Rearing and Experimental Uses of Daphnia: Controlling Animal Nutrition and Assessing Lifespan and Life-History Traits
Paul C. Frost, Paul C. Frost, Samatha L. Caudle, Samatha L. Caudle, Sen Han, Sen Han, Jocelyn M. J. O'Brien, Jocelyn M. J. O'Brien, Stephanie W. Tobin, Stephanie W. Tobin
Abstract
Caloric restriction has been found to extend the lifespan of many organisms including mammals and other vertebrates. With lifespans exceeding months to years, age-related experiments involving fish and mammals can be overtly costly, both in terms of time and funding. The freshwater crustacean, Daphnia , has a relatively short lifespan (∼50 to 100 days), which makes it a cost-effective alternative animal model for longevity and aging studies. Besides age-specific mortality, there are a suite of physiological responses connected to “healthspan” that can be tracked as these animals age including growth, reproduction, and metabolic rates. These responses can be complemented by assessment of molecular and cellular processes connected to aging and health. Lifespan and metabolism of this model organism is responsive to long studied modulators of aging, such as rearing temperature and nutritional manipulation, but also pharmacological agents that target aging, e.g., rapamycin, which adds to its usefulness as a model organism. Here we describe how to culture Daphnia for aging experiments including maintaining laboratory populations of Daphnia mothers, growing algal food, and manipulating nutrition of these animals. In addition, we provide methods for tracking common physiological and longevity responses of Daphnia. This protocol provides researchers planning to use this model organism with methods to establish and maintain Daphnia populations and to standardize their experimental approaches. © 2024 The Authors. Current Protocols published by Wiley Periodicals LLC.
Basic Protocol 1 : Culturing algae for Daphnia food
Basic Protocol 2 : General methods for culturing Daphnia
Basic Protocol 3 : Standardizing and controlling nutrition for experimental Daphnia
Basic Protocol 4 : Monitoring Daphnia lifespan
Basic Protocol 5 : Evaluating Daphnia health: Heart rate and respiration, body mass and growth rates, and reproduction
INTRODUCTION
Aging and its relationship to cellular senescence has been assessed in numerous model organisms that vary in lifespan on a scale of days, weeks and even years, including yeast, Drosophila , C. elegans , and mice (Gems & Partridge, 2013; McKay et al., 2022). One of the main benefits of model organisms with shorter lifespans is being able to manipulate and study aging processes and their cellular/molecular controls across relatively short time periods (e.g., days to weeks). While extrapolation of results from evolutionarily distant model organisms to humans has its limits, the use of model organisms in aging and longevity research is warranted as the molecular changes that underpin aging are generally conserved across animal taxa (Cohen, 2018; Guarente & Keyon, 2000). For example, some aspects of cellular aging, such as dysregulated nutrient sensing, telomere shortening, DNA and mitochondrial damage, and changes in epigenetic patterns, are conserved across different model organisms (López-Otín et al., 2023).
One organism with a relatively short lifespan and fast generation times that is increasingly being used for aging studies is the freshwater crustacean, Daphnia. Daphnia are aquatic herbivores that are found in water bodies around the world (Ebert, 2005). They are an ecologically important organism and have been widely studied with respect to their phenotypic plasticity and eco-evolutionary dynamics (Ebert, 2022; Miner et al., 2012). They have also been used extensively in ecotoxicology, partly due to the relatively low cost and effort needed to maintain laboratory cultures, extensive work on their genetics, and their ability to reproduce parthenogenetically (Tkaczyk et al., 2021). Daphnia have transparent bodies that contain tissues and organ systems (e.g., a digestive tract and circulatory system) with parallels to other invertebrate and vertebrate animals. Daphnia primarily feed on various types of planktonic algae, which can also be easily cultured in the laboratory. In addition, these crustaceans are relatively small (length of ∼5 mm or less), which allows for many animals to be housed in small volumes of water and minimal laboratory space. This also makes it relatively straightforward to manipulate drug concentrations, toxicological stress, nutrition, and/or environmental stress in highly replicated experiments. As an emerging model organism, Daphnia is well-suited for the study of longevity and aging.
The life cycle of Daphnia can involve a mix of asexual and sexual reproductive strategies (Ebert, 2005). After birth, Daphnia development proceeds through a juvenile stage that lasts ∼3 to 4 days before they become reproductively mature, which is evident by the production of eggs in their brood pouch. After this, animals generally molt every 2 to 3 days with new clutches released during each molt. Under non-stressful conditions, Daphnia reproduce clonally with offspring genetically identical (or very similar) to their mother. Environmental cues associated with changes in temperature, reduced food abundance, or high animal densities can result in Daphnia sexually reproducing and/or producing resting eggs called ephippia (Ebert, 2005). In a controlled laboratory environment, Daphnia can live >100 days (Fig. 1), but this lifespan varies widely with rearing temperature and other environmental conditions.
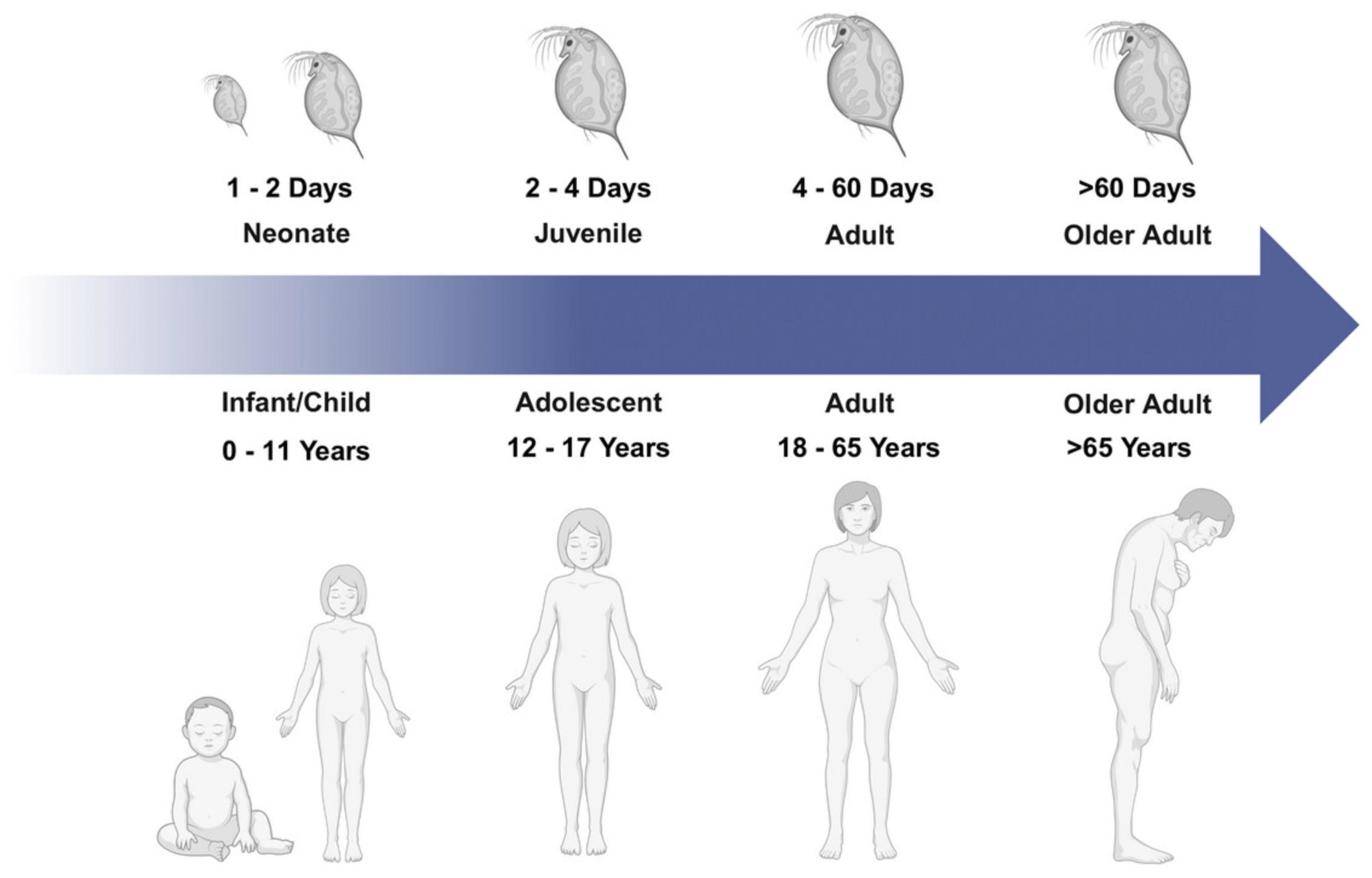
There is a long history of studying the lifespan of Daphnia that goes back nearly 100 years. The earliest studies of Daphnia aging were among the first to document the effects of temperature on an animal's lifespan (MacArthur & Baillie, 1929a, 1929b). These studies and subsequent work on Daphnia ’s lifespan used newborn animals produced synchronously and subsequently tracked their survival under controlled conditions. This approach allows for isolation and study of specific environmental effects on animal metabolism and life-history. Beyond life-history traits (e.g., age to first reproduction, brood size, and survival), there are numerous physiological processes (e.g., growth, respiration, and feeding rates) and body condition metrics (e.g., lipid content and composition) that can be tracked as well during the aging process. With increasing knowledge of the Daphnia genome, there are ever-increasing opportunities to link physiological processes and life-history traits in this animal to molecular and biochemical mechanisms that internally regulate growth, reproduction, and maintenance (e.g., Wagner et al., 2013). These complementary sets of response variables in Daphnia and other metazoans represent a fruitful arena for advancing our understanding of the aging process and its biological controls.
