Synthesis and Application of a Clickable Epoxomicin-Based Probe for Proteasome Activity Analysis
Andres F. Salazar-Chaparro, Andres F. Salazar-Chaparro, Saayak Halder, Saayak Halder, Darci J. Trader, Darci J. Trader
Abstract
The proteasome is a multisubunit protein complex responsible for the degradation of proteins, making it essential in myriad cellular processes. Several reversible and irreversible peptide substrates inspired by known proteasome inhibitors have been developed to visualize it and monitor its activity; however, they have limited commercial availability or possess fluorophores that overlap with other known chemical probes, limiting their simultaneous use. The protocols presented here describe the synthesis of a clickable epoxomicin-based probe followed by the copper-catalyzed installment of an azide-containing fluorophore, and the application of the synthesized peptide in proteasome activity assays by SDS-PAGE and flow cytometry. © 2022 The Authors. Current Protocols published by Wiley Periodicals LLC.
Basic Protocol 1 : Solid-phase synthesis of clickable peptide fragment (2)
Basic Protocol 2 : In-solution coupling of epoxy-ketone moiety to fragment (2)
Basic Protocol 3 : Copper-catalyzed click reaction of (3) with fluorophore of choice
Basic Protocol 4 : Monitoring proteasome activity by SDS-PAGE in HEK-293T cells
Alternate Protocol : Monitoring proteasome activity by flow cytometry in HEK-293T cells
INTRODUCTION
To maintain a functional proteome, an exhaustive network of regulators and chaperones is required to ensure an appropriate balance between protein synthesis, conformational maintenance, and degradation (Hipp, Kasturi, & Hartl, 2019). In eukaryotes, two major systems are responsible for degrading functional and faulty proteins alike, the ubiquitin-proteasome system (UPS) and the autophagy-lysosome pathway (ALP) (Dikic, 2017; Hipp et al., 2019). The UPS is estimated to be responsible for ∼80% of protein turnover and mediates the degradation of mostly short-lived and damaged proteins (Dikic, 2017; Lee & Goldberg, 1998). The ALP is mainly responsible for the degradation of cellular organelles and long-lived proteins and is known to be a crucial adaptive mechanism in response to cellular stress. (Filomeni, De Zio, & Cecconi, 2015).
Given the key role of protein degradation in cellular processes, disruption of proteasome activity has been implicated in a variety of cancers and neurodegenerative diseases (Leestemaker et al., 2017; Orlowski & Dees, 2002; Orlowski et al., 1998; Trader, Simanski, & Kodadek, 2015; Zheng et al., 2016); therefore, modulation of proteasome activity has gained popularity due to its therapeutic potential. Several tools, from peptide-based fluorescent substrates to activity-based probes (ABPs) (Berkers et al., 2007; Coleman & Trader, 2018; Kisselev & Goldberg, 2005; Zerfas, Coleman, Salazar-Chaparro, Macatangay, & Trader, 2020), have been developed to visualize and monitor the activity of this enzyme; however, the structural complexity of these chemical tools represents a synthetic challenge, limiting their availability. Likewise, the lack of derivatives with different reporter tags can restrict their utility when used concomitantly with other chemical probes.
The protocols described here provide a simple and efficient route to the synthesis of a clickable version of epoxomicin (Basic Protocols 1 and 2), a highly potent and selective proteasome inhibitor, and the coupling of an azide-containing reporter tag (Basic Protocol 3). Additionally, SDS-PAGE and flow cytometry-based assays (Basic Protocol 4 and Alternate Protocol) are described for monitoring proteasome activity and the detection of positive and negative modulation in response to small molecules.
Basic Protocol 1: SOLID-PHASE SYNTHESIS OF CLICKABLE PEPTIDE FRAGMENT (2)
This protocol describes the process to obtain fragment (2), required for the synthesis of an epoxomicin-based probe amenable to click chemistry (Fig. 1). This fragment is synthesized using standard solid-phase conditions following Fmoc-based synthesis, from C - to N -terminus, using a high loading Wang-Thr resin, which proved to be high yielding, even at >1 g scale (Fig. 2). Following synthesis of the Thr-Ile-Ile peptide fragment, a PEG2 linker was attached and subsequent addition of an N -methyl propargyl amine was carried out via bromoacetic acid coupling. After cleavage from the resin, the crude material was precipitated using cold diethyl ether and dried. No purification is required at this stage.
