An In Vitro Model to Assess CRS Potential of CAR T Cells Using a Tumor Cell Line and Autologous Monocytes
Yasmin Nouri, Yasmin Nouri, Robert Weinkove, Robert Weinkove, Rachel Perret, Rachel Perret
Abstract
Chimeric antigen receptor (CAR) T cell therapy is an engineered cell therapy where T cells are isolated and genetically modified to contain a synthetic CAR with specificity to a tumor cell antigen. Upon antigen binding, the CAR T cell will initiate signaling cascades that result in lysis of the associated tumor cell. Cytokine release syndrome (CRS) is the primary toxicity associated with CAR T cell therapy and remains a prominent safety issue with currently available commercial products. CRS is driven by interaction of the CAR T cells with endogenous monocytes and macrophages, which can lead to immune cell overactivation and an increase in certain cytokines to supraphysiological levels. Identifying the potential of any given CAR construct to drive toxicities in vivo should be assessed in preclinical models prior to human trials. While there are in vivo mouse models available for this purpose, these are often complex xenograft models available in few centers. Thus, there is a need to develop an in vitro assay for measuring the CRS potential of CAR T cells. The assay described here is a preclinical tool for assessing the propensity of any given CAR construct to produce potentially CRS-driving cytokines following tumor cell and monocyte interactions. This article provides a detailed protocol for target cell preparation and isolation of monocytes from peripheral blood mononuclear cells (PBMCs) autologous to the CAR T cells, as well as protocols for seeding the three cell types in a co-culture assay and collecting/analyzing the cytokines produced via an ELISA or multiplex bead array. © 2023 The Authors. Current Protocols published by Wiley Periodicals LLC.
Basic Protocol 1 : Preparation of K562 target cells
Basic Protocol 2 : Isolation of monocytes from autologous PBMCs
Basic Protocol 3 : Seeding of CAR T cells, monocytes, and K562 cells in 96-well plates
Basic Protocol 4 : Analysis of co-culture supernatants by single-cytokine ELISA
Alternate Protocol : Analysis of co-culture supernatants by multiplex cytokine bead array
INTRODUCTION
Chimeric antigen receptor (CAR) T cell therapy is an engineered cell therapy where patient-derived T cells are genetically modified ex vivo before being reinfused into the patient. The synthetic receptor expressed on the surface of a CAR T cell is a recombinant protein that, by design, is able to redirect the specificity and cytotoxic function of T cells towards an antigen on the surface of a chosen target cell (Sadelain et al., 2013). This rapid and targeted killing bypasses the inherent barriers imposed by MHC restriction in traditional T cell killing (June et al., 2018). Moreover, it mitigates the incremental kinetics required in an MHC-peptide-driven, APC-involving, endogenous T cell immune response (Ho et al., 2003; Sadelain et al., 2003). CAR T cells have aptly been deemed “living drugs” due to their potent sustained anti-cancer effects and potential to develop into long-lived memory cell populations. After seeing early success in various trials, CAR T cell therapy has now become a major arm of the cancer immunotherapy field, showing broad promise in preclinical studies and inducing remissions in patients lasting over a decade (Melenhorst et al., 2022).
Cytokine release syndrome (CRS) is the primary toxicity associated with CAR T cell therapy and remains a prominent safety issue with currently available commercial products. CRS is driven by the interaction of CAR T cells with endogenous monocytes and macrophages, which can lead to immune cell overactivation and an increase in certain cytokines to supraphysiological levels (Guo et al., 2022; Hao et al., 2020; Sachdeva et al., 2019). It presents as fever, hypotension, hypoxemia, and elevated myeloid cell–derived IL-6 and liver-derived C-reactive protein (CRP) (Cheng et al., 2019). Additional cytokines implicated in CRS include IFN-γ, IL-1, MCP-1, IL-10, and GM-CSF (Murthy et al., 2019). The presence and extent of CRS can be determined by factors such as CAR design, antigen choice, tumor type, and tumor burden (Sterner & Sterner, 2021). Immune effector cell–associated neurotoxicity syndrome (ICANS) can also occur as a result of CAR T cell therapy and has potentially life-threatening outcomes. ICANS has an array of clinical presentations, including headache, confusion, word-finding difficulty, encephalopathy, cerebral edema, and seizures (Lee et al., 2019). Cytokines such as IFN-γ, IL-15, IL-6, IL-10, IL-2, and GM-CSF are associated with ICANS (Gust et al., 2020), many of which have also been implicated in CRS. Both CRS and ICANS are relatively common in patients following CAR T cell therapy (Frey & Porter, 2016; Santomasso et al., 2018). Currently, no preventative treatments are available (Sterner & Sterner, 2021).
Upon exposure to tumor antigens, CAR T cells will bind tumor cells via the CAR, initiating a signaling cascade that results in tumor cell lysis through the release of perforin, granzymes, and certain cytokines (Xiao et al., 2021). Following tumor cell lysis, monocytes and macrophages can be activated by damage-associated molecular patterns (DAMPs) (Gong et al., 2020; Liu et al., 2020), as well as by cytokines released from the CAR T cells (Sachdeva et al., 2019; Titov et al., 2018) and direct receptor-ligand interactions on the surface of CAR T cells and monocytes, such as CD40/CD40L (Giavridis et al., 2018). This activation can lead to a self-amplifying feedback loop of pro-inflammatory cytokine production that is driven primarily by myeloid-derived IL-1 and IL-6 and presents as CRS (Xiao et al., 2021; Fig. 1).
