A Primer for Research Scientists on Assessing Mouse Gross and Histopathology Images in the Biomedical Literature
Andrew J. Gifford, Andrew J. Gifford
Abstract
Advances in genomic technologies have enabled the development of abundant mouse models of human disease, requiring accurate phenotyping to elucidate the consequences of genetic manipulation. Anatomic pathology, an important component of the mouse phenotyping pipeline, is ideally performed by human or veterinary pathologists; however, due to insufficient numbers of pathologists qualified to assess these mouse models morphologically, research scientists may perform “do-it-yourself” pathology, resulting in diagnostic error. In the biomedical literature, pathology data is commonly presented as images of tissue sections, stained with either hematoxylin and eosin or antibodies via immunohistochemistry, accompanied by a figure legend. Data presented in such images and figure legends may contain inaccuracies. Furthermore, there is limited guidance for non-pathologist research scientists concerning the elements required in an ideal pathology image and figure legend in a research publication. In this overview, the components of an ideal pathology image and figure legend are outlined and comprise image quality, image composition, and image interpretation. Background knowledge is important for producing accurate pathology images and critically assessing these images in the literature. This foundational knowledge includes understanding relevant human and mouse anatomy and histology and, for cancer researchers, an understanding of human and mouse tumor classification and morphology, mouse stain background lesions, and tissue processing artifacts. Accurate interpretation of immunohistochemistry is also vitally important and is detailed with emphasis on the requirement for tissue controls and the distribution, intensity, and intracellular location of staining. Common pitfalls in immunohistochemistry interpretation are outlined, and a checklist of questions is provided by which any pathology image may be critically examined. Collaboration with pathologist colleagues is encouraged. This overview aims to equip researchers to critically assess the quality and accuracy of pathology images in the literature to improve the reliability and reproducibility of published pathology data. © 2023 The Authors. Current Protocols published by Wiley Periodicals LLC.
INTRODUCTION
Advances in genomic technologies have heralded an abundance of mouse models of human disease, particularly cancer mouse models. These mouse models require detailed phenotyping to characterize the effects of genetic manipulation (Kersten et al., 2017). Accurate pathological characterization of intended and unexpected lesions arising in these models is a critical component of the mouse phenotyping pipeline (Brayton et al., 2001; Cardiff, Miller & Munn, 2014; Schofield et al., 2012). Mouse models are assessed at a microscopic level by examining tissue sections, stained with hematoxylin and eosin (H&E) and immunohistochemically, with a standard light microscope. Pathology data is commonly presented in the biomedical literature as images (photos) of H&E and immunohistochemically stained tissue sections with an explanatory figure legend. For both cancer and non-neoplastic diseases, knowledge of the underlying pathologic processes and the attributes of the mouse models is required to capture an appropriate image for publication. However, the data presented in such images and figure legends may harbor inaccuracies (Ward et al., 2017).
Research scientists encounter pathology images at three stages of the publication process: (1) preparing figures for their own manuscript or grant application, (2) as a reviewer of a manuscript for publication, and (3) in a journal post-publication. A pathologist with relevant mouse histopathology experience should be consulted to ensure accurate figure preparation and to identify errors and inadequacies at manuscript review; however, pathologist input may not always be available. The quality of pathology images and the content and interpretation of these images are critical for accurate reporting of research data. While techniques on how to obtain optimal images from a light microscope have been described in detail (Riley et al., 2020), limited guidance has been provided for non-pathologist research scientists as to what elements are required in an ideal pathology image and figure legend in a research publication.
This overview provides researchers with a framework to understand the elements required in a pathology image and figure legend. The author is employed in an institute investigating childhood cancer; therefore, illustrative examples are drawn from pediatric tumor pathology. Common problems associated with pathology data and images are outlined. The elements of an ideal pathology image and figure legend are detailed. These elements comprise image quality, content, and interpretation, which also serve as the basis for critically assessing an image and figure legend. Understanding relevant human and mouse anatomy and histology is introduced as foundational knowledge. This is followed by guidance for understanding the human disease being studied, which for cancer researchers means grasping human tumor classification to facilitate interpretation of the cognate mouse model. Pathologic processes, such as hemorrhage and necrosis, and tissue artifacts that may affect any tumor are discussed. Importantly, an approach to interpreting immunohistochemistry (IHC) is detailed with emphasis on the requirement for tissue controls and the distribution, intensity, and intracellular location of staining. Finally, practical examples are provided to demonstrate suggestions that may be made to authors to improve the images and figure legends in a research manuscript. A brief explanation of technical terms is provided in the glossary. This overview aims to improve the reproducibility of pathology data in the research literature to the benefit of human health.
THE PROBLEM WITH PATHOLOGY DATA
Insufficient Anatomic Pathologists to Characterize and Review Mouse Model Pathology Data
Anatomic Pathology is the mainstay of clinical diagnostic pathology and is essential for the accurate diagnosis of disease, even in the molecular era. Diseases are assessed at both a macroscopic (gross) and microscopic (histopathologic) level. In routine diagnostic and research practice, microscopic assessment is based on a review of H&E- and IHC-stained tissue sections. In a research setting, tissue is commonly derived from inoculation mouse models, such as patient-derived xenografts (PDXs) in immunodeficient mice, and immunocompetent genetically engineered mouse models (GEMMs). Assessment of H&E- and IHC-stained slides is best performed by a qualified human or veterinary anatomic pathologist (Hoenerhoff et al., 2021). The importance of histopathology for GEMM assessment is attested to by the recent publication of the textbook Pathology of Genetically Engineered and Other Mutant Mice (Sundberg et al., 2022). However, consequent to the well-documented shortage of pathologists (Valli et al., 2007), mouse model histopathology is not uncommonly performed by research scientists. Morphologic interpretation by non-pathologist scientists, coined “do-it-yourself pathology”, may result in erroneous interpretation from which inaccurate conclusions are generated and in the publication of poor-quality images precluding critical appraisal of the data presented (Cardiff et al., 2008; Cuoto & Cardiff, 2008; Ince et al., 2008; Ward et al., 2017). Ince et al. (2008) recommend that journal editors request manuscripts containing pathology phenotyping data be reviewed by pathologists with relevant expertise.
Deficiencies in the Presentation of Imaging Data in the Biomedical Literature
Recent data suggest that biomedical imaging data inaccuracies are widespread and contribute to a lack of experimental reproducibility. In a recent study, 185 of 240 (77%) publications contained figures with microscopic imaging data, of which the majority were immunofluorescence images, and 52% of the figures contained microscopic images. Only 17% of 180 papers with imaging data adequately reported crucial aspects of image acquisition. Furthermore, many articles contained no information on image processing, analysis, or quantification (Marqués et al., 2020). Similarly, of 580 papers analyzed from the plant science, cell biology, and physiology literature, the majority (55% to 72%) contained images, 61% to 88% of which were microscopic images. Only 20% to 57% of the papers contained adequate figure legends, and 5% to 17% of figure legends contained no description of the species or tissue in the image (Jambor et al., 2021).
The frequency of deficiencies in mouse histopathology image data has not been systematically studied to the author's knowledge. However, the likelihood that problems with the interpretation and representation of pathology data are common is supported by a recent survey in the author's institution (unpublished observation). Of 73 respondents, 44 (60%) had no confidence, and a further 22 (30%) had little confidence in being able to identify an inaccurately labeled histopathology figure in a research publication.
Morphologic data from H&E- and IHC-stained slides may be documented by varied means, including a written description of findings, semiquantitative scoring of specific morphologic features, and as images captured with a camera attached to the light microscope. In the biomedical literature, microscopic findings are often captured by representative images illustrating salient morphologic features with an accompanying figure legend. Poor-quality gross and histopathology images, interpretation errors, and figure legends with insufficient or inaccurate details have been published in the peer-reviewed literature (Jambor et al., 2021; Ward et al., 2017). Ward et al. (2017) documented examples where figure legends either do not describe anything in the figure or do not accurately reflect what is in the figure. Other interpretation errors include misidentification of internal organs and structures, misdiagnosis of normal structures as lesional, and misclassification of neoplasia (Cardiff et al., 2008; Ward et al., 2017). It should not be assumed that the pathology data is correct simply because an image is in a published paper. As emphasized by Cardiff et al. (2014a), “Beware of what you see in the hard copy publication!”
FOUNDATIONS OF AN IDEAL PATHOLOGY IMAGE AND FIGURE LEGEND
The foundations of an ideal pathology image and figure legend comprise image quality , image composition , and image interpretation (Fig. 1). These elements produce a figure that clearly demonstrates key morphologic features that are explained by a concise, accurate, and sufficiently detailed description to support the scientific argument proffered in the journal paper. An image must also be an honest representation of the data and not dishonestly manipulated. These foundations apply to both gross pathology images and microscopic images obtained via either whole slide imaging or photography of a region of interest of both non-neoplastic processes and cancer. Image quality is the capacity of an image to communicate visual data to an audience. Image content is what is selected to be included in the image. Image interpretation is how the data presented in the image is reported and forms the basis of conclusions made from the tissue sample.
