Murine Norovirus: Additional Protocols for Basic and Antiviral Studies
Stefan Taube, Christiane E. Wobus, Christiane E. Wobus, Amy M. Peiper, Amy M. Peiper, Alice M. McSweeney, Alice M. McSweeney, Vivienne L. Young, Vivienne L. Young, Maryna Chaika, Maryna Chaika, Miranda Sophie Lane, Miranda Sophie Lane, Marit Lingemann, Marit Lingemann, Joshua M. Deerain, Joshua M. Deerain, Madison S. Strine, Madison S. Strine, Mia Madel Alfajaro, Emily W. Helm, Stephanie M. Karst, Jason M. Mackenzie, Vernon K. Ward, Craig B. Wilen
baculovirus expression
circular polymerase extension reaction
murine norovirus
neonatal infections
tuft cell-enriched murine intestinal enteroid culture
Abstract
Murine norovirus (MNV) is a positive-sense, plus-stranded RNA virus in the Caliciviridae family. Viruses in this family replicate in the intestine and are transmitted by the fecal-oral route. MNV is related to the human noroviruses, which cause the majority of nonbacterial gastroenteritis worldwide. Given the technical challenges in studying human norovirus, MNV is often used to study mechanisms in norovirus biology since it combines the availability of a cell culture and reverse genetics system with the ability to study infection in the native host. Adding to our previous protocol collection, here we describe additional techniques that have since been developed to study MNV biology. © 2023 The Authors. Current Protocols published by Wiley Periodicals LLC.
Basic Protocol 1 : Indirect method for measuring cell cytotoxicity and antiviral activity
Basic Protocol 2 : Measuring murine norovirus genome titers by RT-qPCR
Support Protocol 1 : Preparation of standard
Basic Protocol 3 : Generation of recombinant murine norovirus with minimal passaging
Basic Protocol 4 : Generation of recombinant murine norovirus via circular polymerase extension reaction (CPER)
Basic Protocol 5 : Expression of norovirus NS1-2 in insect cell suspension cultures using a recombinant baculovirus
Support Protocol 2 : Isotope labelling of norovirus NS1-2 in insect cells
Support Protocol 3 : Purification of the norovirus NS1-2 protein
Support Protocol 4 : Expression of norovirus NS1-2 in mammalian cells by transduction with a recombinant baculovirus
Basic Protocol 6 : Infection of enteroids in transwell inserts with murine norovirus
Support Protocol 5 : Preparation of conditioned medium for enteroids culture
Support Protocol 6 : Isolation of crypts for enteroids generation
Support Protocol 7 : Enteroid culture passaging and maintenance
Basic Protocol 7 : Quantification of murine norovirus-induced diarrhea using neonatal mouse infections
Alternate Protocol 1 : Intragastric inoculation of neonatal mice
Alternate Protocol 2 : Scoring colon contents
INTRODUCTION
Murine norovirus (MNV) is a small non-enveloped virus with a plus-sense RNA genome ∼7.5 kb in length that infects laboratory and wild mice (Farkas et al., 2012; Karst & Wobus, 2015; Smith et al., 2012; Thackray et al., 2007). MNV infections can be acute or persistent depending on the virus strain. The first MNV strain, MNV-1, to be isolated causes an acute infection in immunocompetent mice that is characterized by infection of immune cells starting in the small intestine, minimal fecal shedding, and viral clearance from tissues within 1 to 2 weeks (Gonzalez-Hernandez et al., 2014; Grau et al., 2017; Karst et al., 2003). The persistent MNV strains (e.g., MNV-CR6, MNV-CR3) initiate infection in the large intestine and replicate in tuft cells from which they are shed into the stool for weeks to months (Gonzalez-Hernandez et al., 2014; Nice et al., 2013; Wilen et al., 2018). All MNV strains belong to genogroup V in the norovirus genus in the Caliciviridae family (Wobus & Green, 2020). These viruses are related to human noroviruses and share a number of biological characteristics, including fecal-oral spread and intestinal infections. Research on human noroviruses has been technically challenging given the historic lack of cell culture models (Duizer et al., 2004). The discovery of MNV in mice, development of cell culture and reverse genetics systems provided the field with a versatile system to study norovirus biology (Karst et al., 2003; Ward et al., 2007; Wobus et al., 2004). Although significant progress has been made in the development of laboratory cultivation systems for human norovirus (Estes et al., 2019; Ettayebi et al., 2016; Van Dycke et al., 2019), significant challenges remain, including the inability to generate a cell culture-derived virus stock for their molecular study. Thus, MNV continues to provide a powerful tool for the study of norovirus-host interactions and pathogenesis.