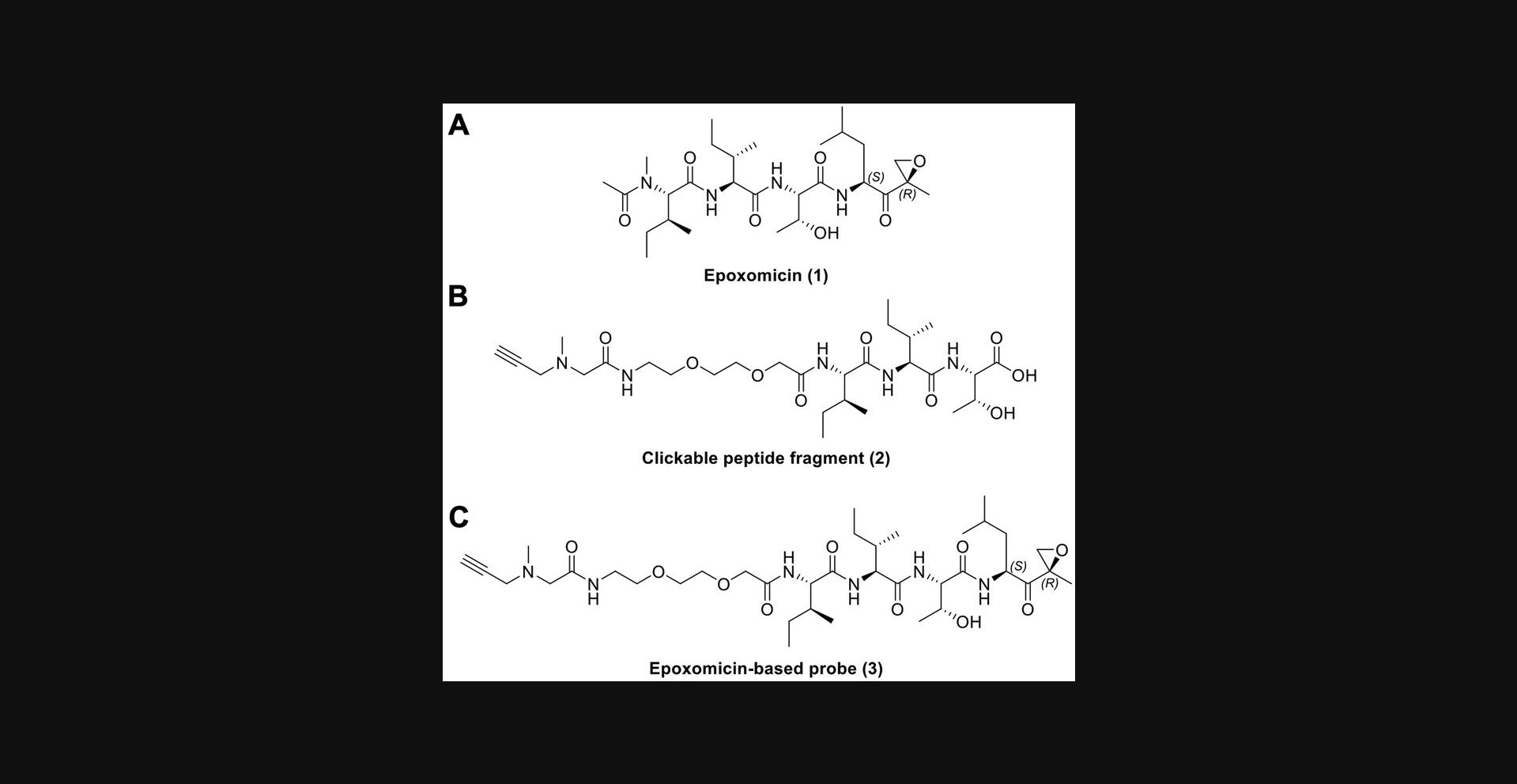
![Details are in the caption following the image (A) Total ion chromatogram (TIC) of the synthesized peptide fragment. While no purification is performed, usually >95% purity is achieved following this protocol. (B) MS spectrum of peak with retention time of 5.8 min. The observed mass (600.4) matches the expected mass of the peptide fragment ([M+H]<sup>+</sup> 600.35). An additional mass observed in the spectrum correlates with the [M+2H]<sup>+</sup> charge state (300.8).](https://static.yanyin.tech/literature_test/cpz1490-fig-0002-m.jpg)
Materials
-
Fmoc-Threonine(tBu)-Wang resin (Fmoc-Thr(tBu)-Wang; Millipore Sigma catalog No. 856017)
-
N- dimethylformamide (DMF)
-
Anhydrous DMF
-
Dichloromethane (DCM)
-
Diethyl ether
-
20% (v/v) piperidine in DMF
-
Fmoc-Isoleucine-OH (Fmoc-Ile-OH; Millipore Sigma catalog No. 852010)
-
Kaiser reagents 1, 2, and 3 (see recipe)
-
Cleavage solution (see recipe)
-
Hexafluorophosphate benzotriazole tetramethyl uronium (HBTU; Fisher catalog No. B16575G)
-
N,N-Diisopropylethylamine (DIPEA)
-
Bromoacetic acid (BAA)
-
N,N -diisopropylcarbodiimide (DIC)
-
N -Methylpropargylamine (Combi-Blocks catalog No. QJ-2154)
-
[2-[2-(Fmoc-amino)ethoxy]ethoxy]acetic acid (PEG2 linker) (Combi-Blocks catalog No. SS-2798)
-
Fritted syringe with caps (Kamush catalog No. PPV006)
-
Room-temperature shaker
-
Scale
-
Vortex
-
Vacuum Manifold (Promega catalog No. A7231)
-
Rotary evaporator
-
Vacuum pump
-
Centrifuge
-
Conical tube
-
Inert gas (argon/nitrogen)
-
Disposable Round-Bottom Rimless Glass Tubes
-
Disposable borosilicate glass Pasteur pipets (9 in.)
-
Heat gun or 95°C heating block/oven
All reactions must be carried out in a ventilated fume hood and wearing the appropriate personal protective equipment (PPE), such as reagent and solvent-suitable gloves and safety glasses.
1.Weigh Fmoc-Thr(tBu)-Wang resin in fritted syringe.
2.Cap the bottom of the syringe and add 2 ml DMF to cover entirely the resin beads.
3.Insert syringe plunger and push far enough to maximize contact between the resin beads and the solvent.
4.Incubate the syringe for 30 to 60 min at room temperature with shaking to allow the resin beads to swell.
5.Remove the plunger and place syringe on manifold to drain the solvent.
6.Cap the bottom of the syringe and add 2 ml of 20% piperidine in DMF to deprotect the Fmoc group.
7.Place syringe plunger back into the syringe and incubate for 30 min at room temperature with shaking. Put syringe on a manifold and drain the solution.
8.Wash resin three times with 2 to 5 ml of DMF and validate complete removal of the Fmoc group by performing a Kaiser test:
-
Add 1 to 2 drops of each of the 3 Kaiser reagents into a small round-bottom rimless glass tube.
-
Using a glass pipette, remove a small amount of resin beads from the syringe and transfer them to the glass tube.
-
Heat the glass tube at ≥95°C for 5 min and check the color of the beads:
A dark blue/purple coloration indicates a positive result (presence of primary amines). Slight to no change in color indicates the absence of primary amines, therefore, a negative result.
If the amount of resin beads is too small to confidently observe, use a white-light microscope to examine them more closely.
If the Kaiser test yields partial or no color change in the resin beads, repeat steps 6–8. If test is positive, proceed.
9.Weigh out 4 equivalents of Fmoc-Ile-OH and 4 equivalents of HBTU. Dissolve in 2 ml DMF then add 8 equivalents of DIPEA to the mixture.
10.Vortex mixture thoroughly and add solution to the resin. Incubate syringe for 1 hr at room temperature.
11.Repeat steps 5–10 using Fmoc-Ile-OH once again. This “double coupling” ensures complete coupling to the resin.
12.Repeat steps 5–10 using PEG2 linker.
13.Repeat steps 3–7.Wash resin with 2 ml anhydrous DMF and perform the Kaiser test.
14.Weigh out enough BAA to make 1 ml of a 2 M solution in dry DMF and prepare 1 ml of 1 M DIC in dry DMF.
15.Mix BAA and DIC solutions and agitate in shaker until a white precipitate is observed.
16.Cap the bottom of the syringe and add the 2 ml mixture of activated BAA. Insert the plunger and incubate at 37°C for 20 min with shaking.
17.Drain solution, wash three times with 2 ml anhydrous DMF and perform the Kaiser test.
18.Prepare 1.5 ml of a 1 M solution of N -methylpropargylamine in anhydrous DMF.
19.Add solution to the resin and incubate at 37°C for 1 hr with shaking.
20.Wash resin three times with 2 ml DMF and three times with DCM. Allow the resin to dry by pulling vacuum through the syringe.
