing immunocompromised mice (Donovan & Brown, 2007), induction of T. gondii infection in mice (see Basic Protocol 1), injection of mice (Donovan & Brown, 2006c), and analysis of lymphocyte reconstitution in scid/scid mice (Roncarolo & Carballido, 2001)
1.House 6- to 8-week-old female C.B-17S SCID mice in filter-top cages within a laminar-flow hood and feed them autoclaved food and water (Donovan & Brown, 2007).
2.Infect the animals either orally or intraperitoneally with 10 to 20 cysts of the T. gondii ME49 strain (see Basic Protocol 1).
3.If the experiments require a model of chronic infection : Treat animals with sulfadiazine (200 mg per liter of drinking water) starting 3 to 10 days post-infection and continue for 3 weeks.
4.For reconstitution experiments (also see Roncarolo & Carballido, 2001 ) : Administer 1 × 107 to 5 × 107 lymphoid cells obtained from either naive or T. gondii -immune mice intravenously or intraperitoneally (for animal injection techniques see Donovan & Brown, 2006c) 1 day prior to cessation of sulfadiazine (for model of reactivation of “chronic” infection).
5.Assess progression of infection and/or immune response (see Support Protocol 1).
Basic Protocol 5: INFECTION WITH THE URACIL AUXOTROPH STRAIN CPS1-1 OR THE TEMPERATURE-SENSITIVE TS-4 STRAIN OF T. GONDII
The RH-derived cps1-1 strain does not have detectable carbamoyl phosphate synthetase II activity (Fox & Bzik, 2002). While it infects host cells, it does not divide in the absence of uracil, enabling vaccination studies without concern for parasite replication and is avirulent in immunocompetent as well as IFN-γ deficient mice. The ts-4 strain is a mutant, derived from the RH strain of T. gondii , which has an impaired growth rate at 38°C (Waldeland & Frenkel, 1983). This strain replicates in the acute phase of infection and can cause disease in immunodeficient mice although it fails to produce tissue cysts in tissues of immunocompetent mice. The ts-4 strain of T. gondii has also been used in vaccination studies.
Materials
- Tachyzoites of the cps1-1 or ts-4 strain of T. gondii (see Support Protocol 3)
- Specific pathogen-free female mice (6 to 8 weeks old) (see Basic Protocol 1)
- Hanks’ balanced salt solution (HBSS) (Current Protocols, 2006)
- PBS (Current Protocols, 2006)
-
- 1-ml syringes with 26-G needles
-
- Additional materials for in vitro culture of T. gondii tachyzoites (see Support Protocol 3), harvest of tachyzoites (see Support Protocol 3), injection of mice (Donovan & Brown, 2006c), harvest of mouse spleens (Reeves & Reeves, 2001), and preparation of spleen cell suspensions (Kruisbeek, 2001)
1a. Maintain cps1-1 tachyzoites by culturing them in human foreskin fibroblasts as described in Support Protocol 3, using culture medium supplemented with 250 nM uracil. Release and harvest tachyzoites (see Support Protocol 3). Remove human cells from tachyzoite suspension (see Support Protocol 3).
1b. Maintain ts-4 tachyzoites by culturing them in human foreskin fibroblasts as described in Support Protocol 3 but set incubator temperature at 33°C. Release and harvest tachyzoites (see Support Protocol 3). Remove human cells from tachyzoite suspension (see Support Protocol 3).
2a. If using cps1-1 T. gondii , inject specific-pathogen-free mice intraperitoneally or intravenously (Donovan & Brown, 2006c) with tachyzoites in PBS.
2b. If using ts-4 T. gondii , inject specific-pathogen-free mice intraperitoneally (Donovan & Brown, 2006c) with 1 × 104 tachyzoites in PBS. After 4 to 8 weeks, administer a booster injection of 2 × 105 tachyzoites intraperitoneally. Repeat the booster injection after another 2 to 4 weeks.
3.Adoptive transfer studies have been performed using ts-4-immunized mice. Harvest spleens aseptically 1 week after the last booster injection (Reeves & Reeves, 2001). Prepare a suspension of spleen cells in HBSS at 1–2 × 108 cells/ml (Kruisbeek, 2001).
4.Using a 1-ml syringe with a 26-G needle, inject 2–4 × 107 cells (0.2 ml of the suspension prepared in the previous step; see Donovan & Brown, 2006c, for injection technique) into the tail vein of naive mice.
Basic Protocol 6: IN VIVO AND IN VITRO MAINTENANCE OF THE EGS STRAIN OF T. GONDII
The study of the formation of bradyzoite-containing tissue cysts was initially limited to in vivo studies in mice. Subjecting mammalian cells infected with tachyzoites to stress conditions, such as an increase in pH or temperature, treatment with IFN-γ or NO inducers, and chemical stress induced by sodium arsenite results in bradyzoite differentiation in vitro (Lüder & Rahman, 2017). The recombinant Type I/III EGS strain of T. gondii has the unique ability to spontaneously form tissue cysts in vitro, facilitating studies of stage conversion (Paredes-Santos et al., 2013).
Materials
- Specific-pathogen-free female CF-1 or Swiss Webster mice (6 to 8 weeks old; The Jackson Laboratory, Charles River Labs, Harlan, or Taconic Farms)
- Sulfadiazine (Sigma, cat. no. S8626)
- Human foreskin fibroblasts (Hs27; ATCC #CRL 1634; Hs68; ATCC #CRL 1635) or LLC-MK2 (CC7; ATCC)
- Tachyzoites of the T. gondii EGS strain (ATCC PRA-396)
- Complete Dulbecco's Modified Eagle Medium (DMEM), 4.5 g/L glucose (Corning, cat. no. 10-013-CV) containing 1% and 10% heat inactivated fetal bovine serum (FBS), 1× penicillin/streptomycin
- Trypsin
- 70% ethanol
- PBS, pH 7.2 (Current Protocols, 2006)
-
- Scissors
- Small forceps
- 1- and 3-ml syringes with 22-G needles
- 3-μm polycarbonate membrane filter
-
- NOTE : All tissue culture incubations are performed in a humidified 37°C, 5% CO2 incubator unless otherwise noted.
Maintenance of EGS strain in mice
1.Resuspend tachyzoites of the EGS strain in PBS (see Support Protocol 4 and Yokoyama et al., 2012). Inject two or three female Swiss-Webster or CF-1 mice with 105 to 106 tachyzoites per mouse (0.2 ml) intraperitoneally (Ferreira et al., 2001).
2.Euthanize mice at least two weeks post-infection (see Support Protocol 1). Collect brain suspension from an infected mouse (see Basic Protocol 1, steps 1 through 5).
3.Infect the animals orally or intraperitoneally with 10 to 20 cysts of EGS T. gondii strain (see Basic Protocol 1). Treat mice with 0.5 mg/ml of sulfadiazine in the drinking water 48 hr after infection for at least 10 days. Tissue cysts isolation from the brain is described in Support Protocol 6.
In vitro tissue cyst culture from EGS-infected mice
4.To prepare in vitro cultures, infect one to two Swiss-Webster or CF1 mice intraperitoneally with 100 to 200 tissue cysts collected from the maintenance mice.
5.Five to seven days post-infections, collect tachyzoites from the peritoneal fluid (see Support Protocol 4, steps 3-4). Remove mouse cells by using a 3-μm filter. Count the number of tachyzoites.
6.Add tachyzoites to a flask of HFF or LLC-MK2 cells at a multiplicity of infection of 10:1.Allow the parasites to interact with the monolayer. Tissue cysts will form in culture after 96 hr of culture.
7.Maintain tissue cysts in culture by keeping HFF or LLC-MK2 at a confluency under 80% and by changing culture medium often to avoid high parasite burden.
8.Passage tissue cysts containing cells by trypsinization of the monolayer and expansion of cells to new flasks.
9.Parasites collected from a 10-day-old infected culture can be used to infect a fresh monolayer of host cells. Allow parasites to interact with host cells for 1 hr at a multiplicity of infection between 5:1 and 10:1.
Support Protocol 1: ASSESSMENT OF PROGRESSION OF INFECTION AND IMMUNE RESPONSE TO T. GONDII
Progression of T. gondii infection and immune response to the parasite can be monitored by the following parameters: signs of illness and time until death; histopathology to assess inflammatory changes and changes in tissue architecture induced by the infection as well as to establish the presence of the parasite in tissues (immunohistochemistry); tissue levels of parasite DNA and mRNA for genes expressed specifically in the tachyzoite stage (parasite load); bioluminescence imaging to examine in real time the progression of infection; cytokine levels in serum and other body fluids; mRNA levels of cytokines and other molecules involved in the immune response against the parasite; flow cytometry to detect changes in the phenotypic composition of leukocytes in different organs, cell activation and T cell expansion; lymphocyte proliferation and cytokine production after in vitro stimulation with T. gondii ; serum titers of T. gondii antibodies. Depending on the purpose of the experiment, the investigator should choose one or more of these parameters as appropriate, as well as the timing of evaluation after infection (see Anticipated Results).
Clinical Assessment
Examine animals twice daily. Record signs of illness (piloerection, huddling, tachypnea, hunched posture, weight loss) as well as mortality rate and survival time. When studying toxoplasmic encephalitis, particular attention should be paid to the period between 4 to 10 weeks post-infection.
Assessment by Histopathology and Immunohistochemistry
Materials
- T. gondii -infected mice (see Basic Protocol 1, 2, or 3)
- Fixative (see recipe)
- Anti-T. gondii polyclonal antibody (BioGenex Laboratories)
- Anti-T. gondii SAG1 monoclonal antibody (Invitrogen)
- Dolichus biflorus agglutinin (DBA; VectorLabs; Deshmukh et al., 2021)
-
- Additional reagents and equipment for euthanasia of mice (Donovan & Brown, 2006b), collection of lymphoid organs (Reeves & Reeves, 2001), and immunohistochemistry (Hofman & Taylor, 2013)
1a. For model of acute T. gondii infection : At different times during the first or second week post-infection, euthanize mice by CO2 inhalation (Donovan & Brown, 2006b) and remove the liver, lung, heart, small and large intestine, and mesenteric lymph nodes (in the case of oral infection) for histopathology.
1b. For models of toxoplasmic encephalitis and toxoplasma retinochoroiditis : Euthanize mice by CO2 inhalation (Donovan & Brown, 2006b) and remove the brain and eyes at least 2 weeks post-infection (usually 4 weeks) or at weekly intervals after the cessation of sulfadiazine when using the model of reactivation in SCID mice (see Basic Protocol 3).
2.Fix portions of these organs overnight at room temperature in a solution containing 10% formalin, 70% ethanol, and 5% acetic acid.
3.Prepare two to four 5-µm-thick sections (with 50 to 100 µm distance between sections) of each organ (Hofman & Taylor, 2013).
4.Stain sections with hematoxylin/eosin to study inflammatory changes in the brain and retina as well as changes in the architecture of the retina and choroid. Process the brain for immunohistochemistry using an anti -T gondii polyclonal antibody to detect T. gondii organisms and antigen (all procedures described in Roederer, 2002). Determine the number of parasitophorous vacuoles that contain T. gondii. Use SAG1 monoclonal antibody (tachyzoite-stage) or Dolichus biflorus aggulutinin (DBA; stains the wall of bradyzoite-containing tissue cysts stage) to evaluate different stages of T. gondii.
Measurement of B1 Gene of T. gondii by Real Time Quantitative PCR
Materials
- T. gondii -infected mice (see Basic Protocol 1, 2, or 3)
- Primers (Bretagne et al., 1993):
- Sense primer: 5′-AACGGGCGAGTAGCACCTGAGGAGA-3′ (1793-1817)
- Antisense primer: 5′-TGGGTCTACGTCGATGGCATGACAAC-3′ (1907-1881)
-
- Additional reagents and equipment for isolation of DNA and PCR
1a. For models of acute T. gondii infection : Remove livers and lungs during the first or second week post-infection.
1b. For models of toxoplasmic encephalitis or toxoplasma retinochoroiditis : Remove brains or eyes at least 2 weeks post-infection.