The following protocols present an update to our 2014 protocol collection (Hwang et al., 2014) and describe updated and recently developed methods to study various aspects of MNV biology. The protocols begin with a description of how to measure antiviral efficacy of compounds using an indirect measure of cell viability (Basic Protocol 1). We then provide an updated protocol to quantify MNV genome copies by RT-qPCR (Basic Protocol 2 and Support Protocol 1). This is followed by a recently developed method for the rapid recovery of MNV directly from virus stocks without the need for bacterial cloning of the viral genome, namely circular polymerase extension reaction (CPER) (Basic Protocol 3). Much remains to be learned about the function of individual viral proteins. Thus, the next set of protocols describes the expression, labelling, and purification of norovirus NS1-2 using a recombinant baculovirus (Basic Protocol 4, Support Protocols 2-4) and provides a blueprint for studying other viral proteins. While the ability of MNV to infect immune cells was the basis for the first cell culture system (Wobus et al., 2004), the discovery of a tropism of persistent MNV strains for intestinal tuft cells is relatively recent (Wilen et al., 2018). To study the interaction of MNV with tuft cells, Basic Protocol 5 and Support Protocols 5-7 describe the generation of intestinal enteroids and tuft-cell enriched enteroids cultures for MNV infection experiments. The unit ends with the MNV diarrhea model and a description of how to infect neonatal mice and quantify diarrhea (Basic Protocol 6, Alternate Protocols 1-2).
CAUTION : Murine norovirus is a Biosafety Level 2 (BSL-2) pathogen in some countries (e.g., USA). Follow all appropriate guidelines and regulations for the use and handling of pathogenic microorganisms.
NOTE : All protocols involving animals must be reviewed and approved by the appropriate Animal Care and Use Committee and must follow regulations for the care and use of laboratory animals.
NOTE : All solutions and equipment coming into contact with living cells must be sterile, and aseptic techniques should be used accordingly.
NOTE : All culture incubations should be performed in a humidified 37°C, 5% CO2 incubator unless otherwise specified.
Basic Protocol 1: INDIRECT METHOD FOR MEASURING CELL CYTOTOXICITY AND ANTIVIRAL ACTIVITY
The assessment of antiviral activity for putative antiviral drugs in the development pipeline facilitates the discovery of new antiviral compounds. Screening of compound libraries for both 50% cytotoxicity concentrations (CC50) and effective concentrations (EC50) is an important step to ascertain the effectiveness of compounds and to assess the medicinal chemistry path being followed. This process is facilitated by a rapid first screen of antiviral activity that can then be followed up with more expensive or intensive methods that directly quantify the virus, such as qRT-PCR (see Basic Protocol 2) or 50% tissue culture infectious dose (TCID50; Basic Protocol 5 in Hwang et al., 2014). Several methods have been developed to calculate the TCID50 and have been summarized recently (Lei et al., 2021), where the authors also provide a spread-sheet calculator as an electronic supplementary material.
Here, we present a method for indirectly assessing cell viability in the presence of MNV and antiviral compounds using CellTiter-Glo (Promega, WI, USA). The reagent lyses cells to release ATP which is then converted to a stable luminescent signal proportional to the health of the cells at the time of harvest. The sensitivity and linear range of CellTiter-Glo allow for the detection of subtle changes in the cells or virus in response to an antiviral that may go undetected with other reagents. The reagent does not require fixing or washing of cells, thereby significantly limiting loss of cells that lift off the plate during infection. This protocol generates both CC50 and EC50 values in technical triplicate on one 96-well plate. This is achieved by seeding plates with an MNV permissible cell line (Haga et al., 2016; Lingemann, 2020; Orchard et al., 2016; Wobus et al., 2004), treating with a dilution series of the antiviral, and infecting with MNV. The plate is incubated for 24 hr until 90% of virally infected control cells are dead, followed by the addition of the CellTiter-Glo reagent. We also illustrate the utility of indirect measurements of virus replication to observe unexpected effects that compound carriers such as dimethyl sulfoxide (DMSO) can have on norovirus infection.