In this article, we provide methods used to culture the model organism, Daphnia , for the study of longevity and aging and with a focus on standardizing its nutrition. We first describe in Basic Protocols 1 to 3 how to culture Daphnia , including the maintenance of populations of mother Daphnia used to generate offspring used in experiments and the preparation of its green algal food. In Basic Protocols 4 and 5, we describe how to quantitatively track Daphnia lifespan and various health responses, such as heart rate, respiration rate, mass and growth rates, and reproduction. An outline of the steps involved in these protocols is shown in Figure 2.
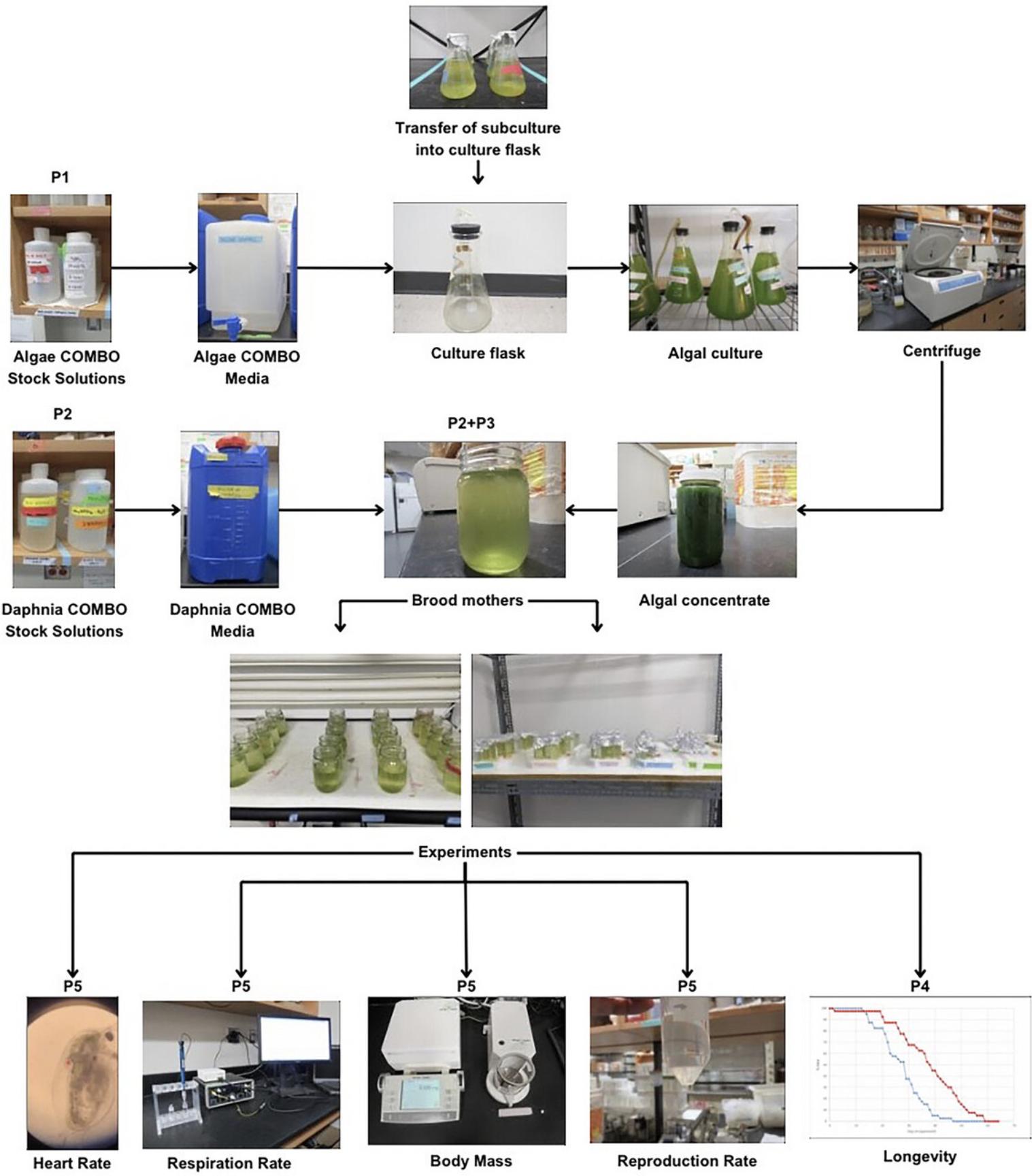
NOTE : All protocols involving animals must be reviewed and approved by the appropriate Animal Care and Use Committee and must follow regulations for the care and use of laboratory animals.
Basic Protocol 1: CULTURING ALGAE FOR Daphnia FOOD
This protocol describes the procedures for establishing and maintaining algal cultures for the purpose of providing food to Daphnia. There are numerous algal species that could be used as food to sustain laboratory populations of Daphnia (Table 1). The choice of an algal food should be considered carefully as different algal and cyanobacterial species vary widely in their inherent nutritional value and digestibility and can dramatically affect the health of your animal cultures. Here we describe how to grow the green alga, Tetradesmus (synonymous with Scenedesmu s), as it is commonly used as a food source for Daphnia , it is easy to grow and is known to be relatively nutritionally complete for Daphnia.
Genus | Taxonomic group | Medium | Source |
---|---|---|---|
Chlamydomonas | Chlorophyta | Table 2 in reference | Spaak and Hoekstra (1995) |
Chlorella | Chlorophyta | Bold's basal | Alva-Martínez et al. (2004) |
Cryptomonas | Cryptomonad | WC medium | Nova et al. (2019) |
Cyclotella | Diatom | WC medium | Müller-Navarra (1995) |
Mallomonas | Chrysophyte | Modified WC medium | Taipale et al. (2019) |
Rhodomonas | Cryptomonad | Modified WC medium | Taipale et al. (2019) |
Stephanodiscus | Diatom | Modified WC medium | Carotenuto et al. (2005) |
Tetradesmus | Chlorophyta | Modified COMBO | This article |
- a
Note that the nutritional quality of these food sources varies greatly due to differences in ingestibility, digestibility, and fatty acid content. While each of these algae can and have been used as a food source for Daphnia, the ease of culturing can differ greatly with some taxa being especially difficult to culture for longer time periods and under semi-sterile conditions. As such, carefully consider these differences when choosing an alga to ensure the laboratory Daphnia populations will have access to enough high-quality food. Detailed Protocols are provided here for the bolded alga, Tetradesmus, which is easy to grow and relatively nutritional replete for Daphnia. Also, details to make medium referenced in the table are found in the provided literature citations.