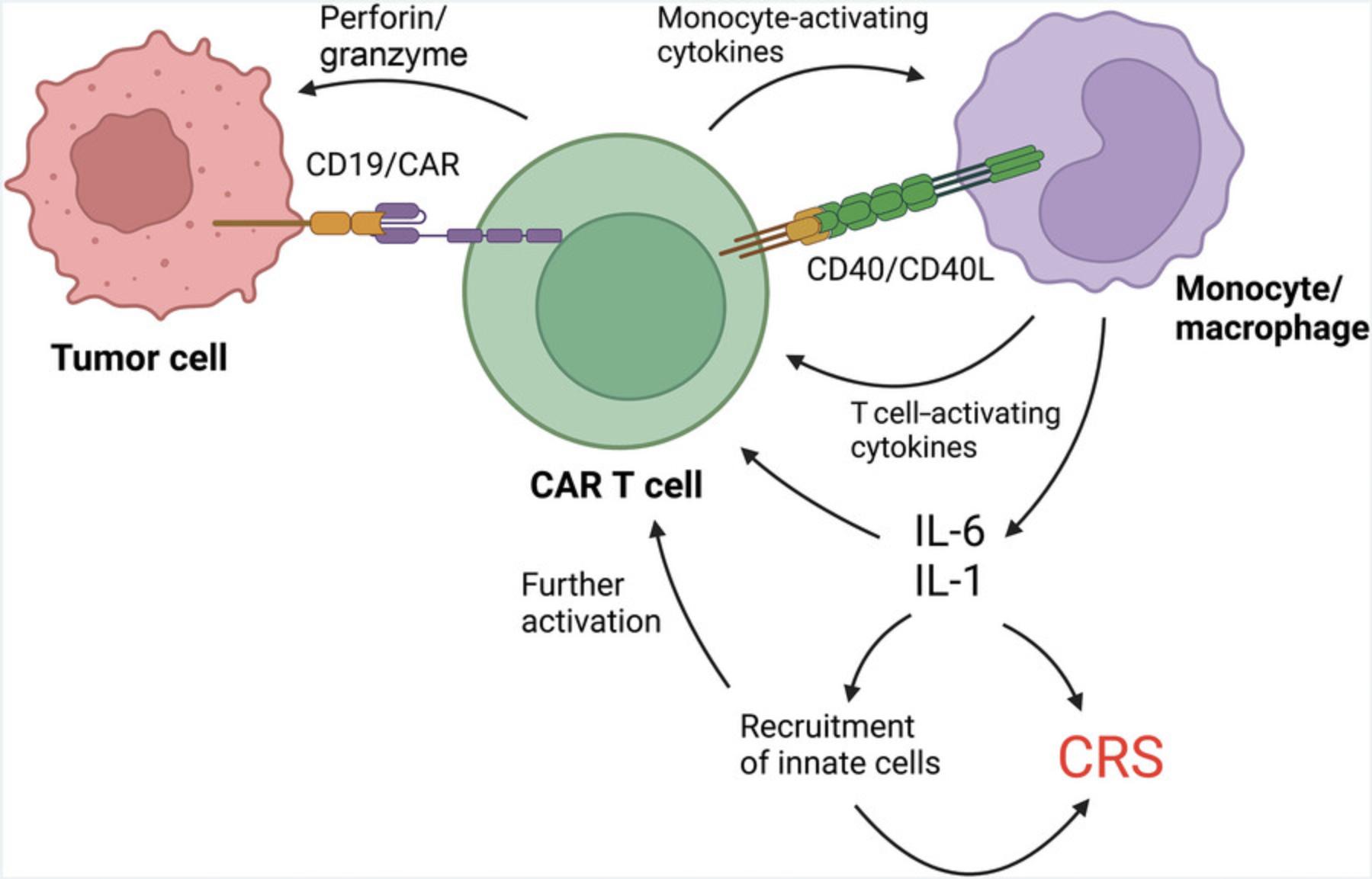
The potential of any given CAR construct to drive toxicities in vivo should be assessed in preclinical models prior to human trials. Although in vivo mouse models are available for this purpose, these are often complex xenograft models (Giavridis et al., 2018; Kenderian et al., 2017; Sterner et al., 2019). To be used in the context of CRS, xenograft models must be adapted to incorporate a human myeloid cell compartment via the administration of umbilical cord–derived human progenitor stem cells to newborn mice. This is a complicated system available at few centers. Using a fully syngeneic mouse model is another in vivo option, but this requires a full redesign of the CAR with murine sequences. As a result, the findings may be less applicable to human CAR T cells. For these reasons, there is a need for an in vitro assay to measure the CRS potential of CAR T cells.
A simplified in vitro model has the advantage of being relatively high-throughput and easily adaptable in regard to target cells used, cell ratios, and number of constructs tested in a single assay. Alongside human CAR T cells and target cells, autologous human monocytes derived from the same donor peripheral blood mononuclear cells (PBMCs) as the CAR T cells are included to model the potentially toxicity-driving interactions that occur between CAR T cells and monocytes in vivo.
The assay described below (Fig. 2) is a preclinical tool for assessing the propensity of any given CAR construct to produce potentially CRS-driving cytokines following tumor cell and monocyte interactions. The specific model described here uses K562 target cells that express CD19 on their surface alongside a CD19-targeting CAR. This model could be adapted for use with alternative target cells and CAR constructs, but optimization may be required to ensure correct cell ratios and assay length are used in this new context. This article first provides detailed protocols for preparing target cancer cells and isolating monocytes from PBMCs autologous to the CAR T cells. The user should follow their own protocol for transduction/preparation of CAR T cells. The article also includes protocols for seeding the three cell types in a co-culture assay and collecting and analyzing the cytokines produced via ELISA or multiplex bead array.
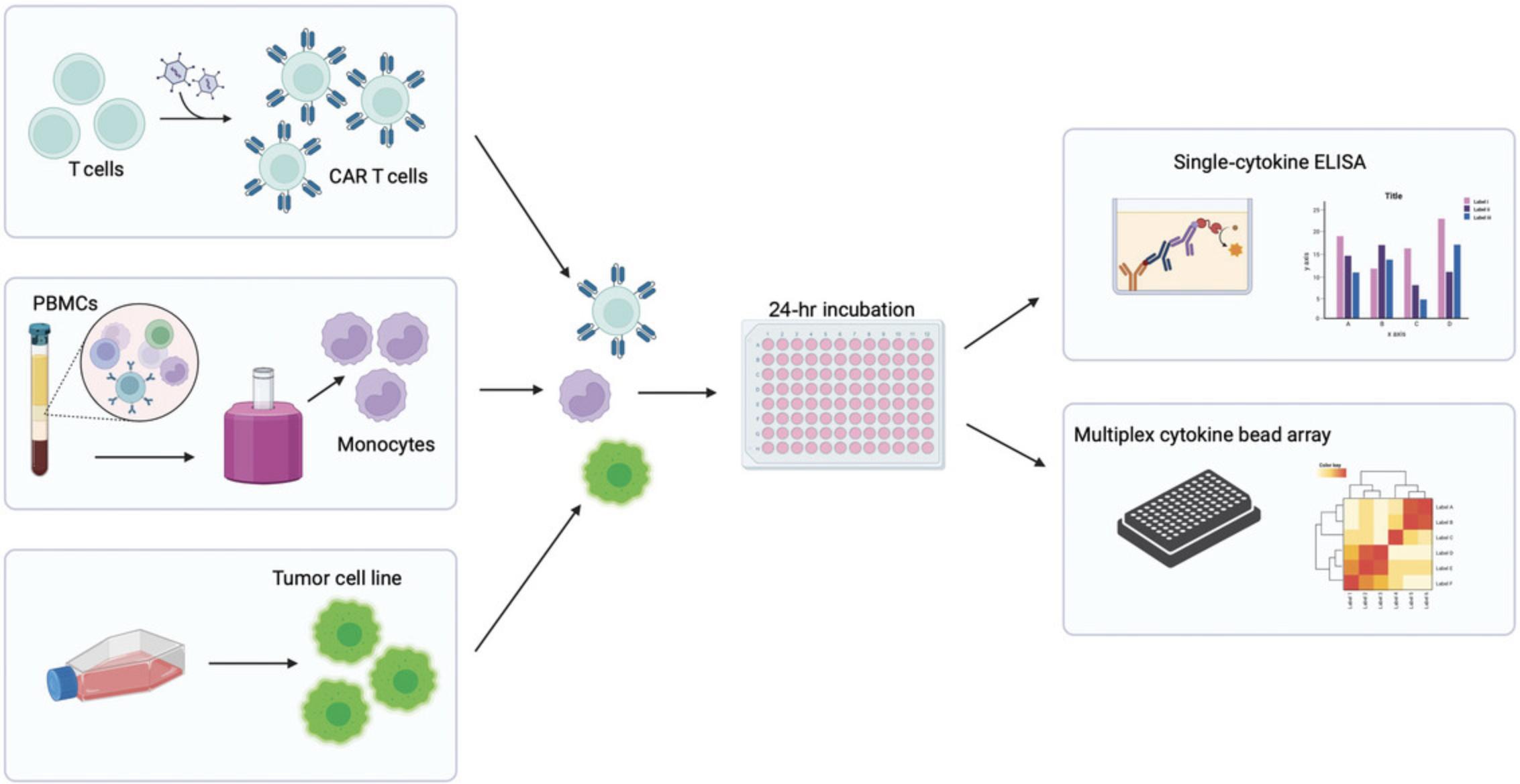
This protocol does not include detailed instructions for generating or culturing CAR T cells, as this is an assay designed to measure the user's own CAR T cell product for CRS potential. CAR T cells should be generated from donor PBMCs using an optimized protocol specific to the CAR T cells being used. CAR T cells should be used fresh from culture following expansion (up to 14 days following transduction) or after being thawed and rested for ∼4 hr. CAR T cell populations should be of the highest purity possible to avoid the confounding effects of untransduced T cells in the culture. If multiple CAR T cells are being tested and compared, they should be equalized for CAR percentage prior to assay seeding.
