Production of Humanized Mice through Stem Cell Transfer
Cameron Phelps, Cameron Phelps, Devra D. Huey, Devra D. Huey, Stefan Niewiesk, Stefan Niewiesk
human immune system (HIS)
human leukocyte antigen A2 (HLA-A2)
human umbilical cord stem cells (HUSCs)
NOD.SCID gamma (NSG) humanized mice
Abstract
The development of “humanized” mice has become a prominent tool for translational animal studies of human diseases. Immunodeficient mice can be humanized by injections of human umbilical cord stem cells. The engraftment of these cells and their development into human lymphocytes has been made possible by the development of novel severely immunodeficient mouse strains. Proven protocols for the generation and analysis of humanized mice in the NSG mouse background are presented here. © 2023 The Authors. Current Protocols published by Wiley Periodicals LLC.
Basic Protocol 1 : Human umbilical stem cell engraftment of neonatal immunodeficient mice
Basic Protocol 2 : Human umbilical stem cell engraftment of 4-week-old immunodeficient mice
Support Protocol 1 : Preparation of human umbilical stem cells
Support Protocol 2 : Submandibular blood collection from humanized mice and analysis of peripheral blood via flow cytometry
INTRODUCTION
Mouse models are widely used in laboratory research and development. The mouse is the preferred experimental animal due to its small size, ease of handling, and short reproductive cycle, as well as the wealth of information that has been generated with mouse models. However, mouse models of human disease pose limitations because of their lack of susceptibility to certain infections and the lack of a human immune system. This impacts the ability to use mice in various fields, for example, the study of human pathogens such as retroviruses. Human immune system (HIS) mouse models are generated by engrafting CD34+ human umbilical stem cells (HUSCs). The NOD.SCID Il2rγ null (NSG) mouse strain has been widely used to perform HUSC engraftment (Shultz et al., 2005). Immunodeficient NSG mice lack functional mouse T cells, B cells, and NK cells and are deficient in cytokine signaling. After transfer of HUSCs, phenotypically normal human immune cells develop in NSG mice. These cells circulate normally and are susceptible to drugs and infections, but generate only poor adaptive immune responses if any. The Jackson Laboratory has developed an array of NSG mice that are transgenic for a variety of human molecules to improve immune function (https://www.jax.org/jax-mice-and-services/find-and-order-jax-mice/nsg-portfolio). Their collection includes strains with human cytokines to promote engraftment of human leukocytes (Coughlan et al., 2016), strains that develop functional cytotoxic T cells specific to the transgene human leukocyte antigen class I (HLA-A2) (Shultz et al., 2010), and strains with knockouts of mouse major histocompatibility complex class II (MHC-II) but inserted with HLA-DQ8 for a human insulin-specific regulatory T cell response (Serr et al., 2016). Basic Protocols 1 and 2 describe the generation of humanized mice by two different methods that are chosen based on the age of the mice and the ease of application. If neonatal mice are humanized within 72 hr of birth, the HUSCs are injected into the liver. If mice are humanized at 4 weeks of age, they are humanized by intravenous tail vein injections. Support Protocols 1 and 2 describe techniques used to handle sensitive HUSCs and monitor leukocyte populations engrafted into humanized mice.
NOTE : All protocols involving live animals must be reviewed and approved by an Institutional Animal Care and Use Committee (IACUC) and must conform to government regulations for the care and use of laboratory animals.
STRATEGIC PLANNING
Immunodeficient Mouse Strains
Two mouse strains, NOD.Cg-Prkdcscid Il2rgtm1Wjll (NSG) and BALB/c-Rag2nul1 IL2rγnull (BRG), are used most often to produce humanized mice. These have either a complete or partial deletion of the IL-2 receptor gamma chain (IL2rγ). The Rag1 or Rag2 genes are inactivated by mutations in NSG mice, whereas both genes are deleted in BRG mice. In both strains, this results in the lack of mature T cells, B cells, or functional NK cells and a deficiency in cytokine signaling (Coughlan et al., 2016; Shultz et al., 2005). Although both strains are proven models for engraftment of HUSCs, one study indicates that NSG mice support HUSC engraftment better than BRG mice (Brehm et al., 2010). NSG and BRG mice are easily obtainable from The Jackson Laboratory and must be bred and maintained in a specific pathogen-free and, if applicable, BSL-2 facility.
Sources of HUSCs
HUSCs are isolated from umbilical cord blood and purified to ≥90% CD34+ cells, a marker for HUSCs. The CD34+ cells are selected using magnetic beads (Miltenyi CliniMACS system; Schumm et al., 1999). In addition to umbilical cord blood (Ishikawa et al., 2005), human stem cells can also be purified from various other human tissues, such as fetal liver, bone marrow (Holyoake et al., 1999), and peripheral blood (Shultz et al., 2005). In our hands, the generation of human lymphocytes in NSG mice was far superior with HUSCs compared to peripheral blood stem cells (unpublished data).
Obtaining human umbilical cord for purification of HUSCs for research requires ethical review approval from an Institutional Review Board (IRB), whereas the purchase of frozen stem cells usually does not require approval. Laboratory-generated HUSCs should be checked by flow cytometry for purity (CD34 expression) and especially the absence of contaminating T cells (CD3, CD4, and CD8 expression), which will lead to a graft-versus-host reaction in mice. Subsequently, small aliquots of purified HUSCs should be tested for adverse reactions (graft-versus-host) in mice. HUSCs from commercial sources are more costly but less labor-intensive to use and usually of very good purity.
Basic Protocol 1: HUMAN UMBILICAL STEM CELL ENGRAFTMENT OF NEONATAL IMMUNODEFICIENT MICE
In this protocol, neonatal immunodeficient pups are injected intrahepatically with HUSCs (Fig. 1; Pearson et al., 2008). Engraftment can also be done intravenously via the intracardiac route or facial vein, intraperitoneally, or by intratibial injection. Prior to engraftment, preconditioning of the pups with low-dose sublethal irradiation is necessary to ensure engraftment, proliferation, and survival of HUSCs.