2.Isolate genomic DNA. Determine the levels of DNA for the B1 gene by subjecting DNA to real time PCR using SYBR GREEN PCR Master Mix. Samples are run in triplicate and normalized against L32 or actin. Use the following PCR program:
Initial step: | 10 min | 95oC | (denaturation) |
40 cycles: | 15 s | 95°C | (denaturation) |
30 s | 60°C | (annealing) | |
30 s | 72°C | (extension) |
RT-PCR to Measure mRNA Levels of Genes that Encode Tachyzoite-specific Antigens SAG-1, SAG-2 and Bradyzoite-Specific Antigen BAG-1
Materials
-
T. gondii -infected mice (see Basic Protocol 1, 2, or 3)
-
SAG-1/SAG-2 primers and internal probes (Gazzinelli, Eltoum et al., 1993)
-
BAG-1 primers (Mahittikorn et al., 2010)
-
For SAG-1 :
- Sense primer: 5′-ATGTCGGTTTCGCTGCACTTC-3′ (311-334)
- Antisense primer: 5′-TCACGCGACACAAGCTGCGATAGAGCC-3′ (1295-1321)
- Probe: 5′-GCTAGTCTCGACACGGCAGGC-3′
-
For SAG-2 :
- Sense primer: 5′-ATGAGTTTCTCAAAGACCACGAGCCTAGC-3′ (182-210)
- Antisense primer: 5′-TTACACAAACGTGATCAACAAACCTGCGAGACC-3′ (710-742)
- Probe: 5′-CGAGGAAGTTGACGACTGTCC-3′
-
For BAG-1 :
- Sense primer: 5′-AGTCGACAACGGAGCCATCGTTATC-3′
- Antisense primer: 5′-ACCTTGATCGTGACACGTAGAACGC-3′
-
Additional reagents and equipment for isolation of mRNA (Ribaudo et al., 2001) and RT-PCR (James, 2001)
1a. For models of acute T. gondii infection : Remove livers and lungs during the first or second week post-infection.
1b. For models of toxoplasmic encephalitis and toxoplasma retinochoroiditis : Remove brains and eyes at least 2 weeks post-infection.
2.Isolate mRNA (Ribaudo et al., 2001). Using semiquantitative reverse transcription-polymerase chain reaction (RT-PCR; also see James, 2001), determine the levels of mRNA for genes expressed specifically in the tachyzoite stage (SAG-1 and/or SAG-2 ; Gazzinelli, Eltoum et al., 1993). Use the following PCR program for the SAG-1 and SAG-2 primers and oligonucleotide probes:
Initial step: | 3 min | 95°C | (denaturation) |
35 cycles: | 1 min | 95°C | (denaturation) |
1 min 2 min |
54°C 72°C |
(annealing) (extension) |
Assessment of Toxoplasmic Encephalitis by Cyst Enumeration
At least 2 weeks post-infection of immunocompetent mice, or at weekly intervals after cessation of sulfadiazine when using the model of reactivation in SCID mice, the number of cysts in the brain can be enumerated to assess infections. The number of cysts present appears to be an important factor in the development of toxoplasmic encephalitis. To determine the number of cysts present, homogenize half of the brain in PBS using a mortar and pestle and count cysts using three 20-µl samples per brain (see Basic Protocol 1 for details of these procedures). The final number of cysts per brain will represent the average of those calculated with each sample. Tissue cysts can also be counted in brain sections stained with anti-T. gondii antibodies. The number of tissue cysts can be determined by examining 100 randomly selected high power fields. Animals with toxoplasmic encephalitis can exhibit not only tissue cysts but also tachyzoites within parasitophorous vacuoles.
In Vivo Assessment of Parasite Growth by Bioluminescence Imaging
Bioluminescence imaging can be utilized as a tool for serial and non-lethal quantification of T. gondii in vivo (Dellacasa-Lindberg et al., 2007; Hitziger et al., 2005). Type I and Type II parasites that express a construct that encodes firefly luciferase have been developed. Exposure to D-luciferin, the substrate for firefly luciferase, results in light emission that can be detected externally by photon detectors such as charge-coupled device (CCD) cameras. D-luciferin is non-toxic, non-immunogenic compound that diffuses widely within the mouse and can even cross the blood brain barrier. At different times after infection with parasites that encode firefly luciferase, mice receive an intraperitoneal injection of 1.5 to 3 mg of D-luciferin potassium salt. Mice are anesthetized and photonic emission is quantitated. There is a direct correlation between photon flux levels and parasite load allowing for in vivo parasite quantification during acute T. gondii infection and recrudescence of infection in the central nervous system.
In Vivo Assessment of T. gondii Stage Conversion Using Dual Fluorescent Stage Specific Reporter Strains of the Parasite
Initial studies of the formation of bradyzoite-containing tissue cysts were limited to brains collected from chronically infected mice. In vitro and in vivo studies of stage conversion have been facilitated by the development of dual fluorescent stage-specific reporter strains of T. gondii (Paredes-Santos et al., 2016; Unno et al., 2009; Zhang et al., 2013). These include the Type II PLK strain and the EGS strain of T. gondii that stably express fluorescent proteins under the control of tachyzoite-specific SAG-1 promoter and bradyzoite-specific BAG-1 promoter. These strains have been used to examine stage conversion in infected mice. In addition, they can be used to examine reactivation of chronic infection in chronically infected mice given dexamethasone (10 mg/L) in their drinking water (Unno et al., 2009; Zhang et al., 2013).
Measurement of Cytokine Expression and Lymphocyte Proliferation to Assess Acute Toxoplasmic Infection or Toxoplasmic Encephalitis
Assessment of acute toxoplasmic infection (see Basic Protocol 1) can be performed at varying times during the first two weeks post-infection by measuring serum cytokine levels. Collect serum samples pre- and post-infection either through tail bleeds (Donovan & Brown, 2006a), or at the time of euthanasia, and store at −70°C. These serum samples as well as peritoneal lavage fluid can be used to measure levels of cytokines and chemokines such as IFN-γ, TNF-α, IL-12, IL-6, IL-10 and MCP-1 by ELISA or bead array kits (Chapter 6). Alternatively, mRNA levels of IFN-γ, TNF- α, IL-1, IL-2, IL-4, IL-6, IL-10, IL-12p35, IL-12/23p40, TGF-β, IL-27 (IL-27p28, EBI3), MCP-1 can be measured by real time quantitative PCR using RNA prepared from various tissue samples including spleen, liver, lung, and peritoneal cells during the first two weeks post-infection (James, 2001). Cytokine and nitric oxide production as well as T cell proliferative responses may also be studied using flow cytometry after in vitro stimulation of splenocytes, lymph node or peritoneal cells with T. gondii antigenic preparations (tachyzoites or parasite lysate antigens) and either concanavalin A, anti- CD3/anti-CD28 mAbs or LPS as controls. Optimal concentrations of antigenic preparations and duration of in vitro stimulation need to be determined by the investigator. Cytokine production can be assessed by ELISA, bead array kits or flow cytometry (to examine intracellular cytokine levels). In vivo T cell replication can be examined by adoptively transferring CFSE- labeled T cells. The use of T. gondii that express model antigen such as OVA, OVA-specific T cell receptor transgenic mice and MHC class I tetramers to detect OVA-specific CD8+ T cells enable the study of in vivo expansion of OVA-reactive T cells using flow cytometry.
Toxoplasmic encephalitis and toxoplasma retinochoroiditis (see Basic Protocol 2) can be assessed at least 2 weeks post-infection by measuring brain and eye levels of mRNA for the above-mentioned molecules (James, 2001). Brain mononuclear cells can be isolated and used for flow cytometry as well as stimulated with T. gondii antigenic preparations as described for acute toxoplasmic infection. If using the toxoplasma reactivation model in SCID mice (see Basic Protocol 3), brains can be collected for real time quantitative PCR analysis of cytokine expression at weekly intervals post cessation of sulfadiazine. When preparing brain or eyes for evaluation of cytokine transcripts, it is important to perfuse the circulatory system with PBS as described in Basic Protocol 2, since contamination with blood may yield erroneous results.
Assessment of Host-Parasite Interactions In Vivo and Ex Vivo Using Multiphoton Microscopy
The development of genetically encoded fluorescent reporters has enabled the in vivo tracking of cells within living tissues using multiphoton microscopy. Host-T. gondii interactions have been studied using parasites that express OVA peptide secreted into the parasitophorous vacuole plus a fluorescent label together with OVA-specific T cell receptor transgenic mice (Chtanova et al., 2009; John et al., 2009; Schaeffer et al., 2009; Wilson et al., 2009). To facilitate tracking of antigen-specific T cells, mice are generated in which only a subset of T cells are OVA-specific and express a fluorescent marker. This can be accomplished by adoptive transfer of TCR transgenic T cells to adult mice or by administration of bone marrow cells from transgenic mice into newborn animals (hematopoietic chimeric mice). These models can be used for in vivo imaging of cellular interactions during primary or recall immune responses in lymphoid organs as well as cellular interactions in the brain of T. gondii infected mice. Lymphoid organs (typically draining lymph nodes) or brains are removed from infected mice euthanized by CO2 asphyxiation. Lymph nodes or slices of brains can be placed in an imaging chamber and perfused with medium at 37°C bubbled with 95% O2 and 5% CO2. Organs are then subjected to multiphoton microscopy.
For studies of primary immune responses to T. gondii infection, naive mice have been adoptively transferred with TCR transgenic T cells (OT1GFP) followed by intraperitoneal infection with transgenic parasites (1 × 104 PruOVA tachyzoites) followed by isolation of draining lymph nodes (John et al., 2009). For studies of recall immunity, hematopoietic chimeric mice immunized with PruOVA (1 × 106 irradiated parasites intraperitoneally) received a subcutaneous challenge with 5 × 106 parasites followed by collection of draining lymph nodes (Chtanova et al., 2009). For studies of cell interactions in the brain, mice that express OT1GFP or OT1CFP T cells via adoptive transfer or generation of hematopoietic chimeras have been infected with PruOVA (4 × 102 to 1 × 104 parasites intraperitoneally) followed by collection of infected brains (Schaeffer et al., 2009; Wilson et al., 2009). The availability of mice that express fluorescent proteins under promoters specific for various cell types or cytokines also allows the in vivo tracking of other aspects of the immune response against the parasite. For studies of parasite trafficking through the blood brain barrier, two-photon microscopy intravital imaging was used in transgenic mice expressing EGFP-Claudin 5 under a Tie2 endothelial cell promoter infected with tdTomato-expressing Type II T. gondii (Schneider et al., 2022). To view immune cell infiltration, anti-CD45 antibodies can be administered intravenously 24 hr before beginning microscopy (Schneider et al., 2022). Whole brains from infected mice can be removed and examined ex vivo using two-photon microscopy (Olivera et al., 2021).
Assessment of Antibody Responses
Collect serum either through tail bleeds (Donovan & Brown, 2006a) or at the time of euthanasia and store at −70°C. Development of T. gondii antibodies can be studied in sera collected between days 7 and 14.Specific IgG antibodies can be detected using the agglutination test (Desmonts & Remington, 1980). IgG titers can be detected in murine sera by ELISA (Lopez Corcino, Gonzalez Ferrer et al., 2019).
Assessment of In Vivo Parasite Dissemination
Depending on the experimental goals, at various times post-infection, leukocyte distribution can be assessed by flow cytometry in various lymphoid tissues including spleen, peritoneum, intestinal mucosa, and mesenteric lymph nodes, as well as other lymph nodes and brain. Parasite load in various organs can be examined using B1 gene qPCR (see above in Support Protocol 1). Multiphoton imaging through either thinned or open skill surgery can be performed to view infection of and egress from endothelial cells as well as breaching of the blood brain barrier (Konradt et al., 2016). Focal areas of parasite infection can be determined through immunohistochemistry of brain sections by staining endothelial cells using Tomato Lectin or anti-CD31 mAb and staining T. gondii using polyclonal or anti-SAG1 antibodies, or the use of fluorescent parasites (see above in Support Protocol 1) (Lopez Corcino, Portillo & Subauste, 2019; Portillo et al., 2019). Flow cytometry can also be used as a method of quantification and detection of fluorescent T. gondii -infected endothelial cells (CD31+ and CD102+) (Konradt et al., 2016).