21.Cleave peptide from resin by adding 3 ml of cleavage solution and incubating the syringe at room temperature for 2 hr.
22.Collect the resulting solution in a conical tube and wash beads three times with 3 ml DCM.
23.Carefully place a stream of argon/nitrogen over the top of the solution to evaporate the mixture of solvents until a viscous residue remains.
24.Add prechilled diethyl ether to the crude material and centrifuge at 4500 × g for 10 min.
25.Decant the diethyl ether and vacuum-dry the resulting product until a solid is observed.
26.Store product at −20°C until coupling of the epoxy-ketone fragment is conducted (Basic Protocol 2).
Basic Protocol 2: IN-SOLUTION COUPLING OF EPOXY-KETONE MOIETY TO FRAGMENT (2)
Once the clickable peptidomimetic fragment is synthesized, an in-solution amide linkage is performed after protection of the Thr hydroxyl group using conventional coupling reagents in tetrahydrofuran (THF). The crude material is dried under vacuum and resuspended in methanol (MeOH) for reverse-phase HPLC purification. The final compound is lyophilized overnight and resuspended in dimethylsulfoxide (DMSO) to be used in cell-based assays or stored at −20°C for subsequent click chemistry of a fluorophore of interest.
Materials
-
Tetrahydrofuran (THF)
-
Acetonitrile (ACN), HPLC grade (Fisher catalog No. AA22927K7)
-
Water HPLC grade (Fisher catalog No. AA22934K2)
-
Methanol (MeOH) optima (Fisher catalog No. A456-500)
-
Trifluoroacetic acid (TFA) (Fisher catalog No. AAA1436518)
-
1-Hydroxybenzotriazole hydrate (HOBt) (Sigma-Aldrich catalog No. 54802)
-
Hexafluorophosphate benzotriazole tetramethyl uronium (HBTU)
-
tert -Butyldimethylsilyl chloride (TBSCl)
-
Imidazole
-
(S)-2-Amino-4-methyl-1-((r)-2-methyloxiran-2-yl)pentan-1-one 2,2,2-trifluoroacetate (Combi-Blocks catalog No. QN-6599)
-
DMSO (molecular biology grade) (Fisher catalog No. ICN19481980)
-
8-ml glass vials with caps
-
0.31-inch stir bars
-
Scale
-
Stir plate
-
Lyophilizer
-
HPLC system: Agilent 1200 preparative system with Zorbax Eclipse XDB-C18 column (9.4 × 250 mm, 5-μm particle size) or comparable system
-
LC/MS
-
Vacuum pump
-
Parafilm
-
Glass container with rubber adaptor for lyophilizer
-
95°C oven
-
Inert gas (argon/nitrogen)
-
Rotary evaporator
1.Place a stir bar inside of the glass vial and place them in the oven the day before the experiment is to be conducted (Vial A). Repeat process for another vial and stir bar (Vial B).
2.Weigh out (2) into Vial A and dissolve material in 1 ml anhydrous THF.
3.Flush argon/nitrogen inside vial A and cap it.
4.Weigh out 2 equivalents of TBSCl and 2.2 equivalents of imidazole. Add them to vial A, flush with argon/nitrogen and cap.
5.Place vial A on stir plate and allow the mixture to stir for 18 hr.
6.Weigh 1.05 equivalents of HBTU and 1.05 equivalents of HOBt and add them to vial A. Add 3 equivalents of DIPEA and flush the vial with argon/nitrogen.
7.Place vial A on stir plate and allow the mixture to react for 30 min.
8.Weigh 1.05 equivalents of (S)-2-Amino-4-methyl-1-((r)-2-methyloxiran-2-yl)pentan-1- 2,2,2-trifluoroacetate and add them to oven-dried vial B. Dissolve the mixture in 0.5 ml anhydrous THF then add 3 equivalents of DIPEA.
9.Flush argon/nitrogen inside vial B and cap. Place vial on stir plate and allow the mixture to react for 5 to 10 min.
10.Transfer solution from vial B to vial A and allow the mixture to react at room temperature for 4 to 5 hr.
11.Concentrate crude material in rotary evaporator and redissolve in MeOH.
12.Purify crude solution by reverse-phase HPLC coupled to a UV-Vis detector at 210 nm, using the following mobile phases: (A) 95% Water/5% Acetonitrile/0.5% TFA (B) 95% Acetonitrile/5% Water and 0.5% TFA. Identify the peaks corresponding to the product material by LC/MS (Figure 3)
![Details are in the caption following the image (A) Total ion chromatogram (TIC) of the clickable epoxomicin-based probe (2) showing purity >95% after purification via reverse-phase HPLC. (B) MS spectrum of peak with retention time of 7.2 min. The observed mass (753.4) matches the expected mass of the epoxomicin probe ([M+H]<sup>+</sup> 753.47 m/z). An additional mass observed in the spectrum correlates with the doubly charged state (377.2 m/z).](https://static.yanyin.tech/literature_test/cpz1490-fig-0003-m.jpg)
13.Combine the fractions containing the desired compound and concentrate them in a rotary evaporator to remove ACN and TFA.
14.Freeze the solution at −80°C and lyophilize until a powder is obtained.
Basic Protocol 3: COPPER-CATALYZED CLICK REACTION OF (3) WITH BODIPY-FL AZIDE
While the synthesized alkynyl fragment (2) possesses an intrinsic electrophile and a peptide recognition sequence for the proteasome in order for this probe be useful for imaging and activity analyses, a reporter tag must be incorporated into the structure before or after in cellulo treatment (i.e., bioconjugation). This protocol details the copper-catalyzed ligation of an azide-containing cell permeable fluorophore with fragment (2) using copper sulfate as the copper source and sodium ascorbate as the reducing agent for generation of copper ions in the +1 oxidation state. The crude material from this protocol is purified using the same HPLC method as described above and lyophilized into a powder (Figure 4). Alternative azido fluorophores can be easily coupled as desired using the same protocol described below.
![Details are in the caption following the image (A) Structure of the synthesized epoxomicin-based probe coupled to a BodipyFL fluorophore (3). (B) Total ion chromatogram (TIC) of the synthesized chemical probe. >95% purity is obtained after purification via reverse-phase HPLC. (C) MS spectrum of the peak with retention time of 6.9 min. The observed mass (1127.6) matches the expected mass of the Bodipy clicked epoxomicin probe ([M+H]<sup>+</sup> 1127.65 m/z). An additional mass observed in the spectrum correlates with doubly charged state (377.2 m/z), and [M+Na]<sup>+</sup> adduct (1149.6 m/z).](https://static.yanyin.tech/literature_test/cpz1490-fig-0004-m.jpg)
For information regarding the bioconjugation of alkyne- or azide-containing biomolecules see Current Protocols in Chemical Biology , 3: 153–162, December 2011.