Materials
-
RAW 264.7 cells (ATCC no. TIB-71)
-
DMEM-GlutaMAX-10 medium (see recipe)
-
Huh7mCD300lf cells (a gift from Prof S. Taube, University Lübeck) (Lingemann, 2020)
-
2’-C-methylcytidine (2-CMC; reference antiviral) (Sigma-Aldrich, cat. no. M4949)
-
MNV-1 virus stock (ATCC no. VR-1937)
-
DMSO (Sigma-Aldrich, cat. no. D5879)
-
CellTiter-Glo luminescent cell viability assay (Promega, cat. no. G8461)
-
75-cm2 flask
-
Cell scraper
-
Cell counter
-
96-well plate, white flat bottom (Greiner Bio-One, cat. no. 655083)
-
Multichannel pipette (capable of volumes up to 100 μl)
-
Filtered pipette tips
-
Orbital plate rocker
-
Incubator, 37°C, 5% CO2
-
96-well plate, clear flat bottom
-
Plate reader capable of reading luminescent signals in a 96-well plate format
Preparation of cells in the test plate
1.Take a 75-cm2 flask with RAW 264.7 cells that have reached 70%-80% confluence.
2.Scrape, count and collect the cells.
3.Seed the test plate (96-well white flat-bottom) with 2 × 104 RAW264.7 cells/well in 100 μl DMEM-GlutaMAX-10 using a multichannel pipette and filtered tips.
4.Gently rock the plate to ensure an even monolayer of cells.
5.Incubate the plates overnight at 37°C, 5% CO2.
Setting up the dilution plate (see Fig. 1A)
6.Prepare inhibitor dilutions in a 96-well round-bottom plate at 4× the final concentration.
7.Add 50 μl DMEM-GlutaMAX-10 to all wells of the dilution plate, except column 2 rows B-G.
8.Add 100 μl 2-CMC at 600 μM to column 2 rows B-G.
9.Using a multichannel pipette, perform a 2-fold serial dilution of the inhibitor, transferring 50 μl from column 2 into column 3.
10.Mix gently up and down three times and repeat the dilution series down to column 10, mix and discard 50 μl from the last column.
Addition of antiviral and MNV to the test plate (see Fig. 1B)
11.Remove 50 μl from wells in columns 2-11 rows B-D of the test plate.
12.Remove 25 μl from wells in columns 2-11 rows E-G of the test plate.
13.Using a multichannel pipette transfer 25 μl of inhibitor from the dilution plate into the respective wells of the test plate.
14.Infect cells with MNV at a final MOI of 0.1 by adding 25 μl virus to columns 2-11 rows B-D of the test plate.
15.Incubate the plate at 37°C, 5% CO2 for ∼24 hr until 90-95% cell death (5%-10% cell viability) is observed in the virus-only control wells (Fig. 2B).
Indirect measurement of cell viability using CellTiter-Glo
16.Thaw CellTiter-Glo reagent and equilibrate the cell plate for 20 min at room temperature.
17.Using a multichannel pipette, add 100 μl CellTiter-Glo reagent to each well.
18.Incubate plate on an orbital plate rocker at 200 RPM for 2 min to lyse cells.
19.Incubate the plate in the dark for 10 min to allow luminescent signal to develop.
20.Measure the luminescence on a plate reader.
21.Calculate the % cell viability.
Basic Protocol 2: MEASURING MURINE NOROVIRUS GENOME TITERS BY RT-qPCR
MNV levels can be assessed through reverse transcription quantitative polymerase chain reaction (RT-qPCR). This technique involves purifying viral RNA, reverse transcribing the RNA into complementary DNA (cDNA) using a reverse primer, and then exponentially amplifying the cDNA. RT-qPCR can be conducted as either a single reaction or in combination with quantitative PCR (qPCR). Ct values obtained from the qPCR values will correlate with the cDNA levels. Comparing the Ct values to a known MNV standard can be used to determine genome or cDNA equivalents.
