A Simple Quantitative Assay for Measuring β-Galactosidase Activity Using X-Gal in Yeast-Based Interaction Analyses
Laura Trimborn, Laura Trimborn, Ute Hoecker, Ute Hoecker, Jathish Ponnu, Jathish Ponnu
Abstract
Yeast-based interaction assays to determine protein-protein and protein-nucleic acid interactions commonly rely on the reconstitution of chimeric transcription factors that activate the expression of target reporter genes. The enzyme β-galactosidase (β-gal), coded by the LacZ gene of Escherichia coli , is a widely used reporter in yeast systems, and its expression is commonly assessed by evaluating its activity. X-gal (5-bromo-4-chloro-3-indolyl-β-d-galactopyranoside) is an inexpensive and sensitive substrate of β-gal, whose hydrolysis results in an intensely blue colored and easily detectable end product, 5,5′-dibromo-4,4′-dichloro-indigo. The insoluble nature of this end product, however, makes X-gal-based assays unsuitable for direct spectrophotometric absorbance quantification. As such, the use of X-gal is mostly restricted to solid-support approaches, such as colony lift or agar plate assays, which often only provide a qualitative readout. In this article, we describe a quantitative solid-phase X-gal assay to measure protein-protein interaction strength in yeast cells using a simple and low-cost experimental setup. We have optimized multiple aspects of the assay, namely sample preparation, reaction time, and quantification method, for speed and consistency. By integrating the use of a freely available ImageJ-based plugin, we have further standardized the assay for reliability and reproducibility. This improved quantitative X-gal assay can be performed in a standard molecular biology lab without the need for any specialized equipment other than an inexpensive and widely accessible smartphone camera. To exemplify the protocol, we provide detailed step-by-step instructions to perform a quantitative X-gal assay to assess the interaction between two Arabidopsis thaliana proteins, SUPPRESSOR OF PHYA-105 1 (SPA1) and PRODUCTION OF ANTHOCYANIN PIGMENT 2 (PAP2). To demonstrate the sensitivity of our assay in detecting weaker interactions, we also compare the results with a liquid-phase assay that uses ONPG (ortho -nitrophenyl-β-galactopyranoside) as a substrate for β-gal. The quantitative X-gal assay described here can easily be adapted for high-throughout interaction studies and protein domain mapping, even in yeast strains with low levels of LacZ expression. © 2022 The Authors. Current Protocols published by Wiley Periodicals LLC.
Basic Protocol 1 : Preparation of competent yeast cells and transformation
Alternate Protocol 1 : In-house preparation of yeast competent cells for use in lithium acetate (LiAc)–mediated yeast transformation
Support Protocol : Long-term storage and revival of frozen yeast strain stocks
Basic Protocol 2 : Measuring β-galactosidase activity via the quantitative X-gal assay
Alternate Protocol 2 : Quantification of interaction strength using liquid ONPG assay
INTRODUCTION
Protein-protein and protein-nucleic acid interactions are central to cellular processes, and their study provides important insights into their roles and the molecular processes they control. Yeast (Saccharomyces cerevisiae)–based assays have been established as powerful and reliable tools for studying physical molecular interactions (Ouwerkerk & Meijer, 2001; Paiano, Margiotta, De Luca, & Bucci, 2019). These assays are based on the modularity of transcription factors, which was initially described for GAL4 (Brent & Ptashne, 1985), a transcription factor that binds to the specific upstream activation sequences (UASs) of galactose-induced genes. GAL4 contains two essential but separable domains: the N-terminal DNA-binding domain (BD), which binds UASs, and the C-terminal transcriptional activation domain (AD), necessary to activate transcription. While BD binds UASs even in the absence of AD (Keegan, Gill, & Ptashne, 1986), GAL4 transcriptional activity (i.e., expression of UASs-harboring genes) can only be restored when the BD and AD are in close proximity, that is, when the AD is recruited to UASs-containing genes (Fields & Song, 1989; Keegan et al., 1986). In a GAL4-based Y2H assay, genes encoding two proteins of interest (POIs) are separately cloned and expressed in compatible yeast strains, with one (the bait) fused to the BD of GAL4 and the other (the prey) to the AD. Interaction between the POIs brings the BD and AD into proximity, which reconstitutes a functional GAL4 transcription factor, leading to the expression of reporter genes harboring the UASs in their promoters (Fields & Song, 1989; also see Figure 1A). While GAL4-based yeast two-hybrid approaches are undoubtedly the most popular, the LexA system is also widely employed and is based on the same principles. This system uses the bacterial LexA protein as BD and a viral protein, VP16 (also known as B42AD), as AD (Criekinge & Beyaert, 1999). Since its development, multiple adaptations of the Y2H approach have been reported to study the interaction of different biomolecules. For instance, yeast one-hybrid (Y1H) uses DNA and protein as bait and prey (Ouwerkerk & Meijer, 2001), while Y3H involves bait-prey interactions via an adjoining hybrid RNA molecule (Cottier et al., 2011) or mediated by a third “bridge” protein (Maruta, Trusov, & Botella, 2016).
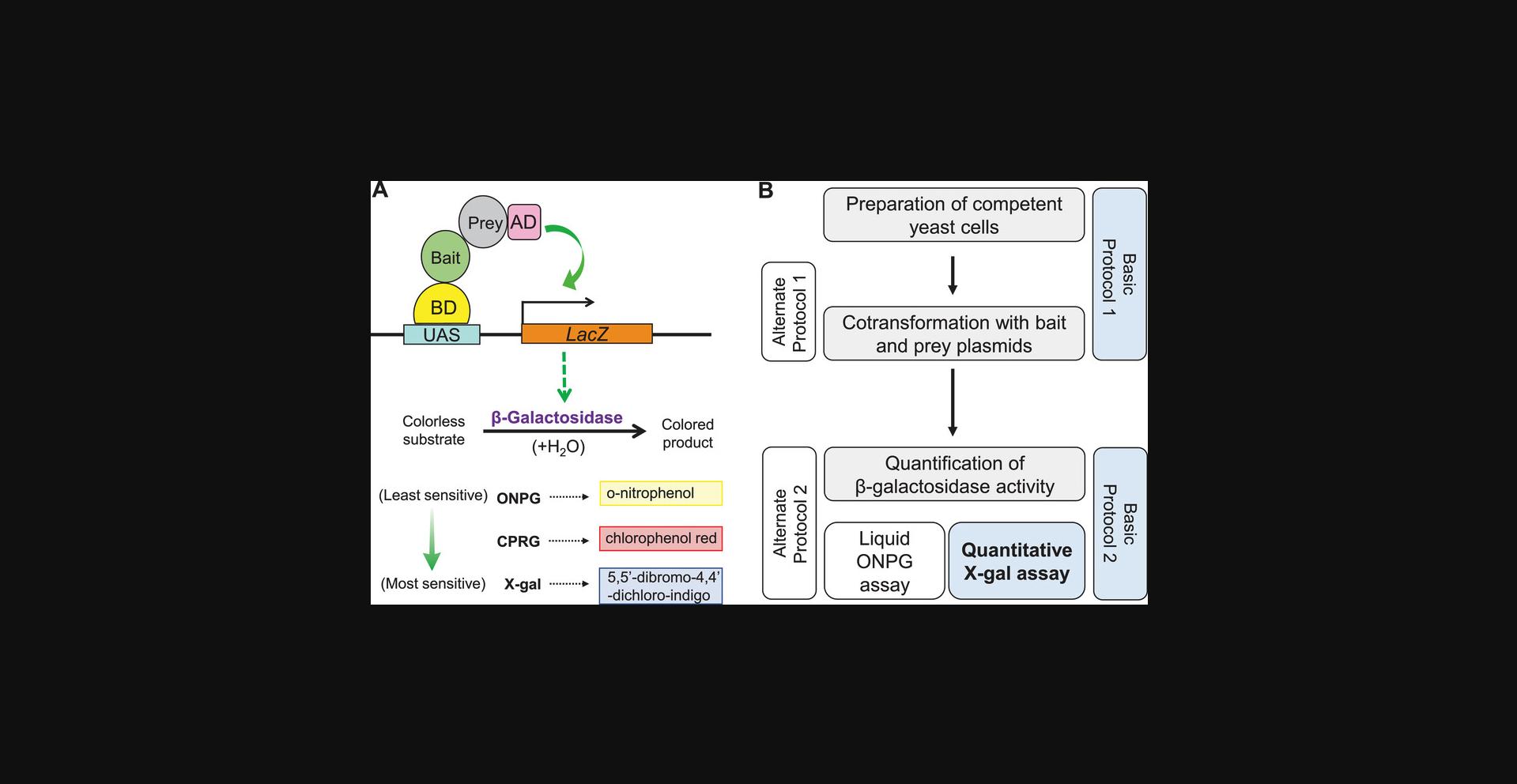
β-Galactosidase (β-gal), encoded by the LacZ gene of Escherichia coli (E. coli), is one of the most versatile reporters used in yeast systems (Rose & Botstein, 1983; Figure 1; Yocum, Hanley, West, & Ptashne, 1984). Several differentially sensitive colorimetric assays, based on various substrates, have been established to monitor β-gal activity in yeast. For example, hydrolysis of one of the most commonly used β-gal substrates, ONPG (ortho -nitrophenyl-β-galactopyranoside), results in a soluble yellow-colored byproduct, o -nitrophenol, which enables quantification of interaction strength via spectrophotometric absorbance measurements (Schaefer, Jovanovic, Kotta-Loizou, & Buck, 2016). On the other hand, X-gal (5-bromo-4-chloro-3-indolyl-β-d-galactopyranoside), commonly used in solid-support approaches such as colony lift or agar plate assays, results in an intensely blue-colored end product, 5,5′-dibromo-4,4′-dichloro-indigo, that can be easily visualized (Golemis & Khazak, 1997; also see Figure 1A). While X-gal assays are more sensitive, this insoluble blue precipitate cannot be used directly for absorbance measurements in liquid form via a spectrophotometer, as it settles down at the bottom of the cuvettes. Purifying and resuspending the precipitate can circumvent this problem in an E. coli –based assay (Levitsky, Toledo-Aral, López-Barneo, & Villadiego, 2013), but this is impractical in yeast systems due to the difficulty in separating the precipitate from yeast debris.
The choice of β-gal substrate depends on the sensitivity needed for the interaction assay. While stronger interactions can be easily quantified using ONPG-based assays, many important interactions are weak, necessitating more sensitive assays, such as those based on X-gal. However, the most commonly used X-gal-based colony lift or agar plate assays allow only visual inspection of the blue color developed, but do not provide quantitative measures of relative interaction strengths. Quantitative data on strength of interactions or affinity are fundamental for understanding protein function, and allowing the formulation of novel hypotheses for building predictive models. As the strength of interactions measured in yeast two-hybrid systems can generally be correlated with affinity measurements done in vivo (Estojak, Brent, & Golemis, 1995), standardization of a quantitative X-gal assay would be of great benefit.
Attempts have been made in the past to quantify the blue color developed in X-gal assays. An initial effort (Serebriiskii, Toby, & Golemis, 2000) was based on the X-gal overlay assay (Duttweiler, 1996), which required specialized skills and involved the more labor-intensive replica-plate technique. In a different approach (Möckli & Auerbach, 2004), pellets of yeast cells from actively growing liquid cultures were treated with X-gal-containing buffer in agarose, which allowed blue color development and analysis in 96-well microtiter plates. Even though both methods differed in sample preparation, they both utilized flatbed scanners for image capture. While partly solving the underlying issue, these methods were not widely adopted, perhaps due to the extended time involved in performing the assays, cumbersome procedures, the specialized skills required, and vague or outdated methods for quantification from scanned images.
Here we describe a simple protocol for the quantification of X-gal activity (quantitative X-gal assay), which we have optimized for speed and consistency. Our method can be performed reliably under standard laboratory conditions with minimum effort. Moreover, we have improved upon previous protocols by integrating the use of modern and inexpensive smartphone cameras and a freely available and advanced image processing plugin that permits much easier and more accurate quantification. To determine the standardized amount of β-gal in the X-gal assay, we also provide a formula to calculate β-gal units, similar to Miller Units used in ONPG assay (Miller, 1972; Zhang & Bremer, 1995).
An outline of the workflow is shown in Figure 1B. Basic Protocol 1 details both the preparation of competent yeast cells, using a suitable yeast strain that harbors the LacZ reporter system, and yeast co-transformation with bait and prey plasmids, using the Frozen-EZ Yeast Transformation IITM Kit (Zymo Research) with minor modifications. In Alternate Protocol 1, we describe competent yeast preparation and co-transformation following the traditional (but more time-consuming) lithium acetate (LiAc)–mediated method. In the Support Protocol, we describe the steps for long-term storage and revival of yeast strain stocks. The co-transformed yeast colonies obtained following either Basic Protocol 1 or Alternate Protocol 1 are then plated, harvested, and processed before proceeding with the quantitative X-gal assay, described in Basic Protocol 2. A flowchart of the main workflow for the quantitative X-gal assay described in Basic Protocols 1 and 2, is depicted in Figure 2. Lastly, in Alternate Protocol 2, we describe a liquid assay using ONPG as substrate for β-gal, which allows direct comparison and demonstrates the enhanced sensitivity of quantitative X-gal assay for detecting weaker interactions.