Materials
-
Deionized water (DI water)
-
Fluorophore azide (e.g., Bodipy-FL azide, Lumiprobe catalog No. 11430)
-
Acetonitrile (ACN) HPLC grade
-
Water HPLC grade
-
Methanol (MeOH) optima
-
Trifluoroacetic acid (TFA)
-
Copper sulfate pentahydrate (CuSO4) (Fisher catalog No. AC197730010)
-
Sodium ascorbate
-
Tris(benzyltriazolylmethyl)amine (THPTA) ligand (Optional) (Lumiprobe catalog No. F4050)
-
8-ml glass vials with caps
-
0.31-inch stir bars
-
Scale
-
Heat plate
-
Lyophilizer
-
HPLC system: Agilent 1200 preparative system with Zorbax Eclipse XDB-C18column (9.4×250 mm, 5-μm particle size) or comparable system
-
LC/MS
-
Sonicator
-
Glass container with rubber adaptor for lyophilizer
-
Inert gas (argon/nitrogen)
-
Rotary evaporator
1.Weigh out (2) and place it in glass vial. Dissolve in 0.5 ml of a 1:1 solution of DMSO:H2O and cap vial.
2.Weigh 1 equivalent of a fluorophore that contains an azide (fluorophore selection will depend on what wavelengths are desired to be detected), 0.1 equivalents of copper sulfate pentahydrate, and 1 equivalent of sodium ascorbate and add it to the glass vial.
Reagent | Solvent | Stock concentration (mM)a | Amount to add (µl) |
---|---|---|---|
Azide fluorophore | DMSO | 10 | 100 |
Sodium ascorbate | DI water | 10 | 100 |
Copper sulfate pentahydrate | DI water | 1 | 100 |
- a
These concentrations can be adjusted by multiplying the concentrations in the table by the number of moles needed. Maintain a final volume ≤1 ml.
3.Place the stir bar inside the vial and flush with argon/nitrogen. Degas the mixture for 5 min by placing the vial in the sonicator bath.
4.Flush the vial once again with argon/nitrogen and cap it. Place the vial on a hot plate and allow the mixture to react at 80°C for 1 hr while stirring.
5.Purify crude solution by reverse-phase HPLC coupled to a UV-Vis detector at 210 nm, using the previously described mobile phases. Identify the peaks corresponding to the product material by LC/MS.
6.Combine the fractions containing the desired compound and concentrate them in a rotary evaporator to remove ACN and TFA.
7.Freeze the solution at −80°C and lyophilize until a powder is obtained.
Basic Protocol 4: MONITORING PROTEASOME ACTIVITY BY SDS-PAGE IN HEK-293T CELLS
The products of the previous steps can be used to assay proteasome activity in HEK-293T cells by SDS-PAGE. The model can be used to monitor proteasome activity in the presence and absence of candidate small molecule modulators in discovery activities. Once cells are cultured and plated, the activity-based probes are added, with or without candidate modulators. After incubation, samples are prepared for separation and analysis by SDS-PAGE.
Materials
-
Frozen vial of HEK-293T cells (ATCC® CRL-3216TM).
-
Dulbecco's Modified Eagle's Medium (DMEM) supplemented with 10% Fetal Bovine Serum (FBS) (Fisher catalog No. MT10027CV)
-
M-PERTM Mammalian Protein Extraction Reagent (Fisher, catalog No. PI78501)
-
Halt™ Protease Inhibitor Cocktail, 100× (ThermoFisher catalog No. 78429)
-
4×Laemmli sample buffer
-
2-mercaptoethanol
-
1× phosphate buffered saline (PBS)
-
Coomassie Brilliant Blue stain (see recipe)
-
Coomassie destaining solution (see recipe)
-
Tris·HCl Glycine Running buffer (see recipe)
-
10-well, 50-μl/well gradient gel (4%–20%) Mini-PROTEAN® TGXTM Precast Protein Gel (Bio-Rad catalog No. 4561094)
-
Protein ladder of choice (e.g., Dual Color, Kaleidoscope)
-
Incubator with CO2
-
37°C water bath
-
Upright, bright-light microscope, with 4× and 20× lenses
-
15-ml sterile conical tubes
-
T-75 cell culture flask
-
6-, 12-, or 24-well plate
-
Hemocytometer with glass coverslip
-
1.5-ml Eppendorf tubes
-
0.6-ml Eppendorf tubes
-
NanoDropTM UV-Vis Spectrophotometer or comparable spectrophotometer, BCA assay kit (ThermoFisher catalog No. 23227), or protein quantitation kit of choice
-
Deionized water
-
Heating block
-
Standard tabletop centrifuge
-
Orbital shaker
-
Biomolecular imager suitable for the selected fluorophore (e.g., Sapphire™ RGBNIR imager)
-
Vacuum pump
-
Vertical electrophoresis chamber and compatible power supply
-
Plastic box with lid
-
Optional: Poly-D-lysine hydrobromide (Sigma-Aldrich, catalog No. P6407)
All culture incubations are performed in a sterile humidified 37°C cell culture incubator with 5% CO2.
A sterile laminar flow biosafety cabinet certified for the type of biosafety level should be used for cellular work. Sterilization of the hood is to be carried out by UV light irradiation 10 min prior to starting any work and by spraying down the surface using 70% (v/v) ethanol in Mili-Q water. All items must be wiped with the ethanol solution as well before placing them inside the hood.
All stock vials containing cells should be kept in a liquid nitrogen freezer until use.
1.Retrieve a frozen vial of HEK-293T cells from the freezer and thaw in a water bath at 37°C.
2.After thawing the vial transfer the suspension to a sterile 15-ml conical tube and gradually dilute it to 10 ml using DMEM with 10% FBS.
3.Centrifuge the conical tube at 300 × g for 3 to 5 min to pellet the cells.
4.Carefully vacuum aspirate the medium and resuspend the pellet in 10 ml DMEM with 10% FBS.
5.Transfer solution to a T-75 culture flask and place it in the CO2 incubator.
6.Once 80% to 90% confluency is reached, remove the flask from the incubator and vacuum aspirate the medium.
7.Gently add 10 ml of 1× PBS to the culture flask to wash the cells and then vacuum aspirate the solution.
8.Add 1 ml of 0.25% trypsin-EDTA solution and return the flask to the incubator for 3 to 5 min.
9.Visually inspect the culture flask to ensure complete detachment of the cells and then proceed to dilute the suspension with 9 ml DMEM with 10% FBS.
10.Transfer the cell suspension to a 15-ml conical tube and centrifuge at 300 × g for 5 min to pellet the cells.