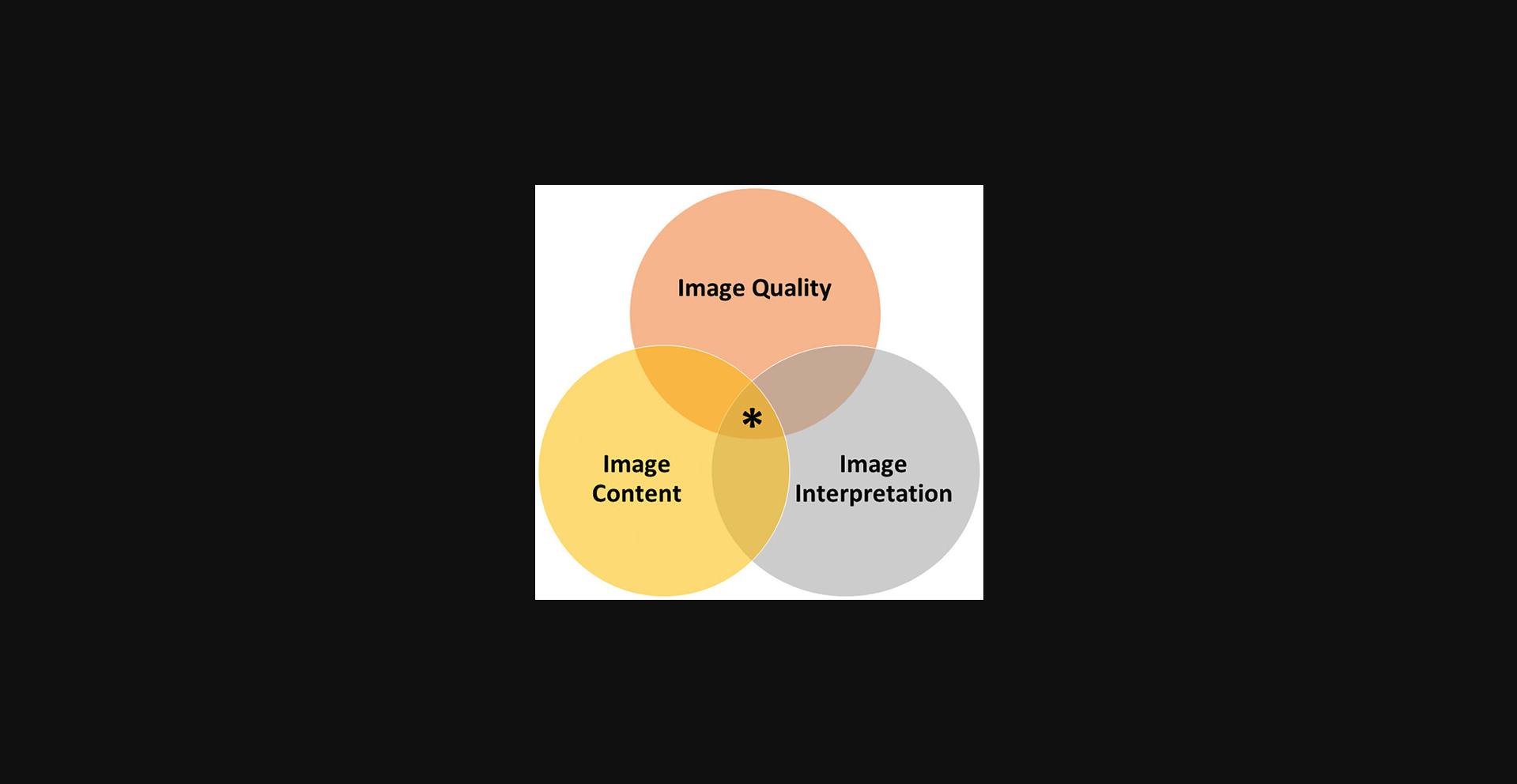
IMAGE QUALITY
Gross Image Quality
Gross images depend on the subject (tissue specimen) and the camera setup. The sample should be logically oriented to demonstrate the features of interest, and the background should be clean (blood and fluid removed with an absorbent material) and clutter-free. A scale marker, such as a ruler, is needed to indicate the true size of the specimen. The camera should be above the specimen, which should fill the frame and be in sharp focus. Lighting should be adequate without shadowing (Jambor et al., 2021; Rampy & Glass, 2016).
Microscopic Image Quality
Many factors affect the integrity of histopathology images, including tissue, equipment, and researcher-controlled factors.
Tissue factors
A high-quality pathology image depends on adequate processing of the biological material. Fresh tissue must be quickly placed in fixative (commonly formalin), sufficiently fixed, uniformly infiltrated with paraffin, and optimally oriented for embedding. The tissue must then be thinly sectioned from the paraffin block without tearing and satisfactorily stained with H&E or IHC stains (Knoblough & Randolph-Habecker, 2018).
Equipment factors
Image quality depends on the quality of the light microscope, camera, and software. Microscope factors include objective lenses and illumination. Camera and software factors include white balance, resolution, brightness, saturation, contrast, and color depth. Images may further be modified by using commercial software such as Adobe Photoshop. Readers are referred to detailed review articles for an explanation of these elements (Goodwin et al., 2018; Riley et al., 2020; Sedgewick, 2017).
Investigator-controlled factors
The size, magnification, and focus of a histopathology image are determined by the investigator capturing the image. In medical and veterinary pathology journals (see Internet Resources), histopathology figures are often allocated a single page containing up to six individual panels. These images provide detailed morphologic information, as the emphasis is on diagnosis and refined characterization of disease entities. In contrast, histopathology images in biomedical research journals are commonly incorporated into multi-panel figures that partially occupy a journal page. The histopathology panel typically contains multiple small images—a practice termed “postage stamp” pathology (Cardiff, Miller et al., 2014). Histopathology images in the research literature consequently are often non-informative—the images are too small for morphologic details to be discerned. The problem is compounded if these small images are captured at low magnification and are not sharply focused.
Improving image quality
To mitigate the limitations of “postage stamp” pathology, the author's approach is to take photographs at high magnification and to size the images as large as possible within the constraints of the journal. Images are captured at 600× magnification (using 60× objective and 10× eyepiece magnification). Including fewer images may be advantageous as it permits those images to be larger. The background must be white-balanced and bright, which may be assisted by increasing the image contrast. A large image at high magnification generally provides sufficient cellular detail when the figure panel size is reduced to meet publication requirements (e.g., Gifford et al., 2021; Kamili et al., 2020). While a scale is recommended for gross images, it is not necessarily mandatory for histopathology images. Scales are typically not employed by pathology journals since the magnification of the tissue section (H&E or IHC stain) is evident by the size of the cellular detail in the image.
IMAGE CONTENT AND INTERPRETATION
Assessing Images of Hematoxylin and Eosin-Stained Tissue Sections
A cancer researcher should become familiar with the anatomy, histology, and human and mouse tumor pathology relevant to the tumor type they are investigating (Fig. 2). Two examples outlining the background knowledge required for understanding different cancer mouse models and, therefore, for interpreting pathology images derived from these models, are outlined in Table 1.
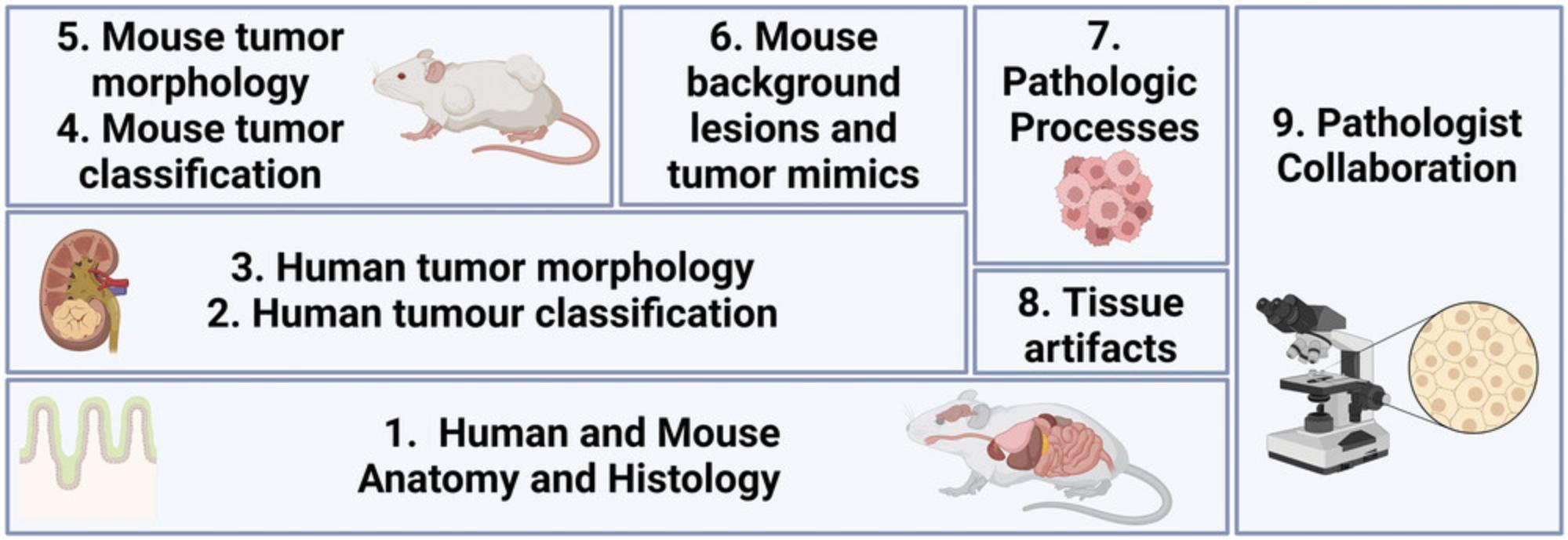
Tumor type | ||
---|---|---|
Background knowledge | Neuroblastoma (NB) | Rhabdomyosarcoma (RMS) |
Mouse model name | Tg(Th-MYCN) 41Waw | NOD.Cg-Prkdcscid Il2rgtm1Wjl/SzJ |
Common mouse model name | Th-MYCN mouse | NSG mouse |
Mouse model type | Transgenic | Patient derived xenograft in immunodeficient mouse |
Relevant normal tissue |
Tumor related: Adrenal gland Sympathetic autonomic ganglia Additional1: Skeletal muscle; Adipose tissue; Lymphoid tissue; Pancreas; Kidney; Blood vessels |
Tumor related: Skeletal muscle Additional1: Fibrocollagenous connective tissue; Adipose tissue; Lymphoid tissue |
Human tumor classification |
INPC Classification2: Neuroblastoma Undifferentiated Poorly-differentiated Differentiating Ganglioneuroblastoma Intermixed Nodular Ganglioneuroma Maturing Mature |
World Health Organisation Classification: Embryonal RMS Alveolar RMS Spindle cell/sclerosing RMS Pleomorphic RMS |
Human tumor morphology |
Neuroblasts (“small round blue cells”) 3 Neuropil Ganglionic differentiation Schwannian stroma4 Mitosis-Karyorrhexis index |
Architecture: Solid architecture Alveolar architecture Cellular morphology: “Small round blue cells” 3 Rhabdomyoblasts Spindle cells Anaplasia |
Mouse tumor classification |
Neuroblastoma Undifferentiated Poorly differentiated |
World Health Organisation Classification:5 Embryonal RMS Alveolar RMS Spindle cell/sclerosing RMS Pleomorphic RMS |
Mouse tumor morphology |
Tumor precursor: Sympathetic autonomic ganglia with neuroblast hyperplasia Tumor: Neuroblasts Neuropil Sympathetic autonomic ganglia6 |
Architecture:5 Solid architecture Alveolar architecture Cellular morphology: “Small round blue cells” Rhabdomyoblasts Spindle cells Anaplasia |
Tissue artifacts |
Poor processing Crush artifact Tissue folding |
Poor processing Crush artifact Tissue folding |
Pathologic processes involving tumor |
Necrosis7 Hemorrhage Calcification |
Necrosis7 Hemorrhage |
Diagnostic Immunohistochemistry | PHOX2B (nuclear) in neuroblasts; S100 (cytoplasmic and nuclear) in Schwannian stroma4 |
Desmin (cytoplasmic) Myogenin (nuclear)8 MyoD1 (nuclear)9 |
Mouse Strain Spontaneous Background Lesions | Teratoma (129S/v)10 |
T-lymphoblastic lymphoma Infections |
Mouse Mimicking Lesions | None known |
Epstein-Barr Virus induced lymphoma11 Xenogeneic graft-versus-host disease |
- 1Non-neoplastic tissue and organs may be present at the periphery of or within excised tumors.