![Details are in the caption following the image Overview of the quantitative X-gal assay. (Top) The competent yeast cells co-transformed with bait and prey plasmids are plated on synthetically defined media lacking leucine and tryptophan (SD/–LW) to select colonies containing both plasmids. For details, see Basic Protocol 1. (Bottom) An example of quantitative X-gal assay using two SD/–LW plates (1 and 2; depicted in gray) containing recombinant yeast colonies expressing different sets of bait and prey fusion proteins. Orange and purple colors represent the fate of colonies from plates 1 and 2, respectively. Three biological replicates (Brep 1, 2 and 3) containing 5 yeast colonies each are prepared per SD/–LW plate by picking, resuspending, and replating on square Petri dishes as droplets or spots. Ten spots are made for each biological replicate, and the three biological replicates from one co-transformation plate (SD/–LW) are placed on three different square plates to avoid “plate effects”. After incubation at 30°C, the cells from each biological replicate (10 spots) are harvested, combined, and processed. The resulting cell suspensions are transferred into a white 96-well plate. For each biological replicate, two technical replicates are prepared [e.g., the technical replicates of Brep1 from SD/–LW plate 1 (orange) are placed in wells A1 and B1]. Column 1 of the 96-well plate contains 3 biological replicates with 2 technical replicates each from the SD/–LW plate 1 (A1 to F1; orange). Likewise, column 2 (A2 to F2; violet) has replicates from SD/–LW plate 2. Well G1 contains negative control without yeast cells. The rest of the wells in the 96-well plate are empty. In the presence of the X-gal master mix, intense blue color development occurs in the samples in which the bait and prey proteins interact (Column 1). The intensity of the blue color can be quantified from the photographs of the 96-well plate using the ImageJ-based plugin ReadPlate 3.0. From the obtained values, the β-galactosidase activity can be calculated and represented graphically. For detailed steps, see Basic Protocol 2.](https://static.yanyin.tech/literature_test/cpz1421-fig-0002-m.jpg)
STRATEGIC PLANNING
The choice of a suitable Y2H system is crucial for performing a successful protein-protein interaction assay. As mentioned earlier, the most commonly used systems are GAL4 and LexA. As no Y2H system is capable of detecting every protein-protein interaction, the system should be chosen based on data available in the literature, and considering the structural and functional aspects of the proteins involved. For example, to study the interactions involving membrane proteins, modified Y2H systems are recommended (Fetchko & Stagljar, 2004). To detect the interaction between two POIs that have never been tested before, both GAL4 and LexA systems could be tested initially via simple assays, such as growth assays on selective media or X-gal-based visual assays (Yeast Protocols Handbook from Clontech). Afterwards, the more sophisticated quantitative assays described here could be performed, in a need-based manner.
The initial step in a standard Y2H assay involves the subcloning of cDNA sequences of POIs into suitable vectors that allow the expression of bait and prey as fusion proteins in compatible yeast strains. Users need to generate the required plasmids before embarking on the protocols described here. Several yeast subcloning vectors are available in both GAL4 and LexA systems, involving different cloning strategies. When choosing the vectors, note that the subcloning vector for expressing the BD-fusion should be compatible with the reporter yeast strain, such that the strain must harbor reporter genes with appropriate UASs that are recognized by the BD. The AD vectors are generally interchangeable, as the function of the AD in Y2H is to activate transcription, and does not depend on the UASs of reporter genes.
The desired way of expressing the POI, either as BD- or AD-fusions, often depends on the nature of the protein. Transcription factors, when used as BD-fusions, frequently activate reporter gene expression constitutively, even in the absence of the AD (i.e., by autoactivation), which can be circumvented either by adding competitive inhibitors like 3-AT (3-amino-1,2,4-triazole) in growth assays involving HIS3 as a reporter, by or swapping the protein from BD to AD in quantitative assays (Criekinge & Beyaert, 1999).
The extent of reporter expression is another criterion to consider when choosing yeast strains. The information on yeast strains typically used in different Y2H systems, their expected reporter expression, and the compatible vectors are detailed in Yeast Protocol Handbook from Clontech (https://static.yanyin.tech/literature/current_protocol/10.1002/cpz1.421/attachments/PT3024-1.pdf). The individual vectors and compatible yeast strains are available commercially (Takara/Clontech) or can be obtained from non-profit plasmid repositories such as Addgene (https://www.addgene.org/). With the advent of modern cloning techniques, versatile vector systems are available for performing Y2H assays, which utilize, for instance, Gateway technology® (Invitrogen, Thermo Fisher Scientific), in vitro site-specific recombination (Hartley, Temple, & Brasch, 2000), ligation-independent cloning like Gibson Assembly® (New England Biolabs; Gibson et al., 2009), or modular cloning techniques (Engler, Kandzia, & Marillonnet, 2008; Gantner et al., 2018). For high-throughput Y2H screening, users are referred to relevant publications (Koegl & Uetz, 2007; Paiano et al., 2019; Striebinger, Koegl, & Bailer, 2013).
We describe here an example of a Y2H quantitative X-gal assay using two specific POIs, SUPPRESSOR OF PHYA-105 1 (SPA1) and PRODUCTION OF ANTHOCYANIN PIGMENT 2 (PAP2), from the model plant Arabidopsis thaliana (Arabidopsis). In plants, SPA proteins, along with CONSTITUTIVELY PHOTOMORPHOGENIC1 (COP1), form the COP1/SPA E3 ubiquitin ligase (Ponnu & Hoecker, 2021), a repressor of light signaling that is most active in the dark, which facilitates, via polyubiquitination, the degradation of many target proteins involved in light-driven growth and development. The transcription factor PAP2 (Borevitz, Xia, Blount, Dixon, & Lamb, 2000; Maier et al., 2013), a degradation target of COP1/SPA complex and a promoter of anthocyanin biosynthesis in the light, interacts with both COP1 and SPA1.
In the following protocols, we use the GAL4 Y2H system to probe the interaction between SPA1 and PAP2 by expressing the respective cDNAs as BD- (bait) and AD-(prey) fusions. To this end, we used, respectively, the BD and AD vectors pAS2-1 (https://www.addgene.org/98135/) and pACT2 (https://www.addgene.org/vector-database/1675/), which have been modified by replacing the multiple cloning site with Gateway® sites, to allow site-specific recombination (Hartley et al., 2000). These modified destination vectors are available from the authors upon request. The pAS2-1 vector contains the DNA sequence encoding the GAL4 binding domain (BD) without the STOP codon, downstream of the ADH1 promoter, followed by AttR1 and AttR2 sites for Gateway® recombination to clone the desired gene in-frame. The AD vector pACT2 contains an SV40 nuclear localization signal, followed by the GAL4 activation domain (AD) without the STOP codon, downstream of the ADH1 promoter, followed by AttR sites. The BD and AD vectors allow the selection of co-transformed yeast colonies in yeast synthetic dropout (SD) media without leucine and tryptophan, due to the presence of TRP1 (nutritional marker gene coding for phosphoribosyl-anthranilate isomerase, for tryptophan biosynthesis) and LEU2 (coding isopropyl malate dehydrogenase, for leucine biosynthesis) cassettes, respectively. The pUC and 2μ origins of replication present in both vectors allow the replication of the plasmid in E. coli and yeast, respectively, making them shuttle vectors suitable for cloning in bacteria and expression in yeast. For selection in E.coli , both vectors carry the ampicillin-resistance gene (Ampr). Between the AttR sites, both vectors contain the ccdB gene, which kills the colony of the ccdB-sensitive strain expressing the empty vector (Bernard, 1996) and facilitates the selection of positive clones. As the first step to construct BD- and AD-fusions, the cDNAs of SPA1 and PAP2 were amplified from Arabidopsis and cloned into an intermediate entry vector, pENTR3C (Gateway®pENTRTM Invitrogen; https://www.addgene.org/vector-database/2527/) via Gibson Assembly® (Gibson et al., 2009; New England Biolabs). The entry clones were verified via Sanger sequencing, and then used for the Gateway® LR reaction employing the LR clonase II master mix (ThermoFisher) with the BD and AD vectors to generate pAS2-1_SPA1 and pACT2_PAP2. Selected colonies obtained after transformation were verified by restriction digestion and Sanger sequencing. The complete list of bait and prey constructs used in this manuscript is provided in Table 1.
Column number | Bait | Prey |
---|---|---|
1 | pAS2-1-SPA1 | pACT2-PAP2 |
2 | pAS2-1-SPA1-WD | pACT2-PAP2 |
3 | pAS2-1-SPA1 | pATC2-GW |
4 | pAS2-1-SPA1-WD | pATC2-GW |
5 | pAS2-1-GW | pACT2-PAP2 |
6 | pAS2-1-GW | pATC2-GW |
- a Column numbers correspond to the columns in the 96-well plate described in Basic Protocol 2 and Figure 5A. SPA1, SUPPRESSOR OF PHYA-105 1; PAP2, PRODUCTION OF ANTHOCYANIN PIGMENT 2; SPA1-WD, the WD40-repeat domain of SPA1; GW, Gateway®.
For the Y2H assay exemplified here, the BD- and AD-fusion clones are transformed into the compatible yeast strain Y190 (Harper, Adami, Wei, Keyomarsi, & Elledge, 1993), auxotrophic for tryptophan, leucine, and cycloheximide (cyhr2). Y190 has two reporter systems: HIS3 , for auxotrophic plate assay, and LacZ , for β-gal assay. Thus, upon bait-prey interaction, Y190 yeast cells can grow in plates without histidine, and the interaction can be analyzed via β-gal assays. The LacZ reporter in Y190 is tightly regulated to avoid false positives. Y190 has a comparatively high expression of LacZ upon induction, making it a suitable strain for both sensitive and less sensitive assays using X-gal and ONPG, respectively, and is thus the strain used here. The non-transformed Y190 can be selected and maintained in media without uracil (Yeast Protocols Handbook, Clontech).
Plates with yeast selection medium are required for Basic Protocols 1 and 2. Depending on the assay systems used (GAL4 or LexA), plates with different synthetically defined (SD) media containing selective dropout (DO) supplements should be prepared in advance and stored at 4°C for up to 4 months (Treco & Lundblad, 1993).
Basic Protocol 1: PREPARATION OF COMPETENT YEAST CELLS AND TRANSFORMATION
This protocol describes the preparation of competent yeast cells using the Y190 strain and subsequent transformation with BD and AD clones (Table 1; also see Strategic Planning). For general guidelines on preparing yeast media and selection plates, please refer to the Yeast Protocols Handbook from Clontech. For the generation of competent yeast cells and transformation, this protocol uses a commercially available kit (Frozen-EZ Yeast Transformation IITM Kit, Zymo Research). The competent cells created via this method can be frozen at −80°C for future use. We first describe how to set up and grow overnight cultures of Y190 and how to prepare competent cells, followed by a method of slow freezing that allows retaining the competency of frozen cells. We then describe the steps involved in yeast transformation using the bait and prey constructs. For a more commonly used but time-consuming transformation method using lithium acetate (LiAc), please refer to Alternate Protocol 1.
Materials
-
Yeast strain: Any compatible strains can be used (see Strategic Planning); here, we use Y190 maintained on Yeast Extract-Adenine-Peptone-Dextrose (YAPD) agar plates (Support Protocol)
-
YAPD liquid medium (see recipe)
-
Frozen-EZ Yeast Transformation IITM Kit (Zymo Research, cat. no. T2001)
-
Bait and prey plasmid DNA (see Strategic Planning and Table 1; >400 ng/μl each)
-
SD/–LW agar medium (see recipe) in 92-mm-diameter agar plates ( Sarstedt, cat. no. 82.1473.001)
-
80% ethanol (see recipe)
-
15-cm-long glass culture tube (VWR, cat. no. 212-0472)
-
Spectrophotometer (e.g., Eppendorf BioPhotometer® D30 or equivalent)
-
1-ml clear-sided polycarbonate single-use cuvettes (BRAND, cat. no. 759115)
-
Sterile 50-ml Erlenmeyer flask (VWR, cat. no. 201-1386 or equivalent)
-
Sterile pipette tips
-
15-ml polycarbonate tubes (Sarstedt, cat. no. 62.554.502 or equivalent)
-
Shaking incubator at 30°C for tubes and plates (Infros-HT Ecotron or equivalent)
-
Pipettes (2.5 μl, 20 μl and 200 μl, 5 ml)
-
Centrifuge 5810 R (Eppendorf or equivalent)
-
Sterile 1.5-ml microcentrifuge tubes (e.g., Sarstedt, cat. no. 72.690.001)
-
Vortex mixer (VWR, Vortex VV3, cat. no. 444-007 or equivalent)
-
Sterile 3-mm glass beads (VWR, cat. no. 1.04015.0500 or equivalent)
-
Biosafety cabinet (e.g., Kojair® BioWizard Silver Line Biosafety cabinet)
-
Parafilm (VWR, cat. no. 291-1212)
Preparation of competent yeast cells
1.Inoculate a single colony of Y190 from a stock agar plate (Support Protocol) into a 15-cm long glass culture tube containing 5 ml of YAPD liquid medium.