Materials
- H2O, deionized
- Algae COMBO stock solutions (see Table 2 for instructions on making stock solutions) made with:
- CaCl2·2H2O (Sigma-Aldrich, cat. no. C7902)
- MgSO4·7H2O (Westlab, cat. no. 225-0546)
- NaHCO3 (Sigma Aldrich, cat. no. S6014)
- Na2SiO3·5 H2O (Sigma-Aldrich, cat. no. 71746)
- H3BO3 (Fisher Scientific, cat. no. A74-500)
- Na2HPO4·H2O (Caldedon, cat. no. 8120-1)
- (NH4)2SO4 (Omnipur, cat. no. 2150)
- Na2EDTA (Sigma-Aldrich, cat. no. E5134)
- FeCl3·6H2O (ThermoFisher, cat. no. 217091000)
- MnSO4·H2O (ThermoFisher, cat. no. A17615.36)
- ZnSO4·7H2O (Sigma-Aldrich, cat. no. Z4750)
- Na2MoO4·2H2O (ACROS, cat. no. 206375000)
- CoCl2·6H2O (Sigma Aldrich, cat. no. 255599)
- CuSO4·5H2O (ThermoFisher, cat. no. 197730010)
- Vitamin B12 (Sigma Aldrich, cat. no. V6629)
- Biotin (Sigma Aldrich, cat. no. B4639)
- Thiamine (Sigma Aldrich, cat. no. T1270)
- 0.1 M HCl (Current Protocols, 2006)
- 0.1 M NaOH (Current Protocols, 2006)
- Algal culture of Tetradesmus obliquus.
Pure cultures can be purchased from phycological culture centers, e.g., Canadian Phycological Culture Centre (https://uwaterloo.ca/canadian-phycological-culture-centre/).
- 20-L plastic carboys (Fisher Scientific, cat. no. 02-963BB or equivalent)
- Micropipettes (Fisher Scientific, cat. no. 14-559-433)
- pH meter
- 125-ml Erlenmeyer flasks for subcultures (Fisher Scientific, cat. no. 10-040D)
- Aluminum foil
- Autoclave
- Fume hood
- Broad spectrum growth lamps (e.g., Sansi PAR30 24W LED Grow Light Bulb)
- 2- to 4-L Erlenmeyer flasks (Fisher Scientific, cat. nos. 10-040M and 10-040P)
- Rubber stoppers (Fisher Scientific, cat. no. 14-130P)
- GAquarium air pump (e.g., Tetra Whisper 10)
- Centrifuge
- 50-ml plastic graduated cylinder (Fisher Scientific, cat. no. 8-550D)
- 1-L plastic bottles (Fisher Scientific, cat. no. 02-896F)
Compound | Stock (g/L) | Final medium (mg/L) | Final medium (μmol/L) | Elements | Elements (mg/L) | Elements (μmol/L) | Volume to add to medium (ml/L) |
---|---|---|---|---|---|---|---|
Seven major compound stocks | |||||||
CaCl2·2H2O (b) | 36.76 | 73.52 | 500 | Ca | 20.0 | 500 | 2 |
Cl | 35.4 | 1000 | |||||
MgSO4·7H2O (b) | 36.97 | 49.29 | 200 | Mg | 4.86 | 200 | 1.33 |
S | 6.39 | 200 | |||||
NaHCO3 (b) | 75.00 | 150 | 1786 | Na | 41.1 | 1786 | 2 |
C | 21.4 | 1786 | |||||
Na2SiO3·5 H2O (b) | 21.21 | 21.21 | 100 | Na | 4.598 | 200 | 1 |
Si | 2.808 | 100 | |||||
H3BO3 (b) | 24.00 | 2.057 | 34.3 | B | 0.360 | 33.3 | 0.086 |
Na2HPO4·H2O | 11.00 | 5.50 | 34.4 | Na | 1.58 | 68.8 | 0.5 |
P | 1.06 | 34.4 | |||||
(NH4)2SO4 | 69.38 | 69.38 | 525 | N | 14.7 | 1050 | 1 |
S | 16.8 | 525 | |||||
Sterner modified algal trace elements (StATE)b | |||||||
Na2EDTA | 8.000 | 8.000 | 23.65 | Na | 1.09 | 47.5 | 1 |
EDTA | 6.95 | 23.8 | |||||
FeCl3·6H2O | 3.380 | 3.380 | 12.5 | Fe | 0.70 | 12.5 | |
Cl | 1.33 | 37.5 | |||||
MnSO4·H2O | 60.8 | 0.608 | 3.60 | Mn | 0.198 | 3.60 | |
S | 0.115 | 3.60 | |||||
ZnSO4·7H2O | 17.2 | 0.172 | 0.59 | Zn | 0.039 | 0.59 | |
S | 0.019 | 0.59 | |||||
Na2MoO4 ·2H2O | 48 | 0.048 | 0.20 | Na | 0.009 | 0.40 | |
Mo | 0.019 | 0.20 | |||||
CoCl2·6H2O | 24 | 0.024 | 0.10 | Co | 0.006 | 0.10 | |
Cl | 0.007 | 0.20 | |||||
CuSO4·5H2O | 24 | 0.024 | 0.096 | Cu | 0.006 | 0.096 | |
S | 0.003 | 0.096 | |||||
Vitamin stock solution (VIM)c | |||||||
B12 | 112.4 | 5.5 × 10-4 | 4.0 × 10-4 | 0.5 | |||
Biotin | 104.1 | 5.0 × 10-4 | 2.0 × 10-3 | ||||
Thiamine | 0.1 | 0.03 |
- a
Elements and their concentrations used in different versions of COMBO media here are based on recipes in Kilham et al. (1998) and Sterner et al. (1993) with modifications. Make each stock by adding and dissolving (with vigorous stirring and moderate heat) the appropriate mass of each compound to distilled water. Store each stock solution in a 1-L plastic bottle at room temperature until needed. Compounds with “(b)” are used to make basal medium. Note that concentrations of major compounds in algal COMBO from Kilham et al. (1998) have been modified and NaNO3 has been replaced with (NH4)2SO4. Prepare Sterner-modified algal trace elements (StATE) as detailed by Sterner et al. (1993) but do not add boron. Also follow instructions in Sterner et al. (1993) for preparing vitamins. Concentrations of (NH4)2SO4 and NaH3PO4 in algae COMBO listed here produce nutrient-replete algae but can be reduced to produce N- and P-limited algae. In the final column, we note the volume (ml) of Each stock to add per L of medium that you are making
- b
To make the ATE solution, add 1 ml/L of each stock solution to ∼750 ml distilled water that contained already dissolved EDTA and Fe and adjust to a final volume of 1 L. This makes the ATE stock solution that you add 1 ml/L to the final medium.
- c
To make VIM, add 1 ml of B12 and biotin primary stock solutions to 100 ml distilled water and add 20 mg thiamine HCl to make an intermediate vitamin stock solution (VIM). Keep this intermediate stock solution frozen and add 0.5 ml/L to final medium.
Preparation of COMBO medium
1.Prepare basal COMBO medium in plastic carboys by adding deionized water and each stock solution (see volumes to add in Table 2) with micropippetes.
2.Make algae COMBO by adding N and P stock solutions, StATE, and VIM to basal COMBO (Table 2).
3.Mix algal COMBO in the carboy well and adjust the pH. Add drops of 0.1 M HCl and 0.1 M NaOH until the pH of the medium is between 7.1 and 7.3.
4.Use algae COMBO medium to grow algal cultures and subcultures as detailed below.
Inoculation and establishment of algal cultures
5.Fill 125-ml subculture flasks with 100 ml of algae COMBO medium.
6.Cover with aluminum foil.
7.Autoclave at 121°C for >20 min.
8.After cooling, add 5 to 10 ml of pure algal culture to each flask in a fume hood and immediately re-cover with aluminum foil cover.
9.Place newly inoculated subcultures under growth lamps with a light:dark cycle of 16:8 hr.
10.On a daily basis, swirl to resuspend algae.
11.Once per week, dilute subcultures by discarding ∼60 ml algae and adding back autoclaved algae COMBO medium.
12.Use these subcultures as needed to start food cultures. After 1 month, discard unused subcultures and restart from step 1.
Set up and maintenance of algal cultures used for Daphnia food
13.Fill 2-L culture flasks with algae COMBO medium.
14.Cover with aluminum foil.
15.Autoclave at 121°C for >20 min.
16.After cooling, add 20 to 40 ml of algal subculture and insert sterilized stopper.
17.Connect air pump tube to stopper using glass and plastic tubing. Verify the presence of vigorous air bubbling.
18.Place under growth lamps with a light:dark cycle of 16:8 hr and at a temperature of 20°C.
19.Check cultures periodically over the next several days for signs of algal growth (i.e., increasing green color).