- 2INPC, International Neuroblastoma Pathology Committee.
- 3“Small round blue cell” is a general descriptive term for the microscopic appearance of cells in certain pediatric malignancies which require ancillary investigations, such as immunohistochemistry and molecular analysis, to establish diagnosis.
- 4Schwannian-stroma is observed in human neuroblastic tumors but is not observed in murine tumors from the Th-MYCN model.
- 5For a xenograft, mouse tumor classification and morphologic features are the same as that for a human tumor since the xenograft is of human origin.
- 6A sympathetic autonomic ganglion within a tumor represents either the ganglion from which the tumor is derived or is entrapped by the tumor.
- 7Necrosis may be seen in both mouse and human tumors pre- and post-treatment.
- 8The extent of nuclear myogenin staining is a clue to the presence of a FOXO1 gene fusion; alveolar RMS harboring a FOXO1 fusion typically exhibits diffuse myogenin staining, while variable myogenin staining is observed in embryonal RMS.
- 9Diffuse nuclear staining for MyoD1 may be seen in spindle cell/sclerosing RMS and raises the possibility of a MyoD1 mutation.
- 10Mice were backcrossed to a 129/SvJ strain.
- 11Epstein-Barr Virus (EBV) induced lymphoma in NSG mice arises from proliferation of patient-derived EBV infected B-lymphocytes and must be identified since it may mimic a successfully engrafted tumor.
Antibody1 | Tumor | Staining location2 | Significant staining |
---|---|---|---|
ALK3 | Epithelioid Inflammatory Myofibroblastic Sarcoma | Perinuclear membrane | Positive |
B-catenin4 |
Desmoid-type fibromatosis Hepatoblastoma Nasal angiofibroma Wnt-activated medulloblastoma |
Cytoplasmic and nuclear | Positive |
CD3 | T-lymphoblastic lymphoma | Cytoplasmic | Positive |
CD20 | B-lymphoblastic lymphoma | Cytoplasmic | Positive |
CD99 (MIC2) | Ewing sarcoma | Cytoplasmic membrane | Positive |
Cytokeratin |
Synovial sarcoma Epithelioid sarcoma |
Cytoplasmic | Positive |
Desmin | Rhabdomyosarcoma | Cytoplasmic | Positive |
EMA |
Ependymoma Astroblastoma |
Perinuclear dot-like | Positive |
H3K27me35 |
Malignant peripheral nerve sheath tumor Diffuse midline glioma, H3 K27-altered Posterior fossa type A ependymoma |
Nuclear | Absent |
INI15 |
Malignant rhabdoid tumor Epithelioid sarcoma |
Nuclear | Absent |
Ki67 | All | Nuclear | Positive |
MYCN | Neuroblastoma | Nuclear | Positive |
MyoD1 | Rhabdomyosarcoma | Nuclear | Positive |
Myogenin | Rhabdomyosarcoma | Nuclear | Positive |
NKX2.2 | Ewing sarcoma | Nuclear | Positive |
PAX5 | B-lymphoblastic lymphoma | Nuclear | Positive |
PHOX2B | Neuroblastoma | Nuclear | Positive |
S100 | Neuroblastoma (Schwannian stroma) | Cytoplasmic and nuclear | Positive |
SATB2 | Osteosarcoma | Nuclear | Positive |
TdT | B- or T-lymphoblastic lymphoma | Nuclear | Positive |
TLE1 |
Synovial sarcoma Malignant peripheral nerve sheath tumor |
Nuclear | Positive |
WT16 |
Wilms tumor Desmoplastic small round cell tumor |
Nuclear | Positive |
- 1Certain antibodies also stain non-neoplastic tissue as follows: desmin, smooth muscle and skeletal muscle; CD20 and PAX5, B-lymphocytes; CD3, T-lymphocytes; S100, nerve fibers; PHOX2B, ganglion cells of enteric nervous system; and TdT, thymic cortical lymphocytes.
- 2Only staining in the expected intracellular compartment should be interpreted as positive/significant.
- 3Perinuclear ALK staining is observed in epithelioid inflammatory myofibroblastic sarcoma harboring a RANBP2-ALK gene fusion.
- 4B-catenin is a cytoplasmic stain with the exception of tumors with Wnt-pathway activation where nuclear staining is observed in addition to cytoplasmic staining.
- 5INI1 and H3K27me3 are normally expressed in all non-neoplastic tissue and tumors that are not INI1- or H3K27me3-deficient.
- 6Cytoplasmic WT1 staining is non-diagnostic. In desmoplastic small round cell tumor, nuclear WT1 positivity is observed for antibodies directed against the C-terminus (not the N-terminus).
Parameter | Descriptors |
---|---|
Distribution | Focal, Patchy or Diffuse |
Intensity | Negative (absent), Blush (very weak), Weak, Moderate or Strong |
Intracellular location | Cytoplasmic membrane, Cytoplasmic, Nuclear or Perinuclear membrane |
Specific examples of descriptions of immunohistochemical staining | |
Antibody (tumor) | Description |
Myogenin (rhabdomyosarcoma) | Patchy moderate to strong nuclear staining in ∼10% of tumor cells1 |
PHOX2B (neuroblastoma) | Diffuse strong nuclear staining |
CD99 (Ewing sarcoma) | Diffuse strong cytoplasmic membrane staining |
INI1 (malignant rhabdoid tumor) | There is loss of nuclear staining for INI1 in tumor cells while retained nuclear staining is observed in endothelial cells and inflammatory cells |
- 1In rhabdomyosarcoma, the percentage of myogenin positive cells is documented because tumors with diffuse staining are more likely to be of alveolar subtype while in embryonal rhabdomyosacoma typically a smaller proportion of tumor cells are myogenin positive.
Be familiar with the appearance of normal human and mouse organs and tissue
Knowing the appearance of the human and mouse organs from which a tumor arises is critical. One must know the “normal range” (anatomy and histology) before being able to understand abnormal (pathology). For example, neuroblastoma researchers should be able to recognize a normal adrenal gland and sympathetic autonomic ganglion, while colon carcinoma investigators should recognize normal colon and lung carcinoma researchers, normal lung. This permits recognition of the misidentification of internal organs (such as pancreas misidentified as adrenal gland or small intestine mistaken for colon) or when a normal anatomical structure is misconstrued as neoplasia (such as when mouse preputial glands are diagnosed as a neoplasm) (Ward et al., 2017).
It is also important to recognize other non-neoplastic tissue elements to avoid misclassification as lesional tissue. Tumor sampling from GEMMs or PDXs often contains non-neoplastic tissue including skeletal muscle, fibrocollagenous connective tissue, adipose tissue, and blood vessels. Sampled large arteries and veins, such as aorta or vena cava, reflect normal blood vessels entrapped by the tumor rather than representing neovascularization.
Normal human and mouse anatomy and histology knowledge can be obtained from excellent textbooks (Histology for Pathologists, 5th ed.; Comparative Anatomy and Histology–A Mouse Rat and Human Atlas, 2nd ed) and by reviewing, at the microscope, non-neoplastic tissues sampled from mice.
Develop an understanding of human tumor classification
Up-to-date knowledge of tumor classification facilitates placing a mouse model in its correct clinicopathological context. For example, it is incumbent upon childhood cancer researchers to understand the classification of pediatric solid tumors such as rhabdomyosarcoma (RMS) and peripheral neuroblastic tumors. The World Health Organization (WHO) Classification of Tumours, known colloquially as the “WHO Blue Books”, is an invaluable resource regularly used by pathologists and clinicians alike (see Internet Resources).
Be familiar with human tumor morphology
Critically assessing a tumor image in a research journal is facilitated by familiarity with the gross and microscopic appearance of the human tumor. For example, peripheral neuroblastic tumors have a very different gross appearance depending upon their maturity (Shimada et al., 1999)—an undifferentiated neuroblastoma is hemorrhagic and friable while a ganglioneuroma is solid and pale. The gross appearance is a clue to the underlying histopathology. Knowledge of tumor morphology may be gained from various sources including specialist pathology textbooks, pathology journals, and online resources such as the WHO Classification of Tumours (see Internet Resources). Reviewing diagnostic patient material with an anatomic pathologist is another excellent way to become familiar with tumor histopathology.
Develop an understanding of current mouse tumor classification
Standardized histopathologic diagnostic criteria for rodent (mouse and rat) neoplastic and non-neoplastic lesions have been published in the International Classification of Rodent Tumors: The Mouse (Mohr, 2001) and by the International Harmonization of Nomenclature and Diagnostic Criteria for Lesions in Rats and Mice (INHAND) initiative (see Internet Resources).
Be familiar with the appearance of the cognate mouse tumor
Knowledge of mouse pathology may be accumulated by directly working with a mouse model. A researcher may learn gross tumor appearance, location, and incidence by performing mouse necropsies. Reviewing the excised mouse tumor samples, tissues, and organs at the microscope with an experienced pathologist is also instructive. The morphologic appearance of specific lesions may be gained from consensus publications such as the INHAND guides or online repositories such as the Mouse Models of Human Cancer Database and Pathbase (Begley et al., 2022). For researchers employing mouse models of neuroblastoma, a pathology resource is available (Gifford et al., 2021). The author will also review mouse tumor samples obtained from PDXs or neuroblastoma GEMMs with researchers at the microscope.
Know the basic pathologic processes that may affect tumors
A basic outline of pathology terms has been provided elsewhere (Cardiff, Miller et al., 2014) and in detail in standard textbooks of pathology. Researchers should understand basic processes—such as acute inflammation (infiltration of neutrophils), chronic inflammation (infiltrate containing lymphocytes, plasma cells and macrophages), hemorrhage (extravasation of erythrocytes into tissue), necrosis (tissue death with loss of nuclear detail), and karyorrhexis (nuclear fragmentation)—and be able to recognize when these terms are correctly or incorrectly reported in a figure legend.