2.Incubate overnight (up to 16 hr) at 30°C with constant shaking at 200 rpm.
3.The next day, measure the optical density at 600 nm (OD600) of a 1:10 dilution of the overnight culture (i.e., 100 μl of the overnight culture in 900 μl YAPD) using a spectrophotometer.
4.In a fresh 50-ml Erlenmeyer flask, dilute the overnight culture with YAPD medium to an OD600 = 0.3 in a final volume of 10 ml. To calculate the amount of overnight culture needed, use the following formula:
\begin{equation*} \frac{{\bm{Vf}{\rm;{}};0.3}}{{ODM{\rm;{}};DF}} \end{equation*}
5.Let the yeast culture grow at 30°C with constant shaking at 200 rpm until the OD600 is between 0.8 and 1.0.
6.Transfer the culture to a 15-ml polycarbonate tube and pellet the cells by centrifuging 4 min at 500 × g , room temperature.
7.Carefully discard the supernatant using a 5-ml pipette, without disturbing the pellet.
8.Add 10 ml of Frozen-EZ Yeast Solution 1 (Frozen-EZ Yeast Transformation IITM Kit, Zymo Research) and carefully resuspend the pellet by pipetting up and down.
9.Pellet the cells again by centrifugation for 4 min at 500 × g , room temperature.
10.Add 1 ml of Frozen-EZ Yeast Solution 2 (Frozen-EZ Yeast Transformation IITM Kit, Zymo Research) to the tube. Resuspend the cells by slowly pipetting up and down.
11.Dispense the cells in 1.5-ml microcentrifuge tubes as 50-μl aliquots. At this point, the competent cells can directly be used for transformation (see next step), or slowly frozen (see note below) and stored at −80°C for future use.
Transformation of competent cells with bait and prey plasmids
12.For each transformation, add 1 μg each of bait and prey plasmids in less than 5 μl of total volume to a clean 1.5-ml microcentrifuge tube. In addition, prepare a control 1.5-ml tube without any plasmid DNA, to rule out contamination in the competent cells, and include the proper positive and negative controls.
13.Thaw the frozen yeast competent cells at room temperature. Add 50 μl of competent cells (aliquot from one tube of competent cells; see step 11) to each microcentrifuge tube.
14.Add 500 μl of Frozen-EZ Yeast Solution 3 (Frozen-EZ Yeast Transformation IITM Kit, Zymo Research) to each tube and mix well by pipetting up and down.
15.Incubate the tubes at 30°C for 45 min. In between, vortex the tubes briefly at least 2-3 times (once in every 10-15 min).
16.Transfer 50-150 μl of each transformation mix onto separate SD/–LW plates and spread using sterile glass beads. To evenly spread the mix, place 4-7 sterile glass beads onto each plate. Then, cover with lid and move each plate forward and backward, and left to right, at least three times in each direction.
17.Open and allow the plates to dry for 10 min in a biosafety cabinet.
18.Remove the glass beads (by pouring them into a bottle containing 80% ethanol) and wrap the plates with Parafilm to prevent loss of moisture from the plates during incubation.
19.Invert the wrapped plates and incubate for 3-4 days at 30°C, until colonies appear.
Alternate Protocol 1: IN-HOUSE PREPARATION OF YEAST COMPETENT CELLS FOR USE IN LITHIUM ACETATE (LiAc)–MEDIATED YEAST TRANSFORMATION
As an alternative to the kit-based preparation of competent yeast cells and transformation described in Basic Protocol 1, we provide here a LiAc-mediated protocol (adapted from the Yeast Protocol Handbook, Clontech). Compared to Basic Protocol 1, this method is more time-consuming, and the competent cells cannot be stored for more than 4 hr after preparation. However, compared to Basic Protocol 1, this method is cheaper, as it does not involve a commercial kit. In this protocol, yeast cells from an overnight culture are pelleted, washed in 1× TE buffer, and resuspended in 1× TE buffer containing LiAc, before being used directly for transformation. The following protocol should yield sufficient competent cells for 16 transformations. For a general overview, see Figure 2.
Materials
-
Yeast strain: Any compatible strains can be used (see Strategic Planning); here, we use Y190 maintained on YAPD agar plates (Support Protocol)
-
Y190 Genotype: MATa, ura3-52, his3-D200, lys2-801, ade2-101, trp1-901, leu2-3, 112, gal4D, gal80D, URA3::GAL1UAS-GAL1TATA-lacZ, cyhr2, LYS2::GALUAS-HIS3TATA-HIS3.Origin of UAS – GAL1; UAS regulated by GAL4; high expression of LacZ upon induction by a positive Y2H interaction.
-
YAPD liquid medium (see recipe)
-
10× TE buffer, pH 7.5 (see recipe)
-
Double-distilled water
-
1 M LiAc (see recipe)
-
50% (w/v) polyethylene glycol (PEG; see recipe)
-
Prey and bait plasmid DNA (see Strategic Planning and Table 1)
-
Optional : Salmon sperm DNA (Thermo Fisher Scientific, cat. no. 15632011)
-
100% DMSO (Carl Roth, cat. no. A994)
-
SD/–LW agar medium (see recipe) in 92-mm-diameter agar plates ( Sarstedt, cat. no. 82.1473.001)
-
15-cm long glass culture tube (VWR, cat. no. 212-0472)
-
1-ml clear-sided polycarbonate single-use cuvettes (BRAND, cat. no. 759115)
-
Spectrophotometer (e.g., Eppendorf BioPhotometer® D30 or equivalent)
-
Centrifuge 5810 R (Eppendorf or equivalent)
-
15-ml polycarbonate tubes (Sarstedt, cat. no. 62.554.502 or equivalent)
-
50-ml tubes
-
Pipettes (2.5 μl, 20 μl and 200 μl, 5 ml)
-
Sterile pipette tips
-
Sterile 1.5-ml microcentrifuge tubes (e.g., Sarstedt, cat. no. 72.690.001)
-
Heat block
-
Vortex mixer (VWR, Vortex VV3, cat. no. 444-007 or equivalent)
-
Shaking incubator at 30°C for tubes and plates (Infros-HT Ecotron or equivalent)
-
30° and 42°C water baths
-
Parafilm (VWR, cat. no. 291-1212)
-
Sterile 3-mm glass beads (VWR, cat. no. 1.04015.0500 or equivalent)
Generation of yeast competent cells
1.Follow Basic Protocol 1, steps 1 to 4, to obtain an actively growing yeast culture at an OD600 between 0.8 and 1.0.
2.Prepare the following working solutions freshly under sterile conditions (for stock solutions, please refer to Reagents and Solutions):
1× TE solution :
10× TE buffer | 1 ml |
Double-distilled water | 9 ml |
TE/LiAc solution :
10× TE buffer | 1 ml |
1 M LiAc | 1 ml |
Double-distilled water | 8 ml |
PEG/LiAc solution :
10× TE buffer | 1 ml |
1 M LiAc | 1 ml |
50% (w/v) PEG | 8 ml |
3.Transfer the yeast culture into two 50-ml tubes and pellet the cells for 5 min at 2000 × g , room temperature.
4.Remove the supernatant and resuspend each pellet in 25 ml of sterile water. Combine all the cells in one of the 50-ml tubes.
5.Centrifuge for 5 min at 2000 × g , room temperature. Remove the supernatant and resuspend the pellet in 1 ml of TE/LiAc solution.
Yeast transformation
6.Follow Basic Protocol 1, step 12, to prepare the samples for transformation.
7.Keep the tubes with the plasmids on ice.
8.Optional : Use salmon sperm DNA. To denature the salmon sperm DNA for use, boil for 5 min in a heat block and cool on ice immediately to avoid renaturing. Add 10 μl of salmon sperm DNA to each tube.
9.Add 60 μl of competent yeast cell solution (from step 5) to 1.5-ml microcentrifuge tubes containing the bait and prey plasmids, or the control.
10.Add 300 μl of PEG/LiAc to each tube and mix by vortexing vigorously for 5 to 10 s.
11.Incubate the samples for 30 min at 30°C in a water bath and gently mix every 10 min.
12.Add 35 μl of DMSO to each sample and mix carefully by inverting the tubes. Incubate the samples at 42°C in a water bath for 20 min and carefully mix them every 5 min.
13.Chill the cells on ice for 1-2 min. Pellet the cells by centrifugation for 2 min at 2000 × g , at room temperature.
14.Remove the supernatant and carefully resuspend the cells in 200 μl of 1× TE buffer.
15.Transfer 100 μl of each sample to different plates with appropriate dropout medium (e.g., SD/-LW) and spread using sterile glass beads. To evenly spread the mix, place four to seven sterile glass beads onto each plate. Then, cover with lid and move the plate forward and backward, and left to right, at least three times in each direction.
16.Follow Basic Protocol 1, steps 17 to 19, and incubate the plates at 30°C until colonies appear.
Support Protocol: LONG-TERM STORAGE AND REVIVAL OF FROZEN YEAST STRAIN STOCKS
The following protocol provides detailed instructions for the long-term storage and subsequent recovery of yeast strains. Long-term storage of yeast strains is achieved by resuspending a single colony of yeast from a fresh YAPD agar plate (the master plate) in YAPD medium and glycerol before transferring to a −80°C freezer. For recovery, a small amount of frozen yeast cells is streaked onto a YAPD agar plate and grown at 30°C until colonies appear. Fresh colonies of yeast strains can be maintained in solid YAPD medium [e. g., YAPD without uracil (–Ura) for Y190] at 4°C for a maximum of 2 weeks. Fresh colonies can be obtained by streaking the old colonies onto new medium. However, repeating this many times (passage) may lead to the accumulation of, unwanted spontaneous mutations. Hence, always revive the yeast cells from frozen glycerol stocks maintained at −80°C.
Materials
-
Yeast strain (e.g., Y190) grown on a YAPD agar plate
-
YAPD liquid medium (see recipe)
-
Sterile 50% (v/v) glycerol in water (see recipe)
-
YAPD agar (see recipe) in polycarbonate disposable Petri dishes, 60 × 15 mm, 10 mm grid size (VWR, cat no. 25384-093 or equivalent)
-
200-μl pipette tips
-
1.5-ml screw-cap microcentrifuge tubes (Sarstedt, cat. no. 72.692.005)
-
Vortex mixer (VWR, Vortex VV3, cat. no. 444-007 or equivalent)
-
Parafilm (VWR, cat. no. 291-1212)
Long-term storage of yeast strains (adapted from Yeast Protocol Handbook, Clontech)
1.Pick a single yeast colony with a diameter of ∼2 mm from the YAPD master plate using a 200-μl pipette tip under sterile conditions.
2.Resuspend the colony in 500 μl of YAPD medium in a 1.5-ml screw-cap tube.
3.Vortex the tube vigorously to disperse the cells.
4.Add 500 μl of 50% (v/v) glycerol to the tube. Tightly close the cap.
5.Invert the tube and vortex several times to mix the cells and glycerol.
6.Label the tube and store at −80°C.
Reviving frozen yeast stocks
7.Scrape small amounts of the frozen glycerol stocks using a sterile 200-μl tip and streak them onto a YAPD agar plate under sterile conditions.
8.Seal the plates with Parafilm, invert and incubate for 3-5 days at 30°C, until yeast colonies reach ∼2 mm diameter. Use these as master plates or working stock.
9.Use the colonies from the above plates for the preparation of competent cells (Basic Protocol 1 or Alternate Protocol 1).
Basic Protocol 2: MEASURING β-GALACTOSIDASE ACTIVITY VIA THE QUANTITATIVE X-gal ASSAY
Here, we describe the quantification of β-gal activity using X-gal as substrate. An overview of the protocol is given in Figure 2.In this protocol, several co-transformed yeast colonies selected on SD plates and belonging to one plasmid combination (e.g., BD-SPA1 + AD-PAP2) are combined, resuspended in sterile water, and plated as droplets on fresh SD plates. The lawn of cells obtained after 48 hr of growth at 30°C is scrapped, pelleted, and used for X-gal assay in 96-well microtiter plates in PBS buffer with agarose. The plates are then incubated at room temperature to allow blue color development, which can be quantified by first taking photographs using a smartphone camera or a DSLR camera and then analyzing the images with an ImageJ-based plugin.