NOTE : All aspects of this assay prior to cytokine analysis should be performed in a class II laminar flow tissue culture hood (e.g., Herasafe 2030i BSC). All cell culture should be performed in a 37°C incubator with 5% CO2.
NOTE : Appropriate informed consent is necessary for obtaining and using human study material.
Basic Protocol 1: PREPARATION OF K562 TARGET CELLS
This protocol describes how to prepare the target cells for the assay. This preparation is specific to the K562 tumor cell line. K562 cells are derived from human lymphoblasts isolated from the bone marrow of a chronic myelogenous leukemia patient. The cells used here have been transduced with human CD19 (K562-CD19). Non-transduced K562 cells are used as an antigen-negative control.
If an alternative tumor cell line is used, appropriate media and passage conditions specific to that cell line should be used. Any tumor cell line being used should express the target antigen at levels comparable to those observed on tumor tissue from patients. Additionally, an antigen-negative control should be used. This should be as close as possible to the antigen-positive cell line but lacking target antigen expression.
Materials
-
K562 target cells (ATCC CLL-243)
-
K562 target cells with stable CD19 expression
-
Complete IMDM (see recipe)
-
Sterile 50-ml Falcon tubes (High Clarity PP, Corning, cat. no. 352070)
-
10- and 25-ml serological pipets (e.g., Costar Strippette, Merck, cat. no. CLS4488)
-
Cell counter, manual or automated
-
Tissue culture microscope (e.g., Olympus)
-
75-cm2 tissue culture flasks (e.g., Nunc EasYFlask, Thermo Fisher Scientific, cat. no. 156472)
1.Thaw 1 × 107 K562 and K562-CD19 cells and resuspend separately in 20 ml complete IMDM in 50-ml centrifuge tubes.
2.Centrifuge cells 4 min at 600 × g , 20°C. Remove supernatant and resuspend cells in 20 ml complete IMDM.
3.Repeat step 2, but resuspend in 10 ml complete IMDM.
4.Count cells and adjust density to 3 × 105 cells/ml.
5.Seed up to 30 ml suspension (9 × 106 cells) in 75-cm2 EasYFlasks. Split cells every third day at a concentration of 3 × 105 cells/ml.
Basic Protocol 2: ISOLATION OF MONOCYTES FROM AUTOLOGOUS PBMCs
Monocytes being used will need to be isolated from the same PBMC donor as was used to generate the CAR T cells. This will mitigate any alloreactivity between the two cell types, which could confound results. Monocytes will need to be isolated immediately prior to assay seeding, in order to avoid the cells becoming too adhesive or altering their phenotype. This protocol describes the isolation of monocytes using the Dynabeads Untouched Human Monocytes Kit (Invitrogen), which allows for isolation of the monocytes via negative selection.
Additional Materials (also see Basic Protocol 1)
-
Human PBMCs autologous to the CAR T cells being used (isolated from blood and stored in liquid nitrogen)
-
Gibco Dulbecco's phosphate-buffered saline (DPBS; Thermo Fisher Scientific, cat. no. 14190144)
-
Isolation buffer (see recipe)
-
Invitrogen Dynabeads Untouched Human Monocytes Kit (Thermo Fisher Scientific, cat. no. 11350D)
-
Sterile 15-ml Falcon tubes (High Clarity PP, Corning, cat. no. 352096)
-
Magnet for bead isolation (e.g., EasySep, Stemcell Technologies, cat. no. 18103)
-
Cell rocker (e.g., Corning LSE Platform Rocker)
1.Thaw 50 × 106 PBMCs and resuspend in 10 ml DPBS in a 15-ml centrifuge tube.
2.Centrifuge 4 min at 600 × g , 20°C, and resuspend in 10 ml DPBS.
3.Centrifuge once more and resuspend in 500 μl isolation buffer.
4.Add 100 μl blocking reagent and 100 μl antibody mix (both supplied in monocyte isolation kit) and mix well by pipetting gently after all components are added.
5.Incubate 20 min at 2°-8°C.
6.While cells are incubating, wash the beads.
-
Vortex vial of beads well (∼20 s) to ensure that they are evenly resuspended.
-
Add 500 μl beads to 5 ml isolation buffer in a 15-ml centrifuge tube.
-
Place tube in a magnetic stand and leave for 4 min.
-
Remove buffer and resuspend beads in 500 μl isolation buffer.
7.Wash cells once by adding 10 ml isolation buffer, centrifuging 4 min at 600 × g , and removing buffer.
8.Resuspend cells in 500 μl isolation buffer.
9.Add 500 μl washed beads to the cells and incubate 15 min at 2°-8°C with gentle rocking.
10.Place tube in the magnetic stand for 4 min to separate the unwanted bead-bound cells.
11.Transfer monocyte-containing supernatant to a new 15-ml tube and place in the magnetic stand for another 4 min.
12.Transfer supernatant to a new tube and count monocytes.
13.Proceed immediately to assay seeding (see Basic Protocol 3).
Basic Protocol 3: SEEDING OF CAR T CELLS, MONOCYTES, AND K562 CELLS IN 96-WELL PLATES
This protocol describes how to seed the assay in a 96-well plate. Before beginning this protocol, the user should have performed Basic Protocols 1 and 2 and have the CAR T cells prepared in culture, ready to be used in the assay.
It is important that an appropriate plate layout be planned in advance in order to determine the total number of cells needed for seeding. The number of target cells, monocytes, and CAR T cells needed will depend on how many CAR T cell products/constructs/conditions are being tested. An example layout is shown in Figure 3.The plate should be set up so that each CAR T cell construct or donor is performed in triplicate. Controls should include antigen-negative target cells, wells without monocytes, wells without CAR T cells, and wells with medium only. An untransduced T cell control can also be used in place of one of the CAR T cell constructs.