Support Protocol 2: MAINTENANCE OF A BANK OF T. GONDII CYSTS OF THE ME49 STRAIN
A bank of ME49 T. gondii is established by infecting Swiss-Webster mice. These animals survive infection. To obtain larger numbers of cysts, this bank may be expanded into either C57BL/6 or CBA/Ca mice prior to the anticipated need for cysts for experiments.
Additional Materials (also see Basic Protocol 1)
- Specific-pathogen-free Swiss-Webster, C57BL/6, and CBA/Ca female mice (6 to 8 weeks old; Charles River Labs, Harlan, Taconic Farms, or The Jackson Laboratory)
- Mouse brains containing tissue cysts of the T. gondii ME49 strain (available through: C. Subauste, carlos.subauste@case.edu ; C. Hunter, chunter@vet.upenn.edu ; E. Denkers, eyd1@cornell.edu)
-
- Additional reagents and equipment for injection of mice (Donovan & Brown, 2006c) and isolation of tissue cysts from mouse brain (see Basic Protocol 1)
1.Obtain suspension of tissue cysts from mouse brain (see Basic Protocol 1).
2.Inject 10 to 20 Swiss-Webster mice intraperitoneally (Donovan & Brown, 2006c) each with 10 to 20 cysts of the ME49 strain.
3.At least 4 weeks before the anticipated need for ME49 cysts, euthanize an infected Swiss-Webster mouse (one mouse is usually sufficient for most purposes) and obtain a suspension of brain cysts (see Basic Protocol 1, steps 1 to 5).
4.Infect C57BL/6 or CBA/Ca mice with 10 to 20 cysts intraperitoneally (see Basic Protocol 1, step 6a).
Support Protocol 3: MAINTENANCE OF T. GONDII TACHYZOITES USING HUMAN FORESKIN FIBROBLASTS
In this protocol, tachyzoites of T. gondii are grown in vitro within mammalian cells. Human foreskin fibroblasts (HFF) are most commonly used.
Materials
- Human foreskin fibroblasts (Hs27; ATCC #CRL 1634; Hs68; ATCC #CRL 1635)
- Tachyzoites of the T. gondii RH strain (ATCC #50174 or NIH AIDS Research and Reference Reagent Program #2859; phone 301-340-0245), ts-4 strain (ATCC #40050), cps1-1 T. gondii (available through D. Bzik , David.J.Bzik@Dartmouth.edu) or transgenic parasites that express a bioluminescent marker (ME49/PTG-firefly luciferase and RH-firefly luciferase, available through A. Barragan, antonio.barragan@ki.se), fluorescent proteins (ME49/PTG-GFP, ATCC 50941; or other transgenic parasites available through D. Roos, droos@sas.upenn.edu) or a model antigen (Prugniaud-OVA, available through D. Roos, droos@sas.upenn.edu)
- Complete DMEM medium, 4.5 g/L glucose (Corning, cat. no. 10-013-CV) containing 1% and 10% heat-inactivated fetal bovine serum
- Uracil (Sigma, cat. no. U1128; to grow cps1-1 T. gondii)
- Chloramphenicol (Sigma, cat. no. C3175; to grow transgenic parasites that express chloramphenicol acetyltransferase as selectable marker)
- Pyrimethamine (Tocris, cat. no. 3918; to grow transgenic parasites that express dihydrofolate reductase as selectable marker
-
- Tissue culture flasks (either 25-cm2 or 75-cm2)
- Inverted phase-contrast microscope
- Cell scraper
- Tabletop centrifuge
- 3.0-µm filter
-
- NOTE : All tissue culture incubations are performed in a humidified 37°C, 5% CO2 incubator unless otherwise noted.
- NOTE : Culture human foreskin fibroblasts in complete DMEM containing 10% FBS in 25- or 75-cm2 tissue culture flasks.
1.Resuspend T. gondii tachyzoites in complete DMEM with 4.5 g/L glucose containing 1% fetal bovine serum (5 ml for a 25-cm2 tissue culture flask or 15 ml for a 75-cm2 flask). Use DMEM containing 10% fetal bovine serum when growing tachyzoites of the Prugniaud strain.
2.Aspirate and discard culture medium from a flask containing a confluent monolayer of human foreskin fibroblasts.
3.Add the medium containing the desired number of tachyzoites, prepared in step 2, to the monolayer and incubate at 37°C (for RH and Prugniaud strains) or 33°C (for temperature-sensitive mutant ts-4 strain).
4.Examine monolayers daily using an inverted phase-contrast microscope.
5.When the majority of fibroblasts contain multiple intracellular tachyzoites, release organisms by destroying the monolayer using a cell scraper.
6.Purify parasites, spin the suspension of parasites and host cells 5 min at 70 × g , 4°C. Parasites will remain in the supernatant while cellular debris will pellet. Transfer the supernatant to new tube and spin 10 min at 900 × g , 4°C. Filtration through a 3.0-µm filter can also be used to purify parasites. Wash parasites in PBS prior to injection into mice.
Support Protocol 4: MAINTENANCE OF T. GONDII TACHYZOITES IN MICE
An alternative protocol for maintenance of tachyzoites of the RH strain is to passage them into mice. Whether the protocol below or Support Protocol 3 is chosen depends on personal preference and facilities available. The procedure described below (intraperitoneal injection) is not an ideal approach for obtaining tachyzoites for experimental use when starting with cysts of the ME49 strain or tachyzoites of the ts-4 strain.
Materials
- Specific-pathogen-free Swiss-Webster female mice (6 to 8 weeks old; Charles River Laboratories, Harlan, or Taconic Farms)
- Tachyzoites of the T. gondii RH strain (ATCC #50174 or NIH AIDS Research and Reference Reagent Program #2859)
- 70% ethanol
- PBS (Current Protocols, 2006)
-
- Tabletop centrifuge
- 1- and 3-ml syringes with 22-G needles
- 50-ml centrifuge tubes
- Microscope slides and 18-mm coverslips
-
- Additional reagents and equipment for thawing or cryopreserved cells (Yokoyama et al., 2012), euthanasia of mice (Donovan & Brown, 2006b), peritoneal lavage of mice (Gonçalves & Mosser, 2015, Basic Protocol 1), assessment of T. gondii infection (see Support Protocol 1), and injection of mice (Donovan & Brown, 2006c)
1.Thaw vial containing tachyzoites (Yokoyama et al., 2012). Centrifuge tachyzoite suspension 10 min at 500 × g , 4°C, and aspirate supernatant. Resuspend in 0.4 ml PBS and inject intraperitoneally half the volume into each of two Swiss-Webster mice.
2.Examine mice twice a day for evidence of illness (see Support Protocol 1). Euthanize animals by CO2 asphyxiation (Donovan & Brown, 2006b) when they exhibit clinical evidence of illness.
3.Clean abdomen with 70% ethanol and incise abdominal wall. Perform peritoneal cavity lavage with 2 to 3 ml of PBS using a 3-ml syringe attached to a 22-G needle (see Gonçalves & Mosser, 2015, Basic Protocol 1 for technique, but use PBS as lavage fluid).
4.Withdraw peritoneal fluid and transfer to a 50-ml centrifuge tube.
5.Make a 1:10 dilution of an aliquot of peritoneal fluid by transferring 0.1 ml of peritoneal fluid to a microcentrifuge tube that contains 0.9 ml PBS.
6.With a 1-ml syringe attached to a 22-G needle, dispense a drop of the diluted peritoneal fluid onto a microscope slide and cover with an 18-mm coverslip. Count the number of tachyzoites per field at 400× magnification.
For 2-day transfer
7a. Inject mice intraperitoneally (Donovan & Brown, 2006c) with 0.2 ml of the original peritoneal fluid preparation if the sample that was diluted 1:10 contains 18 to 20 tachyzoites per field. If the organism count does not fall within that range, increase the volume of injection, or dilute the original peritoneal fluid preparation accordingly.
8a. Euthanize mice (Donovan & Brown, 2006b) 2 days after infection and harvest tachyzoites as in steps 3 to 4.
For 3-day transfer
7b. Inject mice intraperitoneally (Donovan & Brown, 2006c) with 0.2 ml of a diluted peritoneal fluid preparation that contains 12 to 18 tachyzoites per 400× field.
8b. Euthanize mice (Donovan & Brown, 2006b) 3 days after infection and harvest tachyzoites as in steps 3 to 4.
Support Protocol 5: PREPARATION OF T. GONDII LYSATE ANTIGENS
T. gondii antigen preparations are obtained by lysing tachyzoites either through repeated freeze- thaw cycles or by sonication. Although both methods yield preparations that trigger lymphocyte proliferation and cytokine production, the investigator should keep in mind that antigenic composition of these preparations may differ. Provided that the reagents used are low in endotoxin, the protocol presented here is useful in situations where it is desirable to obtain antigenic preparations with low concentrations of endotoxin.
Materials
- Tachyzoites of the RH strain of T. gondii in human foreskin fibroblast culture (see Support Protocol 3; infect at least five 75-cm2 flasks)
- 10× and 1× PBS (Current Protocols, 2006), pH 7.2, 4°C
-
- Tabletop centrifuge and appropriate 15- and 50-ml centrifuge tubes
- Microscope slides and coverslips
- Liquid nitrogen or dry ice/ethanol bath
1.Transfer lysed human foreskin fibroblast culture containing tachyzoites of the RH strain (see Support Protocol 3) to 50-ml centrifuge tube.
2.Centrifuge tachyzoite suspension 10 min at 900 × g , 4°C, and aspirate supernatant.
3.Resuspend pellet in 10 ml of 1× PBS, pH 7.2, and centrifuge 5 min at 70 × g , 4°C, to separate mammalian cells.
4.Collect supernatant, transfer to a fresh centrifuge tube, and centrifuge 10 min at 900 × g , 4°C. Aspirate supernatant.
5.Resuspend the pellet in 1 ml of 1× PBS. Collect 10 µl of T. gondii suspension and dilute with 90 µl of 1× PBS. Place a 9-µl drop on a microscope slide. Cover with a coverslip, count tachyzoites at 400×, and check for contamination with mammalian cells. Resuspend pellet in 10 ml of 1× PBS, then centrifuge 10 min at 900 × g , 4°C, and aspirate the supernatant.
6.Resuspend pellet in sterile distilled water at 4°C so that tachyzoites are a concentration of at least 5 × 108 per ml.
7.Quickly freeze suspension in liquid nitrogen or dry ice/ethanol bath, then rapidly thaw in a 37°C water bath. Repeat at least twice.
8.Reconstitute with sufficient 4°C 10× PBS to bring the lysate to a concentration of 1× PBS. Centrifuge 20 min at 900 × g , 4°C.
9.Collect supernatant, divide into aliquots and transfer immediately to a −70°C freezer.
Support Protocol 6: ISOLATION OF T. GONDII TISSUE CYSTS FROM BRAIN
Our knowledge regarding the processes and mechanisms that regulate tissue cyst formation is still incomplete. Purification of tissue cysts allows for the observation of cysts with variable composition. For example, emergent tissue cysts with premature cyst walls can be fragile and more prone to disruption (Watts et al., 2015). Tissue cyst size or bradyzoite compositions can be directly correlated with the density of the Percoll gradient, a relevant tool for the assessment of the progression of atypical strains during chronic infection, influence of drug treatments, and impact of parasite mutants (Watts et al., 2015; Watts et al., 2017). Additionally, this method can be used to isolate tissue cysts from brain or tissue homogenates from strains within the population of humans and animals (Puvanesuaran et al., 2012).
Materials
- PBS, pH 7.2 (Current Protocols, 2006)
- 70% ethanol
- Percoll (Cytiva, cat. no. 17089101)
- 10× PBS (Current Protocols, 2006)
-
- Scissors
- Forceps
- Mortar and pestle
- 1- or 3-ml syringes with 22-G needles
- Microscope slides and 22-mm × 22-mm coverslips
- 18-G, 1.5-in. (3.8-cm) gavage needle
- Sterile 15- and 50 ml- conical tubes
- Serological pipette or 16-G needle attached to a 5-ml syringe
- Tabletop centrifuge with swinging bucket rotor
- 1.5-ml Eppendorf tubes
1.Harvest and homogenize brain from a mouse chronically infected with T. gondii and dilute homogenate to 4.5 ml of 1× PBS per brain (Basic Protocol 1, steps 1 through 5).