20.Once culture has a noticeable green color, dilute the algal culture daily with autoclaved algae COMBO medium. Do so by pouring 40% to 50% of the algal culture into a 1-L plastic bottle and adding back autoclaved fresh algae COMBO to bring the volume back to 2 L. Quickly remove and replace the rubber stopper to reduce the chances of contamination by non-desired microbes.
21.Save harvested algal cells by centrifuging collected culture outflow 10 min at 5000 × g , room temperature, in a large volume centrifuge. Pour off used medium and resuspend the algal pellet into a smaller volume (200 to 300 ml) of basal COMBO medium.
22.Store concentrated algal food suspensions at 4°C until use. Keep for ≤4 days for experimental use. Keep for ≤1 week if feeding to brood mother Daphnia cultures.
Basic Protocol 2: GENERAL METHODS FOR CULTURING Daphnia
There are two main species of Daphnia used in laboratory experiments. Both D. pulex and D. magna have been extensively studied and each are generally good choices to culture for experimental purposes. It should be recognized that these two species are not closely related and may give different results for a given experiment. In addition, within each species there are many genetically distinct clones that also vary substantially in their physiology. Here we provide methods to grow D. pulex , which are methods that are generally applicable to other species including D. magna and similar small crustaceans. It should be recognized, however, that container size, nutritional conditions, and media formulations may vary with the species being studied.
Mother Daphnia are grown in small groups (i.e., brood mother populations) to produce neonate animals that are then used in experiments. To ensure long-term viability of brood mother populations, multiple populations of Daphnia should be maintained simultaneously. Newly established populations (<3 days old) can be more susceptible to environmental stress and may experience unexpected mass mortality. Retaining older populations until new populations are established helps ensure longer-term viability of the entire lab population. Brood mother populations receive high quantities of algal food to ensure consistency in maternal condition and enough offspring are available for experiments.
Materials
- H2O, deionized
- Daphnia COMBO stock solutions (see Table 3) made with:
- CaCl2·2H2O (Sigma-Aldrich, cat. no. C7902)
- MgSO4·7H2O (Westlab, cat. no. 225-0546)
- NaHCO3 (Sigma Aldrich, cat. no. S6014)
- Na2SiO3·5H2O (Sigma-Aldrich, cat. no. 71746)
- H3BO3 (Fisher Scientific, cat. no. A74-500)
- K2HPO4 (Fisher Scientific, cat. no. P285-500)
- NaNO3 (Fisher Scientific, cat. no. S343-500)
- Na2EDTA (Sigma-Aldrich, cat. no. E5134)
- FeCl3·6H2O (ThermoFisher, cat. no. 217091000)
- MnCl2·4H2O (TCI Chemicals, cat. no. M2095)
- ZnSO4·7H2O (Sigma-Aldrich, cat. no. Z4750)
- Na2MoO4·2H2O (ACROS, cat. no. 206375000)
- CoCl2·6H2O (Sigma Aldrich, cat. no. 255599)
- CuSO4·5H2O (ThermoFisher, cat. no. 197730010)
- Vitamin B12 (Sigma Aldrich, cat. no. V6629)
- Biotin (Sigma Aldrich, cat. no. B4639)
- Thiamine (Sigma Aldrich, cat. no. T1270)
- H2SeO3 (Sigma Aldrich, cat. no. 211176)
- Na3VO4 (Sigma Aldrich, cat. no. S6508)
- LiCl (ACROS, cat. no. 199885000)
- RbCl (ACROS, cat. no. 201270250)
- SrCl2 (ACROS, cat. no. 315081000)
- NaBr (ACROS, cat. no. 246905000)
- KI (ThermoFisher, cat. no. A12704)
- 0.1 M HCl (Current Protocols, 2006)
- 0.1 M NaOH (Current Protocols, 2006)
- Daphnia pulex populations (Fisher Scientific, cat. no. S07269ND)
- Daphnia can be purchased from biological supply companies or may be acquired from academic labs. In both cases, verification of taxonomic status should be completed to ensure the correct species has been received.
- Algal food (see Basic Protocol 1)
Compound | Stock (g/L) | Final medium (mg/L) | Final medium (μmol/L) | Elements | Elements (mg/L) | Elements (μmol/L) | Volume to add to medium (ml/L) |
---|---|---|---|---|---|---|---|
Seven major compound stocks | |||||||
CaCl2·2H2O | 36.76 | 36.76 | 250 | Ca | 10.0 | 250 | 1 |
Cl | 17.7 | 500 | |||||
MgSO4·7H2O | 36.97 | 36.97 | 150 | Mg | 3.65 | 150 | 1 |
S | 4.81 | 150 | |||||
NaNO3 | 85.01 | 85.01 | 1000 | Na | 23.0 | 1000 | 1 |
N | 14.0 | 1000 | |||||
K2HPO4 | 8.71 | 8.741 | 50 | K | 3.91 | 100 | 1 |
P | 1.55 | 50 | |||||
NaHCO3 | 12.60 | 1.08 | 12.9 | Na | 0.30 | 12.9 | 0.086 |
C | 0.15 | 12.9 | |||||
Na2SiO3·5 H2O | 21.21 | 21.21 | 100 | Na | 4.598 | 200 | 1 |
Si | 2.808 | 100 | |||||
H3BO3 | 24.00 | 24.00 | 388.16 | B | 4.20 | 388 | 1 |
Vitamin stock solution (VIM)b | |||||||
B12 | 112.4 | 5.5 × 10-4 | 4.0 × 10-4 | 0.5 | |||
Biotin | 104.1 | 5.0 × 10-4 | 2.0 × 10-3 | ||||
Thiamine HCl | 0.1 | 0.03 | |||||
Algal trace elements solution (ATE)c | |||||||
Na2EDTA | 4.36 | 4.36 | 11.7 | EDTA | 3.42 | 11.7 | 1.0 |
FeCl3·6H2O | 1.0 | 1.00 | 3.7 | Fe | 0.21 | 3.7 | |
Cl | 0.39 | 11.1 | |||||
CuSO4·5H2O | 1.0 | 0.001 | 0.00401 | Cu | 0.000254 | 0.0040 | |
S | 0.000128 | 0.0040 | |||||
ZnSO4·7H2O | 22 | 0.022 | 0.0765 | Zn | 0.005 | 0.0765 | |
S | 0.0024 | 0.0765 | |||||
CoCl2·6H2O | 10 | 0.010 | 0.042 | Co | 0.00248 | 0.042 | |
Cl | 0.00298 | 0.084 | |||||
MnCl2·4H2O | 18 | 0.180 | 0.91 | Mn | 0.05 | 0.91 | |
Cl | 0.0645 | 1.82 | |||||
Na2MoO4·2H2O | 6.0 | 0.006 | 0.0248 | Na | 0.00114 | 0.0496 | |
Mo | 0.00238 | 0.0248 | |||||
H2SeO3 | 1.6 | 0.0016 | 0.0124 | Se | 0.000979 | 0.0124 | |
Na3VO4 | 1.8 | 0.0018 | 0.00979 | Na | 0.000676 | 0.0294 | |
V | 0.0005 | 0.0098 | |||||
Animal trace elements solution (ANIMATE)d | |||||||
LiCl | 310 | 0.31 | 7.31 | Li | 0.05 | 7.31 | 1.0 |
Cl | 0.26 | 7.31 | |||||
RbCl | 70 | 0.07 | 0.58 | Rb | 0.05 | 0.58 | |
Cl | 0.02 | 0.58 | |||||
SrCl2 | 150 | 0.15 | 0.95 | Sr | 0.08 | 0.95 | |
Cl | 0.07 | 1.89 | |||||
NaBr | 16 | 0.016 | 0.16 | Na | 0.004 | 0.16 | |
Br | 0.01 | 0.16 | |||||
KI | 3.3 | 0.0033 | 0.02 | K | 0.0008 | 0.02 | |
I | 0.003 | 0.02 |
- a
Elements and their concentrations are the same as found in Kilham et al. (1998). Make each stock by adding and dissolving (with vigorous stirring and moderate heat) the appropriate mass of each compound to distilled water. Store each stock solution in a 1-L plastic bottle at room temperature until needed. Note the addition of animal trace elements (ANIMATE) to Daphnia COMBO, which is required to meet Daphnia trace element requirement. In the final column, we note the volume (ml) of each stock to add per L of medium that you are making.
- b
To make VIM, add 1 ml B12 and biotin stock solutions to 100 ml distilled water and add 20 mg thiamine HCl to make an intermediate vitamin stock solution (VIM). Keep this intermediate stock solution frozen and add 0.5 ml/L to final medium.