All the steps in Basic Protocol 2 should be performed under sterile conditions. When testing novel interactions, we recommend doing a colony lift assay (Bass, Reece-Hoyes, & Walhout, 2016) on half of the freshly transformed yeast cells. Once blue color development occurs in colony lift assay, proceed with the quantitative X-gal assay using the remaining transformed colonies.
Materials
-
Master plates containing co-transformed yeast colonies from Basic Protocol 1 or Alternate Protocol 1
-
Sterile double-distilled water
-
SD/–LW agar medium (see recipe) in square Petri plates (Greiner bio-one, cat. no. 391-0465)
-
Z-buffer (see recipe)
-
80% (v/v) ethanol (see recipe)
-
Liquid nitrogen
-
0.5% w/v agarose in 1× PBS solution (see recipe)
-
1× PBS, pH 7.4 (see recipe for 10×)
-
β-mercaptoethanol (Sigma-Aldrich, cat. no. M6250)
-
X-gal (PanReac AppliChem, cat. no. A1007,001)
-
Sterile 1.5-ml microcentrifuge tubes (e.g., Sarstedt, cat. no. 72.690.001)
-
Pipettes (2.5 μl, 20 μl and 200 μl, 5 ml)
-
Sterile pipette tips
-
Biosafety cabinet (e.g., Kojair® BioWizard Silver Line Biosafety cabinet)
-
Vortex mixer (VWR, Vortex VV3, cat. no. 444-007 or equivalent)
-
Shaking incubator at 30°C for tubes and plates (Infros-HT Ecotron or equivalent)
-
Parafilm (VWR, cat. no. 291-1212)
-
Heat block/water bath
-
1-ml clear-sided polycarbonate single-use cuvettes (BRAND, cat. no. 759115)
-
Spectrophotometer (e.g., Eppendorf BioPhotometer® D30 or equivalent)
-
London forceps (Finescience, cat. no. 1180-02)
-
Paper towels
-
Centrifuge 5810 R (Eppendorf or equivalent)
-
White 96-well plate with lid (Greiner bio-one, cat. no. 655083 or equivalent)
-
50-ml polycarbonate centrifuge tubes (Sarstedt, cat. no. 62.547.254 or equivalent)
-
Magnetic stirrer (VWR® Low Profile Magnetic Stirrer, cat. no. 10153-690 or equivalent) and stir bars
-
Multi-dispenser pipette (Eppendorf Multipipette® M4 or equivalent)
-
Multichannel pipette (Brand, Transferpipette® S-12, cat. no. 705932 or equivalent)
-
Camera (Canon EOS 90D; DSLR) or smartphone with a camera (minimum 8 megapixels)
-
Fiji software (https://imagej.net/software/fiji/). Any version released after 2018.
-
ReadPlate 3.0 plugin (https://imagej.nih.gov/ij/plugins/readplate/index.html), latest version
-
Microsoft® Excel or similar software for statistical analyses and plotting
Plating transformed yeast cells on SD (DO/IM) medium
1.Add 750 μl of sterile water to 1.5-ml labeled microcentrifuge tubes (three biological replicates for each transformation).
2.Under sterile conditions, pick five colonies per master plate containing co-transformed yeast colonies (from Basic Protocol 1 or Alternate Protocol 1) using 200-μl sterile pipette tips (use one tip per colony) and resuspend in one of the tubes from step 1.This tube represents biological replicate number 1. Similarly, pick 5 additional colonies from the same master plate two more times and resuspend in the remaining tubes, corresponding to biological replicate numbers 2 and 3, respectively. See the schematic given in Figure 2. Repeat this step for all the samples/master plates.
3.Vortex each tube (each biological replicate) for 3 s. Allow a moment for the cells to settle and repeat vortexing. Repeat this at least 3-5 times or until a homogenous yeast cell suspension is obtained.
4.Pipette ten 20-μl droplets from each biological replicate onto separate square Petri plates with SD/–LW agar medium (Figure 2).
5.At this stage, handle the plates carefully to avoid mixing the cell suspensions.
6.Allow the plates to dry for 20 min in a sterile biosafety cabinet.
7.Seal the plates with Parafilm, invert, and incubate at 30°C for 48 hr. Colonies will be visible in each spot after 48 hr.
Harvesting and processing the yeast cells
8.Prepare one labeled 1.5-ml tube per biological replicate (three tubes per sample) to be harvested, each with 1 ml of Z-buffer.
9.Prepare another set of labeled 1.5-ml tubes (one per biological replicate; 3 tubes per sample) with 950 μl of double-distilled water, for measuring the OD600.
10.Clean the London forceps with 80% ethanol and wipe with a paper towel. Scrape the cells from all 10 spots that belong to each biological replicate from the square plate and combine them by resuspending in the respective labeled 1.5-ml tube containing 1 ml of Z-buffer. Mix the cells by vortexing for 3 s.
11.After collecting each biological replicate, clean the forceps with 80% ethanol and wipe with a paper towel.
12.Transfer 50 μl from each tube into the corresponding 1.5-ml tube containing 950 μl of double-distilled water. Mix thoroughly. Measure the OD600 using a spectrophotometer.
13.Based on the results from OD600 measurements, prepare a yeast suspension of OD600 = 5.0 in a total volume of 2 ml of Z-buffer, using the following formula:
\begin{equation*} \hspace*{-13.9pc}Vc = ;\frac{{{{\bm {Vf}}}ODf}}{{ODMDF}}\quad \quad Vz = ;2 - Vc \end{equation*}
14.Centrifuge the samples 10 min at 5000 × g , room temperature.
15.Carefully remove and discard the supernatant by pipetting. Resuspend cells in 100 μl of Z-buffer.
16.Snap-freeze the samples in liquid nitrogen. At this point, the samples can be stored at −80°C for a maximum period of 2 weeks, or immediately used for the quantitative X-gal assay.
Quantitative X-gal assay
17.Thaw the samples at 30°C in a heat block or water bath. Then re-freeze directly in liquid nitrogen. Repeat the freeze-thaw cycles for two additional times, to permeabilize the cells.
18.Prepare two technical replicates for each biological replicate. For each technical replicate, pipette 50 μl of the lysate into one of the wells of a white 96-well plate (Figure 2).
19.Prepare two blank samples in two separate wells of the same 96-well plate, containing 50 μl of Z-buffer each.
20.Prepare the X-gal master mix. The list below shows the reagents and amount needed for one well (one technical replicate) of the 96-well plate, with a total approximate volume of 330 μl/well. Prepare enough volume of the master mix to account for the total number of samples, including the biological replicates, technical replicates, and blank samples. Make sure to prepare an excess of 10% of the total volume of the master mix to account for pipetting errors.
Reagent | Amount per well |
0.5% Agarose in 1× PBS | 0.2 ml |
1× PBS | 0.13 ml |
β-mercaptoethanol | 0.2 μl |
X-gal | 0.001 g |
To prepare the master mix:
- Add the required amounts of 1× PBS and X-gal powder to a 50-ml centrifuge tube. Vortex thoroughly to dissolve the X-gal.
If X-gal takes time to dissolve, the 50-ml tube can be attached to a rocker and shaken for 10-15 min.
- Add the β-mercaptoethanol.
Use a chemical safety cabinet while working with β-mercaptoethanol.
-
Heat the 0.5% agarose in 1× PBS in a bottle until the agarose is fully melted. Cool the solution with constant stirring with a magnetic stirrer until the bottle can be handled with bare hands (no more than 55°C).
-
Add the warm 0.5% agarose in 1× PBS to the tube containing X-gal/ β-mercaptoethanol master mix. Vortex the tube vigorously for 30 s and immediately proceed to the next step.
Vortex the 50-ml tube several times in between, to prevent solidification of the master mix. Alternatively, depending on the assay and number of samples, the master mix can be prepared in a bottle with a magnetic stirrer and placed on a warm heating block with constant stirring. This may provide sufficient time for pipetting the master mix without solidification.
21.Add 330 μl of the master mix to each well of the 96-well plate containing the yeast lysate or the blank samples. Record the starting time of the reaction. Mix the yeast lysate and the master mix thoroughly using a multichannel pipette.
22.Wait for 10 min after finishing the last sample to ensure full solidification in all the wells. Cover the 96-well plate with a lid, seal using Parafilm, and incubate at room temperature and wait for blue color development.
23.Check blue color development visually at regular intervals of approximately 30 to 60 min.
24.Once the blue color appears on the interaction samples—but not on negative control or blanks—record the reaction time elapsed.
25.Open the plates and scan using a smartphone or DSLR camera. Make sure to avoid the reflection of light or flash, as it may affect quantification.
26.Take pictures of the plates at different time points, while recording the time elapsed.
27.Export the images as .jpg (.jpeg) files from the camera into a computer.
Analyzing the photographs using ReadPlate 3.0
28.Install the software Fiji (https://imagej.net/software/fiji/downloads) on a computer.
29.Install ReadPlate 3.0 plugin in Fiji from https://imagej.nih.gov/ij/plugins/readplate/index.html according to the instructions given on the web page.
30.Start Fiji and open the photograph of a plate in Fiji (see Figure 3A). Click File → Open and choose the image from the directory where it was saved (see Figure 4A).