2.Prepare three individual Percoll stock solutions in 15 ml conical tubes:
-
9 ml per brain of 90% Percoll in PBS (0.9 ml 10× PBS + 8.1 ml Percoll).
-
4.5 ml per brain of 40% Percoll in PBS (0.45 ml 10× PBS + 2.25 ml sterile ddH2O + 1.8 ml Percoll).
-
4.5 ml per brain of 20% Percoll in PBS (0.45 ml 10× PBS + 3.15 ml ddH2O + 0.9 ml Percoll).
3.For each brain, transfer the 90% Percoll gradient (9 ml) into a 50-ml conical tube.
4.Using a serological pipette or 16-G needle attached to a 5-ml syringe, draw up 4.5 ml of 40% Percoll and gently layer it on top of the 90% Percoll solution by holding the tube at a 45-degree angle and running the solution along the wall of the 50-ml conical tube.
5.Using this same technique, draw up 4.5 ml of the 20% Percoll solution and layer it on top of the 90% to 40% Percoll solutions.
6.Draw up 4.5 ml of the brain homogenate using a 16-G needle on a 5-ml syringe or a serological pipette. Layer the brain homogenate on the top of the 20% Percoll gradient as described above.
7.Transfer the Percoll gradient into a centrifuge bucket in a cool (4°C) tabletop centrifuge. Centrifuge gradient 15 to 18 min at 1000 × g , 4°C, with full break setting.
8.Prepare ∼18 1.5-ml Eppendorf microcentrifuge tubes to collect 1 ml gradient fractions.
9.A distinct RBC layer will be present at the 90/40% Percoll boundary, and the brain homogenate will remain on the top layer. The tissue cysts are concentrated between these two areas.
10.Remove the brain homogenate from the top of the gradient using a broken serological pipette.
11.Once the brain homogenate is removed, gently pipette up 1 ml of the tissue cyst-containing gradient layer. Place each 1 ml faction into a designated numbered tube.
12.Count the number of tissue cysts in each fraction (Basic Protocol 1, step 4).
13.For further experimentation, dilute fraction samples with PBS (5-fold dilution) and centrifuge 10 min at 1000 × g , 4°C, to pellet tissue cysts.
REAGENTS AND SOLUTIONS
Use deionized, distilled water in all recipes and protocol steps. For common stock solutions, see Current Protocols (2006).
Fixative
- 10% (v/v) 37% formalin
- 70% (v/v) ethanol
- 5% (v/v) glacial acetic acid
- 15% H2O
- Prepare fresh before each use.
COMMENTARY
Background Information
Toxoplasma gondii is found worldwide and is estimated to infect roughly one-third of the world population (Halonen & Weiss, 2014). Seroprevalence rates of T. gondii vary widely with endemic regions of the world, such as Brazil, reaching seropositive rates as high as 80% (Fig 3). Most infections are asymptomatic in humans; however, ocular toxoplasmosis can occur in a subset of immunocompetent hosts (Butler et al., 2012). Up to a quarter of these patients develop blindness in at least one eye. Immunocompromised hosts are at risk for severe disease generally characterized by toxoplasmic encephalitis or systemic toxoplasmosis. The severity of infection can be influenced by the genotype of the parasite (Xiao & Yolken, 2016). Type II strains are the most common ones in North America and Europe and generally result in asymptomatic infections. In contrast, atypical strains common in South America are more likely to cause disease (de Lima Bessa et al., 2021). Virulence of T. gondii strains is determined using mouse models of infection. Type I strains are considered highly virulent to mice with high mortality rates within 10 days post-infection (<10 tachyzoites) while Type II and III strains are considered avirulent with high survival rates post-infection (>1000 tachyzoites).
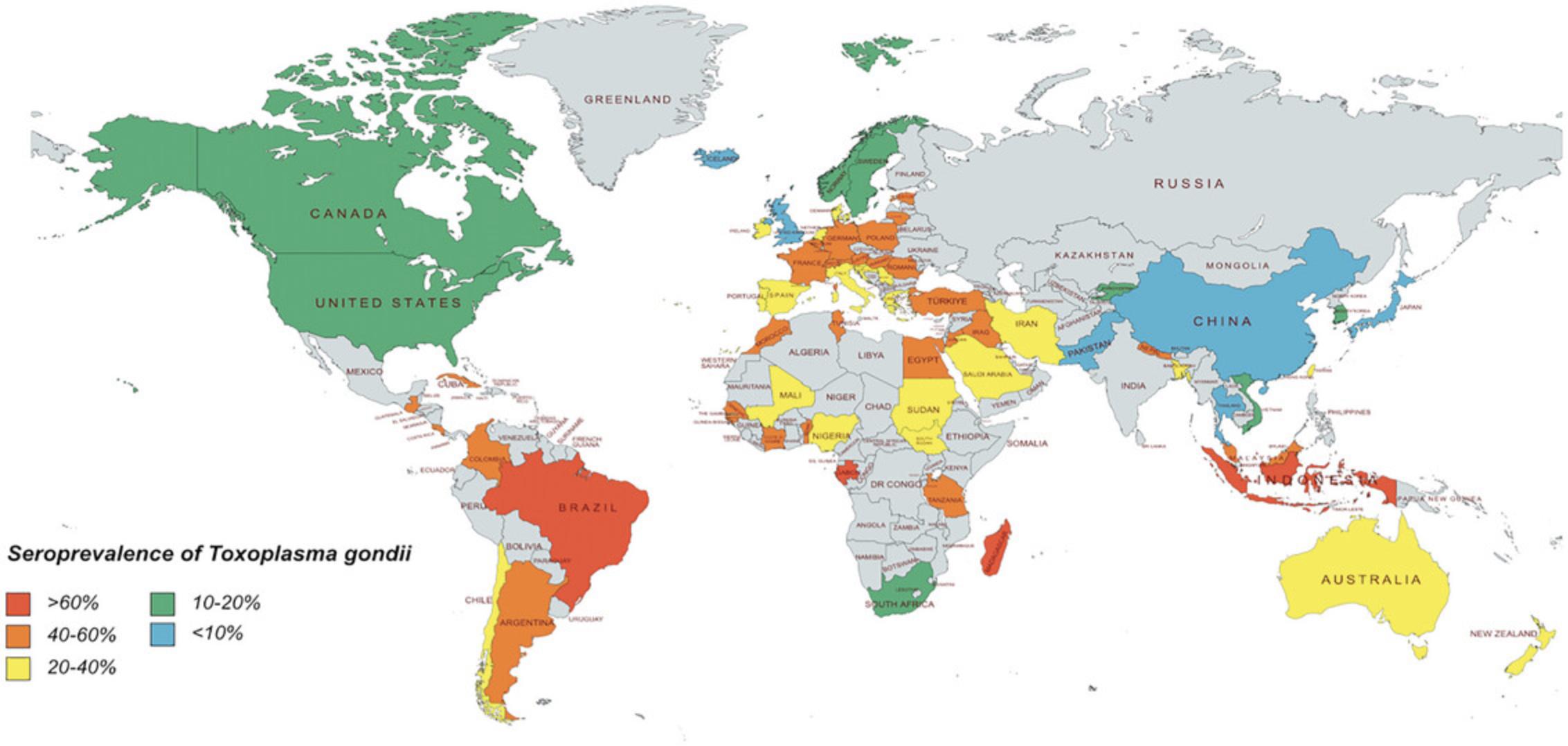
T. gondii exists in three forms: the tachyzoite, which is the asexual, fast-replicating invasive form; the tissue cyst (containing bradyzoites), which persists in tissues of infected hosts during the slow-replicating, chronic phase of the infection; and the oocyst (containing sporozoites), which is produced during the sexual cycle in the intestine of cats (the definitive host). The extraintestinal asexual cycle is present in all incidental hosts and in cats. After ingestion of tissue cysts or oocysts, either bradyzoites or sporozoites, respectively, are released into the intestinal lumen, where they invade surrounding cells, transform into tachyzoites, and disseminate throughout the body via the blood and lymphatics. The tachyzoite requires an intracellular habitat for survival and can infect almost all mammalian cell types. Tachyzoites are found in tissues during the acute stage of the infection or during reactivation of the chronic (latent) infection. Approximately 10 to 14 days after infection, tachyzoites transition into bradyzoite-containing cysts in the tissues of the infected host. This phenomenon appears to be associated with development of immunity and acquisition of resistance to rechallenge with the parasite. Tissue cysts, which contain bradyzoites, can be found in all organs but have a propensity to be localize in myocardial, skeletal, and smooth muscle, and the central nervous system. Tissue cysts appear to persist for the life of the infected host. The enteroepithelial sexual cycle results in formation of oocysts in the intestine of the cat. Oocysts are shed in the feces of the cat and become infectious only after excretion and sporulation occurs. Transmission to intermediate hosts, including humans, occurs mainly by ingestion of undercooked or raw meat containing tissue cysts, ingestion of vegetables or other food products contaminated with oocysts, or by the transplacental route (Fig. 4).