11.Vacuum aspirate the supernatant and resuspend the cell pellet in 10 ml DMEM with 10% FBS by pipetting up and down.
12.Take 30 to 50 µl of the cell suspension and transfer to a 0.6-ml tube.
13.Place the glass coverslip on top of the hemocytometer and gently fill the chambers underneath, on both sides of the apparatus.
14.Place the hemocytometer under the bright-light microscope and count cells to determine the amount of cells per ml.
15.Prepare stock cell suspension based on the plate and number of samples to be used.
16.Aliquot the stock cell suspension into the plate wells and place the plate back in the CO2 incubator.
17.Allow the plate to incubate until 90% to 95% confluency is achieved.
18.Remove the plate from the incubator and dose each well with the synthesized activity-based probe. Place the plate back in the CO2 incubator for 1 hr.
19.Remove the plate from the incubator and vacuum aspirate the medium from each well. Add M-PERTM lysis buffer with 100X HaltTM protease cocktail to each well and agitate the plate on an orbital shaker for 10 min.
-
Dissolve 5 mg of poly-D-lysine hydrobromide in 50 ml of autoclaved Mili-Q water (100 µg/ml).
-
Filter sterilize the resulting solution. Store excess at 4°C.
-
Add solution to each well. Volume is dependent of the plate used. Use values shown in Table2.
-
Let the plate sit for 5 to 30 min at room temperature.
-
Vacuum aspirate the solution from each well and rinse the surface twice using the same volume of PBS.
Plate | Stock suspension (cells/ml) | Volume per well (µl) | Cells per well |
---|---|---|---|
24-well | 40 × 104 | 500 | 200,000 |
12-well | 50 × 104 | 1000 | 500,000 |
6-well | 1 × 106 | 2000 | 2,000,000 |
20.Collect cell lysate in Eppendorf tubes and centrifuge at 14,000 × g for 10 min and 4°C to pellet cell debris.
21.Transfer the supernatant to fresh new tubes and measure the protein concentration following method of choice.
22.Based on the measured protein concentration, aliquot the samples in such a way that the total protein is equal amongst them.
23.Prepare loading buffer using 4× Laemmli sample buffer according to manufacturer instructions and add the necessary volume to each sample.
24.Heat samples in 95°C heat block for 5 to 10 min.
25.Briefly centrifuge the samples and load them in a 10-well, 50-μl/well gradient gel with compatible ladder.
26.Run the gel at 150 V and constant current for 55 min using 1× SDS Tris-HCl glycine running buffer and an electrophoresis cell with compatible power supply.
27.Remove gel cassette from the electrophoresis cell and place the gel in a plastic box. Rinse three times with DI water.
28.Image gel using biomolecular imager suitable for the selected fluorophore.
29.Coomassie stain the gel for 30 to 60 min to ensure equal protein loading, and destain for 4 to 24 hr, or until the background is clear. See example in Figure 5.
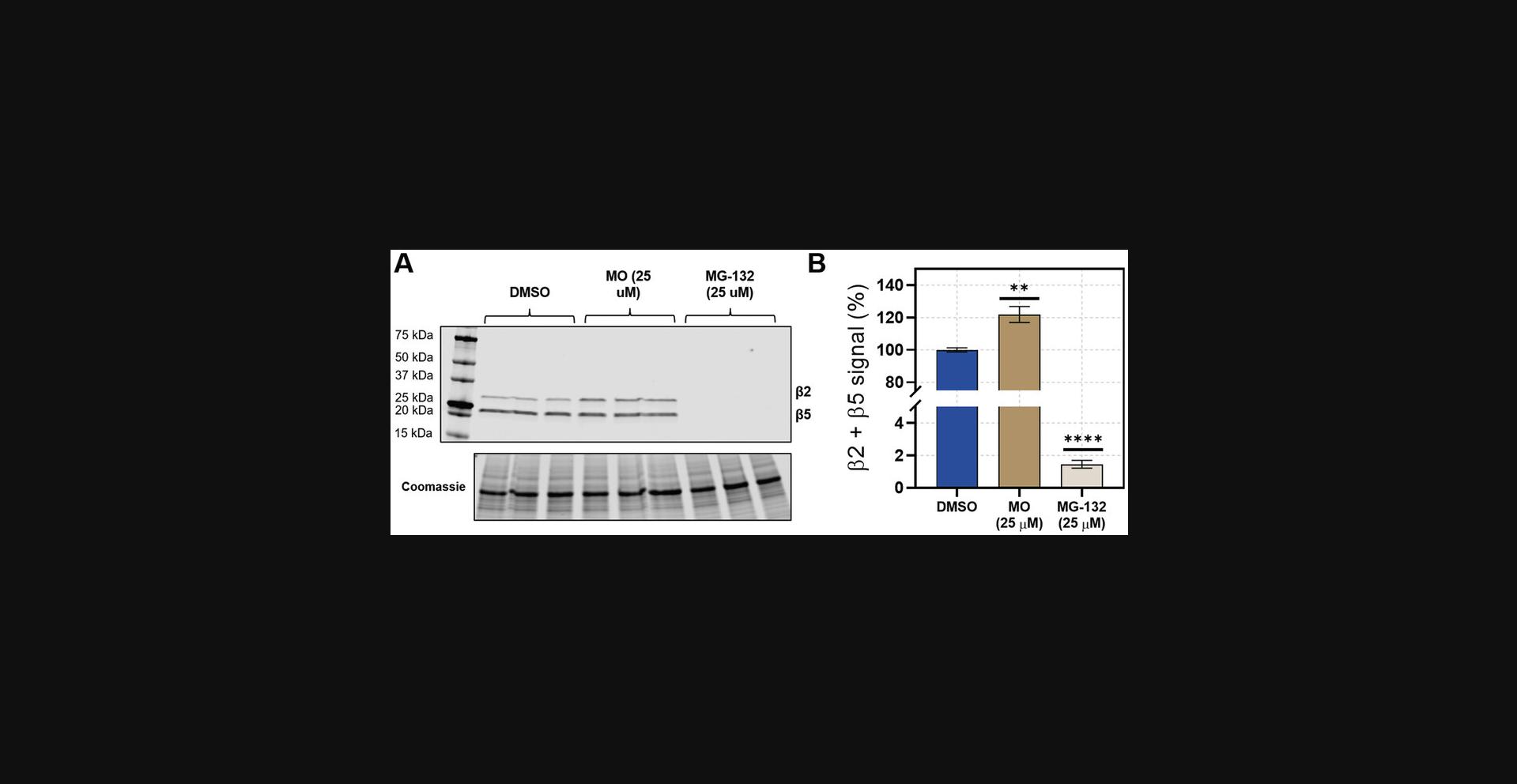
Alternate Protocol: MONITORING PROTEASOME ACTIVITY BY FLOW CYTOMETRY IN HEK-293T CELLS
Analysis of proteasome activity can be performed by other techniques such as confocal microscopy and flow cytometry. This Alternate Protocol is analogous to Basic Protocol 4 with the main difference being the sample preparation for the proteasome activity analysis by flow cytometry instead of SDS-PAGE. Once cells are cultured and plated, the live cell samples are rinsed and resuspended in PBS. A viability dye is used to discriminate live versus dead populations and fluorescence is measured by flow cytometry. Gating is performed sequentially by forward scatter versus side scatter to differentiate cells from debris based on size and granularity. Exclusion of the dead cell population is done based on the viability dye used.