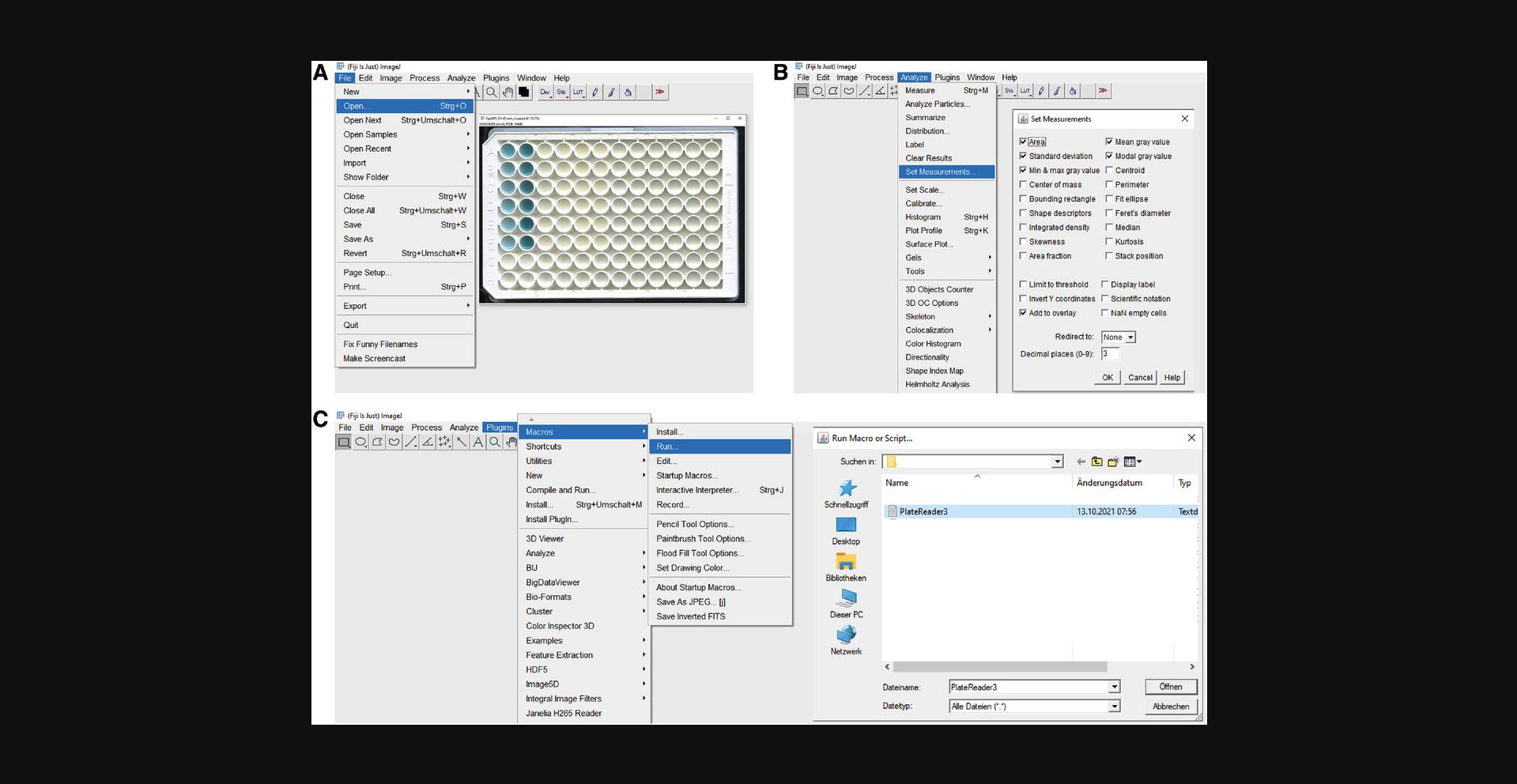
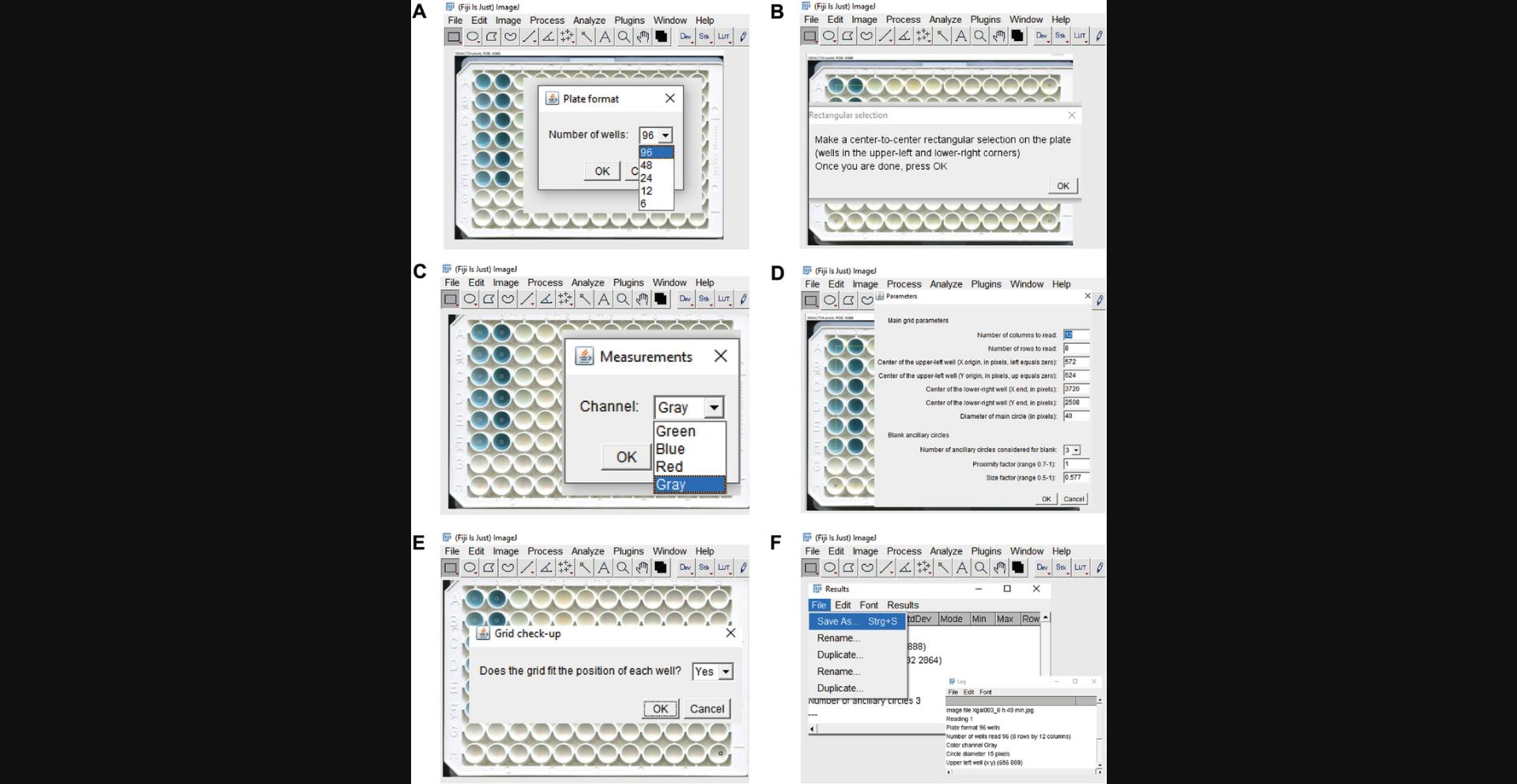
31.Before starting ReadPlate3.0, select the following parameters for measurements (see Figure 3B). Click “Analyze” → “Set Measurements”. Select/tick the following parameters in the resulting window: Area, Standard Deviation, Min&Max Gray value, Mean Gray value, Modal Gray Value, Add to Overlay, and Redirect to None (see Figure 3B). Choose the Decimal places (0-9) as 3 (Figure 3B).
32.Run ReadPlate 3.0 (Figure 3C). Choose Plugins→ Macros→ Run and select ReadPlate3 file from the appropriate directory.
33.Follow the ReadPlate 3.0 instructions:
-
Select “96 wells” as plate format (Figure4A).
-
Make a rectangular selection from the upper-left to the lower-right corner. Once finished, press OK (Figure4B).
-
Select “Gray” as the desired color channel for measurements (Figure4C).
The selected “parameters” shown in Figure4Dare the output according to the previously selected settings.
-
Press “OK” and check whether the final grid shows the main circles centered on each well (see Figure4D).
-
In the following pop-up window, select “Yes” if the grid fits into the positions as desired and the main circles are centered on each well (Figure4E). Click “OK”.
If the main circle is too large/small and does not show up centrally on each well, choose “no” and press “OK”. You will be reverted to the settings window, where the parameter “Diameter of main circle” can be changed. Press “Yes” and confirm “OK” to save the changes.
-
In the next step, two new windows will open, one with the “Log” values showing all parameters set by the user and another with “Results”. Save both files by clicking “File” → “Save As” (see Figure4F). The files will be saved in.csvformat. Add the desired names to each file before saving.
Calculating β-galactosidase activity using Microsoft® Excel
34.Open the .csv file in Excel.
Click “Data” → “From Text” and choose the Results file generated in step 33 from the appropriate directory. Click “Import” → “Save and load”.
35.Calculate β-galactosidase units as follows:
-
Calculate the mean of the technical replicates of the Blank and each sample:\begin{equation*} {\bf{ Mean;}} \bf = \frac{{{\bf {AuncorrTechnRep}}1 \bf + {\bf {AuncorrTechnRep}}\bf 2}}{\bf 2} \end{equation*}
- b.Subtract the blank mean from each sample mean, to obtain corrected value: \begin{equation*} {\bf{Corrected;value;}}\left( {{\bf{BioRep;}}\bf 1} \right) \bf = {\bf{Mean;}}\left( {{\bf{BioRep}}1} \right) - {\bf{Mean;}}\left( {{\bf{Blank}}} \right) \end{equation*}
- c.Calculate the β-galactosidase units: \begin{equation*} {\bf{\beta }} - {\bf{galactosidase;units;}} \bf = \frac{{1,000{\bf{;;Corrvalue}}}}{{{\bf{tVOD}}600}} \end{equation}
- d.
Calculate the mean and the standard deviation from the biological replicates. The values obtained can be plotted in a graph, as shown in Figure 4B. To be considered for the analyses, the data generated from the images should be within the linear range (see step 26). To this end, perform a regression analysis using the values obtained from different time points (see step 26, Figure 5C).
As an example, the raw data and the calculated values for the image shown in Figure 5A are given in Table 2. The β-galactosidase values obtained can be compared with Miller Units (Miller, 1972; Zhang & Bremer, 1995) obtained in an ONPG assay.
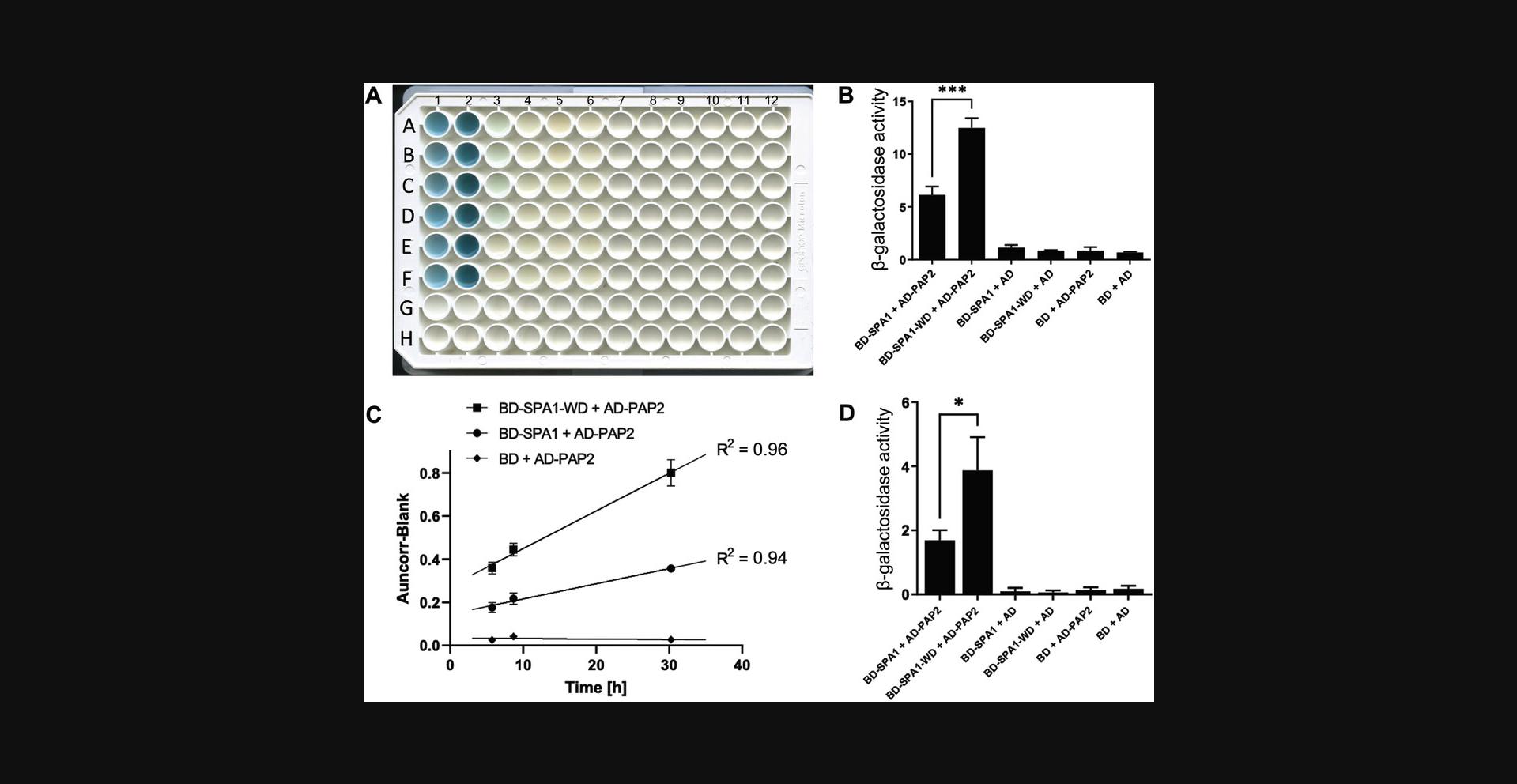
Output of ReadPlate 3.0 | Step 33a | Step 33b | Step 33c | Step 33d | ||||
---|---|---|---|---|---|---|---|---|
Row | Column | Auncorr | Mean (Auncorr technical replicates) | Mean (Sample) - Blank | β-Galactosidase Units | Average (biological replicates) | Standard deviation | |
BD-SPA1 + AD-PAP2 |
A | 1 | 0.292 | 0.2770 | 0.1960 | 6.8170 | 6.1280 | 0.8110 |
B | 1 | 0.262 | ||||||
C | 1 | 0.267 | 0.2630 | 0.1820 | 6.3300 | |||
D | 1 | 0.259 | ||||||
E | 1 | 0.234 | 0.2315 | 0.1505 | 5.2350 | |||
F | 1 | 0.229 | ||||||
BD-SPA1-WD + AD-PAP2 |
A | 2 | 0.416 | 0.4100 | 0.3290 | 11.4430 | 12.4810 | 0.9330 |
B | 2 | 0.404 | ||||||
C | 2 | 0.443 | 0.4475 | 0.3665 | 12.7480 | |||
D | 2 | 0.452 | ||||||
E | 2 | 0.474 | 0.4620 | 0.3810 | 13.2520 | |||
F | 2 | 0.450 | ||||||
BD-SPA1 + AD |
A | 3 | 0.115 | 0.1160 | 0.0350 | 1.2170 | 1.1480 | 0.2510 |
B | 3 | 0.117 | ||||||
C | 3 | 0.119 | 0.1200 | 0.0390 | 1.3570 | |||
D | 3 | 0.121 | ||||||
E | 3 | 0.107 | 0.1060 | 0.0250 | 0.8700 | |||
F | 3 | 0.105 | ||||||
BD-SPA1-WD + AD |
A | 4 | 0.109 | 0.1075 | 0.0265 | 0.9220 | 0.8700 | 0.0522 |
B | 4 | 0.106 | ||||||
C | 4 | 0.105 | 0.1060 | 0.0250 | 0.8700 | |||
D | 4 | 0.107 | ||||||
E | 4 | 0.106 | 0.1045 | 0.0235 | 0.8170 | |||
F | 4 | 0.103 | ||||||
BD + AD-PAP2 |
A | 5 | 0.116 | 0.1165 | 0.0355 | 1.2350 | 0.8640 | 0.3290 |
B | 5 | 0.117 | ||||||
C | 5 | 0.103 | 0.1025 | 0.0215 | 0.7480 | |||
D | 5 | 0.102 | ||||||
E | 5 | 0.100 | 0.0985 | 0.0175 | 0.6090 | |||
F | 5 | 0.097 | ||||||
BD + AD |
A | 6 | 0.097 | 0.0980 | 0.0170 | 0.5910 | 0.6780 | 0.0760 |
B | 6 | 0.099 | ||||||
C | 6 | 0.102 | 0.1020 | 0.0210 | 0.7300 | |||
D | 6 | 0.102 | ||||||
E | 6 | 0.103 | 0.1015 | 0.0205 | 0.7130 | |||
F | 6 | 0.100 | ||||||
Blank | G | 1 | 0.079 | 0.0810 | ||||
G | 2 | 0.083 |
- a
The output of the ReadPlate 3.0, ImageJ analysis sorted by samples is shown. Calculations of β-galactosidase activity are presented, according to Basic Protocol 2.