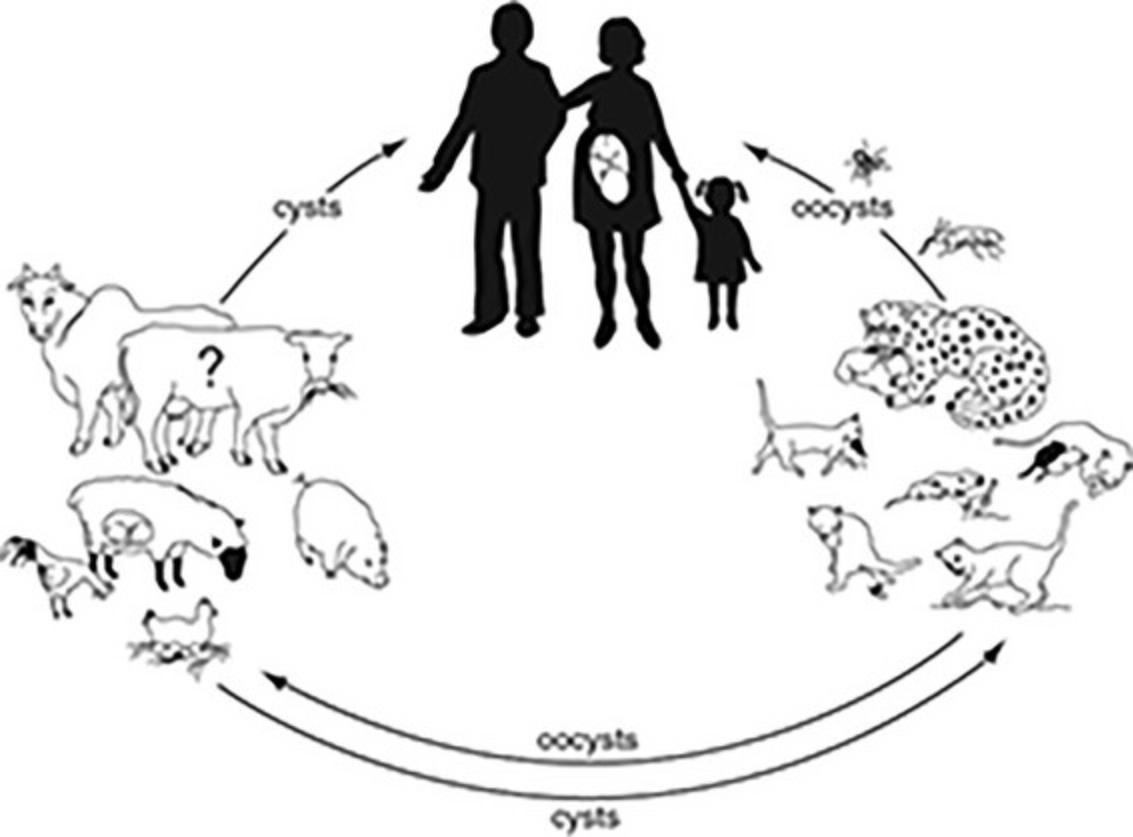
Cell-mediated immunity plays a central role in resistance against T. gondii. In a model in which splenocytes from ts-4-immunized mice were adoptively transferred to naive mice, resistance to acute infection with a virulent strain of T. gondii was dependent on both CD4+ and CD8+ T cells (Gazzinelli et al., 1991; Suzuki & Remington, 1988). Although in this model, CD8+ T cells played the major role in protection against the parasite, experiments performed in other models indicate that CD4+ T cells are also central to the induction of protective immunity during acute T. gondii infection. Thus, depletion of CD4+ T cells prior to acute infection with the ME49 strain prevented acquisition of resistance to challenge with virulent T. gondii (Araujo, 1991). Moreover, reactivation of chronic infection and death occurred after depletion of both CD4+ and CD8+ T cells in C57BL/6 mice chronically infected with the ME49 strain (Gazzinelli et al., 1992). T cells confer protection against the parasite by secretion of IFN-γ. This cytokine has been shown to be protective during both the acute and chronic stages of the infection (Gazzinelli et al., 1991; Suzuki et al., 1988; Suzuki et al., 1989). IFN-γ production is driven by IL-12, a cytokine that is produced in vivo primarily by dendritic cells (Liu et al., 2006). Experiments conducted using SCID mice allowed the identification of a T cell-independent but IFN-γ- dependent mechanism of protection against T. gondii. In this arm of the immune response, IFN-γ production by NK cells is triggered by the T. gondii -mediated secretion of IL-12 and TNF-α by macrophages (Gazzinelli, Hieny et al., 1993; Hunter et al., 1994). Thus, both T cells and NK cells are important sources of IFN-γ during infection. However, although temporarily effective, this innate immune response does not appear to be sufficient to completely control parasite replication (Johnson et al., 1993). In addition to IFN-γ, other cytokines including IL-12, TNF-α, IL-2, IL-6, IL-7, IL-15 have been demonstrated to confer protection against T. gondii infection in mice (Gazzinelli, Eltoum et al., 1993; Gazzinelli, Hieny et al., 1993; Hunter et al., 1994; Hunter et al., 1995; Kasper et al., 1995; Khan and Kasper, 1996; Sharma et al., 1985; Suzuki et al., 1997). Type 1 pro-inflammatory cytokine response induced during infection needs to be regulated to avoid immunopathology. Indeed, C57BL/6 mice infected orally with ME49 T. gondii develop severe ileitis and death that are caused by an overwhelming type 1 cytokine response and not by unrestricted parasite growth (Liesenfeld et al., 1996). IL-10, IL-27 and TGF-β are important to restrain inflammatory responses to T. gondii infection (Gazzinelli et al., 1996; Mennechet et al., 2004; Stumhofer et al., 2006). Although T lymphocytes cytotoxic for cells infected with T. gondii are generated during infection with the parasite (Subauste et al., 1991), it appears that lysis of infected cells plays a secondary role in protection (Denkers et al., 1997). Finally, humoral immunity appears to act in concert with cell-mediated immunity to protect against the infection (Sayles et al., 2000). Enterocytes, intraepithelial lymphocytes, NK cells, NKT cells and CD4+ T cells from the lamina propria contribute to the immune response within the gut (Buzoni-Gatel et al., 2006). Despite the evidence discussed above that T. gondii triggers a potent cell-mediated immune response, the parasite successfully avoids elimination from the host by forming tissue cysts in multiple organs.
Studies of toxoplasmic encephalitis in murine models have demonstrated that susceptibility for this disease is under genetic control. Whereas mice that carry the Ld haplotype develop low numbers of tissue cysts in their brains and are resistant to development of toxoplasmic encephalitis, strains that carry other haplotypes have large numbers of tissue cysts and develop encephalitis (Brown & McLeod, 1990; Brown et al., 1995; Suzuki et al., 1994). Although the importance of the Ld gene in determining susceptibility to this disease is well established, data obtained in mice carrying a mutation in MHC class II molecules and in mice depleted of CD4+ T cells suggest that other factors may also control development of toxoplasmic encephalitis (Araujo, 1991; Brown and McLeod, 1990).
Resistance to acute infection with T. gondii varies among inbred strains of mice. Multiple genes, including H-2 -linked and nonlinked genes, appear to be responsible for determining mortality in mice during acute infection (McLeod et al., 1989). However, in contrast to toxoplasmic encephalitis, the Ld gene does not appear to play a role in determining increased survival after oral infection (Brown et al., 1995). Moreover, susceptibility of mice during the chronic infection does not correlate with that during acute infection (Suzuki et al., 1993).
Critical Parameters and Troubleshooting
The outcome of infection varies widely depending on the strain of mice, virulence of the strain of the parasite, and the route of infection. Thus, these parameters should be carefully considered for the design of experiments that use animal models of T. gondii. In addition, selection of inoculum size is critical, since variations in the dose of T. gondii administered can significantly affect susceptibility to infection among certain inbred strains of mice (Araujo et al., 1976).
Female mice from susceptible strains should be used for induction of toxoplasmic encephalitis (see Basic Protocol 2), since male mice are less prone to develop this form of toxoplasmosis.
For maintenance of a bank of cysts of the ME49 strain (see Support Protocol 2), it is important to avoid repeated serial passage of cysts, since this may increase the virulence of the organism. For this reason, infected Swiss-Webster mice should be kept as a “permanent” bank and used only to infect either C57BL/6 or CBA/Ca mice, which will then become the source of cysts for experiments. Ideally, banks in Swiss-Webster mice should not be set up more than once or twice a year.
Bioluminescence imaging allows for serial, non-lethal quantification of parasite load in various organs (Dellacasa-Lindberg et al., 2007; Hitziger et al., 2005). However, factors such as organ location, tissue density and depth of the source of photon emission can affect the efficacy of external detection of bioluminescence. Indeed, quantification of parasite load in the central nervous system is impaired in conditions of low parasite burden.
Immune cells in animal models of T. gondii infection have been imaged in situ using multi-photon microscopy (Chtanova et al., 2009; John et al., 2009; Schaeffer et al., 2009; Wilson et al., 2009). However, one needs to consider that the removal of organs from the animals to make tissues amenable to imaging may affect the process being studied. In addition, interpretation of cell migration needs to take into account that movements of immune cells may be affected by other non-fluorescent cell types present in tissues. Multiphoton microscopy can be used in combination with more traditional imaging modalities such as immunohistochemistry and flow cytometry.
The use of transgenic parasites that express a model antigen such as OVA has facilitated in vivo studies of T cell responses. However, the responses analyzed are directed against OVA and not an actual parasite antigen. The identification of immunodominant epitopes such as GRA4, GRA6, ROP7, and tgd057 together with the use of epigenetic reprogramming to generate mice where most CD8+ T cells are parasite-specific can enable in vivo studies of T. gondii - specific T cell responses (Blanchard et al., 2008; Kirak et al., 2010; Wilson et al., 2010).
Anticipated Results
Models of acute infection
Nine days after intraperitoneal infection with the ME49 strain of T. gondii , both BALB/c (susceptible) and CBA/Ca (resistant) mice exhibit inflammatory changes in their lungs, hearts, and livers (Suzuki et al., 1993). As early as 3 days after oral infection with the ME49 strain, both resistant (BALB/c) and susceptible (C57BL/6) mice develop areas of inflammation in their livers (Liesenfeld et al., 1996). Seven days post-infection, C57BL/6 mice, but not BALB/c mice, develop massive necrosis of the villi and mucosal cells in the ileum, which is mediated by CD4+ T cells and IFN-γ. Mild inflammation is observed in lungs of both strains of mice 7 days post-infection. Brain, heart, spleen, and large intestine do not show inflammatory changes at this stage.
C57BL/6 mice infected intraperitoneally with the ME49 strain exhibit increased levels of expression of IL-12 p40, IFN-γ, and TNF-α mRNA in their spleens as early as 2 days post-infection (Gazzinelli, Wysocka et al., 1994). Elevated serum levels of IFN-γ and IL-12 p70 can be detected 6 days after infection in C57BL/10 mice (Gazzinelli et al., 1996). In contrast, whereas serum TNF-α is usually not detected in infected animals, peritoneal fluid can show measurable concentrations of this cytokine (Johnson, 1992b). Moreover, peritoneal cells and splenocytes from infected C57BL/6 mice produce IFN-γ and IL-l2 p40 spontaneously, without the need for in vitro stimulation during the first week post-infection (Gazzinelli, Wysocka et al., 1994). Production of IL-4 is usually difficult to detect but it can be identified in infected MyD88-/- mice (Jankovic et al., 2002).
Bioluminescence imaging revealed rapid dissemination of T. gondii including involvement of immunoprivileged organs (brain, eyes, and testes) (Hitziger et al., 2005). Visualization of CD8+ T cell responses within lymph nodes using multi-photon microscopy revealed that primary infection is accompanied by sustained interactions between dendritic cells and CD8+ T cells in the presence of antigen (John et al., 2009). Studies of recall responses revealed that, compared to naive CD8+ T cells, memory CD8+ T cells exhibited more rapid migration and more extensive relocalization to areas of infection and formed antigen-specific clusters near infected macrophages (Chtanova et al., 2009).
T. gondii infection has been reported to induce expansion of CD4+ T cells, CD8+ T cells, γδ T cells, NK cells, and polymorphonuclear cells in the spleen and peritoneal cavities (Hisaeda et al., 1995; Johnson et al., 1993; Scharton-Kersten et al., 1996). The use of OVA-expressing parasites and T cell receptor transgenic mice has also revealed antigen-driven activation, acquisition of effector function and in vivo expansion of T cells (John et al., 2009; Pepper et al., 2004). Oral infection has been reported to induce an increase in the relative percentages of the CD8β+ and Thy-1+ intraepithelial lymphocyte populations (Chardes et al., 1994) and a decrease in the percentage of CD4+ T cells in Peyer's patches from susceptible (C57BL/6) mice (Liesenfeld et al., 1996).
Significant in vitro lymphocyte proliferation in response to T. gondii lysate antigens is detected 14 days after oral infection with the ME49 strain in BALB/c mice and 21 days after oral infection in CBA/Ca mice (Candolfi et al., 1994). However, acute T. gondii infection induces suppression of lymphocyte proliferation in response to concanavalin A (Candolfi et al., 1994; Khan et al., 1995).
Models of toxoplasmic encephalitis and toxoplasma retinochoroiditis
Toxoplasmic encephalitis in susceptible mice manifests by acute focal or diffuse meningoencephalitis with necrosis, microglial nodules, and perivascular mononuclear inflammation. These lesions are multiple and are diffuse in distribution. Tachyzoites are usually found at the periphery of necrotic areas. These findings can be readily detected 4 weeks after infection and increase in severity by 8 to 10 weeks post-infection (Gazzinelli, Eltoum et al., 1993; Suzuki et al., 1991). Toxoplasma retinochoroiditis manifests as distortion of retinal architecture, hypertrophy of retinal pigmented epithelial cell layer, invasion of the retina by these cells and inflammatory infiltrates (Portillo et al., 2010). Criteria for scoring inflammatory process in the brains and eyes of these animals have been described previously (Araujo et al., 1996; Gazzinelli, Eltoum et al., 1993; Gazzinelli, Brezin et al., 1994). Mice susceptible to toxoplasmic encephalitis, such as CBA/Ca, lack evidence of inflammation in their lungs, livers, spleen, or kidneys in the chronic phase of infection (Suzuki et al., 1993).
C57BL/6 mice with toxoplasmic encephalitis and toxoplasma retinochoroiditis upregulate various molecules in the brain and eye including IFN-γ, TNF-α, IL-1, IL-6, IL-10, IL- 12, TGF-β and NOS2 (Gazzinelli, Eltoum et al., 1993; Gazzinelli, Brezin et al., 1994; Hunter et al., 1993; Portillo et al., 2010). In addition, infection at these sites results in recruitment of activated CD4+ and CD8+ T cells that express effector molecules such as IFN-γ and perforin (Schluter et al., 2002). Examination of brain mononuclear cells reveals also an increase in macrophages, NK cells, granulocytes, as well as in vitro secretion of IFN-γ, IL-12, TNF-α, IL-6 and nitric oxide (Reichmann et al., 2000; Schluter et al., 1995; Stumhofer et al., 2006).