Here, we describe an updated protocol based on primers and probe previously described for the quantification of murine norovirus genome (Taube et al., 2009). In this protocol, we use guanidinium thiocyanate-phenol-chloroform extraction, also known as TRIzol extraction because it quickly inhibits RNase activity and inactivates infectious virus. Coupled with Zymo-Spin columns this is an extremely fast and streamlined method to extract MNV genomes from a variety of starting materials, including but not limited to, cell culture lysates, homogenized tissues, or stool samples. Alternatively, silica-column based RNA extraction methods are also very reliable and described previously (Alternate Protocol 4 in Hwang et al., 2014). The RNA extraction should involve a DNase I treatment step to remove DNA contamination. After RNA extraction, a one-step RT-qPCR is performed to titrate the MNV cDNA. Linearized plasmid DNA and in vitro transcribed RNA are widely used as a standard and their respective advantages and disadvantages have been described previously (Hwang et al., 2014). The commercial availability of double-stranded gene fragments of sufficient length has now become an easy and highly reproducible alternative to linearized plasmid DNA.
Materials
-
RNase decontamination solution (15% bleach, 0.05% SDS, 1% NaOH)
-
H2O, nuclease-free
-
70% and 100% ethanol, nuclease-free
-
Direct-zol RNA miniprep kit with TRI reagent (Zymo Research, cat. no. R2051)
-
iTaq universal probes one-step kit (BioRad, cat. no. 1725140)
-
Forward primer (5’ to 3’; desalted and/or HPLC purified):
- GTGCGCAACACAGAGAAACG
-
Reverse Primer (5’ to 3’; desalted and/or HPLC purified):
- CGGGCTGAGCTTCCTGC
-
Probe modified at the 5′-end with a 6-carboxyfluorescein (FAM) and at the 3′-end with a quencher, e.g., black hole quencher 1 (BHQ1) (5’ to 3’; HPLC purified):
-
[6-FAM]-CTAGTGTCTCCTTTGGAGCACCTA-[BHQ1]
-
MNV-1 gBlock (shown is the sequence of the top strand 5’ to 3’; generate as double-stranded DNA):
- GTGCGCAACACAGAGAAACGCAAAAACAAGAAGGCTTCGTCTAAAGCTAGTGTCTCCTTTGGAGCACCTAGCCCCCTCTCTTCGGAGAGCGAAGACGAAATTAATTACATGACCCCTCCTGAGCAGGAAGCTCAGCCCG
-
Two sets of pipettors, one designated for handling RNA and master mix(es) and one designated for handling DNA
-
1000-, 100-, and 10-µl nuclease-free filter tips
-
1.5-ml microcentrifuge tubes, nuclease-free, designated for RNA or DNA/plasmid work
-
Ice
-
Microcentrifuge, 4°C
-
96-well PCR-plate, white, 100 µl volume (Sarstedt, cat. no. 72.1977.23)
-
Optical qPCR-film, DNase-/RNase-free, highly transparent (Sarstedt, cat. no. 95.1999)
-
Plate centrifuge
-
RT-qPCR machine
Viral RNA isolation
1.Prepare an RNA-designated workspace cleaned with RNase decontamination solution, incubate for at least 5 min, then rinse with nuclease-free H2O and nuclease-free 70% ethanol.
2.Extract viral RNA from your sample (i.e., cell culture lysate or homogenized tissue) with the direct-zol RNA miniprep kit with TRI reagent following the manufacturer's recommendations.
3.Elute or resuspend the RNA in 50 µl nuclease-free H2O. Use an RNase-free microcentrifuge tube.
RT-qPCR
The RT-qPCR is performed as previously described (see Alternate Protocol 4 in Hwang et al., 2014) using the iTaq universal probes one-step kit. Be aware that the optics of the qPCR machine need to be compatible with your qPCR master mix and the fluorophore used for the probe.
4.Plan the plate arrangement according to the number of samples, including standard dilutions, a no-template control (NTC) that serves as a general control for extraneous nucleic acid contamination, and a no transcriptase control (NRT) that assesses the amount of DNA contamination present in an RNA preparation.