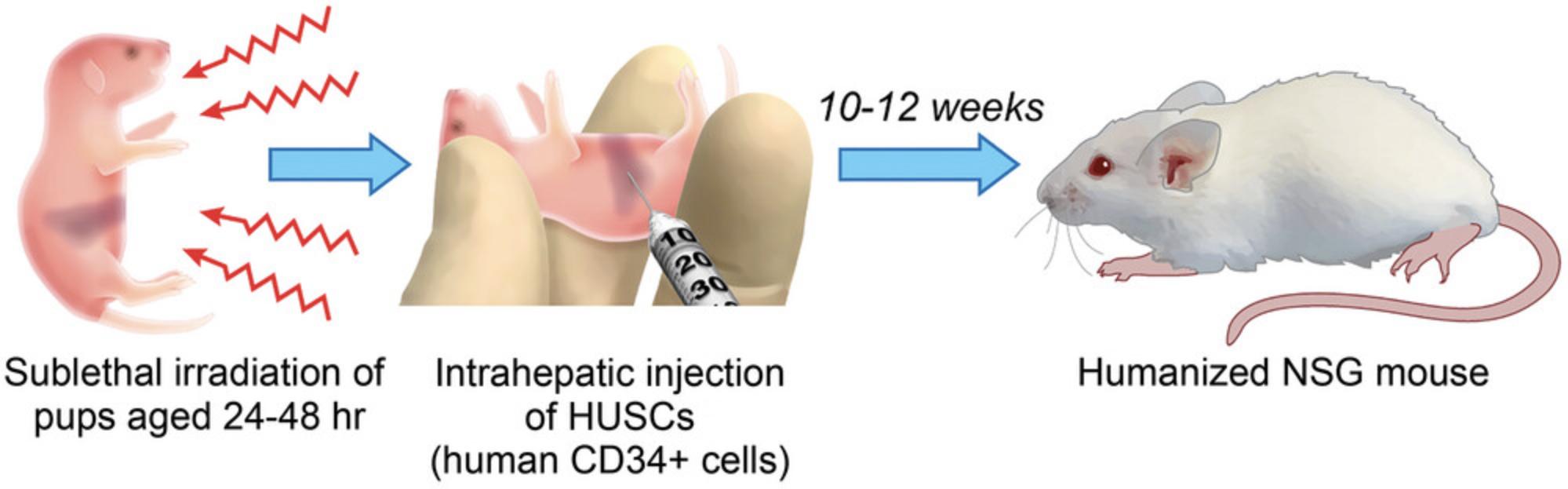
Materials
-
Male and female adult mice: NOD.Cg-Prkdcscid Il2rgtm1Wjl /SzJ (The Jackson Laboratory, IMSR cat. no. JAX:005557, RRID:IMSR_JAX:005557)
-
HUSCs (see Support Protocol 1)
-
Topical, petroleum-based nasal decongestant (e.g., Vicks VapoRub)
-
Autoclaved, ventilated cage or container for housing pups in irradiator chamber
-
Sterile biosafety hood
-
X-ray irradiator (RS 2000 X-ray Biological Irradiator, RadSource)
-
26- to 30-G insulin syringes (BD, cat. no. 328466)
-
Warming lamp or heating pad
1.Pair male and female NSG mice and monitor for new litters (from day 20 onwards).
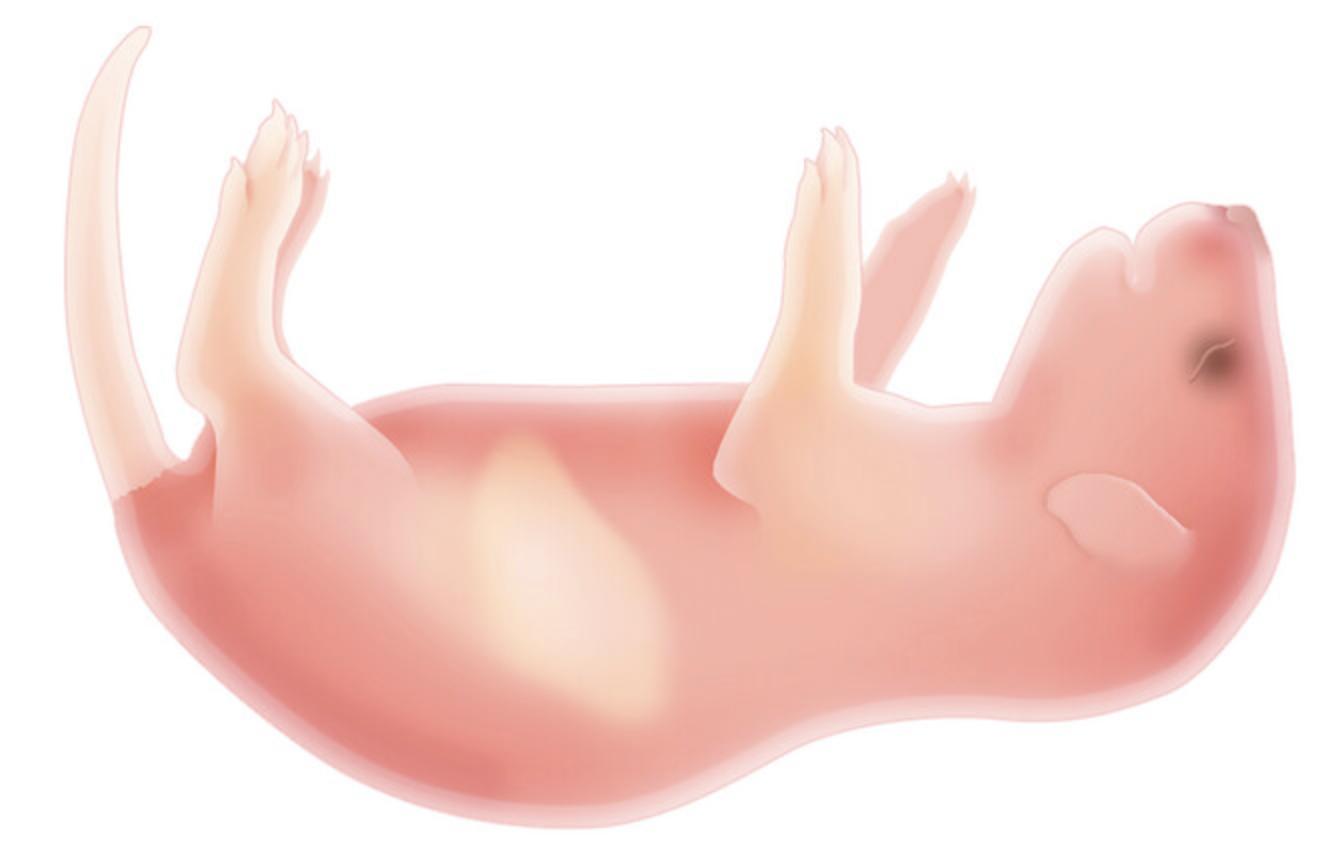
2.On the day of the engraftment, turn on the X-ray irradiator and initiate the warm-up procedure.