Materials
-
Frozen vial of HEK-293T cells (ATCC® CRL-3216TM)
-
Dulbecco's Modified Eagle's Medium (DMEM) supplemented with 10% Fetal Bovine Serum (FBS)
-
1× phosphate buffered saline (PBS)
-
Incubator with CO2
-
Upright, bright-light microscope, with 4× and 20× lenses
-
15-ml sterile conical tubes
-
T-75 cell culture flask
-
1.5-ml Eppendorf tubes
-
TO-PRO™3 Ready Flow™ viability dye (ThermoFisher catalog No. R37170)
-
Ice
-
Ice bucket
-
Cell scraper
-
Standard tabletop centrifuge
-
BD Accuri™ C6 Plus Flow Cytometer or compatible flow cytometer with fluorophore of choice
1.Incubation of cells as well as plating is to be carried out following the procedure described in Basic Protocol 4 (steps 1–17)
2.Remove the plate from the incubator and dose wells with the synthesized activity-based probe. Place the plate back in the CO2 incubator for 1 hr.
Controls | Fluorescent probe | Viability dye |
---|---|---|
Unstained | No | No |
No probe | No | Yes |
No viability dye | Yes | No |
3.Remove the plate from the incubator and place on ice. Carefully vacuum aspirate the DMEM from each well.
4.Add 200 µl cold PBS to each well and gently dislodge the cells from the bottom of the plate using a cell scraper.
5.Collect the cell suspensions in a fresh tube and centrifuge at 4°C for 3 min at 300 × g.
6.Discard supernatant and resuspend in 200 µl of cold PBS. Centrifuge again as in Step 5.
7.Remove supernatant and resuspend in another 200 µl of cold PBS. Add 20 µl of viability dye to all samples excepting the unstained and no viability dye controls. Incubate samples for 20 min at room temperature.
8.Proceed with flow cytometry analysis by recording 50,000 events per sample. See example in Figure 6.
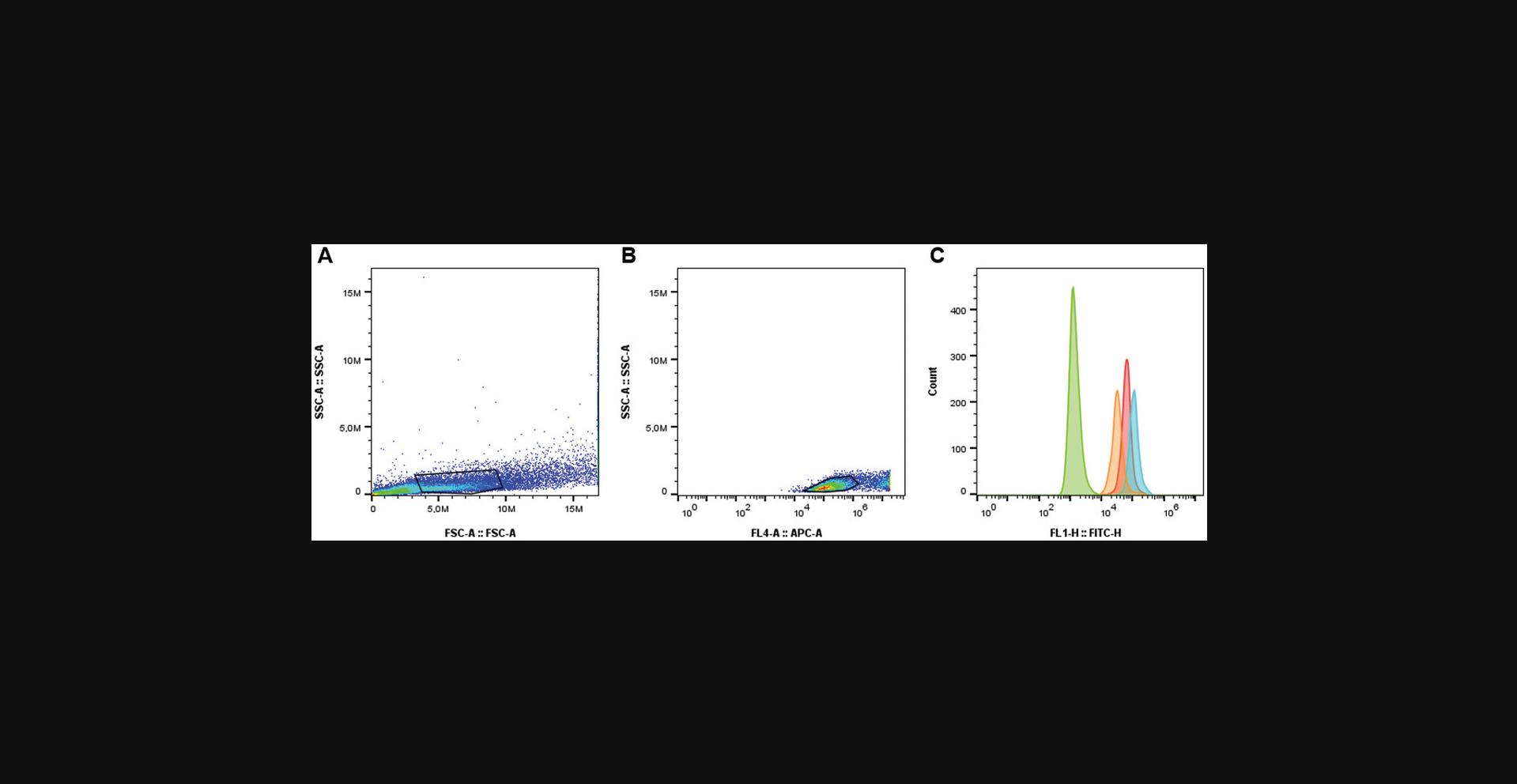
REAGENTS AND SOLUTIONS
D.I. Water should be used for all preparations, unless otherwise noted.