- c
To make the ATE solution, add 1 ml/L of each stock solution to ∼750 ml of distilled water that contained already dissolved EDTA and Fe and adjust to a final volume of 1 L. This makes the ATE stock solution that you add 1 ml/L to the final medium.
- d
To make the ANIMATE, add 1 ml/L of each stock to ∼750 ml distilled water and adjust to a final volume of 1 L. Add 1 ml/L of ANIMATE to final medium.
- 20-L plastic carboys (Fisher Scientific, cat. no. 02-963BB or equivalent)
- 500-ml mason jars
- 3- to 5-ml plastic transfer pipettes (Fisher Scientific, cat. no. 13-680-50)
- Transfer containers, 250-ml beakers (Fisher Scientific, cat. no. FS14000250) or plastic tubs
- 50-ml centrifuge tubes
Preparing Daphnia COMBO
1.Partly fill a plastic carboy with deionized water.
2.Add the correct volume of each stock solution to make the final concentrations as listed for Daphnia COMBO in Table 3.Leave out or modify the concentrations of calcium (Ca), N, or P if you are manipulating these elements in your experiment and want to control supply to your Daphnia populations.
3.Mix the carboy well and adjust the pH of the medium by adding 0.1 M HCl and 0.1 M NaOH until the pH of the medium between 7.1 and 7.3.
4.Store at room temperature and use as needed for Daphnia brood mother cultures and experimentation.
General maintenance and propagation of Daphnia cultures
5.Fill clean 500-ml mason jars with at least 400 ml Daphnia COMBO medium.
6.Start a new population of Daphnia brood mothers by transferring 10 neonates into each jar. Minimize stress on the animals by using a transfer pipette to draw up Daphnia and a small volume of its surrounding water and gently release the animal into its new jar. Set up 5 to 10 jars per generation depending on the number of neonates needed for upcoming experiments.
7.Feed Daphnia brood mothers every second day with concentrated algal food as described in Basic Protocol 1.Use a transfer pipette to add algae to each jar.
Animal age (day) | Daphnia dry mass (μg) | Algal ration (μg C) | Minimum food concentration (mg C/L) | Target food concentration (mg C/L) |
---|---|---|---|---|
0 | 4 | 5 | 0.11 | 1 |
2 | 20 | 25 | 0.56 | 1 |
4 | 50 | 63 | 1.39 | 2 |
6 | 80 | 100 | 2.22 | 3 |
8 | 100 | 125 | 2.78 | 3 |
10 | 120 | 150 | 3.33 | 4 |
12 | 150 | 188 | 4.17 | 5 |
14 | 185 | 231 | 5.14 | 6 |
16 | 215 | 269 | 5.97 | 6 |
18 | 230 | 287 | 6.39 | 7 |
20+ | 240 | 300 | 6.67 | 7 |
- a
Changes in animal dry mass with age are approximate and based on values from previous laboratory measurements of well-nourished reference animals (Mcknight et al. 2023). Algal rations are calculated here as animal mass plus 25% to ensure more than enough food over the ensuing day of feeding. Per animal volume is calculated by dividing the total container volume by the number of animals in each experimental unit. Minimum food concentration is calculated by dividing the algal ration by the per animal volume. In this example, we used a per animal volume of 45 ml as would be the case if you were housing one animal in a 50-ml centrifuge tube (slightly underfilled). Target food concentrations round the minimum food concentration up to the nearest whole number and to be well above the incipient limitation concentration of ∼0.1 mg C/L.
8.After ∼5 to 10 days, depending on the rearing temperature and food level, you will find newly borne Daphnia in your culture jars. For routine maintenance, transfer mother Daphnia into freshly prepared jars of new medium every 2 to 4 days. Mother Daphnia can be distinguished from the newly borne Daphnia based on size, as mothers are much larger (10× by mass) than the offspring. After filling the new set of culture jars with fresh Daphnia COMBO medium (made following Table 3), pour Daphnia from the old jar into a secondary container, usually a small beaker or plastic tube. Gently extract mother Daphnia using transfer pipettes and move these animals into the new jars. Discard neonates and the old medium and repeat for each culture jar. Feed each jar as in step 3.
9.Discard the first set of neonates (usually these appear on day 6 to 9 after starting a new brood mother population) and use neonates from subsequent reproductive events (usually second to fifth brood) to set up the next generation of Daphnia brood mothers. Transfer 10 neonates into new brood mother jars and return to step 1.
10.After verifying that these new brood mother cultures (generation #2) have survived for several days, the older brood mother populations (generation #1) can be discarded.
11.Return to step 2 every 2 weeks to prepare for replacement of current Daphnia brood mother populations and to ensure continuous production of neonates for use in experiments (see next section).
Production of neonate Daphnia for experiments
12.To start a Daphnia experiment, you will need <1-day-old neonates in sufficient numbers to populate your treatments. Ensure all animals are newly born on the first day of the experiment by cleaning out all neonates and juvenile Daphnia from brood mother jars the day before you would like to start the experiment.
13.On the morning of the experiment, inspect culture jars and assess the approximate number of neonates available. If the number appears adequate (based on the calculated number of animals needed for the experimental setup), separate neonates from mother Daphnia and place these animals in a 500-ml beaker containing Daphnia COMBO. Keep a rough count while removing neonates and ensure the number removed from brood mother jars exceeds the required number before setting up the new experiment.
14.After collecting neonates, assign neonates into experimental containers (see Basic Protocols 3 to 5 for details regarding experimental setup) no more than 4 to 6 hr later but within a shorter duration if possible. Randomly select neonates when allocating to experimental containers and randomize by placing animals into containers before assigning treatments.
Creating a genetic bottleneck in a Daphnia population
To maximize genetic similarity of Daphnia cultures, use genetic bottlenecks to eliminate most of the genetic variability among clonal subpopulations prior to starting new sets of experiments.
15.Isolate a single newly borne Daphnia and place it into a 50-ml centrifuge tube containing Daphnia COMBO. Feed with algal food provided in excess (see Table 4 for sample calculations).
16.Check daily for the release of the first brood of neonates. Discard the first brood of neonates.
17.Using one of the subsequent broods, move each neonate into their own 50-ml centrifuge tube containing Daphnia COMBO. Feed these 2nd generation Daphnia sisters as before with excess food.
18.Allocate neonates from brood numbers 2 to 5 from the 2nd generation Daphnia sisters into 500-ml jars and use as brood mothers as detailed above.
Basic Protocol 3: STANDARDIZING AND CONTROLLING NUTRITION FOR EXPERIMENTAL Daphnia
This protocol provides details for feeding animals and guidelines for determining food rations during experiments. For many experiments, the primary aim is to add enough food to prevent starvation and eliminate effects of food limitation. Generally, two pieces of information are needed to determine the needed food ration on any particular day of an experiment: (1) mass of the animal, and (2) volume of water in the experimental container. Previous work has found that a daphnid can consume up to its own mass in food each day (Darchambeau et al., 2003). To ensure animals are not food limited, provide animals food in excess of their estimated mass (see Table 4 for sample calculations). A further complication results from Daphnia being filter feeders, which means their rates of ingestion declines at very low food concentrations (DeMott, 1982). Because of this, adding food to a large volume container could nonetheless result in animal starvation if this results in a low food concentration. Consequently, be sure to add enough food at a high enough concentration to prevent food quantity limitation when feeding Daphnia.
Materials
-
Concentrated algal solutions (see Basic Protocol 1)
-
24-mm glass-fiber filters (VWR, cat. no. 28297-500)
-
Microbalance
-
200-ml filter funnel (VWR, cat. no. 28144-754)
-
Vacuum pump (Fisher Scientific, cat. no. 13-688-810)
-
Vacuum manifold (Fisher Scientific, cat. no. 09-753-39A)
-
Filter forceps (Fisher Scientific, cat. no. XX6200006P)
-
Drying rack (e.g., an ice tray)
-
Drying oven, 60°C
-
1-ml pipettor
Determining the food density of collected algal culture
1.Weigh glass-fiber filters (24-mm) on a microbalance and record pre-weights.
2.Securely place filter into a filter funnel and turn on the vacuum. Pipette 1 to 2 ml of concentrated algae slurry (resulting from Basic Protocol 1) onto the filter. Record volume filtered. Once algae have been collected on the filter and excess water removed, move the filter onto a drying rack (e.g., an ice tray).