3.Count HUSCs and suspend in medium at 3–10 × 104 CD34+ cells/50 µl.
4.Place 24-hr to 48-hr-old pups in a sterile, ventilated cage (typically one litter per cage) in a sterile biosafety hood.
5.Before pups are irradiated and anesthetized, restrain parents and place a small amount of topical, petroleum-based nasal decongestant on their snouts.
6.Place the cage containing pups in the RAD+ reflector block of the irradiator chamber (level 1 of the instrument) and irradiate pups for ∼1 min at 1 Gy.
7.Transfer irradiated pups to a weigh boat on ice until they are anesthetized by the cold. Use cessation of movement as a sign of anesthesia (this could take ∼5 min).
8.Load an insulin syringe with a 26- to 30-G needle with the HUSC suspension.
9.Restrain pups for intrahepatic injection and dispense 50 µl HUSC suspension into the liver.
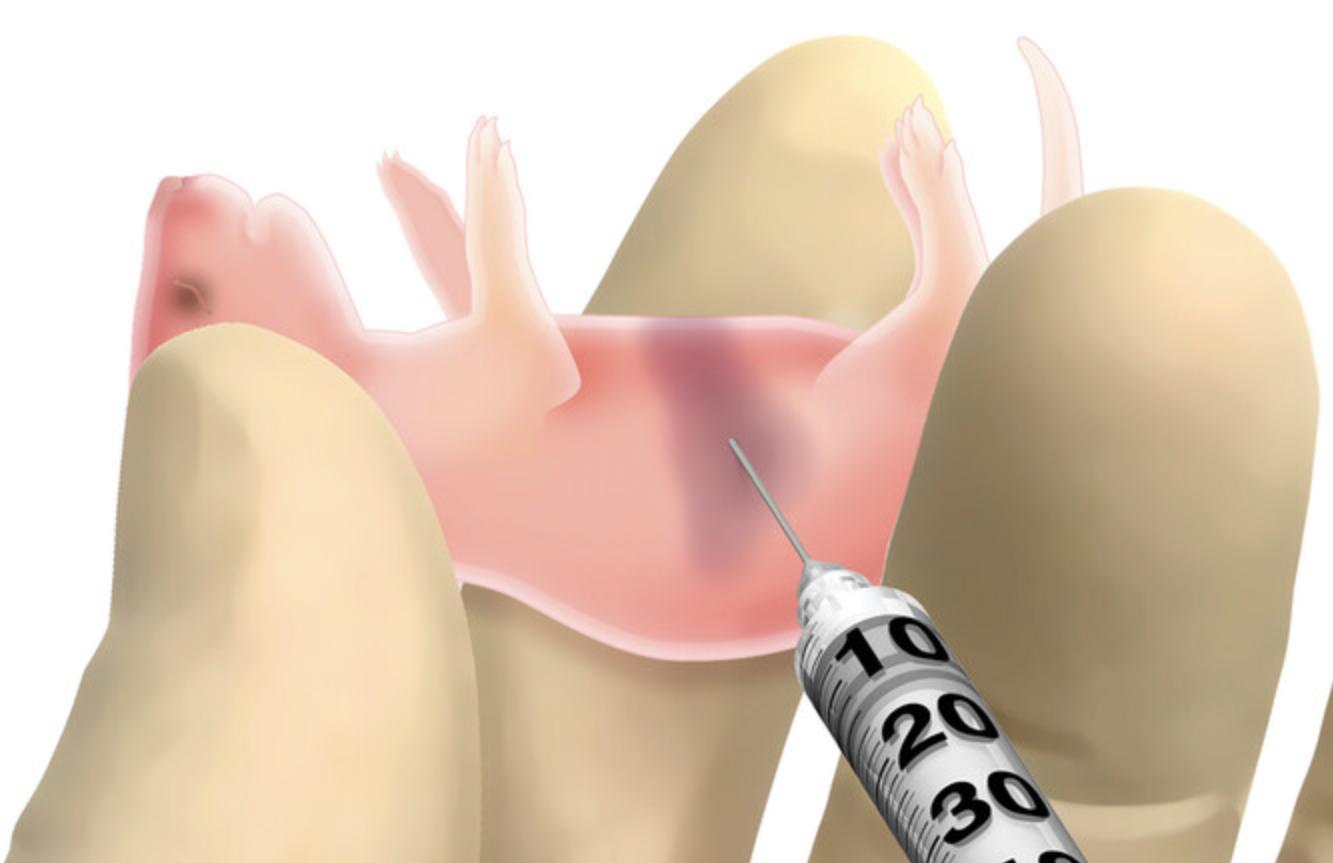
10.Return pups to the parent cage and roll them in the bedding to reduce smells associated with handling. Warm the pups under a warming lamp for 1-2 min.
11.Wean pups at 21-24 days post birth.
12.Verify engraftment of HUSCs starting at 10 weeks of age by using flow cytometry to analyze peripheral blood staining for human CD45+ (see Support Protocol 2).
Basic Protocol 2: HUMAN UMBILICAL STEM CELL ENGRAFTMENT OF 4-WEEK-OLD IMMUNODEFICIENT MICE
To generate humanized mice at the age of 4 weeks, mice are injected with HUSCs by intravenous tail injection. This method can be used for NSG or NSG.HLA-A2 strains.
Additional Materials (also see Basic Protocol 1)
- 4-week-old mice (The Jackson Laboratory):
- NOD.Cg-Prkdcscid Il2rgtm1Wjl /SzJ (IMSR cat. no. JAX:005557, RRID:IMSR_JAX:005557)
- NOD.Cg-Prkdcscid Il2rgtm1Wjl Tg(HLA-A/H2-D/B2M)1Dvs/SzJ (ISMR cat. no. JAX:014570, RRID:IMSR_JAX:014570)
- 28-G insulin syringes (BD, cat. no. 329424)
- Injection platform (Brain Tree Scientific, cat. no. IL-300)
- Alcohol wipes
1.Turn on the X-ray irradiator and initiate the warm-up procedure.