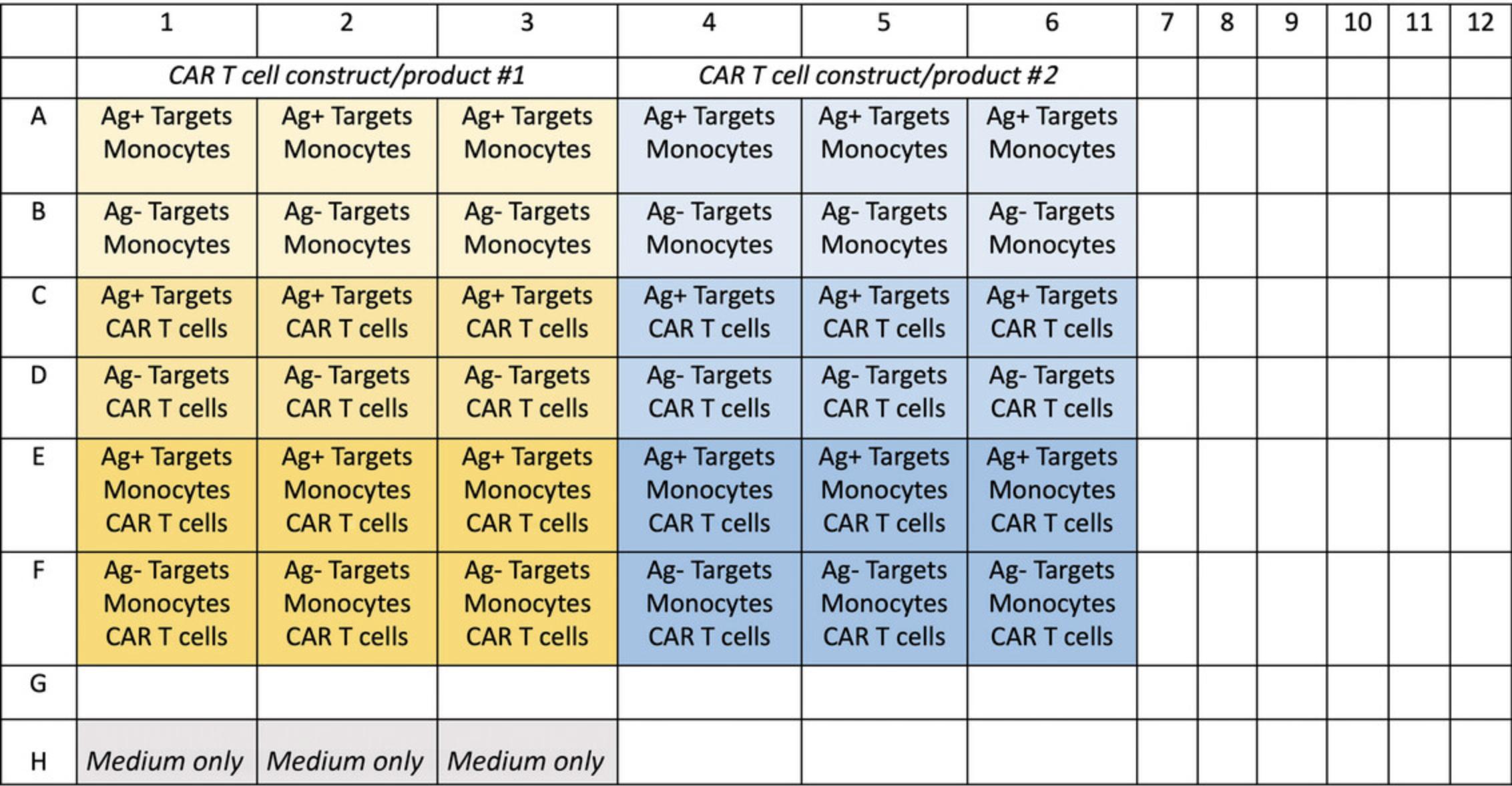
Additional Materials (also see Basic Protocols 1 and 2)
- K562 target cells (see Basic Protocol 1), with and without CD19 expression
- Isolated monocytes (see Basic Protocol 2)
- CAR T cells
- 96-well U-bottom plate (Nunclon Delta Surface, Thermo Fisher Scientific, cat. no. 168136)
- Centrifuge for 96-well plates
1.Collect K562 target cells and CAR T cells from culture and place in 50-ml centrifuge tubes.
2.Count cells and calculate the total number needed for each cell type.
3.Centrifuge all cell types 4 min at 600 × g , 20°C, remove supernatant, and resuspend in complete IMDM at a concentration of 2 × 106 cells/ml.
4.Using your plate plan (e.g., Fig. 3), seed cells as follows to give a 2:1:1 ratio:
- 100 μl CAR T cells (2 × 105 cells)
- 50 μl monocytes (1 × 105 cells)
- 50 μl target cells (1 × 105 cells)
In control wells, replace missing cell type(s) with complete IMDM so that all wells contain 200 μl.
5.Incubate 24 hr at 37°C.
6.Centrifuge plate 2 min at 900 × g.
7.Transfer 160 μl supernatant to a new plate and freeze at −20°C until needed for cytokine analysis (see Basic Protocol 4 and Alternate Protocol).
Basic Protocol 4: ANALYSIS OF CO-CULTURE SUPERNATANTS BY SINGLE-CYTOKINE ELISA
This protocol details how to perform a single-cytokine (in this case IL-6) ELISA with cytokine supernatants collected from the overnight co-culture. It is recommended to perform this protocol while optimizing the assay or as a lower cost pre-screening step. IL-6 is a key cytokine involved in driving CRS and will provide sufficient indication of assay performance during the optimization/pre-screening stage. For a more comprehensive picture of a CAR construct's potential for CRS, Basic Protocol 5 is recommended.
An alternative ELISA for another clinically relevant cytokine, such as IL-1 or IFN-γ, could be used for the same purpose. These alternative/additional ELISAs will need to be optimized separately.
Additional Materials (also see Basic Protocols 1 and 2)
-
ELISA MAX Deluxe Set Human IL-6 kit (BioLegend, cat. no. 430504) including:
- 5× coating buffer
- 200× IL-6 capture antibody
- 5× assay diluent
- IL-6 standard
- 200× IL-6 detection antibody
- 1000× Avidin-HRP
- TMB substrates A and B
-
Distilled water
-
Tween 20 (Sigma-Aldrich, cat. no. P9416)
-
Co-culture supernatants (see Basic Protocol 3)
-
ELISA Stop Solution (2 N H2SO4, R&D Systems, cat. no. DY994)
-
Nunc MaxiSorp ELISA 96-well plate (BioLegend, cat. no. 423501)
-
Multichannel pipette and reagent reservoir
-
Plate sealers (BioLegend, cat. no. 423601)
-
Plate shaker (e.g., Thermo Fisher Scientific, cat. no. 88882006)
-
Plate reader (e.g., Tecan)
-
Data analysis and graphing software (e.g., Excel and Prism)
NOTE : All ELISA reagents should be brought to room temperature before use.
Prepare coated assay plate (day before assay)
1.Prepare 12 ml of 1× coating buffer by diluting 2.4 ml of 5× stock in 9.6 ml distilled water.
2.Add 60 μl of 200× capture antibody to give a 1× solution.
3.Add 100 μl to each well of an ELISA plate using a multichannel pipette. Seal the plate and incubate overnight at 2°-8°C.
Perform ELISA
4.Prepare wash buffer by adding 250 μl Tween 20 to a 500-ml bottle of DPBS.
5.Wash plate four times with 200 μl/well wash buffer.
6.Prepare the blocking agent by diluting 12 ml of 5× assay diluent in 48 ml DPBS. Add 200 μl to each well and incubate 1 hr at room temperature with constant shaking (850 rpm).
7.While the plate is incubating, thaw plate(s) containing co-culture supernatants and dilute samples 1:5 in complete IMDM.
8.Prepare a serial dilution of IL-6 standard according to the kit's instructions.
9.When blocking is complete, wash plate four times with wash buffer.
10.Remove the last wash and add 100 μl of all diluted samples and standards to the plate.
11.Seal plate and incubate 2 hr at room temperature with constant shaking (850 rpm).
12.Wash plate four times with wash buffer.
13.Prepare a 1× solution of detection antibody by adding 60 μl of 200× stock to 12 ml assay diluent.
14.Add 100 μl to each well of the ELISA plate and incubate 1 hr at room temperature with constant shaking (850 rpm).
15.Wash plate four times with wash buffer.
16.Dilute 1000× Avidin-HRP to 1× by adding 12 μl to 12 ml assay diluent.
17.Add 100 μl to each well using a multichannel pipette and incubate 30 min at room temperature with constant shaking (850 rpm).
18.Wash plate five times with wash buffer.
19.Add 6 ml TMB substrate A to 6 ml TMB substrate B and mix briefly by vortexing.
20.Add 100 μl to each well using a multichannel pipette and incubate in the dark until the desired color has developed (∼2-10 min or until standard 4 is visibly blue).
21.Add 100 μl stop solution to each well using a multichannel pipette.
22.Measure absorbance at 450 and 570 nm.