3.Place filters into a drying oven at 60°C for at least 1 hr. This time can vary depending on algae density, volume filtered, and oven temperature. If filters appear moist, allow them to dry longer.
4.When the filters are completely dry, reweigh each one on the microbalance and record post-weights.
5.For each filter, calculate the dry mass (mg) of algae (i.e., difference in pre- and post-weights) and divide by the volume filtered (ml) to calculate the algal biomass (mg dry mass/L) in the concentrated algal food jar. Repeat for 2 to 4 filters and use an average algal biomass estimate to calculate the volume to be added to each animal container to provide the desired food ration.
6.Convert the algal dry mass into algal carbon concentration (mg C/L) by dividing the algal density (mg/L) by 0.5 (under the assumption that ∼50% of algal dry mass is C).
7.Calculate the volume of algae needed to feed the animals:
Volume of concentrated algae to add = [target food C concentration (mg C/L) × container vol (L)]/algal C concentration (mg C/L).
Basic Protocol 4: MONITORING Daphnia LIFESPAN
This protocol explains how to track the lifespan of Daphnia raised under experimental conditions. Assessing lifespan generally involves placing individual animals into separate tubes and tracking the proportion of the population alive after a defined time period or until all animals have died. These data can be used to assess maximum lifespan, average age of death, and other metrics of longevity. Lifespan experiments typically require 50 to 100 days to complete if animals are provided good nutrition and raised at 20°C.
Materials
-
Daphnia neonates
-
Daphnia COMBO medium (Table 3)
-
Algal food (see Basic Protocol 1)
-
100-ml glass beakers (Fisher Scientific, cat. no. 02-555-108)
-
50-ml acid-washed conical centrifuge tubes (Fisher Scientific, cat. no. 14955239)
-
Experimental thermostatic chamber (Thermo Fisher Scientific, cat. no. 3920).
-
Aluminum foil
1.Grow adequate numbers of Daphnia brood mothers (see Basic Protocol 2).
2.On the day preceding a new experiment, remove all neonates from brood mother jars to ensure uniform age among experimental animals. Ensure neonates are from the second to fifth brood.
3.On the first day of the experiment, collect new neonates in 100-ml glass beakers. Place a single neonate in separate experimental containers (i.e., 50-ml centrifuge tubes containing 45 ml Daphnia COMBO).
4.Place Daphnia in an experimental thermostatic chamber.
5.Following Basic Protocol 3, feed animals at the start of the experiment and then daily to provide adequate nutrition. Every 4th day, transfer all Daphnia into clean centrifuge tubes containing fresh Daphnia COMBO medium and the daily food ration.
6.Each day prior to feeding, refill centrifuge tubes with Daphnia COMBO medium to reach 45 ml before feeding.
7.Every day, record the number of alive Daphnia and remove animals that have expired.
Basic Protocol 5: EVALUATING Daphnia HEALTH: HEART RATE AND RESPIRATION, BODY MASS AND GROWTH RATES, AND REPRODUCTION
Using standardized conditions, many aspects of Daphnia ’s life-history, physiology, and biochemistry can be assessed as indicators of their general health. Assessing these indicators provides information on their responses to different types of stress including exposure to different environment stressors. Here we explain how to measure four different common responses related to Daphnia metabolism and life-history.
Materials
-
Experimental Daphnia (i.e., treatment related to desired experimental parameters)
-
Daphnia COMBO medium (see Table 2)
-
Daphnia neonates (see Basic Protocol 2)
-
Algal food (see Basic Protocol 1)
-
Glass microscope slides (Fisher Scientific, cat. no. 12-550-143)
-
Transfer pipettes (Fisher Scientific, cat. no. 13-680-50)
-
Light microscope with 10× magnification
-
Differential counter (Fisher Scientific, cat. no. 13-684-140)
-
Digital camera and video-editing software
-
Pre-weighed aluminum cups
-
Microrespirometer [e.g., Unisense Microrespiration System including rack for vials (MR2-Rack), oxygen sensor in guide (OX-MR), UniAmp Multi Channel (UNIAMP-ADAP), personal computer, Unisense software, 4-ml respiration vials (MR-CH4 BASE), and 4-ml 0-cal chamber (MR-CH 0-CAL)]
-
Water bath
-
Drying oven, 60°C
-
50-ml acid-washed conical centrifuge tubes (Fisher Scientific, cat. no. 14955239)
-
Microbalance
-
Data recording sheets
-
20-ml vials (Fisher Scientific, cat. no. 03-337-14) or 400-ml mason jars
Heart rate
Heart rate is a measure of the cardiac activity of Daphnia and can be related to their metabolic rates. As Daphnia are microscopic animals with very high heart rates [>400 to 500 beats per minute (BPM)], these measurements require the use of a microscope and digital camera. As counting of heart beats is subjective, it is highly recommended that blinding and randomization are used to remove counter bias from these measurements.
1.To begin the heart rate measurements, move an individual Daphnia (taken from an experiment) onto a glass slide using a transfer pipette. Carefully place the slide onto the viewing stage of a light microscope. To reduce movement of the animal during measurement, remove as much liquid from around the animal as possible using a transfer pipette. Do so by placing the tip of the pipette downwards so that it lays completely flat on the slide and slowly siphon off most excess fluid.
2.Under 10× magnification locate the heart, which is found on the dorsal side of the body, about midway down, and focus as necessary. Sometimes higher magnification is needed if the morphology of the animal is atypical, or the animal is very small.
3.As soon as the animal is placed on the slide, start a stopwatch timer, and limit the viewing session to <4 min. This is important to avoid suffocating the animal and/or affecting BPM measurements.
4.When the heart is in view, use a digital camera to record a 15 s clip of the actively beating heart.
5.After the 15 s clip is recorded, remove the glass slide from the microscope and wash the Daphnia into a separate container/jar by pipetting some basal COMBO solution onto the slide and holding it at an angle above the container. Save animals in pre-weighed cups to dry and weigh for mass.
6.Use the recorded video clips to count the number of heartbeats per unit time. As Daphnia have very rapid heartbeats, it is difficult to manually count beats per minute without slowing down the recording. Video clips can be slowed using video-editing software (e.g., Capcut) to ∼0.2× the speed of the original clip. Manually count each beat of the heart frame-by-frame until the recording ends using a click-counter. Once complete, multiply this value by the inverse of the clip speed reduction to obtain the BPM.
Respiration
The rate of oxygen consumption has long been used as a measure of metabolic rate in Daphnia and other animals. This protocol provides a commonly used approach for measuring oxygen consumption in Daphnia. As an aquatic animal, this generally requires the tracking of oxygen concentration in water containing one or more animals isolated from atmospheric O2.
7.Turn on the respirometer and calibrate following the manufacturer's instructions. This calibration generally requires measurement of dissolved O2 in oxygen-free water and in well-oxygenated water.
8.Turn on the water bath and set to the desired water temperature.
9.Prepare respiration vials by filling them with clean Daphnia COMBO and a known number of Daphnia. Prior to adding Daphnia to the respiration vials, rinse animals twice with clean Daphnia COMBO to remove algae.
10.Immediately following addition of Daphnia to the measurement vials, transfer to the water bath. Wait several minutes before measuring oxygen concentrations.
11.Before taking the measurements, insert the respirometer's oxygen probe into the vial and allow readings to stabilize. This generally takes 1 to 3 min.
12.Measure the dissolved O2 concentration for 60 s.
13.After 10 min, re-measure dissolved O2 concentration in the same vial. Repeat again 10 min later.
14.Remove animals and place into pre-weighed aluminum cups. Place tins into drying oven for >2 hr at 60°C and reweigh to estimate animal mass (mg). See “Mass-specific growth rate” below for expanded details on materials required to do this.
15.Calculate mass-specific respiration rate as:
Respiration rate = (slope of dissolved O2 vs time)/(animal mass)
Reproduction
Reproductive metrics from Daphnia can be assessed by counting the number of offspring produced over a defined time period. Reduced reproduction is another indicator of stress, nutritional or otherwise.
16.Place individual Daphnia neonates in separate 50-ml centrifuge tubes.
17.Feed daily (see Basic Protocol 3).
18.Transfer experimental Daphnia to clean tubes every 4 days.
19.Inspect daily for the presence of neonates.
20.Upon detection of neonates, separate these newly borne animals from the mother Daphnia.
21.Every day, count and record the number of collected neonates from each Daphnia.