Kaiser reagents
- Reagent 1: 0.05 g/ml ninhydrin in ethanol (EtOH)
- Reagent 2: 4 g/ml phenol in EtOH
- Reagent 3: 0.2 mM KCN (aqueous) in pyridine
- May be stored up to 6 months at room temperature.
Cleavage solution
- 95% (v/v) TFA
- 2.5% (v/v) DCM
- 2.5% (v/v) Triisopropylsilane (TIPS)
- Use immediately after preparation.
Gel running buffer
- 25 mM Tris
- 192 mM glycine
- Sodium dodecyl sulfate (SDS) 0.1% (w/v)
- pH 8.3, adjust with 1 M HCl or 1 M NaOH
- May be stored up to 2 years refrigerated.
Coomassie staining solution
- 3 g/L Coomassie brilliant blue R-250
- 45% (v/v) ml MeOH
- 45% (v/v) DI water
- 10% (v/v) glacial acetic acid
- Dissolve Coomassie powder in MeOH and mix with DI water and acetic acid. Filter before use.
- May be stored up to 6 months at room temperature.
Coomassie destaining solution
- 50% (v/v) ml MeOH
- 40% (v/v) DI water
- 10% (v/v) glacial acetic acid
- May be reused. Store at room temperature in a well-ventilated space.
COMMENTARY
Background Information
The proteasome is a highly conserved multisubunit complex responsible for the degradation of intracellular proteins in eukaryotic organisms (Dikic, 2017; Lee & Goldberg, 1998). It is essential for ensuring adequate protein levels by the controlled cleavage of functional proteins, as well as misfolded and oxidatively damaged species (Dikic, 2017). Given the pivotal role that such task entails, the proteasome regulates a myriad of cellular processes, including cell cycle progression and immune response (Kito et al., 2020; Zerfas, Maresh, & Trader, 2020).
Dysregulation of this machinery has been implicated in cancer and neurodegenerative diseases. Given the uncontrolled proliferation of cancerous cells, these abnormal cells rely on a robust proteasome system to prevent cell death; therefore, proteasome inhibitors can be valuable tools for their ability to induce apoptosis via different mechanisms (Almond & Cohen, 2002). On the other hand, a decline in proteasome function has been associated with age-related diseases (Hipp et al., 2019; Huang & Figueiredo-Pereira, 2010). Thus, the use small molecule activators of the various isoforms of the proteasome represent an alternative approach to treatment (Leestemaker et al., 2017).
Several fluorescent tools have been developed to monitor proteasome activity (Berkers et al., 2007; Capobianco, Lerner, & Goldman, 1992; Kisselev & Goldberg, 2005; Zerfas et al., 2020), but their synthesis can be challenging, and derivatives with different fluorophores and reporter tags are generally not commercially available. Here we describe an efficient synthesis method to obtain a clickable version of epoxomicin (Basic Protocols 1 and 2), a potent proteasome inhibitor, and the coupling of an azide-containing reporter tag via copper-catalyzed cycloaddition (Basic Protocol 3). Additionally, we describe their use for proteasome activity analysis by SDS-PAGE and flow cytometry (Basic Protocol 4 and Alternate Protocol).
Critical Parameters
Anhydrous solvents
The coupling of the epoxide fragment to the peptide backbone represents the key step towards the synthesis of the final molecule. Given the electrophilic nature of the 3-membered ring, the presence of moisture in the solvents can increase the amount of side products.
Purity of activity-based probe
The selectivity and potency of the epoxomicin-based probe lies on the peptide backbone of the molecule; therefore, impurities with different peptide sequences or stereochemistry can heavily impact these aspects. The purity of the final molecule should be ≥95% as determined by HPLC or LC/MS.
Washing live cells with PBS
In order to obtain accurate and reproducible results of proteasome activity by flow cytometry, live cells should be washed thoroughly to remove excess unreacted probe.
Troubleshooting
See Table 4 for problems that might be encountered, possible causes, and potential solutions.
Problem | Possible cause | Solution |
---|---|---|
Little to no labeling of beta subunits | Cell impermeable fluorophore |
Confirm permeability of the synthesized probe by confocal microscopy. Couple a different fluorophore to the alkyne-based epoxomicin core |
Low concentration of chemical probe | Increase the concentration of the chemical probe or incubate for a longer time. | |
Low protein concentration | Insufficient incubation time | Increase the incubation time. Optimal protein concentrations are achieved between 24 and 72 hr of incubation after plating. |
Poor yield | Non-anhydrous solvents |
Freshly distill solvents before use. Use new bottles and store them properly by purging with an inert gas, taping the pierced septa, and parafilm around the bottle cap. |
Low reaction time | The quality of the reagents might decrease over time; therefore, increasing the reaction time or the number of equivalents used can increase the reaction yields. | |
Little to no change in fluorescence upon treatment with proteasome modulator | Not a modulator of the proteasome isoforms | Validate the ability of the compound to modulate the activity of the proteasome using purified proteasome (20S or 26S). |
Insufficient incubation time | Increase the reaction time. | |
Low potency | Increase the concentration of the molecule if the cell viability is not compromised. | |
Low dynamic range of the synthesized probe |
If positive and negative controls are included in the experimental design, and they fail to elicit a change in labeling compared to the basal level, synthesize a new probe including a longer linker chain. A bigger molecule represents a more challenging substrate to the proteasome isoforms; therefore, the labeling rate will be lower, and the difference between an activated/inhibited proteasome versus a non-modulated proteasome will be more apparent. |
- Possible causes and their corresponding potential solutions are included as well.
Understanding Results
It is expected to obtain >80% yield for the synthesis of the peptide fragment (Basic Protocol 1) when conducting the reaction at 1 g scale or less. The amide coupling performed to obtain the alkyne-based epoxomicin core (Basic Protocol 2) is expected to yield 50% to 70% of the target molecule. The most common side products are obtained as a result of the epoxide opening. Coupling an azide-containing fluorophore to fragment (3) through click chemistry is expected to yield between 60% and 95% of the clicked product, with the purification process being critical to the amount obtained. The reaction typically proceeds without major side products, unless thermolabile or moisture-sensitive reagents are used.
In SDS-PAGE (Basic Protocol 4) and flow cytometry (Alternate Protocol) assays, a change in fluorescence is expected between untreated cells and cells incubated with the probe; likewise, when a proteasome inhibitor or stimulator is included, a shift in fluorescence in the channel corresponding to the fluorophore should be observed.
Time Considerations
Synthesis of fragment (2) is expected to take ∼1 day with overnight concentration under high vacuum to remove residual moisture. Synthesis of fragment (3) followed by HPLC purification is expected to take 1 to 3 days depending on the reaction scale and the HPLC system used. Cell culture can take between 7 and 10 days depending on the concentration of cells in the frozen vial, the incubation time after plating, and the incubation time after dosing the small molecule modulator. Proteasome activity analysis by SDS-PAGE followed by in-gel fluorescence scanning is expected to take ∼5 to 8 hr depending on the number of samples and the gel format used. Analysis by flow cytometry is expected to take between 2 and 5 hr.