Analyze data
23.Transport plate reader files to Excel and subtract all 450-nm values from their corresponding 570-nm values.
24.Average the values of the medium-only wells and subtract from all other well readings to remove medium background.
25.Transfer data to PRISM and log-transform the data using the “Analyze” feature. In the dropdown menu, choose “Transform” and then “X=Log(X)”.
26.Using the “Analyze” option again, fit a non-linear regression line (curve fit) to the log-transformed data.
27.Under “Analyze”, interpolate unknown sample values from the standard curve with a 95% confidence interval.
28.Under “Analyze” and then “Transform”, transform the interpolated values using “X=10^X”.
29.Using the resulting values, generate a bar graph presented as IL-6 concentration in pg/ml (Fig. 4).
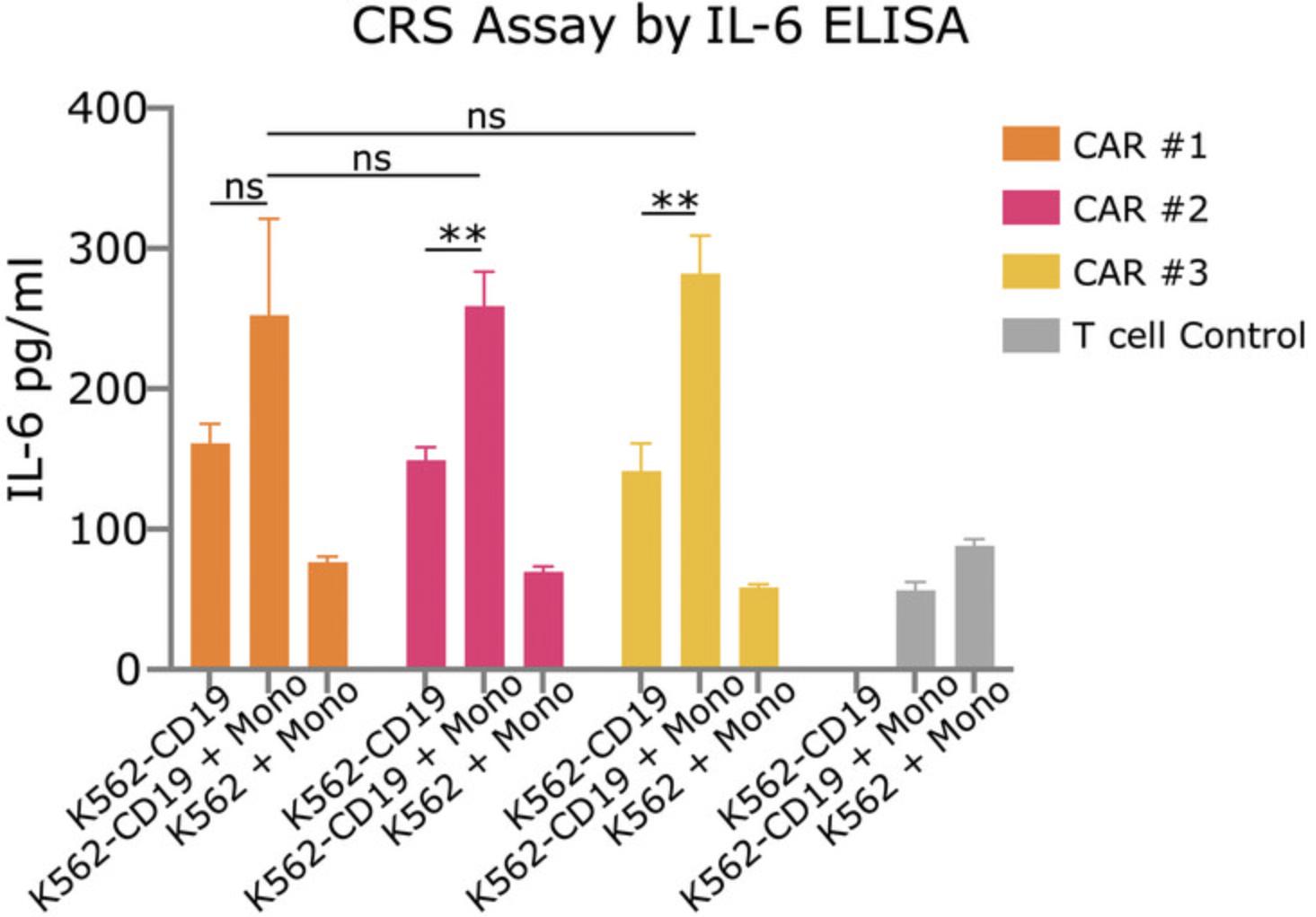
Alternate Protocol: ANALYSIS OF CO-CULTURE SUPERNATANTS BY MULTIPLEX CYTOKINE BEAD ARRAY
This protocol details how to perform the Bio-Plex 27-Plex Cytokine Array. This readout provides information on many of the clinically relevant cytokines for CRS. If a smaller cytokine array is used, it is recommended to ensure it includes IL-6, IFN-γ, GM-CSF, IL-10, and IL-2.Due to its higher cost, this assay should be used following optimization/prescreening with a single-cytokine ELISA.
This protocol is unchanged from the manufacturer's instructions provided with the Bio-Plex 27-Plex Cytokine Array kit. Refer to the generic protocol supplied with kit for more detail.
Materials
- Co-culture supernatants (see Basic Protocol 3)
- Bio-Plex Pro Human Cytokine 27-plex Assay (Bio-Rad, cat. no. M500KCAF0Y)
- Luminex 200 System or equivalent
1.Thaw co-culture supernatants.
2.Use a multichannel pipette to add 196 μl assay medium to the wells of a new 96-well U-bottom plate in a configuration that mirrors the sample plate.
3.Add 4 μl of each sample to the corresponding well of medium to give a 1:50 dilution.
4.Prepare standards following the kit instructions.
5.Vortex 1× Luminex beads for ∼20 s to ensure they are evenly suspended, then add 50 μl beads to each well of the plate.
6.Use the Luminex 200 System to wash the plate two times with 100 μl wash buffer per well.
7.Add 50 μl diluted samples and standards to the plate and incubate 30 min at room temperature on with shaking at 850 rpm.
8.Use the Luminex 200 System to wash the plate three times with 100 μl wash buffer per well.
9.Add 25 μl of 1× detection antibody to each well and incubate 30 min at room temperature with shaking at 850 rpm.
10.Wash as in step 8.
11.Add 50 μl of 1× streptavidin-PE to each well and wrap the plate in foil to shield it from light. Incubate 10 min at room temperature with shaking at 850 rpm.
12.Wash as in step 8.
13.Read plate using the Bio-Plex Manager Software and manufacturer's instructions.
14.Analyze data using Prism or preferred analysis software.