5.Thaw reaction components on ice, mix them thoroughly and centrifuge briefly at maximum speed to collect the content at the bottom of the tubes.
6.Set-up the RT-qPCR reaction by calculating the amount of each component needed (see Table 1). A reaction volume of 20 µl is sufficient.
Component | Initial concentration | Final concentration | Volume | Master mix |
---|---|---|---|---|
1 reaction | 1 reaction | 100 reactions | ||
Master mix | 2× | 1× | 10 µl | 1000 µl |
Fwd primer | 25 µM | 0.3 µM | 0.24 µl | 24 µl |
Rev primer | 25 µM | 0.3 µM | 0.24 µl | 24 µl |
Probe | 25 µM | 0.125 µM | 0.1 µl | 10 µl |
RNA template | – | – | 5 µl | – |
iScript RT | 40× | – | 0.5 µl | 50 µl |
Nuclease-free H2O | – | – | 3.92 µl | 392 µl |
Total | – | – | 20 µl | 1500 µl |
Each | 15 µl |
- a
The volumes and components resemble the recommended reaction setup from BioRad One-Step RT-qPCR kit (cat. no. 1725140, 1725141 and 12013250). To account for pipetting error, a 100-reaction master mix is recommended for a complete 96-well plate.
7.Prepare a master mix in a 1.5-ml nuclease-free tube, containing each component except for the template. The NRT control-mix can be removed from the master mix before adding the reverse transcriptase.
8.Mix thoroughly and pipet 15 µl into the required wells of a 96-well PCR plate.
9.Pipet 5 µl of each sample RNA into the required well. Add nuclease-free H2O to NTC and NRT to match the total volume of the samples.
10.Add the standard last and make sure the standard contains at least six 10-fold dilutions of a control dsDNA (see Support Protocol 1 on preparing the standard).
11.Cover the plate with the optical qPCR film and centrifuge the plate briefly at maximum speed to collect all the volume at the bottom of each well.
12.Load the plate into the RT-qPCR machine and run the RT-qPCR reaction after programming the thermal cycler settings as follows:
- Reverse transcription for 25 min, 50°C
- Activation and initial denaturation for 5 min, 95°C
- 40 cycles of denaturation for 10 s, 94°C, and annealing and elongation for 30 s, 60°C
13.Perform data analysis according to the instrument's specific instructions.
Support Protocol 1: PREPARATION OF STANDARD
Depending on your application, you can use a standard derived from in vitro transcribed RNA, or dsDNA (double-stranded DNA). Both have their advantages and disadvantages. In vitro transcribed RNA needs to be generated, purified, and aliquots must be stored at –80°C. On the other hand, dsDNA standards are easily obtained and highly reproducible but lack the control step for reverse transcription. For dsDNA standards you can use plasmid DNA or commercial custom dsDNA fragments, such as gBlocks from Integrated DNA Technologies (IDT) that comprise the complete amplicon of the qPCR. If you use plasmid DNA, a linearization step followed by column-based purification method is highly recommended, as circular DNA is not as efficiently amplified during cycling because primer/probe binding may be impaired for super-coiled DNA. Copy numbers can be derived from the measured mass of the ssRNA (single-stranded RNA) or dsDNA molecule.
- a. Determine the concentration of the linearized plasmid by spectrophotometry and convert the readout to g/L. Accurate measurements are key here to obtain reproducible results.
- b. Calculate the molecular weight (MW) by multiplying the DNA size (in base-pairs) by 662 g/mol, which is the average weight of a nucleotide pair. Alternatively, online calculators can be used to calculate the precise MW of the DNA based on sequence (MW needs to be multiplied by 2 to account for dsDNA).
- Example: a gBlock corresponding to the size of the PCR amplicon of MNV-1 (139 bp) has a MW of 85,758 Da or 85.76 kDa.
- c. Calculate the molar concentration (M) of your linearized plasmid:
- M = mol/L = (mass [gram]/MW)/1 L
- Example: a gBlock of MNV-1 (139 bp) usually has a concentration of 3000 fM
- 3000 fM = 3 × 10-12 M
- d. The copy number (cp) can be calculated based on Avogadro's constant:
1 M is ∼6.02 × 1023 copies:
* cp = 1 M × 6.02 × 10²³
* cp = **3 × 10<sup>-12</sup>** M × 6.02 × 10²³
* cp = 1.8 × 10<sup>12</sup>
- e. The dsDNA is now adjusted to 2 × 108/µl
- In this example 1.8 × 1012 cp are resuspended in 9 ml nuclease-free H2O.