Recognize tissue artifacts
Manual handling, storage, and processing of mouse tissue may create morphologic changes that impact assessment of the tissue sections. Morphology may be obscured by inadequate formalin fixation and prolonged storage of tissue in ethanol, resulting in brittle tissue, and inadequate processing in the preparation of paraffin blocks (Cardiff, Miller & Munn, 2014; Knoblaugh & Randolph-Habecker, 2018). Neuroblastomas excised from Th-MYCN mice often show crush artifacts at the periphery. The crushed tissue may mimic neuropil, the presence of which changes the diagnosis from undifferentiated neuroblastoma to poorly differentiated neuroblastoma; therefore, the tumor should be examined for neuropil distant to the edge of the specimen.
Be familiar with background lesions occurring in the mouse model
Background lesions are regarded as those occurring commonly in individual mouse strains (Szymanska et al., 2014). It is important not to misinterpret background lesions as being experimentally induced (Brayton et al., 2012; Pettan-Brewer & Treuting, 2011). Immunodeficient mice used in xenograft studies, such as NOD.Cg-Prkdcscid Il2rgtm1Wjl /SzJ (NOD scid gamma, NSG) mice, may develop immunodeficiency-related pathology that mimics a successfully engrafted human tumor. NSG mice may develop B-cell lymphomas following inoculation, secondary to expansion of patient-derived Epstein-Barr virus (EBV)-infected lymphocytes, and may spontaneously develop T-lymphoblastic lymphoma and certain infections (Santagostino et al., 2022; Tillman et al., 2020). Histopathologic confirmation of xenografted tumor material is, therefore, essential to confirm successful engraftment.
Collaborate with a medical or veterinary anatomical pathologist
Anatomical pathologists provide critical input into the design and interpretation of studies involving mouse models of disease (Cardiff, Miller et al., 2014; Hoenerhoff et al., 2021). Pathologists are an important source of practical information on tumor classification and the gross and microscopic appearance of tumors. Pathologists are willing and able teachers of human and mouse histology and pathology. They can provide guidance on gross and microscopic photography and for composing detailed figure legends.
Assessing Images of Immunohistochemically Stained Tissue Sections
Technical aspects of IHC and antibody selection
Technical aspects of IHC are beyond the scope of this manuscript and have been reviewed in detail elsewhere (Ramos-Vara & Miller, 2014; Ward & Rehg, 2014). When selecting a new antibody to optimize, a single band at the expected molecular weight on a western blot indicates that an antibody may be suitable for IHC. Factors affecting the performance of an IHC assay include pre-analytical factors such as fixation delay, duration of formalin fixation, and tissue processing (Engel & Moore, 2011), and analytical factors including the choice of antibody, reaction conditions, and equipment (Ramos-Vara & Miller, 2014; Ward & Rehg, 2014).
Control tissue
Control tissue is critically important for the performance and interpretation of all IHC assays (Hewitt et al., 2014; Ramos-Vara & Miller, 2014; Ward & Rehg, 2014). Tissue controls may be classified as positive or negative controls and as internal or external controls. Positive controls express the protein of interest in sufficient quantities to be detectible in an IHC assay. Negative controls lack tissue expression of the protein of interest. Internal controls are present within the target tissue being assessed, such as epithelium, lymphocytes, or endothelial cells. External controls are from another sample known to express the protein of interest. Diagnostic histopathology laboratories commonly employ an external positive control that is cut onto the same slide as the target tissue, ensuring the IHC assay works on that slide. An internal positive control may or may not be present in the target tissue depending on the antibody.
Antibody staining
This assessment of antibody staining applies to both IHC-stained glass slides and images captured from these slides. IHC staining is always interpreted knowing the H&E appearance of the tissue, ensuring that staining is only interpreted in the correct, well-processed, viable tissue.
Antibody staining may be assessed with respect to distribution , intensity , and intracellular location of staining (Tables 2-3; Figs. 3-7). In routine pathology practice, these factors are typically assessed by visual inspection and reported in a semiquantitative manner. Distribution is categorized as focal, patchy, or diffuse. Intensity is categorized as negative (absent), blush (very weak), weak, moderate, or strong. Intracellular location is categorized as cytoplasmic membrane, cytoplasmic, nuclear, and nuclear membrane. Some antibodies stain more than one cellular compartment. Combining these three parameters to document IHC staining, an investigator may state, for example, “diffuse strong nuclear staining is present”, or “patchy weak cytoplasmic staining is seen”.
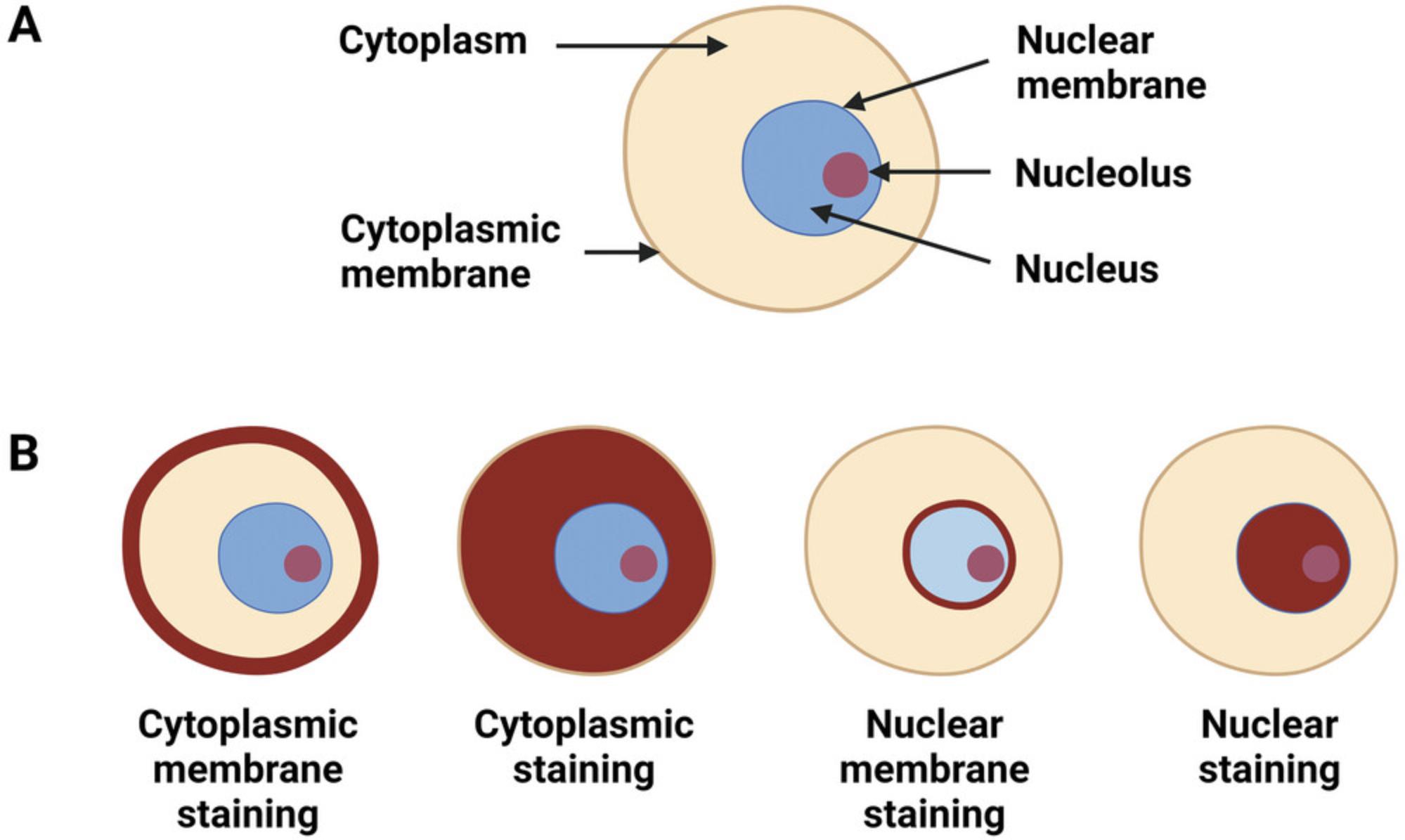
Diagnostically significant staining varies for each antibody. Positive staining is of diagnostic significance for most antibodies (Table 2; Figs. 4-7). For some antibodies, particularly INI1 and H3K27me3, negative staining (i.e., loss of the normal positive staining pattern) is diagnostically significant (Table 2; Fig. 6).