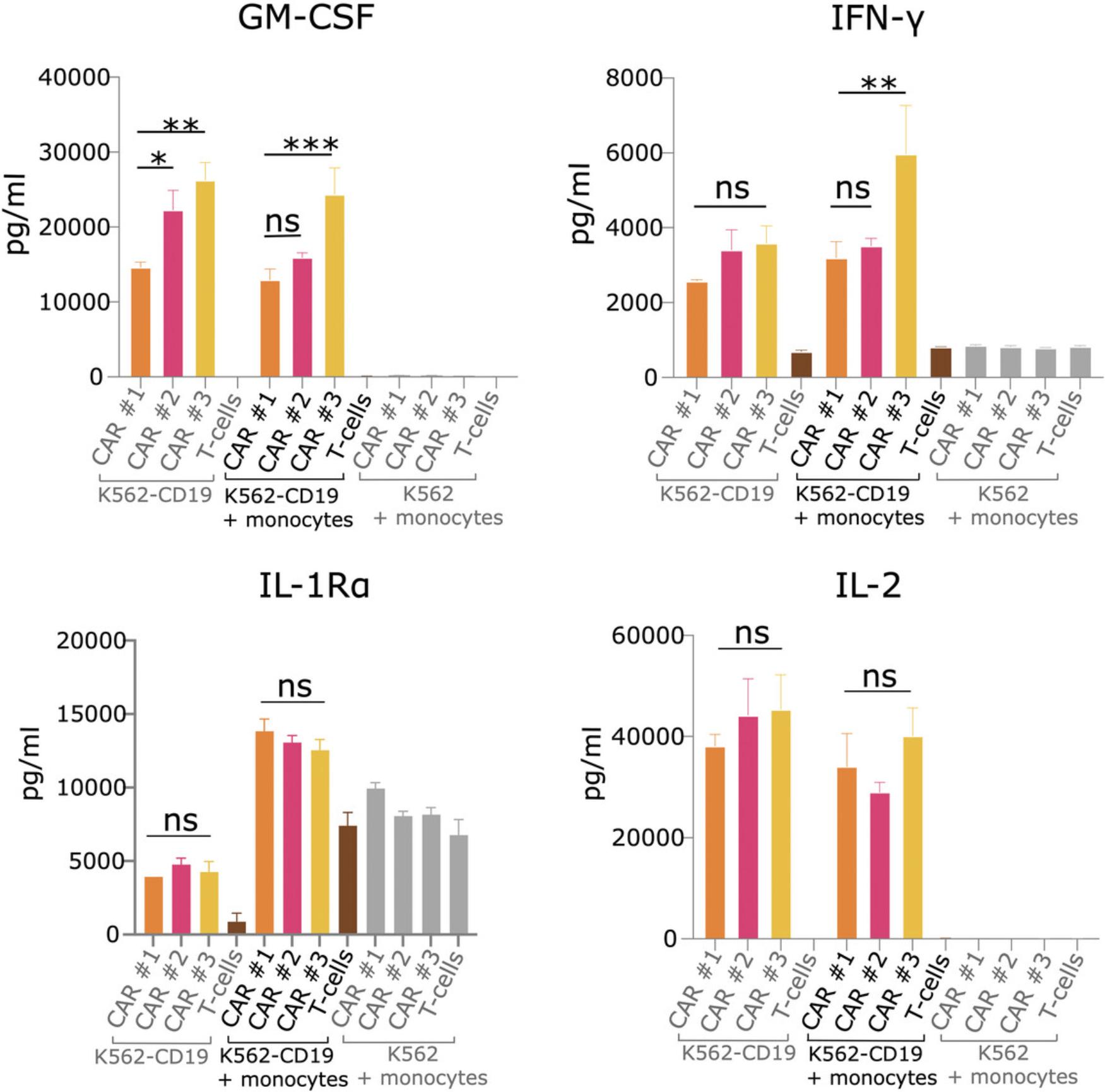
REAGENTS AND SOLUTIONS
Complete IMDM
- 500 ml Gibco Iscove's modified Dulbecco's medium (IMDM; Thermo Fisher Scientific, cat. no. 12440053)
- 50 ml Gibco fetal bovine serum (FBS; Thermo Fisher Scientific, cat. no. 16000044)
- 5 ml Gibco penicillin-streptomycin (Thermo Fisher Scientific, cat. no. 15070063)
- Store up to 1 month at 4C
Isolation buffer
- 50 ml Dulbecco's phosphate-buffered saline (DPBS; Thermo Fisher Scientific, cat. no. 14190144)
- 50 μl FBS (Thermo Fisher Scientific cat. no. 15575020)
- 200 μl 0.5 M EDTA (final 2 mM)
- Store up to 1 month at 4°C
COMMENTARY
Background Information
CAR T cells have provided a significant leap of progress in the treatment of certain hematological malignancies. Despite their high success rate, CAR T cell–related toxicities such as CRS and ICANS are still observed in the majority of patients (Bachy et al., 2022). These toxicities can be life-threatening and may pose an even higher risk when CAR T cells are used against other non-blood malignancies. Understanding the propensity of any given CAR construct to induce toxicities in patients is a critical step in advancing preclinical research to the clinic, and yet this has not historically been prioritized prior to clinical trials.
CRS is driven by the levels of cytokines produced following CAR T cell infusion and activation by target cells. However, assessing cytokine production by CAR T cells in a simple CAR T cell/tumor cell co-culture is not sufficient for assessing CRS risk. This is because CRS pathology can in large part be attributed to the role of endogenous monocytes, which become activated and produce cytokines following CAR T cell infusion (Giavridis et al., 2018; Norelli et al., 2018). This mechanism means that xenografts in NSG mice are also insufficient for assessing CRS risk, as they do not contain an endogenous immune system that can interact with activated tumor cells. Complicated mouse models developed for the purpose of assessing CRS do exist, but are expensive to maintain and require labor-intensive experimental procedures and a high level of specific expertise. For these reasons, they cannot be used as a relatively fast screening tool for CAR constructs. Thus, an in vitro assay containing monocytes alongside tumor cells and CAR T cells can be a useful tool for interrogating CRS (Singh et al., 2017).
While there are clear advantages to the development of an in vitro tool for assessing CRS/ICANS potential, there are also several limitations to this method. First, because this is an in vitro assay, it lacks the tissue architecture of an in vivo model. Second, because the monocytes, CAR T cells, and target cells are all human, it is difficult to disentangle which cytokines are produced by which cell type. These limitations can be addressed with the use of an in vivo mouse xenograft model, although these have their own limitations. Therefore, there remains an important role for an in vitro CRS assay.
It is recommended that this assay be used as a screening tool for CAR T cells that do not have a known toxicity profile. It can be used to assess multiple CAR constructs alongside each other, prior to assessing them in more complicated in vivo models, or furthering them in the research pipeline.
Critical Parameters and Troubleshooting
This assay should be performed using CAR T cells of high quality and purity. Cell ratios are calculated using absolute CAR T cell numbers in the product, and thus CAR T cell percentage in the product should be as close to 100% as possible in order to reduce potential background cytokine production from untransduced T cells in the co-culture. If the CAR T cell population is not of high purity, an enrichment step should be considered. Furthermore, if multiple CAR T cell constructs or products are being compared (e.g.,, the same construct transduced into two different donors), it is critical that the percentage of CAR T cells in the products at the time of assay seeding be nearly identical. If one product is 75% CAR T cells and the other is 85%, untransduced T cells from the same donor should be seeded into the 85% product in order to bring the CAR T cell percentage down to 75%. This ensures there will not be confounding effects on the results from different numbers of untransduced T cells or total cell numbers.
Monocytes should be isolated from thawed PBMCs derived from the blood of the same donor used to generate the CAR T cells in order to mitigate any alloreactivity between the CAR T cells and monocytes. Monocyte isolation should be performed immediately after thawing to prevent the monocytes from adhering to the plasticware in which they were stored.
If more than one type of cytokine readout is performed on different days (for example, two separate single-cytokine ELISAs or a preliminary ELISA and a multiplex array), co-culture supernatants should be divided accordingly before being frozen to avoid multiple freeze/thaw cycles. Supernatants should be frozen in 96-well U-bottom plates, one per readout assay.
Troubleshooting considerations are summarized in Table 1.