Bioluminescence imaging allows for the detection of recrudescence of infection in the brain of immunosuppressed animals (Dellacasa-Lindberg et al., 2007). Multiphoton microscopy has been used to examine the behavior of CD8+ T cells within the brain. These studies revealed that CD8+ T cells contact granuloma-like structures containing parasites and CD11b+ antigen presenting cells and migrate following a reticular system of fibers induced by infection (Schaeffer et al., 2009; Wilson et al., 2009).
SCID mouse model
Seven days after oral infection with the ME49 strain, SCID mice develop inflammation in their lungs associated with the presence of tachyzoites (Hunter et al., 1993). Later in the course of infection (days 14 to 18), tachyzoites and inflammatory lesions are also observed in the heart, liver, and kidney. Tachyzoites associated with mild meningitis, encephalitis, vasculitis, and small necrotic areas can be detected in the brain 14 days post-infection (Hunter et al., 1993; Johnson, 1992a). Whereas SCID mice infected with the ME49 strain die, reconstitution with spleen cells from uninfected mice prior to infection results in survival (Johnson, 1992a).
Sulfadiazine-treated SCID mice not only survive infection, but also develop brain cysts (Johnson, 1992a). Examination of other organs such as lung, heart, kidney, liver, and spleen from infected SCID mice, while on sulfadiazine or after its discontinuation, fails to demonstrate evidence of parasites or of inflammation (Beaman et al., 1994; Johnson, 1992a). Cessation of sulfadiazine results in death of the animal with numerous foci of tachyzoites in their brains (Johnson, 1992a). Reactivation of infection can be prevented by reconstitution with immune but not with nonimmune spleen cells before discontinuation of sulfadiazine (Beaman et al., 1994).
Infection with the cps1-1 or ts-4 strains
Mice immunized with these strains acquire resistance against challenge with virulent strains of T. gondii such as the RH strain (Fox & Bzik, 2002; Waldeland & Frenkel, 1983).
Time Considerations
Mice used as a source of cysts of the ME49 strain should be sacrificed for this purpose at least 1 month after infection. Depending of the model used, the course of T. gondii infection may take from 1 to 10 weeks. Histopathologic evaluation including immunohistochemistry requires ∼3 days. It takes ∼2 days to evaluate the levels of mRNA for different cytokines and parasite-specific genes. Measurement of cytokine concentrations by ELISA can be completed in 1 or 2 days. In vitro stimulation of lymphocytes with T. gondii requires between 1 to 7 days depending on the purpose of the experiment (evaluation of cytokine production or T cell proliferation).
Immunization with the ts-4 strain can be completed in 6 weeks. Either serial passage of tachyzoites or infection of mice with cysts requires 1 to 2 hr. Finally, preparation of T. gondii lysate antigens can be accomplished in 2 hr.
Acknowledgments
C.S.S is supported by NIH grants EY018341, EY019250 and P30 EY11373. A.H. is supported by NIH grant EY035156.
Author Contributions
Carlos S. Subauste : Writing original draft, writing review and editing. Alyssa Hubal : Writing review and editing.
Conflict of Interest
The authors declare no conflict of interest.
Open Research
Data Availability Statement
All data are provided in the manuscript.
Literature Cited
- Araujo, F. G. (1991). Depletion of L3T4+ (CD4+) T lymphocytes prevents development of resistance to Toxoplasma gondii in mice. Infection and Immunity , 59, 1614–1619. https://doi.org/10.1128/iai.59.5.1614-1619.1991
- Araujo, F. G., Williams, D. M., Grumet, F. C., & Remington, J. S. (1976). Strain-dependent differences in murine susceptibility to toxoplasma. Infection and Immunity , 13, 1528–1530. https://doi.org/10.1128/iai.13.5.1528-1530.1976
- Araujo, F. G., Suzuki, Y., & Remington, J. S. (1996). Use of rifabutin in combination with atovaquone, clindamycin, pyrimethamine, or sulfadiazine for treatment of toxoplasmic encephalitis in mice. European Journal of Clinical Microbiology & Infectious Diseases, 15, 394–397.
- Beaman, M. H., Araujo, F. G., & Remington, J. S. (1994). Protective reconstitution of the SCID mouse against reactivation of toxoplasmic encephalitis. Journal of Infectious Diseases , 169, 375–383. https://doi.org/10.1093/infdis/169.2.375
- Blackwell, J. M., Roberts, C. W., & Alexander, J. (1993). Influence of genes within the MHC on mortality and brain cyst development in mice infected with Toxoplasma gondii : Kinetics of immune regulation in BALB H-2 congenic mice. Parasite Immunology , 15, 317–324. https://doi.org/10.1111/j.1365-3024.1993.tb00616.x
- Blanchard, N., Gonzalez, F., Schaeffer, M., Joncker, N. T., Cheng, T., Shastri, A. J., Robey, E. A., & Shastri, N. (2008). Immunodominant, protective response to the parasite Toxoplasma gondii requires antigen processing in the endoplasmic reticulum. Nature Immunology , 9, 937–944. https://doi.org/10.1038/ni.1629
- Bosch-Driessen, L., Berendschot, T., Ongkosuwito, J., & Rothova, A. (2022). Ocular toxoplasmosis: Clinical features and prognosis of 154 patients. Ophthalmology , 109(5), 869–878. https://doi.org/10.1016/S0161-6420(02)00990-9
- Bretagne, S., Costa, J. M., Vidaud, M., Tran van Nhieu, J., & Fleury-Feith, J. (1993). Detection of Toxoplasma gondii by competitive DNA amplification of bronchoalveolar lavage samples. Journal of Infectious Diseases , 168, 1585–1588. https://doi.org/10.1093/infdis/168.6.1585
- Brown, C. R., & McLeod, R. (1990). Class I MHC genes and CD8+ T cells determine cyst number in Toxoplasma gondii infection. Journal of Immunology , 145, 3438–3441. https://doi.org/10.4049/jimmunol.145.10.3438
- Brown, C. R., Hunter, C. A., Estes, R. G., Beckmann, E., Forman, J., David, C., Remington, J. S., & McLeod, R. (1995). Definitive identification of a gene that confers resistance against Toxoplasma cyst burden and encephalitis. Immunology , 85, 419–428.
- Butler, N. J., Furtado, J. M., Winthrop, K. L., & Smith, J. R. (2012). Ocular toxoplasmosis II: Clinical features, pathology, and management. Clinical & Experimental Ophthalmology, 41(1), 95–108.
- Buzoni-Gatel, D., Schulthess, J., Menard, L. C., & Kasper, L. H. (2006). Mucosal defences against orally acquired protozoan parasites, emphasis on Toxoplasma gondi i infections. Cellular Microbiology , 8, 535–544. https://doi.org/10.1111/j.1462-5822.2006.00692.x
- Candolfi, E., Hunter, C. A., & Remington, J. S. (1994). Mitogen- and antigen-specific proliferation of T cells in murine toxoplasmosis is inhibited by reactive nitrogen intermediates. Infection and Immunity , 62, 1995–2001. https://doi.org/10.1128/iai.62.5.1995-2001.1994
- Chardes, T., Buzoni-Gatel, D., Lepage, A., Bernard, F., & Bout, D. (1994). Toxoplasma gondii oral infection induces specific cytotoxic CD8α/β+ Thy-1+ gut intraepithelial lymphocytes, lytic for parasite- infected enterocytes. Journal of Immunology , 153, 4596–4603. https://doi.org/10.4049/jimmunol.153.10.4596
- Chtanova, T., Han, S.-J., Schaeffer, M., van Dooren, G. G., Herzmark, P., Striepen, B., & Robey, E. A. (2009). Dynamics of T cell, antigen-presenting cell, and pathogen interactions during recall responses in the lymph node. Immunity , 31, 342–355. https://doi.org/10.1016/j.immuni.2009.06.023
- Courret, N., Darche, S., Sonigo, P., Milon, G., Buzoni-Gatel, D., & Tardieux, I. (2006). CD11c- and CD11b-expressing mouse leukocytes transport single Toxoplasma gondii tachyzoites to the brain. Blood , 107(1), 309–316. https://doi.org/10.1182/blood-2005-02-0666
- Current Protocols. (2006). Commonly used reagents. Current Protocols in Microbiology , 00, A.2A.1–A.2A.15. https://doi.org/10.1002/9780471729259.mca02as00
- de Lima Bessa, G., de Almeida Vitor, R. W., & dos Santos Martins-Duarte, E. (2021). Toxoplasma gondii in South America: A differentiated pattern of spread, population structure and clinical manifestations. Parasitology Research , 120(9), 3065–3076. https://doi.org/10.1007/s00436-021-07282-w
- Dellacasa-Lindberg, I., Hitziger, N., & Barragan, A. (2007). Localized recrudescence of Toxoplasma infections in the central nervous system of immunocompromised mice assessed by in vivo bioluminescence imaging. Microbes and Infection , 9, 1291–1298. https://doi.org/10.1016/j.micinf.2007.06.003
- Denkers, E. Y., Yap, G., Scharton-Kersten, T., Charest, H., Butcher, B., Caspar, P., Hieny, S., & Sher, A. (1997). Perforin-mediated cytolysis plays a limited role in host resistance to Toxoplasma gondii. Journal of Immunology , 159, 1903–1908. https://doi.org/10.4049/jimmunol.159.4.1903
- Deshmukh, A., Gurupwar, R., Mitra, P., Aswale, K., Shinde, S., & Chaudhari, S. (2021). Toxoplasma gondii induces robust humoral immune response against cyst wall antigens in chronically infected animals and humans. Microbial Pathogenesis , 152, 104643. https://doi.org/10.1016/j.micpath.2020.104643
- Desmonts, G., & Remington, J. S. (1980). Direct agglutination test for diagnosis of Toxoplasma infection: Method for increasing sensitivity and specificity. Journal of Clinical Microbiology , 11, 562–568. https://doi.org/10.1128/jcm.11.6.562-568.1980
- Diehl, K., Hull, R., Morton, D., Pfister, R., Rabemampianina, Y., Smith, D., Vidal, J. M., & van de Vorstenbosch, C. (2001). A good practice guide to the administration of substances and removal of blood, including routes and volumes. Journal of Applied Toxicology , 21, 15–23. https://doi.org/10.1002/jat.727
- Donovan, J., & Brown, P. (2006a). Blood collection. Current Protocols in Immunology , Chapter 1, 1.7.1–1.7.9. https://doi.org/10.1002/0471142735.im0107s73
- Donovan, J., & Brown, P. (2006b). Euthanasia. Current Protocols in Immunology , Chapter 1, 1.8.1–1.8.4. https://doi.org/10.1002/0471142735.im0108s73
- Donovan, J., & Brown, P. (2006c). Parenteral injections. Current Protocols in Immunology , Chapter 1, 1.6.1–1.6.10. https://doi.org/10.1002/0471142735.im0106s73
- Donovan, J., & Brown, P. (2007). Managing immunocompromised animals. Current Protocols in Immunology , Chapter 1, 1.2.1–1.2.5. https://doi.org/10.1002/0471142735.im0102s77
- Dubey, J. P., Lago, E. G., Gennari, S. M., & Jones, J. L. (2012). Toxoplasmosis in humans and animals in Brazil: High prevalence, high burden of disease, and epidemiology. Parasitology , 139(11), 1375–1424. https://doi.org/10.1017/S0031182012000765
- Ferreira, A. M., Martins, M. S., & Vitor, R. W. A. (2001). Virulence for BALB/c mice and antigenic diversity of eight Toxoplasma gondii strains isolated from animals and humans in Brazil. Parasite , 8, 99–105. https://doi.org/10.1051/parasite/2001082099
- Fox, B. A., & Bzik, D. J. (2002). De novo pyrimidine biosynthesis is required for virulence of Toxoplasma gondii. Nature , 415, 926–929. https://doi.org/10.1038/415926a
- Gazzinelli, R. T., Hakim, F. T., Hieny, S., Shearer, G. M., & Sher, A. (1991). Synergistic role of CD4+ and CD8+ T lymphocytes in IFN-γ production and protective immunity induced by an attenuated Toxoplasma gondii vaccine. Journal of Immunology , 146, 286–292. https://doi.org/10.4049/jimmunol.146.1.286
- Gazzinelli, R. T., Xu, Y., Hieny, S., Cheever, A., & Sher, A. (1992). Simultaneous depletion of CD4+ and CD8+ T lymphocytes is required to reactivate chronic infection with Toxoplasma gondii. Journal of Immunology , 149, 175–180.