Alternate Protocol 2: QUANTIFICATION OF INTERACTION STRENGTH USING LIQUID ONPG ASSAY
This protocol describes the steps involved in quantifying protein-protein interaction strength using ONPG as a substrate of β-gal. We have included this protocol to demonstrate the increased sensitivity of the quantitative X-gal assay (Basic Protocol 2) in detecting interactions, compared to the commonly used ONPG assay. In the presence of β-gal, ONPG is hydrolyzed to galactose and o -nitrophenol (Figure 1). The yellow color of o -nitrophenol can be measured spectrophotometrically. Note that the ONPG assay cannot be used in yeast strains with low LacZ activity, such as AH109.
Materials
-
Master plates containing co-transformed yeast, from Basic Protocol 1 or Alternate Protocol 1
-
Z-buffer (see recipe)
-
Z-buffer + β-mercaptoethanol (see recipe)
-
Z-buffer + ONPG (see recipe)
-
Liquid nitrogen
-
1 M Na2CO3 (see recipe)
-
Sterile double-distilled water
-
80% v/v ethanol (see recipe)
-
SD/–LW 92-mm-diameter agar plates (see recipe; Sarstedt, cat. no. 82.1473.001)
-
Biosafety cabinet (e.g., Kojair® BioWizard Silver Line Biosafety cabinet)
-
Sterile 1.5-ml microcentrifuge tubes (e.g., Sarstedt, cat. no. 72.690.001)
-
Heat block/water bath (at 30°C)
-
Centrifuge 5810 R (Eppendorf or equivalent)
-
50-ml polycarbonate centrifuge tubes (Sarstedt, cat. no. 62.547.254 or equivalent)
-
Multi-dispenser pipette (Eppendorf Multipipette® M4 or equivalent)
-
Vortex mixer (VWR, Vortex VV3, cat. no. 444-007 or equivalent)
-
Pipettes (2.5 μl, 20 μl and 200 μl, 5 ml)
-
Sterile pipette tips
-
Multi-channel pipette (Brand, Transferpipette® S-12, cat. no. 705932 or equivalent)
-
Shaking incubator at 30°C for tubes and plates (Infros-HT Ecotron or equivalent)
-
White 96-well plate with lid (Greiner bio-one, cat. no. 655083 or equivalent)
-
96-well flat transparent plate (Greiner Bio-One, cat. no. 675101 or equivalent suited to the plate reader)
-
Plate reader (Tecan Lifesciences, Infinite® 200 PRO or equivalent)
Plating transformed yeast cells on SD medium
1.Prepare yeast plates with droplets following Basic Protocol 2, steps 1 to 7, with the following exception: in step 1, use 1 ml of sterile water instead of 750 μl.
Harvesting and processing of yeast cells
2.Follow Basic Protocol 2, steps 8 to 16, with the following exceptions:
-
In step 8, prepare 1.5-ml tubes with 900 μl double-distilled water instead of 950 μl.
-
In step 13, calculate the final volume as 300 μl of yeast suspension with OD600= 1.0, instead of a total volume of 2 ml with OD600= 5.0.
Liquid ONPG-assay
3.Prepare all buffers freshly for the ONPG assay. Prepare enough Z-buffer + β-mercaptoethanol to have 700 μl per sample. Prepare enough Z-buffer + ONPG to have 160 μl per sample.
4.Follow step 17 of Basic Protocol 2 to make the yeast cells permeable.
5.Give the tubes a short spin in the tabletop centrifuge. Pipette 150 μl of each lysate into two new 1.5-ml tubes (technical replicates). Prepare a blank with 150 μl of Z-buffer.
6.Add 700 μl of Z-buffer + β-mercaptoethanol to each tube, including the blank, using a multi-dispenser pipette.
7.Add 160 μl Z-buffer + ONPG to each tube and the blank. Record the start time when adding Z-buffer + ONPG to the first tube.
8.Incubate tubes at 30°C in an incubator with shaking until yellow color develops. Check once every 15-30 min for the appearance of the yellow color. Use the blank and negative controls against a white background to assess the intensity of the yellow color.
9.Once color development is at an appropriate stage (the tubes with the POIs show yellow color, but not the negative controls), record the stop time and immediately stop the reaction by adding 400 μl of 1 M Na2CO3 to each tube. Determine the time elapsed (subtract the end time in minutes from the start time).
10.Centrifuge the samples for 10 min at maximum speed in a tabletop centrifuge at room temperature to pellet the cell debris.
11.Transfer 200 μl of the supernatant from each sample, including the blank, into a 96-well plate. Measure OD420 using a TECAN plate reader.
12.Calculate β-galactosidase activity (Miller units) according to the following formula:
\begin{equation*} \hspace*{-1.3pc}{\bf{\beta }} - {\bf{galactosidase;activity;}}\left( {{\bf{Miller}}} \right){\bf{;units;}} = \frac{{\bf 1,000{\bf{;;OD}}\bf 420}}{{{\bf{tVOD}}\bf 600}} \end{equation}
REAGENTS AND SOLUTIONS
Agarose/PBS solution, 1×
Add the following components to a glass bottle:
Agarose (0.5% final concentration; VWR Peqlab, cat. no 732-2789P) | 0.5 g |
1× PBS [dilute from 10× PBS (see recipe) with water] | up to 100 ml |
Microwave until the agarose is completely dissolved. Do not overboil, to avoid evaporation of the buffer, which will change the concentration. Let the solution cool down until it can be handled with bare hands (maximum 55°C) before use.
Ethanol, 80% (v/v)
Add the following components to a glass bottle:
Ethanol (Carl Roth, cat. no. K928.3) | 80 ml |
Double-distilled water | up to 100 ml |
Mix well and store at room temperature.
Glucose, 40% (w/v)
Add 400 ml of double-distilled water to a glass beaker. Place the beaker with a magnetic stirrer on a magnetic heating block, turn on the heat, and start stirring. Allow the water to get warm. Add 200 g of glucose (Sigma Aldrich, cat. no. 49159) and stir until completely dissolved. Avoid boiling the solution. Make up the final volume to 500 ml with water. Dispense in a glass bottle and filter-sterilize. Store up to 3 months at room temperature.
Glycerol, 50% (v/v)
Add the following components to a glass bottle:
Glycerol (Sigma-Aldrich, cat. no. 200-289-5) | 5 ml |
Double-distilled water | up to 10 ml |
Mix glycerol and water by stirring. Autoclave and store at room temperature for up to 1 year.
Lithium acetate (LiAc), 1 M
In a glass beaker, combine:
Lithium acetate dihydrate (Sigma Aldrich, cat. no. 6108-17-4) | 10.2 g |
Double-distilled water | up to 100 ml |
Dissolve by stirring. Dispense in a glass bottle and autoclave. Store at 4°C for up to 6 months.
PBS, pH 7.4, 10×
- In a glass beaker, combine:
NaCl (Merck Millipore, cat. no. 567440) | 8 g |
KCl (Merck Millipore, cat. no. 529552) | 0.2 g |
Na2HPO4⋅2 H2O (Merck Millipore, cat. no. 1370361000) | 1.4 g |
KH2PO4 (Carl Roth, cat. no. 3904.1) | 0.24 g |
Double-distilled water | up to 80 ml |
Dissolve by stirring. Adjust the pH to 7.4 using KOH. Make up the total volume to 100 ml with distilled water and dispense into a glass bottle. Store at room temperature for up to 1 year.
Polyethylene glycol (PEG), 40% (w/v)
To a glass bottle, add 10 g of PEG (MW 3350; Sigma, cat. no. 202444). Then, slowly add water to 20 ml with constant stirring (using a magnetic stirrer). Autoclave. The remaining PEG will dissolve after autoclaving.
SD (synthetically defined)/–LW medium
In a glass beaker combine:
Yeast nitrogen base without amino acids (Sigma-Aldrich, cat. no. Y0626) | 6.7 g |
Adenine hemisulfate (Sigma-Aldrich; cat. no. A9126) | 40 mg |
Yeast synthetic drop-out supplement (–Leu, –Trp; Clontech, cat. no. 630417) | 0.7 g |
Double-distilled water | Up to 900 ml |
Dissolve by stirring. Adjust the pH to 5.8 using KOH. Add 18 g of Agar-Agar Kobe I (Carl Roth, cat. no. 5210.2).
Make up the total volume to 950 ml with distilled water and dispense into a 1-liter glass bottle with a magnetic stirrer. Autoclave and add 50 ml of sterile 40% (w/v) glucose (see recipe). Stir well to mix and pour the warm medium into sterile plates (approximately 25 ml per 92-mm-diameter round plate and 50 ml per square Petri plate). Let the medium solidify and then store plates at 4°C for up to 2 weeks.
Sodium carbonate (Na2CO3), 1 M
In a glass bottle, combine:
Na2CO3 (Carl Roth, cat. no. P028) | 10.6 g |
Double-distilled water | up to 100 ml |
Dissolve by stirring and store at room temperature.
TE buffer, 10×, pH 7.5
To a glass beaker add:
1 M Tris⋅HCl, pH 7.5 (see recipe; 100 mM final concentration) | 10 ml |
Disodium EDTA (10 mM final concentration; Carl Roth, cat. no. 8043.2) | 0.37 g |
Double-distilled water | to 80 ml |
Mix and dissolve by stirring. Adjust the pH to 7.5 using HCl. Make up the total volume to 100 ml with distilled water and dispense into a glass bottle. Store up to 1 year at room temperature.
Tris⋅HCl, 1 M, pH 7.5
To a glass beaker add:
Tris base (VWR, cat. no. 103156X) | 9.1 g |
Double-distilled water | up to 90 ml |
Dissolve by stirring. Adjust the pH to 7.5 using HCl. Make up the total volume to 100 ml with distilled water and dispense into a glass bottle. Autoclave and store up to 1 year at room temperature.
YAPD (yeast extract-adenine-peptone-dextrose) medium
To a glass beaker, add:
Bacto-Peptone (Thermo Fisher Scientific, cat. no. 211677) | 20 g |
Bacto yeast extract (Thermo Fisher Scientific, cat. no. 212750) | 10 g |
Adenine hemisulfate (Sigma-Aldrich; cat. no. A9126) | 100 mg |
Double-distilled water | up to 900 ml |
Dissolve by stirring. Adjust the pH to 5.8 by adding KOH. Add 20 g of glucose (Sigma Aldrich, cat. no. 49159).
For solid medium in plates, add 18 g of BD DifcoTM Agar (BD Biosciences, cat. no. 214530). Make up the total volume to 1 liter with water and dispense into a glass bottle. Autoclave and then pour 25 ml of the warm medium into a sterile 92-mm-diameter plate (Sarstedt, cat. no. 82.1473.001). Let the medium solidify and then store the plates at 4°C for up to 2 weeks.
Z-buffer, 1×
To a glass beaker, add:
Na2HPO4⋅2H2O (Merck, cat. no. 71643) | 10.7 g |
NaH2PO4⋅H2O (Merck, cat. no. 106345) | 5.5 g |
KCl (Merck Millipore, cat. no. 529552) | 0.75 g |
MgSO4⋅7H2O (Merck Millipore, cat. no. 105886) | 0.246 g |
Double-distilled water | up to 950 ml |
Dissolve by stirring. Adjust the pH to 7.0 using NaOH. Make up the total volume to 1 liter with water and dispense into a glass bottle. Autoclave and store up to 1 year at room temperature.