Problem | Possible cause | Solution |
---|---|---|
Cytokine concentrations fall outside range of standard curve (ELISA or Bio-Plex) | Supernatant samples are too concentrated or dilute | Find optimal dilution by performing ELISA with multiple dilutions (e.g., 1:2, 1:10, 1:50) |
Triplicate error bars are large | Uneven seeding of wells | Ensure that cells are vortexed/mixed well before plating to ensure they are homogenously distributed in solution |
Ensure pipetting technique is consistent | ||
Use a multichannel pipette | ||
Fewer monocytes are isolated that expected | Monocytes may be sticking to plasticware during isolation | Begin isolation immediately after thawing cells, as allowing them to rest after thawing may cause them to become “sticky” |
Understanding Results
This assay can be used as a way to measure the cytokine production of CAR T cells in the presence of autologous monocytes. Due to the nature of CAR T cell–associated toxicities, which are known to be largely driven by CAR T cell/monocyte interactions, this assay can act as a tool for identifying the potential of a given CAR T cell product to induce toxicities. Three cell types are needed for this assay: CAR T cells (or untransduced T cell control), monocytes (autologous to the CAR T cells), and target cells that express the CAR antigen (along with an antigen-negative control). This protocol does not include a CAR T cell transduction/expansion protocol, as this will be highly specific to the user's own requirements for their CAR T cells. The intention of this protocol is to aid in the screening of already-generated CAR T cell products. Basic Protocol 1, which describes culturing of the target cells, is specific to K562/K562-CD19 target cells. These are useful cell lines if the CAR being investigated targets CD19. They are easily cultured and should grow relatively quickly. The protocol has been performed correctly if the cells need splitting every 3-4 days and viability is high. If alternative target cells are used, a culture protocol specific to those cells should be used. Monocyte isolation should be performed within one hour of thawing in order to prevent monocytes from adhering to the plasticware they are thawed into. The protocol has been successful if the monocyte yield is approximately 10% of the number of PBMCs.
The IL-6 ELISA results, shown in Figure 4, are an example of an expected result for Basic Protocol 3. With the right controls, maximum cytokine production should be seen in wells containing CAR T cells with antigen-expressing target cells and monocytes. Exclusion of the monocytes should result in decreased production of IL-6, and the use of antigen-negative target cells should result in a further reduction. Wells containing untransduced T cells should produce little IL-6. The three different CAR T cell constructs compared in Figure 4 do not produce significantly different levels of IL-6, but it is possible for IL-6 production to vary significantly depending on the constructs being tested and how they interact with monocytes and target cells in the well.
The 27-plex cytokine array provides information on the levels of 27 different cytokines. Figure 5 shows an example of four different clinically relevant cytokines from one 27-plex array. As with the ELISA, untransduced T cell and antigen-negative target cell control wells should produce little cytokines. Depending on the cytokine being looked at and the contribution of monocytes to its production, the monocyte-excluding wells may or may not have lower levels of cytokine in comparison to wells with monocytes and antigen-expressing target cells. Additionally, depending on the CAR T cell constructs being tested and the differences in their signaling properties, differences between the constructs may not be consistent across all cytokines. In Figure 5, CAR T cell construct #3 displays the highest levels of GM-CSF and IFN-γ, but there is no significant difference among constructs for other cytokines such as IL-1Rα or IL-2. To get a feel for the overall cytokine profiles of the constructs, a heatmap can be generated, but this will not give detailed information on specific levels. As displayed here, it may therefore be more beneficial to look at cytokine graphs individually with a focus on clinically relevant cytokines.
Lastly, it is important to remember that the relationship between in vitro cytokine production and the induction of CRS or ICANS in vivo is not entirely straightforward. Although the levels of several cytokines (such as IFN-γ, GM-CSF, and IL-6) can give a good indication of the propensity of a CAR T cell product to induce toxicities, there is no single predicting cytokine with a direct correlation or threshold level to use as a measure. Therefore, it is important to interpret results in a way that takes into account the overall cytokine profile secreted by the co-culture cells (rather than a single cytokine) and use that as a general indication of potential toxicity rather than a direct prediction. Furthermore, it is imperative that this assay be done using CAR T cells generated from multiple donors, as human cells can vary significantly in cytokine production. As a reference point for constructs between assays, it may be useful to include a construct for comparison that is known to induce toxicities or known to be safe in vivo.
Time Considerations
Target cells should be passaged a minimum of two times before being used in the assay. This should take ∼7-10 days. Monocyte isolation will take 2-3 hr and should be done the day of assay setup. The co-culture procedure has been shown to work well with a 24-hr incubation. Depending on the CAR T cell product being tested and the target cells being used, optimal culture time may vary. Supernatants can then be collected from the co-culture, frozen down at −20°C, and thawed at a later date for cytokine analysis. After thawing, cytokine analysis of supernatants can be performed in 1 day.
Acknowledgments
Y. N. developed the assay protocol under the supervision of R. W. and R. P. at the Malaghan Institute in Wellington, New Zealand. Figures 1 and 2 were generated using BioRender. Funding for this article was provided by the Health Research Council of New Zealand, the University of Otago, and the Malaghan Institute of Medical Research.
Open access publishing facilitated by University of Otago, as part of the Wiley - University of Otago agreement via the Council of Australian University Librarians.
Author Contributions
Yasmin Nouri : Conceptualization, data curation, formal analysis, methodology, writing (original draft); Robert Weinkove : Conceptualization, funding acquisition, project administration, resources, supervision, writing (review and editing); Rachel Perret : Conceptualization, funding acquisition, project administration, resources, supervision, writing (review and editing).
Conflict of Interest
The authors declare no conflict of interest.
Open Research
Data Availability Statement
The data, tools, and material (or their sources) that support the protocol are available from the corresponding author upon reasonable request.