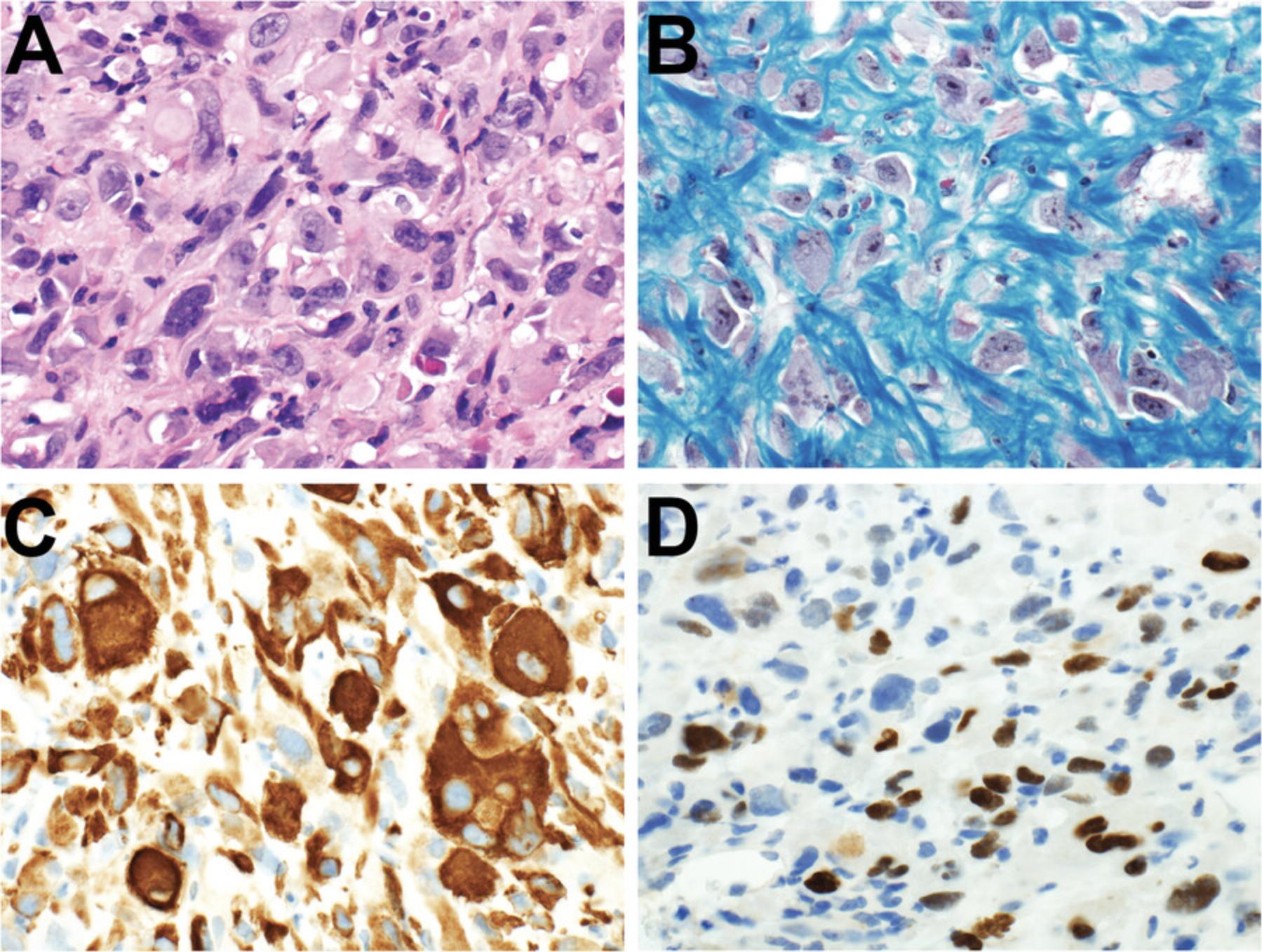
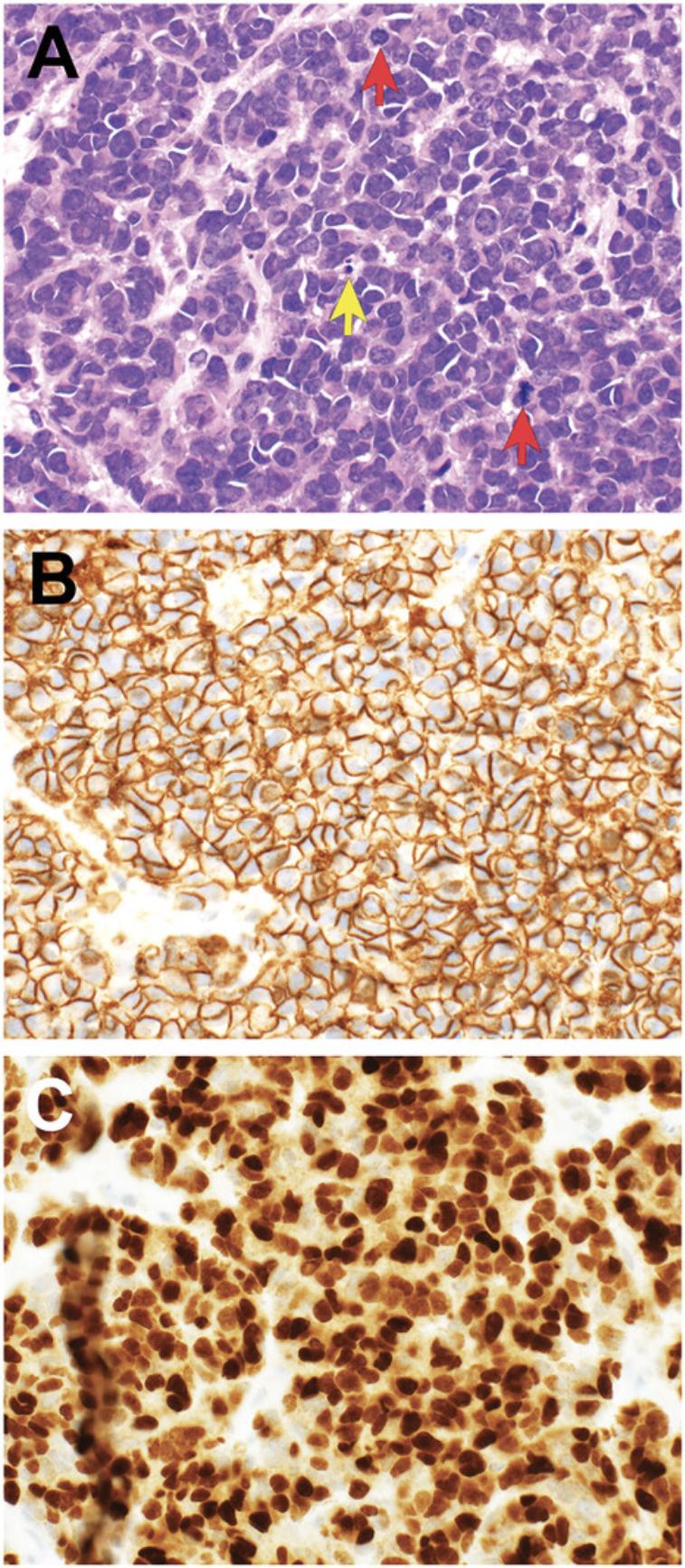
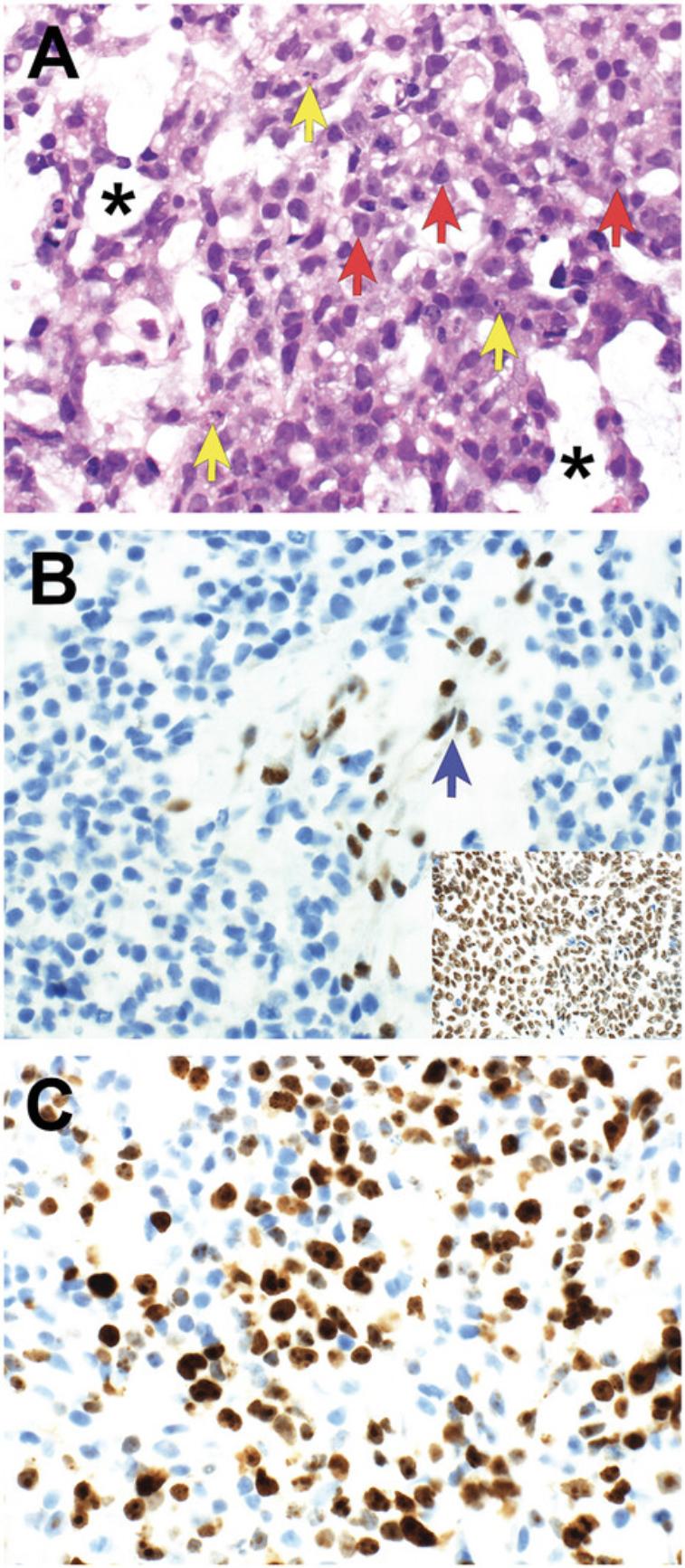
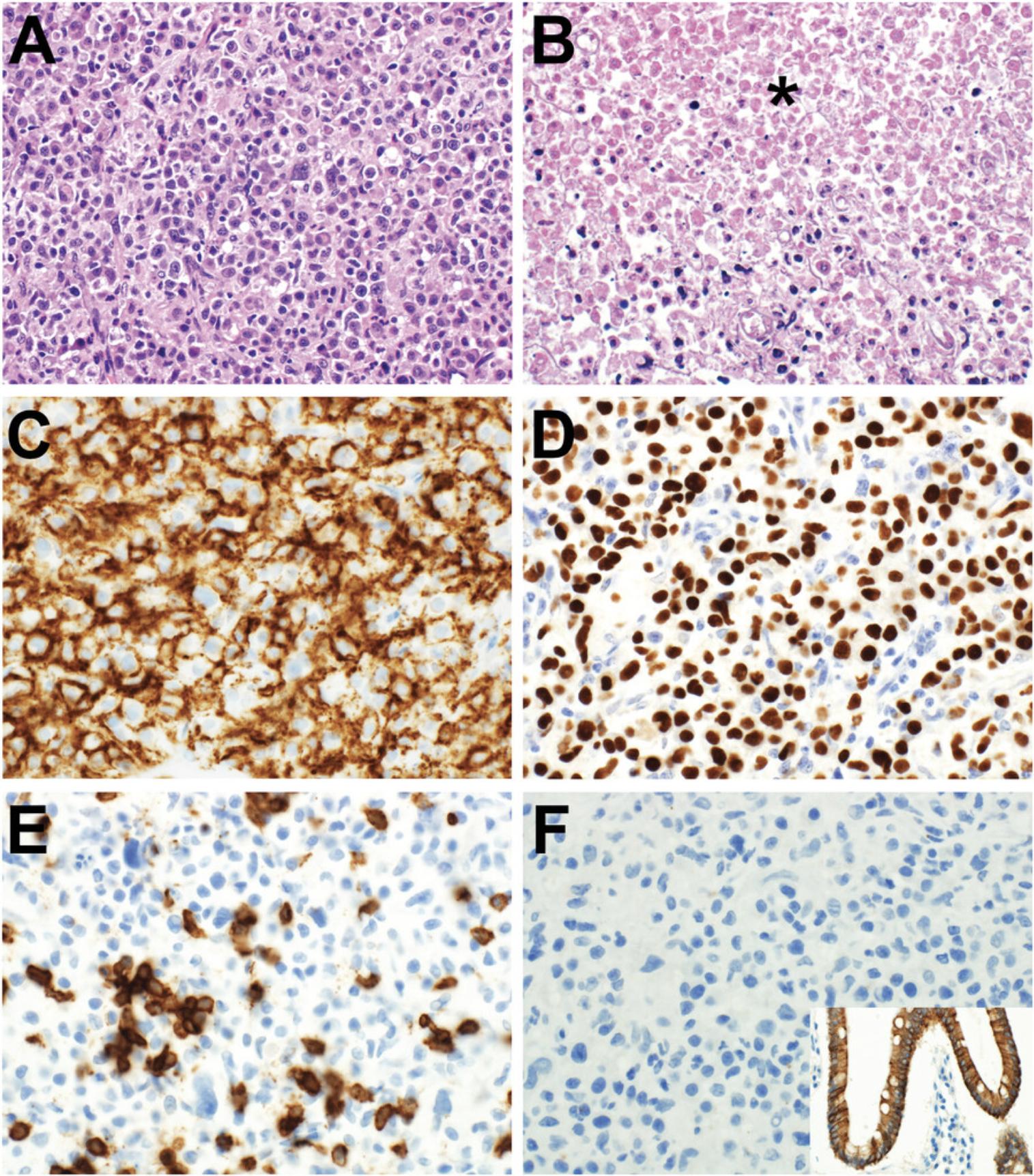
Similar antibody staining may be expected in mouse tumor tissue, human tumor tissue, PDXs and cell cultures derived from either primary patient samples or PDXs. For example, we have documented nuclear staining for the transcription factor PHOX2B in mouse neuroblastoma (Gifford et al., 2021), neuroblastoma PDXs (Kamili et al., 2020), and neuroblastoma cell cultures derived from primary patient tumor tissue (Xie et al., 2021).