22.Optional: Place neonates in pre-weighed aluminum cups and dry at 60°C until dry. Reweigh to obtain mass of reproduction and individual neonate mass.
23.Continue the daily count of neonate production until the end of the desired observation period or until the death of the mother Daphnia.
Mass-specific growth rate
Mass-specific growth rates give the rate of mass production over the juvenile growth period. This measurement can be a good indicator of health as it is sensitive to temperature, nutrition, and toxin exposure.
24.To start the experiment, collect neonates (Basic Protocol 2) from brood mothers.
25.Place 25 to 30 neonates into a pre-weighed aluminum cup and repeat 3 or 4 times. After drying in an oven at 60°C, re-weigh the 3 to 4 cups on the microbalance. Use these data to estimate the mass of individual neonates.
26.Allocate remaining neonates to experimental containers to start the experiment.
27.Feed with algal food (see Basic Protocol 3).
28.After 4 to 6 days, move individual or groups of Daphnia into pre-weighed aluminum cups. Record the number of neonates in each cup.
29.Dry the cups in a drying oven at 60°C until completely dry (∼1 to 2 days).
30.Re-weigh aluminum cups to determine the total dry mass of experimental animals.
31.Calculate the dry mass per Daphnia by dividing the net dry mass by the number of saved animals.
32.Calculate the mass-specific growth rate (MSGR) using the formula:
COMMENTARY
Critical Parameters and Troubleshooting
See Tables 5 and 6 for troubleshooting guides for growing food algae and Daphnia and for completing Daphnia experiments.
Problem | Possible cause | Solution |
---|---|---|
Poor algal growth | Medium made incorrectly or reagent left out | Remake stock solutions, ensure medium pH is correct |
Algal culture is contaminated with undesired bacteria, fungus, or algae | Examine culture under microscope for non-desired organisms, restart cultures from original source culture | |
Light and/or carbon dioxide limitation | Ensure light source is wide-spectrum and that cultures are vented and/or bubbled | |
Algal cultures are too old | Restart cultures from original source cultures | |
Unexpected Daphnia mortality | Too little or too much food | Redo and check algal dry weight estimates food calculations, measure algal density in Daphnia container to verify correct concentrations |
Incorrect medium formulation | Remake medium with attention to stock volumes being added, check medium pH | |
Handling stress | Increase size of pipette aperture; use more care to reduce/eliminate physical harm when picking up |
Problem | Possible cause | Solution |
---|---|---|
Heart rate | ||
Poor repeatability | Handling stress | Limit the observational periods to ≤4 min |
Inaccurate real time data collection using a manual approach |
Record 15 s video clips, reduce playback to 0.2× speed, and use to calculate BPM |
|
Temperature changes during data collection | Keep jars in a water bath set to 25°C within close vicinity of the microscope to limit temperature fluctuation | |
Respiration | ||
Poor repeatability or unlikely O2 readings | Uncalibrated O2 sensors | Repeat calibration procedures as specified by manufacturer |
Low sensitivity or no O2 response | O2 sensors too old | Purchase new O2 sensor |
Very high O2 consumption rates | Algal/bacteria contamination | Use clean Daphnia COMBO medium and transfer animals through clean medium before transferring into respiration chamber |
Very low O2 consumption rates | Too few animals | Adjust number of animals in respiration chambers until O2 consumption is measurable |
Reproduction | ||
High variability in reproduction among replicates | Poor counting of neonates | Carefully check holding tubes for neonates each day and count neonates by holding beaker over lamp |
Unexpected low survival rates | Medium made incorrectly | Remake medium after checking on stock concentrations |
Little to no reproduction | Incorrect feeding | Recalculate feeding rations and more carefully dispense food |
Growth rate | ||
Values (too high or too low) or overly variable | Medium made incorrectly | Remake medium after checking on stock concentrations |
Incorrect feeding | Recalculate feeding rations and more carefully dispense food | |
Neonate mass estimates incorrect | Reweight neonates using more animals per subsample | |
Calculation incorrect | Check formula and input values | |
Balance issues (uncalibrated or not accurate enough) | Use microbalance that can weigh as little as ≤1 µg |
Basic Protocol 1
The elemental composition of the algal COMBO is critical in ensuring good algal nutrition. Changes to these recipes, in particular to the N and P concentrations, can dramatically affect the growth and viability of algal cultures. The age of algal cultures also is important in producing a constant and high-quality food for laboratory Daphnia populations. As algal cultures age (e.g., are older than 2 to 3 weeks), there are increased chances that they will become contaminated with other algal or bacterial taxa, which can affect the health and production of the cultures. When centrifuged, there should be a tight algal pellet after the spent medium is poured off. Soft, flocculent pellets can indicate that the algal culture needs to be replaced.
Basic Protocol 2
It is important to maintain a regular schedule of feeding brood mother populations, cycling through subsequent generations, and to remove neonates soon after they are born. This ensures that mother Daphnia populations, who will produce neonates for use in experiments, do not experience starvation or other stressful conditions due to the build of waste products in their media.
Basic Protocol 3
Be careful when calculating and adding food rations to experimental Daphnia. Small errors can compound themselves and result in lower than desired food quantity for your experimental animals. Starvation can modify responses of Daphnia to other experimental manipulations and should be avoided.
Basic Protocol 4
Precise estimation of Daphnia longevity and age-specific survival depends on having an adequate starting population size. Smaller populations (e.g., <20 animals) will give survival estimates with less certainty whereas larger populations, when multiplied by the number of treatment combinations, can result in unsustainably large experiments. Consider running multiple experimental runs to increase sample size for each experimental treatment.
Basic Protocol 5
It is critical that experiments use well-nourished neonates of the same age (e.g., <1 day) and then control the same nutritional and environmental conditions over the course of the animal's lifespan.
Understanding Results
Basic Protocol 1
The primary result of Basic Protocol 1 are vibrant green algal cultures (See Fig. 2). The outflow from a well-maintained and healthy culture will produce a dry mass concentration of algae of ∼0.05 to 0.2 mg/ml. This thus yields ∼50 to 200 mg of algal dry mass per 1 L of algal culture when centrifuged.
Basic Protocol 2
There are two primary metrics with which to judge the health of laboratory Daphnia populations. The first is a high survival rate of neonates into adulthood. Typically, well-maintained and healthy Daphnia populations will experience <10% death over the first 20 days of life. Secondly, you should see ample reproduction on a regular schedule. Specifically, the first offspring should appear ∼6 to 9 days after a new population is established with day 0 neonate Daphnia. After this, Daphnia will reproduce about every 3 days with ∼10 neonates per mother.
Basic Protocol 3
To assess results of this protocol, you can create extra experimental units and sacrifice to see if desired food quantities are present. The measured algal dry mass should match that calculated to meet or exceed the minimum food requirements. In addition, Daphnia growth and reproductive rates close to the maximum (see below) are further evidence that nutritional requirements are being met.
Basic Protocol 4
When monitoring lifespan, there is a typical survival curve where ∼90% to 100% survival should be seen over the first 2 to 3 weeks of the experiment. This will be followed by a period where most of the population will expire over the following weeks with a few animals exhibiting longer lifespan. Under control conditions (e.g., good food and no stress), average lifespan will be ∼30 to 40 days at 25°C. It should be noted that this survival curve and lifespan in Daphnia is very temperature sensitive and varies by species and clone.
Basic Protocol 5
Each of these health indices are very sensitive to food, temperature, and other environmental conditions and can differ among daphnid species and clones. For Daphnia pulex raised on high food quantity at 20°C, the following are typical values for each response variable.
Heart rate: 400 BPM. Mass-specific respiration rate: 0.3 μmol O2 mg dry mass–1 h–1. Mass-specific growth rate: 0.5 day−1. Age of first reproduction: 7 days. Eggs per brood: 10 to 20. Inter-brood duration: 2 to 3 days.
Time Considerations
Basic Protocol 1
Preparation of algal COMBO medium: 2 to 4 hr to make media stocks and one 20-L batch takes ∼0.5 to 1 hr to mix and titrate. Inoculation and establishment of algal cultures: Setting up new cultures with autoclaved medium takes ∼5 min per culture. Set up and maintenance of algal cultures used for Daphnia food: Diluting algae takes ∼5 min per culture/flask. Centrifuging algae requires ∼15 to 30 min (including balancing and centrifugation).