2.Count HUSCs and suspend in medium at 3–10 × 104 CD34+ cells/50 µl.
3.Place 4-week-old adult mice in a sterile, ventilated cage (up to five per cage) in a sterile biosafety cabinet.
4.Place the cage in the RAD+ reflector block of the irradiator chamber (level 1 of the instrument) and irradiate mice at 1.5 Gy for ∼2 min.
5.Return irradiated mice to their cages.
6.Prior to engraftment, warm one to several mice by placing a heat lamp within a few inches of the cage (this should take ∼30 min).
7.Load an insulin syringe with a 28-G needle with the HUSC suspension.
8.Place the first mouse under the dome on the injection platform and then slide the tail through the slot so that it is facing you. Secure the tail by gently holding it with thumb and index finger, then wipe the tail with an alcohol wipe.
9.Grab the tail with the thumb and index finger from top and bottom and twist it so that one of the four tail veins faces up.
10.Slightly pull and bend the tail so the surface of the bend (the injection site) is flat. Hold the needle parallel to the tail, insert it bevel-up into the vein, and inject 50 μl suspension.
11.Return mouse to its cage and repeat for all remaining mice.
12.After 8 weeks, verify engraftment of HUSCs starting at 10 weeks of age by using flow cytometry to analyze peripheral blood staining for human CD45+ (see Support Protocol 2).
Support Protocol 1: PREPARATION OF HUMAN UMBILICAL STEM CELLS
Human CD34+ hematopoietic stem cells are isolated from human cord blood to alter immunodeficient mice. For sources of HUSCs, see Strategic Planning. This protocol describes how to thaw the cells and prepare them for injection.
Materials
-
Hematopoietic growth medium (HPGM; Bioz, cat. no. PT-3926)
-
Fetal bovine serum (FBS)
-
Frozen HUSCs
-
70% ethanol
-
StemSpan CC100 (human cytokine cocktail; Stem Cell Technologies, cat. no. 02690)
-
37°C water bath
-
15-ml tubes
-
37°C incubator
1.Prepare 30 ml HPGM with 10% FBS and warm in a 37°C water bath.
2.Wipe the exterior of a vial of frozen cells (typically 106 cells) with 70% ethanol and thaw quickly in a 37°C water bath.
3.Aseptically transfer the entire volume of thawed cells to a 15-ml tube.
4.Rinse vial that the cells were frozen in with 1 ml warm medium and add it dropwise (over ∼1 min) to the 15-ml tube while swirling.
5.Slowly add warm medium dropwise to the cells, while swirling, until a total volume of 5 ml is reached over a 3-min period.
6.Slowly add medium dropwise up to a total volume of 15 ml over ∼5-10 min, gently swirling after each addition.
7.Centrifuge suspension for 15 min at 200 × g , room temperature.
8.Remove most of the supernatant by pipet, being careful not to disturb the pellet (leave a few milliliters of supernatant behind).
9.Slowly add fresh medium to a volume of 15 ml while gently swirling.
10.Centrifuge 15 min at 200 × g , room temperature.
11.Carefully remove supernatant by pipet, leaving 1-2 ml.
12.Gently resuspend pellet in a known volume of HPGM and count cells.
13.Add 1× StemSpan CC100 to the cells.
14.Place cells in a 37°C incubator until ready to use.
Support Protocol 2: SUBMANDIBULAR BLOOD COLLECTION FROM HUMANIZED MICE AND ANALYSIS OF PERIPHERAL BLOOD VIA FLOW CYTOMETRY
Flow cytometry is an easy method for monitoring human stem cell engraftment in the peripheral blood of humanized mice. Peripheral blood from the facial vein can be collected into a microtainer tube containing EDTA and stained using antibodies specific for human markers.
Materials
-
Engrafted mice (see Basic Protocol 1 or 2)
-
Fluorescently labeled antibodies (Thermo Fisher Scientific):
- Human CD45 FITC (clone HI30)
- Human CD4 Superbright 600 (clone RPA-T4)
- Human CD8 Superbright 436 (clone HIT8a)
- Human CD3 PE Cyanine 5.5 (clone SK7)
-
UltraComp eBeads Plus Compensation Beads (Invitrogen, cat. no. 01-3333-42)
-
BD FACS Lysing Solution (BD Biosciences, cat. no. 349202)
-
Flow buffer: phosphate-buffered saline (PBS) with 0.1% fetal calf serum (FCS) and 0.02% NaN3
-
EDTA blood collection tubes (e.g., BD Biosciences Microtainer tubes, cat. no. 365974)
-
21-G BD PrecisionGlide Needles (cat. no. 305165)
-
12 × 75–mm round-bottom disposable test tubes (Falcon, cat. no. 352052)
-
Test tube shaker
-
Flow tubes: 12 × 75–mm round-bottom disposable test tubes (Falcon, cat. no. 352052
-
Flow cytometer (e.g., Attune NxT, Life Technologies)
1.Restrain mice and collect 50-200 µl whole blood by submandibular bleed into one EDTA collection tube per mouse.
2.For each sample, resuspend blood and transfer 50 µl to a labeled 12 × 75–mm flow tube. Use the remaining blood for unstained controls and compensation beads.
3.Stain each sample by adding 5 µl of each antibody (typically 0.5 μg per sample). Do not add antibody to unstained controls.
4.Cover samples to protect from light and shake at 150 rpm for 30 min at room temperature.
5.While samples are staining, prepare the compensation bead controls:
-
Vortex the bottle of beads briefly and then add one drop to a separate flow tube for each fluorophore.
-
Add 0.5 μl of each antibody to the respective tubes. Cover and vortex for ∼5-10 s.
-
Incubate at 4°C for 30 min.
6.Add 200 ml of 1× BD FACS Lysing Solution to each sample tube. Vortex for ∼5-10 s and incubate 15 min at room temperature, protected from light.
7.While samples are lysing, finish the preparation of the compensation beads:
-
Add 2 ml flow buffer per tube and centrifuge 5 min at 400 ×g.