Z-buffer + β-mercaptoethanol
For the liquid ONPG assay, 700 μl per sample of Z-buffer + β-mercaptoethanol is required. Prepare fresh before use. Mix the following components in a 50-ml tube:
β-mercaptoethanol (Sigma-Aldrich, cat. no. M6250) | 54 μl |
Z-buffer (see recipe) | Up to 20 ml |
Z-buffer + ONPG
160 μl per sample is required for liquid ONPG assay. Prepare fresh before use. Mix the following components in a 50-ml tube.
o-Nitrophenyl β-D-galactopyranoside (ONPG; Sigma Aldrich, cat. no. N1127-5G) | 40 mg |
Z-buffer (see recipe) | Up to 10 ml |
Mix well by vortexing or placing the tube in a shaker until the ONPG dissolves. Always store the ONPG at −20°C.
COMMENTARY
Background Information
The yeast Saccharomyces cerevisiae is one of the most extensively studied eukaryotic model organisms. Irrespective of the field or organism of study, yeast is also a powerful molecular biology tool to probe protein-protein or protein-nucleic acid interactions. The Y2H assay was developed more than 30 years ago (Fields & Song, 1989) to detect the physical interaction between POIs. Among the techniques available today to test protein-protein interactions, including co-immunoprecipitation, mass spectrometry, and others, the relatively simple and less time-consuming Y2H approaches are still favored by researchers worldwide. Decades of research have resulted in numerous yeast strains, compatible expression plasmids, and libraries that enable the study of interactions involving almost any type of proteins. Most of the available yeast-based assays use β-galactosidase as a reporter for the detection and quantification of protein-protein interactions. This enzyme, encoded by the bacterial gene LacZ , can react with several different substrates producing colored compounds, many of which are amenable to spectrophotometric detection and quantification.
Among the colorimetric methods, ONPG is a widely used substrate to measure interaction strength, and in ONPG-based assays, researchers quantify the yellow-colored o -nitrophenol produced in the presence of β-galactosidase. However, ONPG assays are less sensitive in capturing weak interactions compared to assays that use another inexpensive and commonly used substrate, X-gal. In fact, X-gal-based assays are the most sensitive assays for β-galactosidase currently in use, resulting in an intensely blue-colored end product. This product, however, is insoluble and cannot easily be quantified using spectrophotometric methods. Therefore, the use of X-gal is mostly restricted to qualitative approaches such as the colony lift assay, plate assay, and X-gal overlay assay (Möckli & Auerbach, 2004). Efforts have been made in the past to standardize a quantitative assay based on X-gal (Möckli & Auerbach, 2004; Serebriiskii et al., 2000). These assays, however, were not widely adopted, perhaps due to the extended time involved, the requirement of specialized skills, and outdated quantification methods. To bridge this gap, we have standardized a quantitative assay based on X-gal (quantitative X-gal assay, described here). We have performed several experiments to optimize the assay for speed and consistency in sample preparation, incubation, and method of quantification. Our assay can be performed reliably using standard molecular biology lab equipment and a smartphone camera. Moreover, we have integrated the use of a freely available, ImageJ-based ReadPlate plugin for quick and accurate measurements. The quantitative X-gal assay is sensitive enough to capture weak interactions that assays based on other substrates, like ONPG, may fail to detect. Furthermore, our assay is highly scalable and can be adapted to domain mapping or high-throughput screening experiments, even when using yeast strains with low inducible LacZ expression.
Among the currently used X-gal-based assays, the quantitative X-gal assay described here provides an easy and rapid way of detecting and comparing protein interaction strengths. While powerful, LacZ -based assays in general also suffer from various disadvantages, which also apply to the quantitative X-gal assay described here. For example, many proteins when fused with BD show autoactivation and, hence, produce a blue color in the X-gal assay, leading to false-positive results. Using protein fragments or specific domains instead of full-length proteins, swapping proteins of interest between BD and AD, and performing the experiments with the essential positive and negative controls are, however, expected to circumvent most of the problems associated with LacZ reporter assays. As such, we hope that the quantitative X-gal assay described in this protocol may contribute to identifying novel protein interaction networks and characterizing new molecular mechanisms.
Critical Parameters
Preparation of competent yeast cells and transformation
When preparing competent cells, the health and growth phase of the inoculum is crucial. Hence, colonies from a fresh plate (not older than a week) are recommended. Using older colonies tends to give unreliable results in our hands, probably owing to the differences in the health and growing capacity of individual colonies. Fresh plates streaked with yeast cells from a glycerol stock and grown at 30°C for 3 days give the best results, in our experience.
While preparing the competent cells, growth phase is an important parameter to consider. When harvesting yeast cells, using an OD600 of 0.8 to 1.0 ensures high transformation efficiency. Instead of this OD-based approach, yeast cells in a very small volume (10 μl) can be counted using a hemacytometer (Gietz & Schiestl, 2007b) to calculate the cell density in the culture. However, this is more time-consuming and unnecessary in routine yeast transformations where ultra-high transformation efficiency is not required. In our experience, the competent yeast cells derived from cultures with OD600 of more than 1.0 have decreased efficiency and are not recommended for high-throughput assays such as Y2H screening.
During transformation, it is important to include negative and positive controls to rule out contamination in competent cells and false positives.
As yeast plates are rich in sugars, they support the growth of bacterial and fungal contaminants. To prevent contamination, prepare the plates and perform all the subsequent steps under a sterile environment in a biosafety cabinet.
Quantitative X-gal assay
When testing novel interactions, we recommend doing a colony lift assay (Bass et al., 2016) on half of the freshly transformed yeast cells. This will provide an initial indication of whether the assay and the system are suitable for further studies. Once blue color development occurs in the colony lift assay, proceed with the quantitative X-gal assay using the remaining transformed colonies. Since the colony lift assay can be performed within 2-3 days, the remaining colonies would still be fresh enough to perform the quantitative X-gal assay.
While performing the quantitative X-gal assay, using yeast cells not older than 2 weeks after transformation gives the most reliable results. In our hands, older colonies have given inconsistent results, perhaps due to differences in the health and viability of individual colonies, reflecting the overall growth at the time of harvesting. After harvesting the cells, it is critical to perform at least 3-4 freeze-thaw cycles using liquid nitrogen and a 30°C heat block or water bath, to ensure the permeability of yeast cells. While mixing the X-gal solution with permeable yeast cells, proper mixing ensures equal distribution of X-gal and, hence, uniform blue color throughout the well. When handling many samples, pipette 8 samples at a time using a multichannel pipette, and mix up and down by pipetting. To avoid the solidification of the X-gal-agarose mix, a magnetic stirrer with a heating function can be used in a fume hood. Recording the time of the start of the assay and the end of the assay after color development is an important step that is needed for calculating β-gal activity. During incubation, check regularly while the color develops, especially when testing new interactions. The appropriate time to photograph the plate is when the POIs show blue color and the negative controls remain colorless. Incubating for a longer time will enhance the blue color, but will also do so in the negative controls. If the blue color develops too quickly, see the Troubleshooting section for suggestions.
While taking pictures of 96-well microtiter plates, make sure to avoid using the flash, as it interferes with the ReadPlate measurements. The output from the ReadPlate 3.0 analysis contains the “Auncorr” (A uncorrected) value which is specific to the white plates described in this assay. In case a transparent plate is preferred, use the “Acorr” (A corrected) value, which is corrected for differences in local illumination. While testing specific interactions between two POIs, it is necessary to perform regression analysis using values obtained from different time points (Figure 5C) to ensure that the assay is performed within the linear range.
Troubleshooting
Table 3 lists some common problems with the protocols, their causes, and potential solutions.
Problem | Possible causes | Suggestions |
---|---|---|
Liquid yeast cultures do not grow or grow very slowly | Incorrect yeast medium | Check whether the liquid medium was made correctly. If necessary, make fresh medium. |
Yeast cells from stock cells are too old | Do not use yeast cells older than 1 week, as the viability of cells may decrease upon extended storage at 4°C. | |
Transformation efficiency is very low or no colonies are visible | Suboptimal yeast competent cells |
Make sure to follow all critical parameters for the preparation of yeast competent cells. Use freshly made competent cells. |
One of the plasmids is toxic to the cells or has a poor plasmid quality |
Plate the co-transformation mix on SD/–L or SD/–W plates (depending on the vectors used) and select for either prey or bait plasmid to check whether one of the plasmids is causing the problem. Extract plasmids freshly and check the quality via spectrophotometric measurements using NanoDrop. Plasmids extracted by the midi-prep method work better than plasmids extracted via the mini-prep method, at least in our hands. |
|
No color development | Cells lysis incomplete | Repeat the assay and add more freeze-thaw cycles. |
Cell lysate contains reduced amounts of β-galactosidase |
Incubate the samples for a longer time. Repeat the assay using cells at a higher OD600. Alternatively, use more X-gal (increase amount of X-gal up to 0.1 g/ml). |
|
Color is too intense | Cell lysate contains high amounts of β-galactosidase |
Decrease the assay incubation time. Decrease the β-galactosidase concentration by using a lower number of cells. |
Samples turn blue immediately after adding the X-gal master mix | Cell lysate contains high β-galactosidase concentrations | Decrease β-galactosidase by using a lower OD600 (i.e., lower number of cells). |
X-gal master mix turns solid before pipetting is finished | Pipetting too slow or too many samples at a time |
Try using a multi-dispenser pipette to decrease pipetting time. Prepare the master mix in a bottle and add a magnetic stirrer. Keep the bottle in a heating magnetic block and keep stirring while pipetting. |
Master mix not vortexed throughout the whole procedure | Prepare the master mix in a bottle and add a magnetic stirrer. Keep the bottle in a heating magnetic block and keep stirring while pipetting | |
Blue color development in the negative controls | Bait plasmid autoactivates the LacZ reporter gene expression |
Try swapping the POIs. For instance, clone one of the POIs as an AD-fusion, instead of BD-fusion, and the same with the other POI. Consider using specific protein segments (e.g., a specific domain), instead of the entire protein. |
Degradation of X-gal due to too long incubation time |
Reduce the incubation time. Use a higher number of cells (higher OD600) in the assay, to check if the blue color develops faster. |
|
Blue color develops too quickly | High OD600 of cells used for the assay | Try to use a lower cell density reflected by a lower OD600. For example, if the blue color develops too quickly when the OD600 is 5.0, reduce the OD600 to 2.0 and repeat the assay. |
Understanding Results
Carefully following the procedures described in these protocols guarantees the typically expected results in each step. In Basic Protocol 1, step 19, the growth of several yeast colonies (105 to 106 colonies per 1 μg of circular plasmid used) is expected on the selection plates. A higher number of colonies indicates higher transformation efficiency and ensures that the selected colonies contain both the bait and prey plasmids. In step 7 of Basic Protocol 2, the yeast plates ready for harvesting should contain a lawn of cells within each spot. This is an indication that the master plates contain healthy colonies that can grow again on the selection plates. In Basic Protocol 2, step 24, intense blue color in the sample containing bait and prey suggests that the two POIs physically interact with each other, and that the BD and AD have been brought into enough proximity to restore the activity of the GAL4 transcription factor. The activity of GAL4 on the UAS upstream of LacZ subsequently results in the expression of β-galactosidase, which hydrolyzes X-gal to produce the blue-colored end product.
Similarly, in Basic Protocol 2, in step 33, the “Auncorr” value is the output of the ReadPlate 3.0 analysis. Higher values than the control samples (such as BD- or AD-only) indicate a possible interaction between the POIs. The values obtained after the calculation of β-galactosidase activity can be directly correlated with interaction strengths. For example, SPA1-WD interacts more strongly with PAP2 than the full-length SPA1 (Figure 5B). WD-containing proteins COP1 and SPA1 possess a valine proline (VP) motif-binding pocket that facilitates the interaction with the target proteins, such as PAP2 (Ponnu, 2020; Ponnu, Riedel, Penner, Schrader, & Hoecker, 2019). Given that the WD domain interacted more strongly with PAP2 (Figure 5B) than the full-length protein, this suggests that the N-terminal domain of SPA1 may mask or interfere with the PAP2 interaction to some extent, as observed for the cryptochrome 2-SPA1 interaction (Ponnu et al., 2019).