Basic Protocol 2
Preparing Daphnia COMBO: 2 to 4 hr to make media stocks and one 20-L batch takes ∼0.5 to 1 hr to mix and titrate. General maintenance and propagation of Daphnia cultures: 0.5 to 1.0 hr to feed, remove neonates, and set up new populations.
Basic Protocol 3
Determining the food density of collected algal culture requires 2 to 4 hr to concentration of algae, prepare and dry filters, and reweigh filters before calculating density.
Basical Protocol 4
4 to 6 hr to set up on first day and 1 to 4 hr daily after that to assess survival and count/remove neonates.
Basic Protocol 5
Heart rate: 1 to 2 hr to film Daphnia. Respiration: 4 to 6 hr to isolate Daphnia , incubate in respirometer, and dry/weigh animals afterwards. Reproduction: 2 to 6 hr daily to count number of new neonates across the experiment. Mass-specific growth rates: 1 to 3 hr to isolate animals in aluminum tins, dry, and reweigh tins.
Acknowledgments
This work was supported by an NSERC Discovery grant awarded to P.C.F. We thank all former Frost laboratory members, who helped develop and test these methods. Also, we thank Uzair Khan and an anonymous reviewer for comments on an earlier draft of this manuscript.
Author Contributions
Paul Frost : Conceptualization; methodology; project administration; resources; supervision; writing original draft; writing review and editing. Samantha Caudle : Formal analysis; methodology; writing original draft; writing review and editing. Sen Han : Formal analysis; methodology; writing original draft; writing review and editing. Jocelyn O'Brien : Writing original draft; writing review and editing. Stephanie Wales Tobin : Conceptualization; project administration; supervision; writing original draft; writing review and editing.
Conflict of Interest
The authors declare no conflict of interest.
Open Research
Data Availability Statement
Data sharing not applicable to this article as no datasets were generated or analyzed during the current study.
Literature Cited
- Alva-Martínez, A. F., Sarma, S. S. S., & Nandini, S. (2004). Population growth of Daphnia pulex (Cladocera) on a mixed diet (Microcystis aeruginosa with Chlorella or Scenedesmus). Crustaceana , 77, 973–988.
- Boersma, M. (1997). Offspring size and parental fitness in Daphnia magna. Evolutionary Ecology , 11, 439–450. https://doi.org/10.1023/A:1018484824003
- Carotenuto, Y., Wichard, T., Pohnert, G., & Lampert, W. (2005). Life-history responses of Daphnia pulicaria to diets containing freshwater diatoms: Effects of nutritional quality versus polyunsaturated aldehydes. Limnology and Oceanography , 50(2), 449–454. https://doi.org/10.4319/lo.2005.50.2.0449
- Cohen, A. A. (2018). Aging across the tree of life: The importance of a comparative perspective for the use of animal models in aging. Biochimica et Biophysica Acta Molecular Bases of Disease , 1864(9), 2680–2689. https://doi.org/10.1016/j.bbadis.2017.05.028
- Current Protocols. (2006). Commonly Used Reagents. Current Protocols in Microbiology , 00, A.2A.1–A.2A.15. https://doi.org/10.1002/9780471729259.mca02as00
- Darchambeau, F., Faerøvig, P. J., & Hessen, D. O. (2003). How Daphnia copes with excess carbon in its food. Oecologia , 136, 336–346. https://doi.org/10.1007/s00442-003-1283-7
- DeMott, W. R. (1982). Feeding selectivities and relative ingestion rates of Daphnia and Bosmina. Limnology and Oceanography , 27(3), 518–527. https://doi.org/10.4319/lo.1982.27.3.0518
- Ebert, D. (2005). Ecology, epidemiology and evolution of parasitism in Daphnia. Evolution , 3.
- Ebert, D. (2022). Daphnia as a versatile model system in ecology and evolution. EvoDevo , 13(1), 16. https://doi.org/10.1186/s13227-022-00199-0
- Gems, D., & Partridge, L. (2013). Genetics of longevity in model organisms: Debates and paradigm shifts. Annual Review of Physiology , 75, 621–644. https://doi.org/10.1146/annurev-physiol-030212-183712
- Guarente, L., & Kenyon, C. (2000). Genetic pathways that regulate ageing in model organisms. Nature , 408(6809), 255–262. https://doi.org/10.1038/35041700
- Kilham, S. S., Kreeger, D. A., Lynn, S. G., Goulden, C. E., & Herrera, L. (1998). COMBO: A defined freshwater culture medium for algae and zooplankton. Hydrobiologia , 377(1–3), 147–159. https://doi.org/10.1023/A:1003231628456
- López-Otín, C., Blasco, M. A., Partridge, L., Serrano, M., & Kroemer, G. (2023). Hallmarks of aging: An expanding universe. Cell , 186(2), 243–278. https://doi.org/10.1016/j.cell.2022.11.001
- MacArthur, J. W., & Baillie, W. H. T. (1929a). Metabolic activity and duration of life. I. Influence of temperature on longevity in Daphnia magna. JEZ , 53(2), 221–242. https://doi.org/10.1002/jez.1400530205
- MacArthur, J. W., & Baillie, W. H. T. (1929b). Metabolic activity and duration of life. II. Metabolic rates and their relation to longevity in Daphnia magna. JEZ , 53(2), 243–268. https://doi.org/10.1002/jez.1400530206
- McKay, A., Costa, E. K., Chen, J., Hu, C. K., Chen, X., Bedbrook, C. N., Khondker, R. C., Thielvoldt, M., Singh, P. P., Wyss-Coray, T., & Brunet, A. (2022). An automated feeding system for the African killifish reveals the impact of diet on lifespan and allows scalable assessment of associative learning. eLife , 11, e69008. https://doi.org/10.7554/eLife.69008
- McKnight, E., Jones, C., Pearce, N., & Frost, P. (2023). Environmental stress and the morphology of Daphnia pulex. Physiological and Biochemical Zoology , 96, 438–449. https://doi.org/10.1086/728316
- Miner, B. E., de Meester, L., Pfrender, M. E., Lampert, W., & Hairston, N. G. (2012). Linking genes to communities and ecosystems: Daphnia as an ecogenomic model. Proceedings of the Royal Society B , 279(1735), 1873–1882. https://doi.org/10.1098/rspb.2011.2404
- Müller-Navarra, D. C. (1995). Biochemical versus mineral limitation in Daphni a. Limnology and Oceanography , 40(7), 1209–1214. https://doi.org/10.4319/lo.1995.40.7.1209
- Nova, C. C., Bozelli, R. L., Spitzy, A., & Müller-Navarra, D. (2019). Living in a browning environment: Effects on Daphnia ’s growth and fatty acid pattern. Limnology and Oceanography , 64(1), 18–31. https://doi.org/10.1002/lno.11016
- Spaak, P., & Hoekstra, J. R. (1995). Life history variation and the coexistence of a Daphnia hybrid with its parental species. Ecology , 76(2), 553–564. https://doi.org/10.2307/1941213
- Starke, C. W., Jones, C. L., Burr, W. S., & Frost, P. C. (2021). Interactive effects of water temperature and stoichiometric food quality on Daphnia pulicaria. Freshwater Biology , 66(2), 256–265. https://doi.org/10.1111/fwb.13633
- Sterner, R. W., Hagemeier, D. D., Smith, W. L., & Smith, R. F. (1993). Phytoplankton nutrient limitation and food quality for Daphnia. Limnology and Oceanography , 38(4), 857–871. https://doi.org/10.4319/lo.1993.38.4.0857
- Szabelak, A., & Bownik, A. (2021). Behavioral and physiological responses of Daphnia magna to salicylic acid. Chemosphere , 270, 128660. https://doi.org/10.1016/j.chemosphere.2020.128660
- Taipale, S. J., Aalto, S. L., Galloway, A. W., Kuoppamäki, K., Nzobeuh, P., & Peltomaa, E. (2019). Eutrophication and browning influence Daphnia nutritional ecology. Inland Waters , 9(3), 374–394. https://doi.org/10.1080/20442041.2019.1574177
- Tkaczyk, A., Bownik, A., Dudka, J., Kowal, K., & Ślaska, B. (2021). Daphnia magna model in the toxicity assessment of pharmaceuticals: A review. Science of The Total Environment , 763, 143038. https://doi.org/10.1016/j.scitotenv.2020.143038
- Wagner, N. D., Hillebrand, H., Wacker, A., & Frost, P. C. (2013). Nutritional indicators and their uses in ecology. Ecology Letters , 16(4), 535–544. https://doi.org/10.1111/ele.12067