-
Aspirate supernatant and resuspend beads in 0.5-1 ml flow buffer.
8.Wash samples by adding 2 ml flow buffer to each tube and centrifuging 5 min at 200 × g. Aspirate supernatant.
9.Add 0.5-1 ml flow buffer to each tube and analyze on a flow cytometer.
-
Briefly vortex each sample immediately before analysis.
-
Use a flow cytometer that can read four-color cytometry. Collect at least 10,000 events.
COMMENTARY
Background Information
Humanized mouse models have become increasingly important tools in the study of human diseases. One potential problem in the generation of humanized mice is the development of graft-versus-host disease after transplantation of HUSCs. Signs of graft-versus-host disease in mice may include a hunched posture, hair loss, weight loss, and early death. NSG mouse strains have been reported to be the better model for engraftment of HUSCs due to faster expansion of human CD45+ cells, but this may also explain the development of graft-versus-host disease at a faster rate (Ali et al., 2012; Brehm et al., 2010). The absence of T cells in the HUSC preparation is important for avoiding graft-versus-host disease. In addition to the classical T cell–based disease, the development of a macrophage-based granulomatous disease has been described (Huey et al., 2018). Granulomatosis was observed in various organs with both mouse and human macrophages.
Another challenge of the humanized mouse model is the development of human lymphocytes that are not immunocompetent following inoculation of HUSCs (Villaudy et al., 2011). Part of the problem seems to be that mouse cytokines do not fully support the development of human lymphocytes. Two approaches have been pursued to overcome these hurdles: (1) the use of additional human fetal tissue and (2) the generation of human cytokines in humanized mice. In the first approach, mice are surgically transplanted with human fetal liver and thymus (the BLT mouse), which allows for development of functional human leucocytes (Lan et al., 2006). However, this model requires surgical expertise and the availability of bone marrow, liver, and thymus from a human fetus. Moreover, and the use of fetal tissue for research is not legal in parts of the United States and other countries. Another approach is the generation of a transgenic mouse expressing human MHC and cytokine genes in NSG or BRG mice (Billerbeck et al., 2011; Shultz et al., 2010; Strowig et al., 2011; Willinger et al., 2011), which has resulted in a partial restoration of immune function. A third approach to facilitate the development of humanized mice is to use a viral vector system to deliver human genes into the mouse model prior to injection of HUSCs. NSG mice transduced with adeno-associated virus (AAV) expressing human HLA-A2 and human cytokines such as IL-3, IL-15, and GM-CSF have resulted in increased levels of functional human CD45+ cells (Huang et al., 2014, 2015).
Critical Parameters and Troubleshooting
Mouse breeding and care
For establishment of an NSG mouse breeding colony, mice must be kept under specific pathogen-free (SPF) conditions. Mice become sexually active between 6 and 8 weeks of age. Young NSG mice usually breed better than older mice, and performance usually is best if mice are paired at 5-6 weeks of age. Breeding performance and litter size may depend on various factors such as barometric pressure, temperature, humidity, noise, handling of pregnant mice, husbandry enrichments, and the overall health of the breeding pair. In our hands, one-on-one breeding (mating one male and one female) has established stable breeding and reduction of cannibalism. In contrast, trio breeding and continuous breeding have been reported but may not be as successful. The traditional mouse diet contains 6% fat (LabDiet, cat. no. 5K52; Teklad LM-485, cat. no. 7912). Breeding success can be supported by feeding a diet containing higher fat and therefore higher energy content (e.g., Teklad S-2335, cat. no. 7904). Under optimal breeding conditions, an NSG litter should an average of eight pups per litter with a range of two to twelve pups. The majority of NSG breeders stop breeding by 7-8 months of age. To keep the breeding colony active, breeder pairs that have not had a litter in 60 days or consistently produce small litters should be replaced, as should breeder pairs that cannibalize their pups more than once. To reduce cannibalism after handling pups, applying a small amount of Vicks VapoRub to the parents’ snouts (National Research Council, 2003) and gently smearing the pup with bedding from the cage will mask human olfactory scents that have been transferred to the pups.
NSG mice are immunodeficient and therefore prone to infections such as Enterococcus spp., Klebsiella oxytoca, Staphylococcus aureus, Pseudomonas , and Corynebacterium bovis (Foreman et al., 2011), which can easily be dealt with by immunocompetent mice. To keep NSG mice healthy and reduce disease outbreaks, proper aseptic handling and housing practices should be performed under strict barrier conditions. Suggested strict barrier practices include the use of microisolator cages; autoclaving of all food, water, bedding, and cages prior to entering the room; and manipulating cages inside a biosafety cabinet. All personnel should wear personal protective equipment including disposable gowns, hair bonnets, masks, and disinfected gloves. All cages should be changed at least biweekly, although weekly changes are ideal. In monitoring animal health, the testing of microorganisms typical for immunodeficient animals (see above) must be included.
In recent years, C. bovis has become a pathogen of concern. It is a gram-positive bacterium identifiable by clinical signs of alopecia and dermatitis. It has been demonstrated that C. bovis interferes with human cell engraftment of immunodeficient mice (NSG) whether the mice are symptomatic or asymptomatic (Vedder et al., 2019). Transmission of C. bovis occurs by keratin flake shedding by mice and by fomites that contaminate the facility by personnel. Because it is also transmitted through contaminated cell lines, all cell lines should be tested prior to injection. It is highly recommended to routinely monitor the presence of C. bovis by PCR from feces, maintain sterile housing conditions by vaporized hydrogen peroxide (VHP), and meticulously clean equipment with a disinfectant containing accelerated hydrogen peroxide (aHP) such as Oxiver (Miedel et al., 2018).
Another crucial parameter for the generation of humanized mice is the state of the HUSCs. Human CD34+ cells are isolated from cord blood mononuclear cells via positive immunomagnetic separation (Schumm et al., 1999) and should express CD34+ to ≥90%. HUSCs are sensitive and diminish in their efficacy if not thawed properly (see Support Protocol 1).