- f. The PCR pipetting scheme requires 5 µl template/standard. From a 2 × 108/µl stock this equals 1 × 109 copies per PCR well.
Additional Materials (also see Basic Protocol 2)
8-strip PCR tubes (optional)
1.Generate a standard curve by performing a series of at least six 10-fold serial dilution, changing tips between each dilution-step. A suitable dilution range for a standard set is from 107 to 102 copies per well.
2.Calculate the genome copies per well if the entire well was used in the RNA extraction. For this, consider that only 1/10 of your RNA extraction (5 µl out of 50 µl elution volume) was analyzed. To obtain the genome-equivalents per well (GE / well) multiply your GE reading from the qPCR machine with your dilution factor, in this case 10.
3.Calculate genome copies per ml if you have used a specific volume for the RNA extraction. Consider that only 1/10 of your RNA extraction (5 µl out of 50 µl elution volume) was analyzed. Additionally, consider the sample volume used in the lysis reaction, e.g., if you used 500 µl of a virus stock together with the TRI-reagent, multiply your copy numbers by 2 to bring it up to 1 ml. In total for this example, multiply by 20 to obtain GE/ml.
Basic Protocol 3: GENERATION OF RECOMBINANT MURINE NOROVIRUS WITH MINIMAL PASSAGING
Reverse genetics provides an invaluable technique for exploring norovirus biology, allowing for the introduction of specific mutations into a cDNA clone of the viral genome via site-directed mutagenesis (Liu & Naismith, 2008) and subsequent recovery of the recombinant virus through transfection of genomic cDNA or in vitro transcribed RNA into permissive cells. The original DNA-based reverse genetics protocol is based on a publication by Ward et al. (2007). An RNA-based protocol was later developed in the laboratory of Ian Goodfellow (Arias et al., 2012), which allowed minimal passaging by directly transfecting capped RNA transcripts into BV-2 cells using the specialized Neon electroporation system (Life Technologies). An account of the available DNA and RNA based reverse genetics systems has previously been summarized (see Basic Protocols 3 and 4 in Hwang et al., 2014).
Generation of infectious MNV from cDNA is technically challenging in naturally susceptible cell lines such as RAW264.7 cells or BV-2 due to difficulties in efficiently transfecting these cells. The identification of mCD300lf as an entry receptor for MNV (Orchard et al., 2016) has now enabled the generation of susceptible cell lines from any permissive cell line, thus overcoming the limitation in the choice of easily transfectable cell lines. Huh7 human hepatoma cells are easily transfectable with a variety of transfection reagents and have been transduced to stably express mCD300lf generating Huh7mCD300lf (Lingemann, 2020). Lentiviruses are the tool of choice for fast and efficient generation of stable homogeneous cell lines for gene expression (Zufferey et al., 1998). A protocol for the lentivirus vector generation (Zufferey et al., 1998) is available from the Trono lab website (Lentivector Toolbox, see Internet Resources). Here, we provide an updated RNA-based reverse genetics protocol for MNV (based on Arias et al., 2012) using Huh7mCD300lf cells with minimal passaging. The protocol can also be easily applied to cDNA from the Ward et al. (2007) system. Transfection of Huh7mCD300lf with MNV.CW1 wild type in vitro transcribed RNA typically yields between 1 × 105 and 1 × 107 TCID50/ml within 48 to 96 hr post transfection while DNA transfection typically yields ∼1 log less.