Interpretation of IHC staining must take into consideration the intracellular location of the protein (Ward & Rehg, 2014). If antibody staining is observed in a different intracellular compartment, it should not be interpreted as positive. For example, if a protein is expressed in the nucleus, and only cytoplasmic IHC staining is observed, the staining should be disregarded. Similarly, if cytoplasmic membrane staining is expected and only cytoplasmic staining is seen, the observed staining should be ignored. Specific antibody staining must also be distinguished from non-specific staining (Gown, 2016; Ward & Rehg, 2014).
Folded tissue on a glass slide and intracellular pigments, such as hemosiderin, may non-specifically stain in an IHC assay and should not be interpreted as positive staining. Common problems with interpretation of IHC staining are outlined in Table 4.
IHC Problem | Scenario | Interpretation | Action2 |
---|---|---|---|
No H&E-stained image | Panel of IHC images provided in figure though no image of an H&E stained tissue section is included. | IHC staining should only be interpreted in light of the H&E appearance of the tissue. e.g., if positive IHC staining is present, an H&E stained section will facilitate whether staining is present in viable tumor, necrotic tumor and/or non-neoplastic tissue also present in the tissue section. | Request an adequate H&E-stained image be included. |
No external positive control tissue is documented for an antibody | Tissue stained with antibody is negative (i.e., no antibody staining is present). | Unable to interpret staining pattern on slide. For a negative stain, without a positive control, it is uncertain whether the negative staining is due to true negative staining or technical failure of the assay. | An appropriate positive control must be documented. Include a statement that control tissue stained as expected with the antibody. Images of positive control tissue may be included. |
Internal positive control tissue has not stained | This is relevant to antibodies where the significant result is loss of positive staining in a tumor. e.g., INI1 staining in a malignant rhabdoid tumor: Diagnostic staining is loss of nuclear INI1 staining in tumor cells. As INI1 stains all normal tissues, staining should be seen in vascular endothelial cells and inflammatory cells. If endothelial and inflammatory cell staining is absent there is a problem. | Unable to conclude that the antibody staining is reliable. Absent staining may be related to pre-analytical factors affecting tissue antigenicity or technical failure of the assay. | Staining needs to be repeated. Appropriate staining of internal positive control tissue, along with external positive control tissue, must be documented. |
Only cytoplasmic staining seen for nuclear antibody | Antibody is directed against an antigen known to be located in the nucleus (examples of nuclear antibodies are in Table 2). Only cytoplasmic staining is seen in the slide/image. | Staining should be ignored and cannot be regarded as evidence of positive staining. | Image should not be included in manuscript. Request image showing positive nuclear staining. Staining needs to be repeated with an optimized antibody. |
Only cytoplasmic staining seen for cytoplasmic membrane antibody | Antibody is directed against an antigen known to be located in the cytoplasmic membrane (e.g., CD99/MIC2 in Ewing sarcoma). Only cytoplasmic staining is seen in the slide/image. | Staining should be ignored and cannot be regarded as evidence of positive staining. | Image should not be included in manuscript. Request image showing positive cytoplasmic membrane staining. Staining needs to be repeated with an optimized antibody. |
Excess background staining | Antibody stains all tissue on the slide/image. | Should not be interpreted as positive staining. | Image should not be included in manuscript. Staining needs to be repeated with an optimized antibody. |
Staining is present in necrotic tissue | Necrosis is present in the tissue section, confirmed with an H&E stained slide. Antibody staining is present within necrosis. | Staining should be ignored. | Image should not be included in manuscript. Assess immunostaining in viable tissue. |
Staining is strongest at edge of tissue | Antibody staining is strongest at the edge of the tissue section and is weak to absent in the remainder of the tissue section. | Staining should be ignored. This likely represents “edge effect” artifact. | Image should not be included in manuscript. Assess staining away from edge of tissue section. |
Staining is present in cellular pigment | Tissue containing hemosiderin3 or melanin4 pigment is stained brown in an IHC assay. | Staining should be ignored. | Image should not be included in manuscript. Assess staining in tissue tissue lacking pigment. |
Staining is present in folded tissue | Folding of tissue sections occur during slide preparation. These folds may entrap reagents in an IHC assay and appear positively stained. | Staining should be ignored. | Image should not be included in manuscript. Assess staining in non-folded tissue. |
- 1The features described here apply to interpretation of both the original tissue sections on glass slides and the images captured (photos) of the tissue sections for publication.
- 2“Action” refers to steps that may be taken in either the investigator's own work or recommendations that can be made to a journal if reviewing a manuscript.
- 3Hemosiderin is brown refractile pigment deposited in tissue following hemorrhage. Hemosiderin is commonly present within macrophages (hemosiderin-laden macrophages) and stains blue with a Perls Prussian Blue stain.
- 4Melanin, present in skin macrophages (melanophages), may be identified with a melanin bleach stain, Fontana-Masson stain or Schmorl stain.
PATHOLOGY-BASED FIGURE LEGENDS
The composition of a figure legend draws upon the investigator's knowledge of human and mouse disease processes, anatomy, and histology, and the ability to interpret IHC staining. It is generally clear from the wording of a figure legend whether the author is sufficiently literate in “pathology-speak” (Cardiff, Miller et al., 2014). One approach is to state what is in the image without further interpretation (Cardiff, Miller et al., 2014; Ward et al., 2017): “H&E staining (Panel A) and myogenin immunohistochemistry (Panel B) in rhabdomyosarcoma”. Whilst this detail may be correct, the information conveyed to the reader is limited. An alternative approach to describe what is in the image and offer an interpretation: “Embryonal rhabdomyosarcoma showing hypercellular and hypocellular areas and several tumor cells, consistent with rhabdomyoblasts, with bright eosinophilic cytoplasm and cross-striations (H&E stain) (Panel A). Diagnostic immunohistochemical staining for myogenin demonstrating moderate-strong nuclear staining in a small proportion of tumor cells (Panel B).” This detailed approach conveys more information and confidence that the author understands the pathology data being presented. It is recommended that pathologist input is sought for writing pathology-based figure legends.
ILLUSTRATIVE EXAMPLES CRITICALLY ASSESSING PATHOLOGY IMAGE DATA IN THE PUBLISHED LITERATURE
These guidelines may be applied by approaching any pathology image in the literature with a series of questions (Table 5). The questions are grouped according to the image parameters outlined above: image quality, image content, and image interpretation. If the answer to any of the questions highlights an issue with the image, then changes should be recommended. Examples of pathology images for which improvements could made, including examples drawn from review of the author's previous publications, are presented in Table 6.
Image parameter | Question |
---|---|
Image quality |
|
Image content | Gross images:
|
Image interpretation |
|
Issue type | Publication figure | Image/ stain type | Description | Action1 | Reference | |
---|---|---|---|---|---|---|
1 | Image quality | 5, 6A, 7, and 8B | Gross, H&E | Gross image is too dark. H&E images have different background lighting; images with a darker background are adjacent to images with a bright background. | Ensure images are not darkened following uploading to journal. Aim for similar background brightness in all images prior to submission. | Gifford et al., 2021 |
2 | Image quality and Image interpretation | 6B | H&E, IHC | Images small, magnification too low and images not in sharp focus precluding assessment of morphologic details. No description of morphologic details in figure legend. | Increase image size and capture images at higher magnification to demonstrate cellular detail. Provide a description of morphologic findings in figure legend. | Tsoli et al., 2018 |
3 | Image interpretation | 3A | Orcein elastic, IHC | Figure legend lists features (“bd, interlobular bile duct; ha, hepatic artery branch”) not labeled in the accompanying image. | Add annotation to image or remove reference to the anatomical structure in figure legend. | Kamili et al., 2020 |
4 | Image quality, Image content, and Image interpretation | 1E, i-v | H&E | Images too dark obscuring morphologic details. An organ is incorrectly identified (1Eiii shows pancreas which is designated adrenal gland in figure legend 1Eiv). Figure legend description for panels Eiii-v does not match what is shown in images. | Increase image brightness. Re-label organ as pancreas, or take new image showing adrenal gland. Reorder images or figure legend so images and description match. | Webb et al., 2020 |
5 | Image content and Image interpretation | 2 | IHC | What is shown in figure does not match description in figure legend. Stated in figure legend that tumors “expressed high levels” of MYCN and PHOX2B. These are nuclear stains. The images show patchy minimal nuclear staining and predominant cytoplasmic staining. | Appears to be a technical issue with IHC staining. Repeat MYCN and PHOX2B staining. Antibody re-optimization or alternate antibody clones may be required. Photos should be re-taken following optimized antibody staining. | Ke et al., 2015 |
- 1“Action” refers to recommendations that could have been proposed prior to publication, during manuscript preparation or while under review following submission. The intention is not to correct these images as they currently stand in the published literature.