Host mouse strains
Many different strains of immunodeficient mice have been developed that are based on SCID mice or the understanding derived from this strain that RAG1 and RAG2 are crucial for B cell and T cell receptor development. Nonobese diabetic/severe combined immunodeficient (NOD/SCID) mice lack functional B cells and T cells and have reduced NK cell activity (Shultz et al., 1995). The creation of NOD.Cg-Prkdc scid Il2rgtm1Wjl /SzJ (NSG) and BALB/c-Rag2–/–Il2rg–/– (BRG) mice that lack the IL2rγ gene has led to effective hosts for HUSC engraftment. The lack of the IL2rγ chain blocks NK cell development and results in further defects in innate immunity (Cao et al., 1995). The irradiation of mice before injection of HUSCs can pose an equipment problem depending on the institutional setup. Recently, a NOD.Cg-KitW-41J Tyr+ Prkdcscid Il2rgtm1Wjl /ThomJ (NB-SGW) mouse was developed to support engraftment of HUSCs without irradiation (Jackson Laboratory, cat. no. 026622). This model is a cross between NOD.Cg -Prkdcscid Il2rgtm1Wjl /SzJ (NSG) and C57BL/6J-KitW-41J /J (C57BL/6. Kit W41). In our hands, the level of human lymphocytes in these mice after reconstitution with HUSCs was comparable to that in NSG mice after irradiation. NB-SGW mice also had 9× higher numbers of human lymphocytes compared to nonirradiated NSG mice at 12 weeks post engraftment (McIntosh et al., 2015). This model provides an excellent alternative to researchers who do not have access to an irradiation source.
Transgenic mice based on the immunodeficient NSG mouse model are a useful tool for investigating human diseases, host immune responses, and the effects of gene knockin and knockout (https://www.jax.org/jax-mice-and-services/find-and-order-jax-mice/nsg-portfolio). Immunodeficiency allows engraftment of HUSCs due to deficiencies in functional T, B, and NK cells, which can then be specialized for specific research needs by introduction of human genes. The Jackson Laboratory provides a plethora of transgenic mouse strains that are updated regularly. For example, they produce the NSG.HLA.A2 mouse (strain #014570) that is immunodeficient, expresses HLA class I heavy and light chains, and produces functional CTLs that are HLA.A2 specific. In addition, the transgenic mouse NSG-HLA-DQ8 (strain #026561) expresses HLA-DQ8 and has the knockout allele H2-Ab1. When these mice are humanized, they do not express mouse MHC class II but do produce a human insulin–specific FOXP3+ Treg response to insulin mimetopes (Serr et al., 2016). Another useful option is the NSGS mouse (#:013062), which is immunodeficient and carries human IL-3, GM-CSF, and SCF. These human transgenes aid in the development of myeloid and regulatory T cells (Coughlan et al., 2016).
Host age
An alternative to neonatal engraftment of HUSCs is engraftment in adult mice. When deciding the appropriate age for engraftment, one must consider the time and cost advantages of managing and engrafting mice. Neonatal engraftment can be optimal because pups stay with the parents until weaning, reducing per diem housing costs. The disadvantage of neonatal mice is that they must be injected within 24-72 hr after birth (Brehm et al., 2010) and cannibalism is more widespread than with adults. Nonetheless, we have been successful at humanizing NSG mice at 72 hr after birth. It has been reported that engrafted neonatal NSG mice support human T cell development better than engrafted adults (Brehm et al., 2010), but we have not found a difference in T cell development between mice humanized at birth versus 4 weeks of age. Engraftment of 4-week-old animals may be useful when additional manipulations are necessary. Another consideration may be the maturation of the animals. After infection with HTLV-1, mice humanized at birth succumbed faster to leukemia development than mice humanized at 4 weeks of age (Phelps and Niewiesk, unpublished data).
Alternate engraftment approaches
Engraftment methods differ between neonatal and adult mice. Basic Protocol 1 describes intrahepatic injections (Traggiai et al., 2004) in neonates, but other routes for neonates include intraperitoneal (Gimeno et al., 2004), intravenous via the facial vein (Ishikawa et al., 2002), and intracardiac (Brehm et al., 2010). Humanizing adult immunodeficient mice can be done by intravenous (Shultz et al., 2005) and intratibial injections.
Anticipated Results
Neonatal HIS mice
Human peripheral blood lymphocytes (HPBLs) are detectable in the blood of HUSC-engrafted neonatal mice via flow cytometry. Human CD45+ cells are detectable as early as 4 weeks post-inoculation. The earliest time at which a human T cell population is detectable is 10-12 weeks post-inoculation. If engraftment was successful, the percentage of human CD45+ cells is 10%-60% of the total white blood cells at this time point. The remaining cells are mouse cells (mostly macrophages and neutrophils) and can be summarily stained with antibodies against mouse CD45 or lineage-specific markers.
Adult HIS mice
For humanization of adult mice, different strains may be used and we have been successful with both NSG and NSG.HLA-A2 mice. Human CD45+ cells are detected by flow cytometry and are routinely measured at 8 weeks after humanization. In successfully engrafted mice, human CD45+ cells comprise 10%-55% of the total leukocyte population. There is no significant difference between NSG mice and a transgenic mouse strain (NSG.HLA-A2) in our experiments. T lymphocytes are present at this point but make up less than 10% of the total population. Most leukocytes in NSG mice are human B cells (∼80%), with the remaining population being composed of mouse leukocytes.
Time Considerations
The time required to inject and engraft neonatal NSG mice depends on litter size, stem cell preparation, irradiation, injection, and the time needed for human cells to develop. Breeder mice must be monitored during the gestation period (∼21 days). Before preparation of HUSCs, it is crucial to check the pups for the presence of the milk spot (which indicates that pups are viable) and for the absence of cannibalism. Preparation of injection of HUSCs can be accomplished in ∼4 hr. First, preparation of the cells takes ∼2-3 hr. Pups are then exposed to radiation at 1 Gy to aid in HUSC engraftment. The irradiation time will depend on the emission of the radiation source. Most irradiators require <5 min for a 1-Gy exposure. After anesthetization on ice (∼5 min), an experienced researcher can complete intrahepatic injections for a litter of eight pups in ∼8 min. Pups are then returned to their parents and the development of human cells can be verified after 10-12 weeks post-inoculation.
For 4-week-old mice, there are a few differences in the amount of time required for humanization. Mice are used at 4 weeks of age for easier injection of the tail vein. Irradiation takes only 2 min, but the animals must be warmed for 30 min before injection. An experienced researcher can complete injections for ten mice in ∼30 min. After humanization, it takes 8 weeks for human leukocytes to develop and become detectable in the mouse.