Following Basic Protocol 2 for analyzing the linearity of the quantitative X-gal assay using pictures taken at different time points should result in a graph similar to the one shown in Figure 5C. In this case, the regression fit and the R2 value depicted show that the quantitative X-gal assay lies within the linear range, which is essential for a reliable quantitative interaction assay. Figure 5C shows that the quantitative X-gal assay progresses linearly, like the ONPG assay. Alternate Protocol 2 (the ONPG assay), when properly conducted, should result in a similar interaction pattern than that of the quantitative X-gal assay. However, a comparatively lower statistical significance was observed between the interactions of full-length SPA1 and SPA1-WD than in quantitative X-gal assay, likely due to the lower assay sensitivity in the case of ONPG (Figure 5D).
Time Considerations
By using the same vector systems described in the protocols, preparation of the bait and prey constructs could be finished within 10 days. Preparation of frozen yeast competent cells usually takes 2 days; thereafter, the transformation procedure can be completed within less than a day using the frozen cells. Following Alternate Protocol 1, every yeast transformation takes 3 days to complete, and the competent cells produced via this method cannot be stored for more than 4 hr after preparation. A protocol for storing the competent cells prepared via the LiAc method is available (Gietz & Schiestl, 2007a); however, it did not work in our hands. Growing yeast droplets and sample collection can be finished in 2 days. On the third day, the X-gal assay can be started and prolonged up to a fourth or fifth day depending on the strength of interaction and the nature of the assay. Calculation of β-galactosidase activity takes less than an hour to finish, depending on the number of samples.
Following Basic Protocols 1 and 2, the whole assay can be performed within 6 days, starting from yeast transformation. Our protocol does not necessitate finishing the whole experiment in a single run, but instead offers flexible ways of working. For example, after the preparation of frozen yeast competent cells, transformation can be performed any time during the next 6 months. Once transformed colonies are obtained, further steps can be done any time within the next 15 days. After harvesting the cells and freezing them in Z-buffer at −80°C, the quantitative X-gal assay can be performed any time within the next 2 weeks.
Acknowledgments
The authors would like to thank Dr. José María Delfino (University of Buenos Aires, Argentina) for support in troubleshooting the ReadPlate plugin version 1.2 during optimization of this protocol; AG Hülskamp (University of Cologne) for pAS2-1-GW and pACT2-GW empty vectors; and Maike Hansen, Yuri Ando, and Zhen Cao (AG Hoecker, University of Cologne) for testing the efficiency of the quantitative X-gal assay using various proteins from Arabidopsis thaliana and Physcomitrium patens.
Author Contributions
Laura Trimborn : data curation, investigation, methodology, software, visualization, writing original draft; Ute Hoecker : funding acquisition, supervision, writing review and editing; Jathish Ponnu : conceptualization, data curation, formal analysis, investigation, methodology, supervision, validation, writing original draft, writing review and editing.
Conflict of Interest
The authors declare no conflict of interest.
Funding Information
This research was supported by the Deutsche Forschungsgemeinschaft (DFG) HO2793/3-4 and under Germany's Excellence Strategy EXC2048/1 project ID 390686111
Open access funding enabled and organized by Projekt DEAL.
Open Research
Data Availability Statement
The data, tools, and material (or their sources) that support the protocol are available from the corresponding author upon reasonable request.
Literature Cited
- Bass, J. I. F., Reece-Hoyes, J. S., & Walhout, A. J. M. (2016). Colony lift colorimetric assay for β-galactosidase activity. Cold Spring Harbor Protocols , 2016, pdb.prot088963. doi: 10.1101/pdb.prot088963.
- Bernard, P. (1996). Positive selection of recombinant DNA by CcdB. BioTechniques , 21, 320–323. doi: 10.2144/96212pf01.
- Borevitz, J. O., Xia, Y., Blount, J., Dixon, R. A., & Lamb, C. (2000). Activation tagging identifies a conserved MYB regulator of phenylpropanoid biosynthesis. The Plant Cell , 12, 2383–2393. doi: 10.1105/tpc.12.12.2383.
- Brent, R., & Ptashne, M. (1985). A eukaryotic transcriptional activator bearing the DNA specificity of a prokaryotic repressor. Cell , 43, 729–736. doi: 10.1016/0092-8674(85)90246-6.
- Cabrera, E., Welch, L. C., Robinson, M. R., Sturgeon, C. M., Crow, M. M., & Segarra, V. A. (2020). Cryopreservation and the freeze–thaw stress response in yeast. Genes , 11, 835. doi: 10.3390/genes11080835.
- Cottier, S., Mönig, T., Wang, Z., Svoboda, J., Boland, W., Kaiser, M., & Kombrink, E. (2011). The yeast three-hybrid system as an experimental platform to identify proteins interacting with small signaling molecules in plant cells: Potential and limitations. Frontiers in Plant Science , 2, 101.
- Criekinge, W. V., & Beyaert, R. (1999). Yeast two-hybrid: State of the art. Biological Procedures Online , 2, 1–38. doi: 10.1251/bpo16.
- Duttweiler, H. M. (1996). A highly sensitive and non-lethal β-galactosidase plate assay for yeast. Trends in Genetics , 12, 340–341. doi: 10.1016/S0168-9525(96)80008-4.
- Engler, C., Kandzia, R., & Marillonnet, S. (2008). A one pot, one step, precision cloning method with high throughput capability. PLoS ONE , 3 ( 11 ) , e3647. doi: 10.1371/journal.pone.0003647.
- Estojak, J., Brent, R., & Golemis, E. A. (1995). Correlation of two-hybrid affinity data with in vitro measurements. Molecular and Cellular Biology , 15, 5820–5829. doi: 10.1128/MCB.15.10.5820.
- Fetchko, M., & Stagljar, I. (2004). Application of the split-ubiquitin membrane yeast two-hybrid system to investigate membrane protein interactions. Methods , 32, 349–362. doi: 10.1016/j.ymeth.2003.10.010.
- Fields, S., & Song, O. (1989). A novel genetic system to detect protein–protein interactions. Nature , 340, 245–246. doi: 10.1038/340245a0.
- Gantner, J., Ordon, J., Ilse, T., Kretschmer, C., Gruetzner, R., Löfke, C., … Stuttmann, J. (2018). Peripheral infrastructure vectors and an extended set of plant parts for the Modular Cloning system. PLoS ONE , 13, e0197185. doi: 10.1371/journal.pone.0197185.
- Gibson, D. G., Young, L., Chuang, R.-Y., Venter, J. C., Hutchison, C. A., & Smith, H. O. (2009). Enzymatic assembly of DNA molecules up to several hundred kilobases. Nature Methods , 6, 343–345. doi: 10.1038/nmeth.1318.
- Gietz, R. D., & Schiestl, R. H. (2007a). Frozen competent yeast cells that can be transformed with high efficiency using the LiAc/SS carrier DNA/PEG method. Nature Protocols , 2, 1–4. doi: 10.1038/nprot.2007.17.
- Gietz, R. D., & Schiestl, R. H. (2007b). High-efficiency yeast transformation using the LiAc/SS carrier DNA/PEG method. Nature Protocols , 2, 31–34. doi: 10.1038/nprot.2007.13.
- Golemis, E. A., & Khazak, V. (1997). Alternative yeast two-hybrid systems. The interaction trap and interaction mating. Methods in Molecular Biology (Clifton, N.J.) , 63, 197–218.
- Harper, J. W., Adami, G. R., Wei, N., Keyomarsi, K., & Elledge, S. J. (1993). The p21 Cdk-interacting protein Cip1 is a potent inhibitor of G1 cyclin-dependent kinases. Cell , 75, 805–816. doi: 10.1016/0092-8674(93)90499-G.
- Hartley, J. L., Temple, G. F., & Brasch, M. A. (2000). DNA cloning using in vitro site-specific recombination. Genome Research , 10, 1788–1795. doi: 10.1101/gr.143000.
- Keegan, L., Gill, G., & Ptashne, M. (1986). Separation of DNA binding from the transcription-activating function of a eukaryotic regulatory protein. Science , 231, 699–704. doi: 10.1126/science.3080805.
- Koegl, M., & Uetz, P. (2007). Improving yeast two-hybrid screening systems. Briefings in Functional Genomics , 6, 302–312. doi: 10.1093/bfgp/elm035.
- Levitsky, K. L., Toledo-Aral, J. J., López-Barneo, J., & Villadiego, J. (2013). Direct confocal acquisition of fluorescence from X-gal staining on thick tissue sections. Scientific Reports , 3, 2937. doi: 10.1038/srep02937.
- Maier, A., Schrader, A., Kokkelink, L., Falke, C., Welter, B., Iniesto, E., … Hoecker, U. (2013). Light and the E3 ubiquitin ligase COP1/SPA control the protein stability of the MYB transcription factors PAP1 and PAP2 involved in anthocyanin accumulation in Arabidopsis. The Plant Journal , 74, 638–651. doi: 10.1111/tpj.12153.
- Maruta, N., Trusov, Y., & Botella, J. R. (2016). Yeast three-hybrid system for the detection of protein-protein interactions. In J. R. Botella & M. A. Botella (Eds.), Plant signal transduction: Methods and protocols methods in molecular biology (pp. 145–154). New York, NY: Springer. doi: 10.1007/978-1-4939-3115-6_12 [Accessed February 17, 2022].
- Miller, J. (1972). Experiments in molecular genetics. Cold Spring Harbor, NY: Cold Spring Harbor Laboratory.
- Möckli, N., & Auerbach, D. (2004). Quantitative β-galactosidase assay suitable for high-throughput applications in the yeast two-hybrid system. BioTechniques , 36, 872–876. doi: 10.2144/04365PT03.
- Ouwerkerk, P. B. F., & Meijer, A. H. (2001). Yeast one-hybrid screening for DNA-protein interactions. Current Protocols in Molecular Biology , 55, 12.12.1–12.12.12. doi: 10.1002/0471142727.mb1212s55.
- Paiano, A., Margiotta, A., De Luca, M., & Bucci, C. (2019). Yeast two-hybrid assay to identify interacting proteins. Current Protocols in Protein Science , 95, e70. doi: 10.1002/cpps.70.
- Ponnu, J. (2020). Molecular mechanisms suppressing COP1/SPA E3 ubiquitin ligase activity in blue light. Physiologia Plantarum , 169, 418–429. doi: 10.1111/ppl.13103.
- Ponnu, J., & Hoecker, U. (2021). Illuminating the COP1/SPA ubiquitin ligase: fresh insights into its structure and functions during plant photomorphogenesis. Frontiers in Plant Science , 12, 486.
- Ponnu, J., Riedel, T., Penner, E., Schrader, A., & Hoecker, U. (2019). Cryptochrome 2 competes with COP1 substrates to repress COP1 ubiquitin ligase activity during Arabidopsis photomorphogenesis. Proceedings of the National Academy of Sciences , 116, 27133–27141. doi: 10.1073/pnas.1909181116.
- Rose, M., & Botstein, D. (1983). Construction and use of gene fusions to lacZ (beta-galactosidase) that are expressed in yeast. Methods in Enzymology , 101, 167–180. doi: 10.1016/0076-6879(83)01012-5.
- Schaefer, J., Jovanovic, G., Kotta-Loizou, I., & Buck, M. (2016). Single-step method for β-galactosidase assays in Escherichia coli using a 96-well microplate reader. Analytical Biochemistry , 503, 56–57. doi: 10.1016/j.ab.2016.03.017.
- Serebriiskii, I. G., Toby, G. G., & Golemis, E. A. (2000). Streamlined yeast colorimetric reporter activity assays using scanners and plate readers. BioTechniques , 29, 278–288. doi: 10.2144/00292st03.
- Striebinger, H., Koegl, M., & Bailer, S. M. (2013). A high-throughput yeast two-hybrid protocol to determine virus-host protein interactions. Virus-Host Interactions , 1064, 1–15. doi: 10.1007/978-1-62703-601-6_1.
- Treco, D. A., & Lundblad, V. (1993). Preparation of yeast media. Current Protocols in Molecular Biology , 23, 13.1.1-13.1.7.
- Yocum, R. R., Hanley, S., West, R., & Ptashne, M. (1984). Use of lacZ fusions to delimit regulatory elements of the inducible divergent GALJ-GALIO promoter in Saccharomyces cerevisiae. Molecular and Cellular Biology , 4(10), 1985–1998.
- Zhang, X., & Bremer, H. (1995). Control of the Escherichia coli rrnB P1 Promoter Strength by ppGpp. Journal of Biological Chemistry , 270, 11181–11189. doi: 10.1074/jbc.270.19.11181.
Internet Resources
Fiji, ImageJ-based free software.
ReadPlate 3.0, free ImageJ plugin.
ImageJ user guide.
Frozen-EZ Kit protocol.
Thermo Fisher manual for GW vectors.
Yeast Protocol Handbook from Clontech.
Citing Literature
Number of times cited according to CrossRef: 1
- Da Wang, Kaixian Chen, Zheng Wang, Huali Wu, Yiming Li, Research progress on interferon and cellular senescence, The FASEB Journal, 10.1096/fj.202400808RR, 38 , 16, (2024).