Literature Cited
- Bachy, E., le Gouill, S., Di Blasi, R., Sesques, P., Manson, G., Cartron, G., Beauvais, D., Roulin, L., Gros, F. X., Rubio, M. T., Bories, P., Bay, J. O., Llorente, C. C., Choquet, S., Casasnovas, R. O., Mohty, M., Guidez, S., Joris, M., Loschi, M., … Morschhauser, F. (2022). A real-world comparison of tisagenlecleucel and axicabtagene ciloleucel CAR T cells in relapsed or refractory diffuse large B cell lymphoma. Nature Medicine , 28(10), 2145–2154. https://doi.org/10.1038/s41591-022-01969-y
- Cheng, J., Zhao, L., Zhang, Y., Qin, Y., Guan, Y., Zhang, T., Liu, C., & Zhou, J. (2019). Understanding the mechanisms of resistance to CAR T cell therapy in malignancies. Frontiers in Oncology , 9, 1237. https://doi.org/10.3389/fonc.2019.01237
- Frey, N. V., & Porter, D. L. (2016). Cytokine release syndrome with novel therapeutics for acute lymphoblastic leukemia. Hematology , 2016(1), 567–572. https://doi.org/10.1182/asheducation-2016.1.567
- Giavridis, T., van Der Stegen, S. J. C., Eyquem, J., Hamieh, M., Piersigilli, A., & Sadelain, M. (2018). CAR T cell-induced cytokine release syndrome is mediated by macrophages and abated by IL-1 blockade. Nature Medicine , 24(6), 731–738. https://doi.org/10.1038/s41591-018-0041-7
- Gong, T., Liu, L., Jiang, W., & Zhou, R. (2020). DAMP-sensing receptors in sterile inflammation and inflammatory diseases. Nature Reviews Immunology , 20, 95–112. https://doi.org/10.1038/s41577-019-0215-7
- Guo, H., Qian, L., & Cui, J. (2022). Focused evaluation of the roles of macrophages in chimeric antigen receptor (CAR) T cell therapy associated cytokine release syndrome. Cancer Biology and Medicine , 19, 333–342. https://doi.org/10.20892/j.issn.2095-3941.2021.0087
- Gust, J., Ponce, R., Liles, W. C., Garden, G. A., & Turtle, C. J. (2020). Cytokines in CAR T cell–associated neurotoxicity. Frontiers in Immunology , 11, 3271. https://doi.org/10.3389/fimmu.2020.577027
- Hao, Z., Li, R., Meng, L., Han, Z., & Hong, Z. (2020). Macrophage, the potential key mediator in CAR-T related CRS. Experimental Hematology and Oncology , 9, 1–12. https://doi.org/10.1186/s40164-020-00171-5
- Ho, W. Y., Blattman, J. N., Dossett, M. L., Yee, C., & Greenberg, P. D. (2003). Adoptive immunotherapy: Engineering T cell responses as biologic weapons for tumor mass destruction. Cancer Cell , 3, 431–437. https://doi.org/10.1016/S1535-6108(03)00113-2
- June, C. H., O'Connor, R. S., Kawalekar, O. U., Ghassemi, S., & Milone, M. C. (2018). CAR T cell immunotherapy for human cancer. Science , 359, 1361–1365. https://doi.org/10.1126/science.aar6711
- Kenderian, S. S., Ruella, M., Shestova, O., Kim, M., Klichinsky, M., Chen, F., Kengle, N., Lacey, S., Melenhorst, J., June, C. H., & Gill, S. I. (2017). Ruxolitinib prevents cytokine release syndrome after CAR T cell therapy without impairing the anti-tumor effect in a xenograft model. Biology of Blood and Marrow Transplantation , 23(3), S19–S20. https://doi.org/10.1016/j.bbmt.2016.12.003
- Lee, D. W., Santomasso, B. D., Locke, F. L., Ghobadi, A., Turtle, C. J., Brudno, J. N., Maus, M. V., Park, J. H., Mead, E., Pavletic, S., Go, W. Y., Eldjerou, L., Gardner, R. A., Frey, N., Curran, K. J., Peggs, K., Pasquini, M., Dipersio, J. F., van den Brink, M. R. M., … Neelapu, S. S. (2019). ASTCT consensus grading for cytokine release syndrome and neurologic toxicity associated with immune effector cells. Biology of Blood and Marrow Transplantation , 25, 625–638. https://doi.org/10.1016/j.bbmt.2018.12.758
- Liu, Y., Fang, Y., Chen, X., Wang, Z., Liang, X., Zhang, T., Liu, M., Zhou, N., Lv, J., Tang, K., Xie, J., Gao, Y., Cheng, F., Zhou, Y., Zhang, Z., Hu, Y., Zhang, X., Gao, Q., Zhang, Y., & Huang, B. (2020). Gasdermin E-mediated target cell pyroptosis by CAR T cells triggers cytokine release syndrome. Science Immunology , 5(43). https://doi.org/10.1126/sciimmunol.aax7969
- Melenhorst, J. J., Chen, G. M., Wang, M., Porter, D. L., Chen, C., Collins, M. A., Gao, P., Bandyopadhyay, S., Sun, H., Zhao, Z., Lundh, S., Pruteanu-Malinici, I., Nobles, C. L., Maji, S., Frey, N. V., Gill, S. I., Loren, A. W., Tian, L., Kulikovskaya, I., … June, C. H. (2022). Decade-long leukaemia remissions with persistence of CD4+ CAR T cells. Nature , 602(7897), 503–509. https://doi.org/10.1038/s41586-021-04390-6
- Murthy, H., Iqbal, M., Chavez, J. C., & Kharfan-Dabaja, M. A. (2019). Cytokine release syndrome: Current perspectives. ImmunoTargets and Therapy , 8, 43–52. https://doi.org/10.2147/itt.s202015
- Norelli, M., Camisa, B., Barbiera, G., Falcone, L., Purevdorj, A., Genua, M., Sanvito, F., Ponzoni, M., Doglioni, C., Cristofori, P., Traversari, C., Bordignon, C., Ciceri, F., Ostuni, R., Bonini, C., Casucci, M., & Bondanza, A. (2018). Monocyte-derived IL-1 and IL-6 are differentially required for cytokine-release syndrome and neurotoxicity due to CAR T cells. Nature Medicine , 24(6), 739–748. https://doi.org/10.1038/s41591-018-0036-4
- Sachdeva, M., Duchateau, P., Depil, S., Poirot, L., & Valton, J. (2019). Granulocyte macrophage colony-stimulating factor inactivation in CAR T cells prevents monocyte-dependent release of key cytokine release syndrome mediators. Journal of Biological Chemistry , 294(14), 5430–5437. https://doi.org/10.1074/jbc.AC119.007558
- Sadelain, M., Brentjens, R., & Rivière, I. (2013). The basic principles of chimeric antigen receptor design. Cancer Discovery , 3, 388–398. https://doi.org/10.1158/2159-8290.CD-12-0548
- Sadelain, M., Rivière, I., & Brentjens, R. (2003). Targeting tumours with genetically enhanced T lymphocytes. Nature Reviews Cancer , 3, 35–45. https://doi.org/10.1038/nrc971
- Santomasso, B. D., Park, J. H., Salloum, D., Riviere, I., Flynn, J., Mead, E., Halton, E., Wang, X., Senechal, B., Purdon, T., Cross, J. R., Liu, H., Vachha, B., Chen, X., Deangelis, L. M., Li, D., Bernal, Y., Gonen, M., Wendel, H. G., … Brentjens, R. J. (2018). Clinical and biological correlates of neurotoxicity associated with CAR T cell therapy in patients with B-cell acute lymphoblastic leukemia. Cancer Discovery , 8(8), 958–971. https://doi.org/10.1158/2159-8290.CD-17-1319
- Singh, N., Hofmann, T. J., Gershenson, Z., Levine, B. L., Grupp, S. A., Teachey, D. T., & Barrett, D. M. (2017). Monocyte lineage–derived IL-6 does not affect chimeric antigen receptor T cell function. Cytotherapy , 19(7), 867–880. https://doi.org/10.1016/j.jcyt.2017.04.001
- Sterner, R. C., & Sterner, R. M. (2021). CAR-T cell therapy: Current limitations and potential strategies. Blood Cancer Journal , 11, 1–11. https://doi.org/10.1038/s41408-021-00459-7
- Sterner, R. M., Sakemura, R., Cox, M. J., Yang, N., Khadka, R. H., Forsman, C. L., Hansen, M. J., Jin, F., Ayasoufi, K., Hefazi, M., Schick, K. J., Walters, D. K., Ahmed, O., Chappell, D., Sahmoud, T., Durrant, C., Nevala, W. K., Patnaik, M. M., Pease, L. R., … Kenderian, S. S. (2019). GM-CSF inhibition reduces cytokine release syndrome and neuroinflammation but enhances CAR-T cell function in xenografts. Blood , 133(7), 697–709. https://doi.org/10.1182/blood-2018-10-881722
- Titov, A., Petukhov, A., Staliarova, A., Motorin, D., Bulatov, E., Shuvalov, O., Soond, S M., Piacentini, M., Melino, G., Zaritskey, A., & Barlev, N A. (2018). The biological basis and clinical symptoms of CAR-T therapy-associated toxicities. Cell Death and Disease , 9, https://doi.org/10.1038/s41419-018-0918-x
- Xiao, X., Huang, S., Chen, S., Wang, Y., Sun, Q., Xu, X., & Li, Y. (2021). Mechanisms of cytokine release syndrome and neurotoxicity of CAR T cell therapy and associated prevention and management strategies. Journal of Experimental and Clinical Cancer Research , 40, 1–23. https://doi.org/10.1186/s13046-021-02148-6