Acknowledgments
This work was supported by the National Cancer Institute through P01CA100730 Retrovirus Models of Cancer (PI Patrick Green, Director of Animal Model Core, Stefan Niewiesk).
Author Contributions
Cameron Phelps : Investigation, methodology, writing (original draft); Devra D. Huey : Methodology; Stefan Niewiesk : Conceptualization, funding acquisition, investigation, supervision, writing (review and editing).
Conflict of Interest
The authors declare no conflict of interest.
Open Research
Data Availability Statement
This manuscript details procedures. No data are available.
Literature Cited
- Ali, N., Flutter, B., Rodriguez, R. S., Sharif-Paghaleh, E., Barber, L. D., Lombardi, G., & Nestle, F. O. (2012). Xenogeneic graft-versus-host-disease in NOD-scid IL-2Rγnull mice display a T-effector memory phenotype. PLoS One , 7(8), e44219. https://doi.org/10.1371/journal.pone.0044219
- Billerbeck, E., Barry, W. T., Mu, K., Dorner, M., Rice, C. M., & Ploss, A. (2011). Development of human CD4+ FoxP3+ regulatory T cells in human stem cell factor, granulocyte-macrophage colony-stimulating factor, and interleukin-3-expressing NOD-SCID IL2Rγnull humanized mice. Blood , 117(11), 3076–3086. https://doi.org/10.1182/blood-2010-08-301507
- Brehm, M. A., Cuthbert, A., Yang, C., Miller, D. M., DiIorio, P., Laning, J., Burzenski, L., Gott, B., Foreman, O., Kavirayani, A., Herlihy, M., Rossini, A. A., Shultz, L. D., & Greiner, D. L. (2010). Parameters for establishing humanized mouse models to study human immunity: Analysis of human hematopoietic stem cell engraftment in three immunodeficient strains of mice bearing the IL2rγnull mutation. Clinical Immunology , 135(1), 84–98. https://doi.org/10.1016/j.clim.2009.12.008
- Cao, X., Shores, E. W., Hu-Li, J., Anver, M. R., Kelsail, B. L., Russell, S. M., Drago, J., Noguchi, M., Grinberg, A., Bloom, E. T., Paul, W. E., Katz, S. I., Love, P. E., & Leonard, W. J. (1995). Defective lymphoid development in mice lacking expression of the common cytokine receptor γ chain. Immunity , 2(3), 223–238. https://doi.org/10.1016/1074-7613(95)90047-0
- Coughlan, A. M., Harmon, C., Whelan, S., O'Brien, E. C., O'Reilly, V. P., Crotty, P., Kelly, P., Ryan, M., Hickey, F. B., O'Farrelly, C., & Little, M. A. (2016). Myeloid Engraftment in humanized mice: Impact of granulocyte-colony stimulating factor treatment and transgenic mouse strain. Stem Cells and Development , 25(7), 530–541. https://doi.org/10.1089/scd.2015.0289
- Foreman, O., Kavirayani, A., Griffey, S., Reader, R., & Shultz, L. (2011). Opportunistic bacterial infections in breeding colonies of the NSG mouse strain. Veterinary Pathology , 48(2), 495–499. https://doi.org/10.1177/0300985810378282
- Gimeno, R., Weijer, K., Voordouw, A., Uittenbogaart, C. H., Legrand, N., Alves, N. L., Wijnands, E., Blom, B., & Spits, H. (2004). Monitoring the effect of gene silencing by RNA interference in human CD34+ cells injected into newborn RAG2-/- γc-/- mice: Functional inactivation of p53 in developing T cells. Blood , 104(13), 3886–3893. https://doi.org/10.1182/blood-2004-02-0656
- Holyoake, T. L., Nicolini, F. E., & Eaves, C. J. (1999). Functional differences between transplantable human hematopoietic stem cells from fetal liver, cord blood, and adult marrow. Experimental Hematology , 27(9), 1418–1427. https://doi.org/10.1016/S0301-472X(99)00078-8
- Huang, J., Li, X., Coelho-dos-Reis, J. G., Wilson, J. M., & Tsuji, M. (2014). An AAV vector-mediated gene delivery approach facilitates reconstitution of functional human CD8+ T cells in mice. PLoS One , 9(2), e88205. https://doi.org/10.1371/journal.pone.0088205
- Huang, J., Li, X., Coelho-Dos-Reis, J. G., Zhang, M., Mitchell, R., Nogueira, R. T., Tsao, T., Noe, A. R., Ayala, R., Sahi, V., Gutierrez, G. M., Nussenzweig, V., Wilson, J. M., Nardin, E. H., Nussenzweig, R. S., & Tsuji, M. (2015). Human immune system mice immunized with Plasmodium falciparum circumsporozoite protein induce protective human humoral immunity against malaria. Journal of Immunological Methods , 427, 42–50. https://doi.org/10.1016/j.jim.2015.09.005
- Huey, D. D., Bolon, B., la Perle, K. M. D., Kannian, P., Jacobson, S., Ratner, L., Green, P. L., & Niewiesk, S. (2018). Role of wild-type and recombinant human T-cell leukemia viruses in lymphoproliferative disease in humanized NSG mice. Comparative Medicine , 68(1), 4–14.