Acknowledgments
This work was supported through a start-up package from Purdue University College of Pharmacy, the Purdue University Center for Cancer Research (NIH grant P30CA023168), and a grant from the NIH-NIGMS (R21 GM131206).
Author Contributions
Andres F. Salazar-Chaparro : Formal analysis, Investigation, Methodology, Original draft, review, and editing; Saayak Halder : Investigation, Review and editing; Darci J. Trader : Project administration, Review and editing.
Conflict of Interest
Prof. Trader is a shareholder and consultant for Booster Therapeutics, GmbH. Other authors declare no conflict of interest.
Open Research
Data Availability Statement
The data that support the findings of this study are available via https://doi.org/10.1002/cbic.202100710.
Literature Cited
- Almond, J. B., & Cohen, G. M. (2002). The proteasome: A novel target for cancer chemotherapy. Leukemia , 16(4), 433–443. doi: 10.1038/sj.leu.2402417
- Berkers, C. R., van Leeuwen, F. W. B., Groothuis, T. A., Peperzak, V., van Tilburg, E. W., Borst, J., … Ovaa, H. (2007). Profiling proteasome activity in tissue with fluorescent probes. Molecular Pharmaceutics , 4(5), 739–748. doi: 10.1021/mp0700256
- Capobianco, J. O., Lerner, C. G., & Goldman, R. C. (1992). Application of a fluorogenic substrate in the assay of proteolytic activity and in the discovery of a potent inhibitor of Candida albicans aspartic proteinase. Analytical Biochemistry , 204(1), 96–102. doi: 10.1016/0003-2697(92)90145-W
- Coleman, R. A., & Trader, D. J. (2018). Development and application of a sensitive peptide reporter to discover 20S proteasome stimulators. ACS Combinatorial Science , 20(5), 269–276. doi: 10.1021/acscombsci.7b00193
- Dikic, I. (2017). Proteasomal and autophagic degradation systems. Annual Review of Biochemistry , 86, 193–224. doi: 10.1146/annurev-biochem-061516-044908
- Filomeni, G., De Zio, D., & Cecconi, F. (2015). Oxidative stress and autophagy: The clash between damage and metabolic needs. Cell Death & Differentiation, 22(3), 377–388. doi: 10.1038/cdd.2014.150
- Hipp, M. S., Kasturi, P., & Hartl, F. U. (2019). The proteostasis network and its decline in ageing. Nature Reviews Molecular Cell Biology , 20(7), 421–435. doi: 10.1038/s41580-019-0101-y
- Huang, Q., & Figueiredo-Pereira, M. E. (2010). Ubiquitin/proteasome pathway impairment in neurodegeneration: Therapeutic implications. Apoptosis: An International Journal on Programmed Cell Death , 15(11), 1292–1311. doi: 10.1007/s10495-010-0466-z
- Kisselev, A. F., & Goldberg, A. L. (2005). Monitoring activity and inhibition of 26S proteasomes with fluorogenic peptide substrates. Methods in Enzymology , 398, 364–378. doi: 10.1016/S0076-6879(05)98030-0
- Kito, Y., Matsumoto, M., Hatano, A., Takami, T., Oshikawa, K., Matsumoto, A., & Nakayama, K. I. (2020). Cell cycle-dependent localization of the proteasome to chromatin. Scientific Reports , 10(1), 5801. doi: 10.1038/s41598-020-62697-2
- Lee, D. H., & Goldberg, A. L. (1998). Proteasome inhibitors: Valuable new tools for cell biologists. Trends in Cell Biology , 8(10), 397–403. doi: 10.1016/S0962-8924(98)01346-4
- Leestemaker, Y., de Jong, A., Witting, K. F., Penning, R., Schuurman, K., Rodenko, B., … Ovaa, H. (2017). Proteasome activation by small molecules. Cell Chemical Biology , 24(6), 725–736.e7. doi: 10.1016/j.chembiol.2017.05.010
- Orlowski, R. Z., & Dees, E. C. (2002). The role of the ubiquitination-proteasome pathway in breast cancer: Applying drugs that affect the ubiquitin-proteasome pathway to the therapy of breast cancer. Breast Cancer Research , 5(1), 1. doi: 10.1186/bcr460
- Orlowski, R. Z., Eswara, J. R., Lafond-Walker, A., Grever, M. R., Orlowski, M., & Dang, C. V. (1998). Tumor growth inhibition induced in a murine model of human Burkitt's lymphoma by a proteasome inhibitor. Cancer Research , 58(19), 4342–4348.
- Trader, D. J., Simanski, S., & Kodadek, T. (2015). A reversible and highly selective inhibitor of the proteasomal ubiquitin receptor Rpn13 is toxic to multiple myeloma cells. Journal of the American Chemical Society , 137(19), 6312–6319. doi: 10.1021/jacs.5b02069
- Zerfas, B. L., Coleman, R. A., Salazar-Chaparro, A. F., Macatangay, N. J., & Trader, D. J. (2020). Fluorescent probes with unnatural amino acids to monitor proteasome activity in real-time. ACS Chemical Biology , 9(15), 2588–2596. doi: 10.1021/acschembio.0c00634
- Zerfas, B. L., Maresh, M. E., & Trader, D. J. (2020). The immunoproteasome: An emerging target in cancer and autoimmune and neurological disorders. Journal of Medicinal Chemistry , 63(5), 1841–1858. doi: 10.1021/acs.jmedchem.9b01226
- Zheng, Q., Huang, T., Zhang, L., Zhou, Y., Luo, H., Xu, H., & Wang, X. (2016). Dysregulation of ubiquitin-proteasome system in neurodegenerative diseases. Frontiers in Aging Neuroscience , 8, 303. doi: 10.3389/fnagi.2016.00303
Citing Literature
Number of times cited according to CrossRef: 2
- Phetole Mangena, Cell Mutagenic Autopolyploidy Enhances Salinity Stress Tolerance in Leguminous Crops, Cells, 10.3390/cells12162082, 12 , 16, (2082), (2023).
- Samantha Nelson, Timothy J. Harris, Christine S. Muli, Marianne E. Maresh, Braden Baker, Chloe Smith, Chris Neumann, Darci J. Trader, Elizabeth I. Parkinson, Discovery and Development of Cyclic Peptide Proteasome Stimulators, ChemBioChem, 10.1002/cbic.202300671, 25 , 3, (2023).