CONCLUDING REMARKS
The ability to critically assess the content and interpretation of gross and microscopic pathology images requires specific knowledge that is achievable for research scientists. Pathology is a scientific discipline that relies upon pattern recognition. Research scientists are not expected to possess the diagnostic acumen of an experienced, formally trained pathologist. However, with a focused knowledge base of human and mouse gross anatomy, histology, and pathology (relevant to their research field), scientists can critically assess pathology images in the literature. Researchers are then equipped to identify problems with image quality and accuracy and to recommend changes to these images.
ACKNOWLEDGMENTS
Children's Cancer Institute Australia is affiliated with UNSW Sydney and Sydney Children's Hospitals Network. The xenograft models photographed for this manuscript were generated by the Zero Childhood Cancer Precision Medicine Program at the Children's Cancer Institute with the support of Children's Cancer Institute Animal Facility. The Zero Childhood Cancer Precision Medicine Program was funded by the Australian Federal Government Department of Health. Zero Childhood Cancer is a joint initiative led by the Children's Cancer Institute and Sydney Children's Hospital, Randwick. Figures 2 and 3 were created with BioRender.com.
Open access publishing facilitated by University of New South Wales, as part of the Wiley - University of New South Wales agreement via the Council of Australian University Librarians.
AUTHOR CONTRIBUTIONS
Andrew J. Gifford : Conceptualization; formal analysis; methodology; original draft, review, and editing.
CONFLICT OF INTEREST
The author declares no conflict of interest.
Open Research
DATA AVAILABILITY STATEMENT
Data is available on request.
LITERATURE CITED
- Begley, D. A., Schofield, P. N., & Sundberg, J. P. (2022). The mouse online: Open mouse biology and pathology data resources for biomedical resources. In J. P. Sundberg, P. Vogel, & J. M. Ward (Eds.) Pathology of Genetically Engineered and Other Mutant Mice (pp. 6–21). Wiley Blackwell. https://doi.org/10.1002/9781119624608.ch2
- Brayton, C., Justice, M., & Montgomery, C. A. (2001). Evaluating mutant mice: Anatomic pathology. Veterinary Pathology , 38(1), 1–19. https://doi.org/10.1354/vp.38-1-1
- Brayton, C. F., Treuting, P. M., & Ward, J. M. (2012). Pathobiology of aging mice and GEM: Background strains and experimental design. Veterinary Pathology , 49(1), 85–105. https://doi.org/10.1177/0300985811430696
- Cardiff, R. D., Ward, J. M., & Barthold, S. W. (2008). 'One medicine–one pathology': Are veterinary and human pathology prepared? Laboratory Investigation; A Journal of Technical Methods and Pathology , 88(1), 18–26. https://doi.org/10.1038/labinvest.3700695
- Cardiff, R. D., Miller, C. H., & Munn, R. J. (2014a). Analysis of mouse model pathology: A primer for studying the anatomic pathology of genetically engineered mice. Cold Spring Harbor Protocols , 2014(6), 561–580. https://doi.org/10.1101/pdb.top069922
- Cardiff, R. D., Miller, C. H., & Munn, R. J. (2014b). Mouse tissue fixation. Cold Spring Harbor Protocols , 2014(5), pdb.prot073403. https://doi.org/10.1101/pdb.prot073403
- Couto, S. S., & Cardiff, R. D. (2008). The genomic revolution and endocrine pathology. Endocrine Pathology , 19(3), 139–147. https://doi.org/10.1007/s12022-008-9042-2
- Engel, K. B., & Moore, H. M. (2011). Effects of preanalytical variables on the detection of proteins by immunohistochemistry in formalin-fixed, paraffin-embedded tissue. Archives of Pathology & Laboratory Medicine, 135(5), 537–543. https://doi.org/10.5858/2010-0702-RAIR.1
- Gifford, A. J., Murray, J., Fletcher, J. I., Marshall, G. M., Norris, M. D., & Haber, M. (2021). A primer for assessing the pathology in mouse models of neuroblastoma. Current Protocols , 1(11), e310. https://doi.org/10.1002/cpz1.310
- Goodwin, P. C., Johnson, B., & Frevert, C. W. (2018). 4 - Microscopy, Immuno-Histochemistry, Digital Imaging, and Quantitative Microscopy. In P. M. Treuting, S. M. Dintzis, & K. S. Montine (Eds.), Comparative anatomy and histology ( 2nd ed., pp. 53–66). Academic Press. https://doi.org/10.1016/B978-0-12-802900-8.00004-X
- Gown, A. M. (2016). Diagnostic immunohistochemistry: What can go wrong and how to prevent it. Archives of Pathology & Laboratory Medicine, 140(9), 893–898. https://doi.org/10.5858/arpa.2016-0119-RA
- Hewitt, S. M., Baskin, D. G., Frevert, C. W., Stahl, W. L., & Rosa-Molinar, E. (2014). Controls for immunohistochemistry: The Histochemical Society's standards of practice for validation of immunohistochemical assays. Journal of Histochemistry and Cytochemistry , 62(10), 693–697. https://doi.org/10.1369/0022155414545224
- Hoenerhoff, M. J., Meyerholz, D. K., Brayton, C., & Beck, A. P. (2021). Challenges and opportunities for the veterinary pathologist in Biomedical Research. Veterinary Pathology , 58(2), 258–265. https://doi.org/10.1177/0300985820974005
- Ince, T. A., Ward, J. M., Valli, V. E., Sgroi, D., Nikitin, A. Y., Loda, M., Griffey, S. M., Crum, C. P., Crawford, J. M., Bronson, R. T., & Cardiff, R. D. (2008). Do-it-yourself (DIY) pathology. Nature Biotechnology , 26(9), 978–979. https://doi.org/10.1038/nbt0908-978
- Jambor, H., Antonietti, A., Alicea, B., Audisio, T. L., Auer, S., Bhardwaj, V., Burgess, S. J., Ferling, I., Gazda, M. A., Hoeppner, L. H., Ilangovan, V., Lo, H., Olson, M., Mohamed, S. Y., Sarabipour, S., Varma, A., Walavalkar, K., Wissink, E. M., & Weissgerber, T. L. (2021). Creating clear and informative image-based figures for scientific publications. PLoS Biology , 19(3), e3001161. https://doi.org/10.1371/journal.pbio.3001161
- Kamili, A., Gifford, A. J., Li, N., Mayoh, C., Chow, S. O., Failes, T. W., Eden, G. L., Cadiz, R., Xie, J., Lukeis, R. E., Norris, M. D., Haber, M., McCowage, G. B., Arndt, G. M., Trahair, T. N., & Fletcher, J. I. (2020). Accelerating development of high-risk neuroblastoma patient-derived xenograft models for preclinical testing and personalised therapy. British Journal of Cancer , 122, 680–691. https://doi.org/10.1038/s41416-019-0682-4
- Ke, X. X., Zhang, D., Zhao, H., Hu, R., Dong, Z., Yang, R., Zhu, S., Xia, Q., Ding, H. F., & Cui, H. (2015). Phox2B correlates with MYCN and is a prognostic marker for neuroblastoma development. Oncology Letters , 9(6), 2507–2514. https://doi.org/10.3892/ol.2015.3088
- Kersten, K., de Visser, K. E., van Miltenburg, M. H., & Jonkers, J. (2017). Genetically engineered mouse models in oncology research and cancer medicine. EMBO Molecular Medicine , 9(2), 137–153. https://doi.org/10.15252/emmm.201606857
- Knoblaugh, S. E., & Randolph-Habecker, J. (2018). 3 - Necropsy and Histology. In P. M. Treuting, S. M. Dintzis, & K. S. Montine (Eds.), Comparative Anatomy and Histology ( 2nd ed., 23–51). Academic Press. https://doi.org/10.1016/B978-0-12-802900-8.00003-8
- Marqués, G., Pengo, T., & Sanders, M. A. (2020). Imaging methods are vastly underreported in biomedical research. eLife , 9, e55133. https://doi.org/10.7554/eLife.55133
- U. Mohr (Ed.). (2001). International classification of rodent tumors: The mouse. Heidelberg, Germany: Springer-Verlag.