- Ishikawa, F., Livingston, A. G., Wingard, J. R., Nishikawa, S.-I., & Ogawa, M. (2002). An assay for long-term engrafting human hematopoietic cells based on newborn NOD/SCID/β2-microglobulinnull mice. Experimental Hematology , 30(5), 488–494. https://doi.org/10.1016/S0301-472X(02)00784-1
- Ishikawa, F., Yasukawa, M., Lyons, B., Yoshida, S., Miyamoto, T., Yoshimoto, G., Watanabe, T., Akashi, K., Shultz, L. D., & Harada, M. (2005). Development of functional human blood and immune systems in NOD/SCID/IL2 receptor γ chainnull mice. Blood , 106(5), 1565–1573. https://doi.org/10.1182/blood-2005-02-0516
- Lan, P., Tonomura, N., Shimizu, A., Wang, S., & Yang, Y.-G. (2006). Reconstitution of a functional human immune system in immunodeficient mice through combined human fetal thymus/liver and CD34+ cell transplantation. Blood , 108(2), 487–492. https://doi.org/10.1182/blood-2005-11-4388
- McIntosh, B. E., Brown, M. E., Duffin, B. M., Maufort, J. P., Vereide, D. T., Slukvin, I. I., & Thomson, J. A. (2015). Nonirradiated NOD,B6.SCID Il2rγ-/-KitW41/W41 (NBSGW) mice support multilineage engraftment of human hematopoietic cells. Stem Cell Reports , 4(2), 171–180. https://doi.org/10.1016/j.stemcr.2014.12.005
- Miedel, E. L., Ragland, N. H., & Engelman, R. W. (2018). Facility-wide eradication of Corynebacterium bovis by using PCR-validated vaporized hydrogen peroxide. Journal of the American Association for Laboratory Animal Science: JAALAS , 57(5), 465–476. https://doi.org/10.30802/AALAS-JAALAS-17-000135
- National Research Council. (2003). Guidelines for the care and use of mammals in neuroscience and behavioral research. National Academies Press.
- Pearson, T., Greiner, D. L., & Shultz, L. D. (2008). Creation of “humanized” mice to study human immunity. Current Protocols in Immunology , 81, 15.21.1–15.21.21. https://doi.org/10.1002/0471142735.im1521s81
- Schumm, M., Lang, P., Taylor, G., Kuci, S., Klingebiel, T., Buhring, H. J., Geiselhart, A., Niethammer, D., & Handgretinger, R. (1999). Isolation of highly purified autologous and allogeneic peripheral CD34+ cells using the CliniMACS device. Journal of Hematotherapy , 8(2), 209–218. https://doi.org/10.1089/106161299320488
- Serr, I., Fürst, R. W., Achenbach, P., Scherm, M. G., Gökmen, F., Haupt, F., Sedlmeier, E. M., Knopff, A., Shultz, L., Willis, R. A., Ziegler, A. G., & Daniel, C. (2016). Type 1 diabetes vaccine candidates promote human Foxp3(+) Treg induction in humanized mice. Nature Communications , 7, 10991. https://doi.org/10.1038/ncomms10991
- Shultz, L. D., Lyons, B. L., Burzenski, L. M., Gott, B., Chen, X., Chaleff, S., Kotb, M., Gillies, S. D., King, M., Mangada, J., Greiner, D. L., & Handgretinger, R. (2005). Human lymphoid and myeloid cell development in NOD/LtSz-scid IL2Rγnull mice engrafted with mobilized human hemopoietic stem cells. The Journal of Immunology , 174(10), 6477–6489. https://doi.org/10.4049/jimmunol.174.10.6477
- Shultz, L. D., Saito, Y., Najima, Y., Tanaka, S., Ochi, T., Tomizawa, M., Doi, T., Sone, A., Suzuki, N., Fujiwara, H., Yasukawa, M., & Ishikawa, F. (2010). Generation of functional human T-cell subsets with HLA-restricted immune responses in HLA class I expressing NOD/SCID/IL2rγnull humanized mice. Proceedings of the National Academy of Sciences of the United States of America , 107(29), 13022–13027. https://doi.org/10.1073/pnas.1000475107
- Shultz, L. D., Schweitzer, P. A., Christianson, S. W., Gott, B., Schweitzer, I. B., Tennent, B., McKenna, S., Mobraaten, L., Rajan, T. V., & Greiner, D. L. (1995). Multiple defects in innate and adaptive immunologic function in NOD/LtSz-scid mice. The Journal of Immunology , 154(1), 180–191.
- Strowig, T., Rongvaux, A., Rathinam, C., Takizawa, H., Borsotti, C., Philbrick, W., Eynon, E. E., Manz, M. G., & Flavell, R. A. (2011). Transgenic expression of human signal regulatory protein alpha in Rag2–/– γc–/– mice improves engraftment of human hematopoietic cells in humanized mice. Proceedings of the National Academy of Sciences of the United States of America , 108(32), 13218–13223. https://doi.org/10.1073/pnas.1109769108
- Traggiai, E., Chicha, L., Mazzucchelli, L., Bronz, L., Piffaretti, J.-C., Lanzavecchia, A., & Manz, M. G. (2004). Development of a human adaptive immune system in cord blood cell-transplanted mice. Science , 304(5667), 104–107. https://doi.org/10.1126/science.1093933
- Vedder, A. R., Miedel, E. L., Ragland, N. H., Balasis, M. E., Letson, C. T., Engelman, R. W., & Padron, E. (2019). Effects of Corynebacterium bovis on engraftment of patient-derived chronic-myelomonocytic leukemia cells in NSGS mice. Comparative Medicine , 69(4), 276–282. https://doi.org/10.30802/AALAS-CM-18-000138
- Villaudy, J., Wencker, M., Gadot, N., Gillet, N. A., Scoazec, J.-Y., Gazzolo, L., Manz, M. G., Bangham, C. R., & Dodon, M. D. (2011). HTLV-1 propels thymic human T cell development in “human immune system” Rag2-/- gamma c-/- mice. PLoS Pathogens , 7(9), e1002231. https://doi.org/10.1371/journal.ppat.1002231
- Willinger, T., Rongvaux, A., Takizawa, H., Yan-copoulos, G. D., Valenzuela, D. M., Murphy, A. J., Auerbach, W., Eynon, E. E., Stevens, S., Manz, M. G., & Flavell, R. A. (2011). Human IL-3/GM-CSF knock-in mice support human alveolar macrophage development and human immune responses in the lung. Proceedings of the National Academy of Sciences of the United States of America , 108(6), 2390–2395. https://doi.org/10.1073/pnas.1019682108
Internet Resources
NSG mouse strains __
* <https://www.jax.org/strain/05557>
* <https://www.jax.org/strain/026622>
* <https://www.jax.org/strain/026561>
* <https://www.jax.org/strain/014570>
* <https://www.jax.org/strain/013062>
HUSCs __
* <https://www.lonza.com/products-services/bio-research/primary-cells/hematopoietic-cells/cord-blood-cells/cd34.aspx>
Videos __
* <https://www.youtube.com/watch?v=niTVnEAHOko>
Submandibular bleeding technique.
* <https://www.youtube.com/watch?v=8TqphNj6-z0>
Tail vein injection.