- Gazzinelli, R. T., Eltoum, I., Wynn, T. A., & Sher, A. (1993). Acute cerebral toxoplasmosis is induced by in vivo neutralization of TNF-α and correlates with the down-regulated expression of inducible nitric oxide synthase and other markers of macrophage activation. Journal of Immunology , 151, 3672–3681. https://doi.org/10.4049/jimmunol.151.7.3672
- Gazzinelli, R. T., Hieny, S., Wynn, T. A., Wolf, S., & Sher, A. (1993). Interleukin 12 is required for the T-lymphocyte-independent induction of interferon γ by an intracellular parasite and induces resistance in T-cell-deficient hosts. Proceedings of the National Academy of Sciences of the United States of America , 90, 6115–6119. https://doi.org/10.1073/pnas.90.13.6115
- Gazzinelli, R. T., Brezin, A., Li, Q., Nussenblatt, R. B., & Chan, C. C. (1994). Toxoplasma gondii : Acquired ocular toxoplasmosis in the murine model, protective role of TNF-α and IFN-γ. Experimental Parasitology , 78, 217–229. https://doi.org/10.1006/expr.1994.1022
- Gazzinelli, R. T., Wysocka, M., Hayashi, S., Denkers, E. Y., Hieny, S., Caspar, P., Trinchieri, G., & Sher, A. (1994). Parasite-induced IL-12 stimulates early IFN-γ synthesis and resistance during acute infection with Toxoplasma gondii. Journal of Immunology , 153, 2533–2543. https://doi.org/10.4049/jimmunol.153.6.2533
- Gazzinelli, R. T., Wysocka, M., Hieny, S., Scharton-Kersten, T., Cheever, A., Kuhn, R., Muller, W., Trinchieri, G., & Sher, A. (1996). In the absence of endogenous IL-10, mice acutely infected with Toxoplasma gondii succumb to a lethal immune response dependent on CD4+ T cells and accompanied by overproduction of IL-12, IFN-γ, and TNF-α. Journal of Immunology , 157, 798–805. https://doi.org/10.4049/jimmunol.157.2.798
- Gonçalves, R., & Mosser, D. M. (2015). The isolation and characterization of murine macrophages. Current Protocols in Immunology , 111, 14.1.1–14.1.16. https://doi.org/10.1002/0471142735.im1401s111
- Halonen, S., & Weiss, L. M. (2014). Toxoplasmosis. Handbook of Clinical Neurology , 114, 125–145. https://doi.org/10.1016/B978-0-444-53490-3.00008-X
- Hisaeda, H., Nagasawa, H., Maeda, K., Maekawa, Y., Ishikawa, H., Ito, Y., Good, R. A., & Himeno, K. (1995). γδ T cells play an important role in hsp65 expression and in acquiring protective immune responses against infection with Toxoplasma gondii. Journal of Immunology , 154, 244–251. https://doi.org/10.4049/jimmunol.155.1.244
- Hitziger, N., Dellacasa, I., Albiger, B., & Barragan, A. (2005). Dissemination of Toxoplasma gondii to immunoprivileged organs and role of Toll/interleukin-1 receptor signalling for host resistance assessed by in vivo bioluminescence imaging. Cellular Microbiology , 7, 837–848. https://doi.org/10.1111/j.1462-5822.2005.00517.x
- Hofman, F. M., & Taylor, C. R. (2013). Immunohistochemistry. Current Protocols in Immunology , 103, 21.4.1–21.4.26. https://doi.org/10.1002/0471142735.im2104s103
- Howe, D. K., & Sibley, L. D. (1995). Toxoplasma gondii comprises three clonal lineages: Correlation of parasite genotypes with human disease. Journal of Infectious Diseases , 172, 1561–1566. https://doi.org/10.1093/infdis/172.6.1561
- Hunter, C. A., Abrams, J. S., Beaman, M. H., & Remington, J. S. (1993). Cytokine mRNA in the central nervous system of SCID mice infected with Toxoplasma gondii : Importance of T-cell- independent regulation of resistance to T. gondii. Infection and Immunity , 61, 4033–4044. https://doi.org/10.1128/iai.61.10.4038-4044.1993
- Hunter, C. A., Subauste, C. S., van Cleave, V. H., & Remington, J. S. (1994). Production of gamma interferon by natural killer cells from Toxoplasma gondii -infected SCID mice: Regulation by interleukin-10, interleukin-12 and tumor necrosis factor alpha. Infection and Immunity , 62, 2818–2824. https://doi.org/10.1128/iai.62.7.2818-2824.1994
- Hunter, C. A., Candolfi, E., Subauste, C. S., van Cleave, V., & Remington, J. S. (1995). Studies on the role of interleukin-12 in acute murine toxoplasmosis. Immunology , 84, 16–20.
- James, S. P. (2001). Detection of cytokine mRNA expression by PCR. Current Protocols in Immunology , Chapter 10, 10.23.1–10.23.10. https://doi.org/10.1002/0471142735.im1023s10
- Jankovic, D., Kulberg, M. C., Hieny, S., Caspar, P., Collazo, C. M., & Sher, A. (2002). In the absence of IL-12, CD4+ T cell response to intracellular pathogens fails to default to a Th2 pattern and are host protective in an IL-10-/- setting. Immunity , 16, 429–439. https://doi.org/10.1016/S1074-7613(02)00278-9
- John, B., Harris, T. H., Tait, E. D., Wilson, E. H., Gregg, B., Ng, L. G., Mrass, P., Roos, D. S., Dzierszinski, F., Weninger, W., & Hunter, C. A. (2009). Dynamic imaging of CD8+ T cells and dendritic cells during infection with Toxoplasma gondii. PLoS Pathogens , 5, e1000505. https://doi.org/10.1371/journal.ppat.1000505
- Johnson, A. M. (1984). Strain-dependent, route of challenge-dependent, murine susceptibility to toxoplasmosis. Zeitschrift Fur Parasitenkunde , 70, 303–309. https://doi.org/10.1007/BF00927816
- Johnson, L. L. (1992a). SCID mouse models of acute and relapsing chronic Toxoplasma gondii infections. Infection and Immunity , 60, 3719–3724. https://doi.org/10.1128/iai.60.9.3719-3724.1992
- Johnson, L. L. (1992b). A protective role for endogenous tumor necrosis factor in Toxoplasma gondii infection. Infection and Immunity , 60, 1979–1983. https://doi.org/10.1128/iai.60.5.1979-1983.1992
- Johnson, L. L., van der Vegt, F. P., & Havell, E. A. (1993). Gamma interferon-dependent temporary resistance to acute Toxoplasma gondii infection independent of CD4+ or CD8+ lymphocytes. Infection and Immunity , 61, 5174–5180. https://doi.org/10.1128/iai.61.12.5174-5180.1993
- Kasper, L. H., Matsuura, T., & Khan, I. A. (1995). IL-7 stimulates protective immunity in mice against the intracellular pathogen, Toxoplasma gondii. Journal of Immunology , 155, 4798–4804. https://doi.org/10.4049/jimmunol.155.10.4798
- Khan, I. A., Matsuura, T., & Kasper, L. H. (1995). IL-10 mediates immunosuppression following primary infection with Toxoplasma gondii in mice. Parasite Immunology , 17, 185–195. https://doi.org/10.1111/j.1365-3024.1995.tb00888.x
- Khan, I. A., & Kasper, L. H. (1996). IL-15 augments CD8+ T cell-mediated immunity against Toxoplasma gondii infection in mice. Journal of Immunology , 157, 2103–2108. https://doi.org/10.4049/jimmunol.157.5.2103
- Kirak, O., Frickel, E. M., Grotenberg, G. M., Suh, H., Jaenisch, R., & Ploegh, H. L. (2010). Transnuclear mice with predefined T cell receptor specificities against Toxoplasma gondii obtained via SCNT. Science , 328, 243–248. https://doi.org/10.1126/science.1178590
- Konradt, C., Ueno, N., Christian, D., Delong, J., Pritchard, G., Herz, J., Bzik, D., Koshy, A., McGavern, D., Lodoen, M., & Hunter, C. (2016). Endothelial cells are a replicative niche for entry of Toxoplasma gondii to the central nervous system. Nature Microbiology , 1, 16001. https://doi.org/10.1038/nmicrobiol.2016.1
- Kruisbeek, A. M. (2001). Isolation of mouse mononuclear cells. Current Protocols in Immunology , Chapter 3. https://doi.org/10.1002/0471142735.im0301s39
- Lambert, H., Hitzigler, N., Dellacasa, I., Svensson, M., & Barragan, A. (2006). Induction of dendritic cell migration upon Toxoplasma gondii infection potentiates parasite dissemination. Cellular Microbiology , 8(10), 1611–1623. https://doi.org/10.1111/j.1462-5822.2006.00735.x
- Liesenfeld, O., Kosek, J., Remington, J. S., & Suzuki, Y. (1996). Association of CD4+ T cell–dependent, interferon-γ-mediated necrosis of the small intestine with genetic susceptibility of mice to peroral infection with Toxoplasma gondii. Journal of Experimental Medicine , 184, 597–607. https://doi.org/10.1084/jem.184.2.597
- Liu, C. H., Fan, Y. T., Dias, A., Esper, L., Corn, R. A., Bafica, A., Machado, F. S., & Aliberti, J. (2006). Cutting edge: Dendritic cells are essential for in vivo IL-12 production and development of resistance against Toxoplasma gondii infection in mice. Journal of Immunology , 177, 31–35. https://doi.org/10.4049/jimmunol.177.1.31
- Lopez Corcino, Y., Gonzalez Ferrer, S., Eliana Mantilla, L., Trikeriotis, S., Yu, J. S., Kim, S., Hansen, S., Portillo, J. A., & Subauste, C. S. (2019). Toxoplasma gondii induces prolonged host epidermal growth factor receptor signalling to prevent parasite elimination by autophagy: Perspectives for in vivo control of the parasite. Cellular Microbiology , 21(10), e13084. https://doi.org/10.1111/cmi.13084
- Lopez Corcino, Y., Portillo, J. A., & Subauste, C. S. (2019). Epidermal growth factor receptor promotes cerebral and retinal invasion by Toxoplasma gondii. Scientific Reports , 9, 669. https://doi.org/10.1038/s41598-018-36724-2
- Lüden, C., & Rahman, T. (2017). Impact of the host on Toxoplasma stage differentiation. Microbial Cell , 4(7), 203–211.