- Pettan-Brewer, C., & Treuting, P. M. (2011). Practical pathology of aging mice. Pathobiology of Aging & Age-related Diseases, 1, https://doi.org/10.3402/pba.v1i0.7202
- Ramos-Vara, J. A., & Miller, M. A. (2014). When tissue antigens and antibodies get along: Revisiting the technical aspects of immunohistochemistry—the red, brown, and blue technique. Veterinary Pathology , 51(1), 42–87. https://doi.org/10.1177/0300985813505879
- Rampy, B. A., & Glassy, E. F. (2016). Pathology gross photography: The beginning of digital pathology. Clinics in Laboratory Medicine , 36(1), 67–87. https://doi.org/10.1016/j.cll.2015.09.008
- Riley, R. S., Almenara, J., & Fuller, C. E. (2020). Digital Micrographs in Pathology. In R. Pelc, W. Walz, & J. R. Doucette (Eds), Neurohistology and Imaging Techniques. Neuromethods (Vol. 153). Humana. https://doi.org/10.1007/978-1-0716-0428-1_14
- Santagostino, S. F., Monette, S., Piersigilli, A., Godfrey, V. L., & HogenEsch, H. (2022). Immunodeficient and humanized mice. In J. P. Sundberg, P. Vogel, & J. M. Ward (Eds.), Pathology of genetically engineered and other mutant mice. Wiley Blackwell. (pp. 132–158). https://doi.org/10.1002/9781119624608.ch9
- Schofield, P. N., Vogel, P., Gkoutos, G. V., & Sundberg, J. P. (2012). Exploring the elephant: Histopathology in high-throughput phenotyping of mutant mice. Disease Models & Mechanisms, 5(1), 19–25. https://doi.org/10.1242/dmm.008334
- Sedgewick, J. (2017). Practical considerations when altering digital images. Current Protocols Essential Laboratory Techniques , 14, A.3B.1–A.3B.33. https://doi.org/10.1002/cpet.11
- Shimada, H., Ambros, I. M., Dehner, L. P., Hata, J., Joshi, V. V., & Roald, B. (1999). Terminology and morphologic criteria of neuroblastic tumors: Recommendations by the International Neuroblastoma Pathology Committee. Cancer , 86(2), 349–363. https://doi.org/10.1002/(SICI)1097-0142(19990715)86:2<349::AID-CNCR20>3.0.CO;2-Y
- J. P. Sundberg, P. Vogel, & J.M. Ward (Eds.) (2022). Pathology of genetically engineered mice and other mutant mice. Wiley Blackwell. https://doi.org/10.1002/9781119624608
- Szymanska, H., Lechowska-Piskorowska, J., Krysiak, E., Strzalkowska, A., Unrug-Bielawska, K., Grygalewicz, B., Skurzak, H. M., Pienkowska-Grela, B., & Gajewska, M. (2014). Neoplastic and nonneoplastic lesions in aging mice of unique and common inbred strains contribution to modeling of human neoplastic diseases. Veterinary Pathology , 51(3), 663–679. https://doi.org/10.1177/0300985813501334
- Tillman, H., Janke, L. J., Funk, A., Vogel, P., & Rehg, J. E. (2020). Morphologic and immunohistochemical characterization of spontaneous lymphoma/leukemia in NSG mice. Veterinary Pathology , 57(1), 160–171. https://doi.org/10.1177/0300985819882631
- Tsoli, M., Wadham, C., Pinese, M., Failes, T., Joshi, S., Mould, E., Yin, J. X., Gayevskiy, V., Kumar, A., Kaplan, W., Ekert, P. G., Saletta, F., Franshaw, L., Liu, J., Gifford, A., Weber, M. A., Rodriguez, M., Cohn, R. J., Arndt, G., … Ziegler, D. S. (2018). Integration of genomics, high throughput drug screening, and personalized xenograft models as a novel precision medicine paradigm for high risk pediatric cancer. Cancer Biology & Therapy, 19(12), 1078–1087. https://doi.org/10.1080/15384047.2018.1491498
- Valli, T., Barthold, S. W., Ward, J. E., Brayton, C., Nikitin, A., Borowsky, A. D., Bronson, R. T., Cardiff, R. D., Sundberg, J., & Ince, T. (2007). Over 60% of NIH extramural funding involves animal-related research. Veterinary Pathology , 44(6), 962–963. https://doi.org/10.1354/vp.44-6-962
- Ward, J. M., & Rehg, J. E. (2014). Rodent immunohistochemistry: Pitfalls and troubleshooting. Veterinary Pathology , 51(1), 88–101. https://doi.org/10.1177/0300985813503571
- Ward, J. M., Schofield, P. N., & Sundberg, J. P. (2017). Reproducibility of histopathological findings in experimental pathology of the mouse: A sorry tail. Lab Animal , 46(4), 146–151. https://doi.org/10.1038/laban.1214
- Webb, E. R., Lanati, S., Wareham, C., Easton, A., Dunn, S. N., Inzhelevskaya, T., Sadler, F. M., James, S., Ashton-Key, M., Cragg, M. S., Beers, S. A., & Gray, J. C. (2020). Immune characterization of pre-clinical murine models of neuroblastoma. Scientific Reports , 10(1), 16695. https://doi.org/10.1038/s41598-020-73695-9
- Xie, J., Kumar, A., Dolman, M. E. M., Mayoh, C., Khuong-Quang, D. A., Cadiz, R., Wong-Erasmus, M., Mould, E. V. A., Grebert-Wade, D., Barahona, P., Kamili, A., Tsoli, M., Failes, T. W., Chow, S. O., Arndt, G. M., Bhatia, K., Marshall, G. M., Ziegler, D. S., Haber, M., … Gifford, A. J. (2021). The important role of routine cytopathology in pediatric precision oncology. Cancer Cytopathology , 129(10), 805–818. https://doi.org/10.1002/cncy.22448
KEY REFERENCES
- Comparative Anatomy and Histology—A Mouse Rat and Human Atlas, 2nd edition (2018). Treuting, P.M., Dintzis, S.M., and Montine, K.S. (Eds.). Academic Press.
- Histology for Pathologists, 5th edition (2019). Mills, S.E. Lippincott Williams & Wilkins (LWW).
INTERNET RESOURCES
This is the online resource for the World Health Organization Classification of Tumours. It represents the up-to-date pathologic classification of human tumors.
This is the online resource for the International Harmonisation of Nomenclature and Diagnostic Criteria (INHAND) which publishes guidelines defining the pathology of rodent (mouse and rat) non-proliferative and proliferative lesions.
- Pathology Journals.
- The American Journal of Surgical Pathology (https://journals.lww.com/ajsp)
- Modern Pathology (https://www.nature.com/modpathol)
- Histopathology (https://onlinelibrary.wiley.com/journal/13652559)
- Veterinary Pathology (https://journals.sagepub.com/home/VET)
- Toxicologic Pathology (https://journals.sagepub.com/home/TPX)
These journals are the standard publications for human and veterinary diagnostic pathology. Emerging tumor entities are described in these journals, upon which updates of tumor classification are based. These journals contain examples of well-presented pathology figures and well-written figure legends.
GLOSSARY
Background lesions : Lesions commonly and naturally occurring in a particular mouse strain. When assessing genetically engineered mice, knowledge of background lesions occurring in that mouse strain is important for distinguishing changes due to genetic manipulation from those occurring naturally in the mouse strain.
Genetically Engineered Mouse Models (GEMMs) : Mouse models of human disease, including both cancer and non-neoplastic diseases, generated by manipulation of the mouse genome. GEMMs may be produced by several methodologies. Transgenic mice are produced by injection of recombinant DNA (transgene) into the mouse pronucleus with random insertion into the mouse genome. Targeted mutagenesis produces mice in which an exogenous gene or a mutation in an endogenous gene has been introduced into the mouse genome (knock-in mouse) or mice in which a gene has been deleted and/or inactivated (knock-out mouse). More recently, GEMMs have been created via genome editing with programmable endonucleases (e.g., CRISPR-Cas9).
Hematoxylin and eosin (H &E) staining: A method of staining tissue sections for viewing with a light microscope. Hematoxylin is a basic dye that binds to acidic structures within cells and stains the cell nucleus a purple-blue color. Eosin is an acidic dye that binds to basic structures within cells and stains the cytoplasm a pinkish color. The term “eosinophilic” refers to structures stained with eosin; for example, “eosinophilic cytoplasm”.
Histopathology : The examination and description, using a light microscope, of changes in the appearance (morphology) of organ and tissue samples resulting from disease processes. All samples are processed and stained with H&E for assessment. Tumor samples are stained immunohistochemically to detect the presence or absence of relevant proteins, to permit an accurate diagnosis. In medical and veterinary diagnostic pathology, the information provided by the clinical context of the patient and the morphology and immunohistochemical staining of the tissue sample is synthesized in a written report in which a diagnosis is rendered. Histology, in contrast, is the study of normal tissues and their component cells and extracellular structures with a light microscope.
Immunohistochemical (IHC) staining : A method of assessing protein expression in tissue sections. The method commonly involves the binding of specific antibodies to antigens (proteins) present in formalin-fixed paraffin-embedded tissue sections, followed by a colored histochemical reaction for antigen detection, using 3,3′-diaminobenzadine tetrachloride (DAB) as the chromagen. When a particular protein is present in the tissue section (i.e., antigen-antibody binding is present), the DAB chromagen imparts a brown color that is visible on the glass slide with a light microscope.
Neuropil : Thin neuritic processes extending from neuroblasts within a neuroblastoma tumor.
Patient-derived EBV infected lymphocytes : Relevant to the assessment of human tumor patient-derived xenografts in immunodeficient mice. When human tumor cells are engrafted into an immunodeficient mouse, they are sometimes accompanied by inflammatory cells such as lymphocytes. Epstein-Barr virus (EBV) infection is common. EBV infects B-lymphocytes, the proliferation of which is controlled by an intact immune system. The proliferation of EBV-infected B-lymphocytes is unchecked in an immunodeficient mouse; the mass that develops in the mouse is derived from these lymphocytes rather than the patient's original tumor. Histopathologic assessment of xenografts is important to confirm that the xenograft recapitulates the patient's original tumor and not a proliferation of EBV-infected lymphocytes.
Peripheral neuroblastic tumors : Family of tumors arising from the sympathetic nervous system. The most common extracranial tumor in young children. Tumors show a spectrum of maturity (differentiation) and are designated neuroblastoma at the immature end of the spectrum, ganglioneuroma (GN) at the mature end of the spectrum, with g anglioneuroblastoma (GNB) representing an intermediate form. Neuroblastoma contains <50% nerve-like tissue, termed Schwannian stroma. GNB and GN contain >50% Schwannian stroma; a component of neuroblastoma is present in GNB and is absent in GN.
Rhabdomyosarcoma (RMS) : Family of malignant soft-tissue tumors showing evidence of skeletal muscle differentiation. Most common soft tissue sarcoma in children. Subtypes in the pediatric age range consist of embryonal RMS, alveolar RMS and spindle cell/sclerosing RMS. Embryonal RMS, the most common subtype, arises in children in the head and neck and genitourinary system. Alveolar RMS arises in slightly older children and adolescents, involves the distal extremities, harbors a FOXO1 gene rearrangement and is more biologically aggressive than embryonal RMS. Spindle cell/sclerosing RMS is rarer and is divided into congenital spindle cell RMS, harboring a gene fusion between VGLL2, SRF or TEAD1 with NCOA2 or CITED2 ; MYOD1 -mutant spindle cell/sclerosing RMS; and intraosseous spindle cell RMS with a TFCP2 or NCOA2 gene fusion. MYOD1 -mutant and intraosseous spindle cell RMS have a poor prognosis.