- McLeod, R., Skamene, E., Brown, C. R., Eisenhauer, P. B., & Mack, D. G. (1989). Genetic regulation of early survival and cyst number after peroral Toxoplasma gondii infection of AxB/BxA recombinant inbred and B10 congenic mice. Journal of Immunology , 143, 3031–3034. https://doi.org/10.4049/jimmunol.143.9.3031
- Mahittikorn, A., Wickert, H., & Sukthana, Y. (2010). Toxoplasma gondii : Simple duplex RT-PCR assay for detecting SAG1 and BAG1 genes during stage conversion in immunosuppressed mice. Experimental Parasitology , 124, 225–231. https://doi.org/10.1016/j.exppara.2009.10.003
- Mennechet, F. J. D., Kasper, L. H., Rachinel, N., Minns, L. A., Luangsay, S., Vanderwalle, A., & Buzoni-Gatel, D. (2004). Intestinal intraepithelial lymphocytes prevent pathogen-driven inflammation and regulate the Smad/T-bet pathway of lamina propria CD4+ T cells. European Journal of Immunology , 34, 1059–1067. https://doi.org/10.1002/eji.200324416
- Olivera, G., Ross, E., Peuckert, C., & Barragan, A. (2021). Blood-brain barrier-restricted translocation of Toxoplasma gondii from cortical capillaries. Elife , 10, e69182. https://doi.org/10.7554/eLife.69182
- Pappas, G., Roussos, N., & Falagas, M. E. (2009). Toxoplasmosis snapshots: Global status of Toxoplasma gondii seroprevalence and implications for pregnancy and congenital toxoplasmosis. International Journal for Parasitology , 39, 1385–1394. https://doi.org/10.1016/j.ijpara.2009.04.003
- Paredes-Santos, T. C., Martins-Duarte, E. S., Vitor, R. W. A., de Souza, W., Attias, M., & Vommaro, R. C. (2013). Spontaneous cystogenesis in vitro of a Brazilian strain of Toxoplasma gondii. Parasitology International , 62, 180–188. https://doi.org/10.1016/j.parint.2012.12.003
- Paredes-Santos, T. C., Tomita, T., Yan Fen, M., de Souza, W., Attias, M., Vommaro, R. C., & Weiss, L. M. (2016). Development of dual fluorescent stage specific reporter strain of Toxoplasma gondii to follow tachyzoite and bradyzoite development in vitro and in vivo. Microbes and Infection , 18(1), 39–47. https://doi.org/10.1016/j.micinf.2015.09.016
- Pepper, M., Dzierszinski, F., Crawford, A., Hunter, C. A., & Roos, D. S. (2004). Development of a system to study CD4+-T cell responses to transgenic ovalbumin-expressing Toxoplasma gondii during toxoplasmosis. Infection and Immunity , 72, 7240–7246. https://doi.org/10.1128/IAI.72.12.7240-7246.2004
- Portillo, J.-A. C., Okenka, G., Reed, E., Subauste, A., van Grol, J., Gentil, K., Komatsu, M., Tanaka, K., Landreth, G., Levine, B., & Subauste, C. S. (2010). The CD40-autophagy pathway is needed for host protection despite IFN-γ-dependent immunity and CD40 induces autophagy via control of p21 levels. PLoS ONE , 5(12), e14472. https://doi.org/10.1371/journal.pone.0014472
- Portillo, J.-A. C., van Grol, J., Saffo, S., Lopez Corcino, Y., Bzik, D., Ward, N., Dubyak, G., Rojas, R., Toosi, Z., & Subauste, C. S. (2019). CD40 in endothelial cells restricts neural tissue invasion by Toxoplasma gondii. Infection and Immunity , 87(8), e00868–18. https://doi.org/10.1128/IAI.00868-18
- Puvanesuaran, V. R., Ibrahim, N., Noordin, R., & Balakrishnan, V. (2012). Isolation of viable Toxoplasma gondii cysts from brain samples for oral infection. European Review for Medical and Pharmacological Sciences , 16(9), 1179–1183.
- Reeves, J. P., & Reeves, P. A. (2001). Removal of lymphoid organs. Current Protocols in Immunology , Chapter 1. https://doi.org/10.1002/0471142735.im0109s01
- Reichmann, G., Walker, W., Villegas, E. N., Craig, L., Cai, G., Alexander, J., & Hunter, C. A. (2000). The CD40/CD40 ligand interaction is required for resistance to toxoplasmic encephalitis. Infection and Immunity , 68, 1312–1318. https://doi.org/10.1128/IAI.68.3.1312-1318.2000
- Ribaudo, R., Gilman, M., Kingston, R. E., Choczynski, P., & Sacchi, N. (2001). Preparation of RNA from tissues and cells. Current Protocols in Immunology , Chapter 10, 10.11.1–10.11.14. https://doi.org/10.1002/0471142735.im1011s04
- Roederer, M. (2002). Multiparameter FACS analysis. Current Protocols in Immunology , Chapter 5. https://doi.org/10.1002/0471142735.im0508s49
- Roncarolo, M. G., & Carballido, J. M. (2001). Construction of human-SCID chimeric mice. Current Protocols in Immunology , Chapter 4, 4.8.1–4.8.17. https://doi.org/10.1002/0471142735.im0408s25
- Sayles, P. C., Gibson, G. W., & Johnson, L. L. (2000). B cells are essential for vaccination-induced resistance to virulent Toxoplasma gondii. Infection and Immunity , 68, 1026–1033. https://doi.org/10.1128/IAI.68.3.1026-1033.2000
- Schaeffer, M., Han, S.-J., Chtanova, T., van Dooren, G. G., Herzmark, P., Chen, Y., Roysam, B., Striepen, B., & Robey, E. A. (2009). Dynamic imaging of T cell-parasite interactions in the brains of mice chronically infected with Toxoplasma gondii. Journal of Immunology , 182, 6379–6393. https://doi.org/10.4049/jimmunol.0804307
- Scharton-Kersten, T. M., Wynn, T. A., Denkers, E. Y., Bala, S., Grunvald, E., Hieny, S., Gazzinelli, R. T., & Sher, A. (1996). In the absence of endogenous IFN-γ, mice develop unimpaired IL-12 responses to Toxoplasma gondii while failing to control acute infection. Journal of Immunology , 157, 4045–4054. https://doi.org/10.4049/jimmunol.157.9.4045
- Schluter, D., Hein, A., Dorries, R., & Deckert-Schluter, M. (1995). Different subsets of T cells in conjunction with natural killer cells, macrophages, and activated microglia participate in the intracerebral immune response to Toxoplasma gondii in athymic nude and immunocompetent rats. American Journal of Pathology , 146, 999–1007.
- Schluter, D., Meyer, T., Kwok, L.-Y., Montesinos-Rongen, M., Lutjen, S., Strack, A., Schmitz, M. L., & Deckert, M. (2002). Phenotype and regulation of persistent intracerebral T cells in murine Toxoplasma encephalitis. Journal of Immunology , 169, 315–322. https://doi.org/10.4049/jimmunol.169.1.315
- Schneider, C., Figueroa Velez, D., Orchanian, S., Shallberg, L., Agalliu, D., Hunter, C., Gandhi, S., & Lodoen, M. (2022). Toxoplasma gondii dissemination in the brain is facilitated by infiltrating peripheral immune cells. Host-Microbial Interactions , 13, e02838–22.
- Sharma, S., Hofflin, J., & Remington, J. S. (1985). In vivo recombinant IL-2 enhances survival against lethal challenge with T. gondii. Journal of Immunology , 135, 4160–4163. https://doi.org/10.4049/jimmunol.135.6.4160
- Stumhofer, J. S., Laurence, A., Wilson, E. H., Huang, E., Tato, C. M., Johnson, L. M., Villarino, A. V., Huang, Q., Yoshimura, A., Sehy, D., Saris, C. J. M., O'Shea, J. J., Hennighausen, L., Ernst, M., & Hunter, C. A. (2006). Interleukin 27 negatively regulates the development of interleukin 17-producing T helper cells during chronic inflammation of the central nervous system. Nature Immunology , 7, 937–945. https://doi.org/10.1038/ni1376
- Subauste, C. S., Koniaris, A. H., & Remington, J. S. (1991). Murine CD8+ cytotoxic T lymphocytes lyse Toxoplasma gondii –infected cells. Journal of Immunology , 147, 3955–3959. https://doi.org/10.4049/jimmunol.147.11.3955
- Suzuki, Y., Orellana, M. A., Schreiber, R. D., & Remington, J. S. (1988). Interferon-γ: The major mediator of resistance against Toxoplasma gondii. Science , 240, 516–518. https://doi.org/10.1126/science.3128869
- Suzuki, Y., & Remington, J. S. (1988). Dual regulation of resistance against Toxoplasma gondii infection by Lyt-2+ and Lyt-1+, L3T4+ T cells in mice. Journal of Immunology , 140, 3943–3946. https://doi.org/10.4049/jimmunol.140.11.3943
- Suzuki, Y., Conley, F. K., & Remington, J. S. (1989). Importance of endogenous IFN-γ for prevention of toxoplasmic encephalitis in mice. Journal of Immunology , 143, 2045–2050. https://doi.org/10.4049/jimmunol.143.6.2045
- Suzuki, Y., Joh, K., Orellana, M. A., Conley, F. K., & Remington, J. S. (1991). A gene(s) within the H-2D region determines the development of toxoplasmic encephalitis in mice. Immunology , 74, 732–739.
- Suzuki, Y., Orellana, M. A., Wong, S. Y., Conley, F. K., & Remington, J. S. (1993). Susceptibility to chronic infection with Toxoplasma gondii does not correlate with susceptibility to acute infection in mice. Infection and Immunity , 61, 2284–2288. https://doi.org/10.1128/iai.61.6.2284-2288.1993
- Suzuki, Y., Joh, K., Kwon, O. C., Yang, Q., Conley, F. K., & Remington, J. S. (1994). MHC class I gene(s) in the D/L region but not the TNF-α gene determines development of toxoplasmic encephalitis in mice. Journal of Immunology , 153, 4649–4654. https://doi.org/10.4049/jimmunol.153.10.4649
- Suzuki, Y., Rani, S., Liesenfeld, O., Kojima, T., Lim, S., Nguyen, T. A., Dalrymple, S. A., Murray, R., & Remington, J. S. (1997). Impaired resistance to the development of toxoplasmic encephalitis in interleukin-6-deficient mice. Infection and Immunity , 65, 2339–2345. https://doi.org/10.1128/iai.65.6.2339-2345.1997
- Unno, A., Suzuki, K., Batanova, T., Cha, S.-Y., Jang, H.-K., Kitoh, K., & Takashima, Y. (2009). Visualization of Toxoplasma gondii stage conversion by expression of stage-specific dual fluorescent proteins. Parasitology , 136, 579–588. https://doi.org/10.1017/S0031182009005836
- Waldeland, H., & Frenkel, J. K. (1983). Live and killed vaccines against toxoplasmosis in mice. Journal of Parasitology , 69, 60–65. https://doi.org/10.2307/3281275
- Watts, E., Zhao, Y., Dhara, A., Eller, B., Patwardhan, A., & Sinai, A. (2015). Novel approaches reveal that Toxoplasma gondii bradyzoites within tissue cysts are dynamic and replicating entities in vivo. MBio , 6(5), e01155–15. https://doi.org/10.1128/mBio.01155-15
- Watts, E., Dhara, A., & Sinai, A. (2017). Purification Toxoplasma gondii tissue cysts using percoll gradients. Current Protocols in Microbiology , 45, 20C.2.1–20C.2.19.
- Wilson, D. C., Grotenberg, G. M., Liu, K., Zhao, Y., Frickel, E. M., Gubbels, M. J., Ploegh, H. L., & Yap, G. S. (2010). Differential regulation of effector- and central-memory responses to Toxoplasma gondii infection by IL-12 revealed by tracking of Tgd057-specific CD8+ T cells. PLoS Pathogens , 19, e1000815. https://doi.org/10.1371/journal.ppat.1000815
- Wilson, E. H., Harris, T. H., Mrass, P., John, B., Tait, E. D., Wu, G. F., Pepper, M., Wherry, E. J., Dzierszinski, F., Roos, D. S., Haydon, P. G., Laufer, T. M, Weninger, W., & Hunter, C. A. (2009). Behaviour of parasite specific effector CD8+ T cells in the CNS and visualization of a kinesis-associated system of reticular fibers. Immunity , 30, 300–311. https://doi.org/10.1016/j.immuni.2008.12.013
- Xiao, J., & Yolken, R. (2016). Strain hypothesis of Toxoplasma gondii infection on the outcome of human diseases. Acta Physiologica , 213(4), 828–845. https://doi.org/10.1111/apha.12458
- Yokoyama, W. M., Thompson, M. L., & Ehrhardt, R. O. (2012). Cryopreservation and thawing of cells. Current Protocols in Immunology , Appendix 3, A.3G.1–A.3G.5. https://doi.org/10.1002/0471142735.ima03gs99
- Zhang, H., Zhang, Y., Cao, J., Zhou, Y., Wang, N., & Zhou, J. (2013). Determination of stage conversion in vitro and in vivo by construction of transgenic Toxoplasma gondii that stably express stage-specific fluorescent proteins. Experimental Parasitology , 134, 275–280. https://doi.org/10.1016/j.exppara.2013.03.015