Additional Materials (also see Basic Protocols above)
-
MNV cDNA (pT7: MNV 3’Rz) plasmid (a gift from Prof I. Goodfellow, University of Cambridge)
-
NheI restriction enzyme (NEB, cat. no. R0131S)
-
MAXIscript T7 transcription kit (Thermo Fisher, cat. no. AM1314M), or similar
-
ScriptCap m7G capping system (CellScript, cat. no. C-SCCE0625) or faustovirus capping enzyme (NEB, cat. no. M2081L)
-
0.05% Trypsin in PBS with 0.02% EDTA (GENAXXON bioscience, cat. no. C4261.0110)
-
Cell culture medium (see recipe)
-
Opti-MEM I (Thermo Fisher, cat. no. 31985070)
-
Lipofectamine MessengerMAX for RNA-based transfection (Thermo Fisher, cat. no. LMRNA003)
-
Fugene HD transfection reagent for DNA-based transfection (Promega, cat. no. E2311)
-
BV-2 cells (a gift from Prof I. Goodfellow, University of Cambridge)
-
NanoDrop 2000 spectrophotometer, or similar
-
6-well cell culture plate
-
Additional reagents and equipment for phenol/chloroform extraction (see Current Protocols, 1994) and agarose gel electrophoresis (see Voytas, 2000).
Synthesis of infectious capped MNV transcripts
1.Digest 10-20 µg of the plasmid containing the wild-type MNV cDNA (pT7:MNV3’Rz) with NheI to obtain linear DNA. Table 2 shows a typical restriction setup for NheI digestion.
Components | Final concentration | Volume (µl) |
---|---|---|
10× CutSmart buffer (NEB) | 1× | 20 |
NheI-HF 20 U/µl (NEB) | 0.3 U/µl | 3 |
cDNA (pT7:MNV3’Rz) | 100 ng/µl | Variable |
ddH2O | - | add to 200 |
2.Incubate at 37°C for 2 hr. Keep at least 2 µl from the linearized DNA for later electrophoresis analysis.
3.Purify the linearized DNA by phenol/chloroform extraction, ensuring RNase-free conditions. Determine DNA concentration measuring the absorbance at 260 nm by spectrometry (e.g., NanoDrop).
4.Ensure complete linearization and condition of purified DNA by gel electrophoresis.
5.Perform the in vitro transcription from the purified, linearized DNA using T7 RNA polymerase from the MAXIscript T7 transcription kit (Invitrogen). Table 3 shows a typical reaction for the MAXIscript T7 transcription kit.
Reagent | Final concentration | Volume (µl) |
---|---|---|
10× buffer | 1× | 4 |
rNTPs (10 mM each) | 0.5 mM each | 8 (2 each) |
T7 enzyme (15 U/µl) | 0.75 U/µl | 2 |
Linear DNA | 100 ng/µl | (3-4 µg) ∼10-15 |
RNase-free ddH2O | – | Up to 40 |
6.Incubate at 37°C for 2 hr. Digest the DNA with 1 µl TURBO DNase I (MAXIscript T7 transcription kit, 2 U/µl) at 37°C for 30 min to ensure complete DNA digestion. Keep back at least 2 µl from the in vitro transcription for electrophoresis analysis.
7.Purify the RNA to remove unincorporated nucleotides, enzymes and other impurities using TRI reagent for RNA extraction (see step 2 of Viral RNA Isolation in Basic Protocol 2). Elute the RNA with 69 µl RNase-free-ddH2O by centrifugation, and determine RNA concentration and purity by measuring the absorbance at 260 nm by spectrometry (e.g., NanoDrop). Keep at least 2 µl RNA for electrophoresis analysis.
8.Perform capping with the ScriptCap m7G capping system. Alternatively, the faustovirus capping enzyme can be used. Cap the purified RNA by first denaturing at 65°C for 5-10 min and immediately store on ice. A typical ScriptCap reaction is shown in Table 4.
Reagent | Per 100 µl reaction | Notes |
---|---|---|
Denatured IVT RNA | (50-60 µg) 69 µl | |
10× capping buffer | 10 µl | Dissolve at 37°C, thaw at room temperature, vortex before use |
10 mM GTP | 10 µl | |
2 mM SAM | 5 µl | Dilute fresh from 20 mM stock |
RNase inhibitor | 2.5 µl | ScriptGuard RNase Inhibitor |
Capping enzyme | 4 µl | As little as 1 µl can also be used |
9.Incubate at 37°C for 1.5 hr. Reserve 2 µl for electrophoresis analysis (Fig. 4). Capped RNA can be used straight away or stored at −80°C.
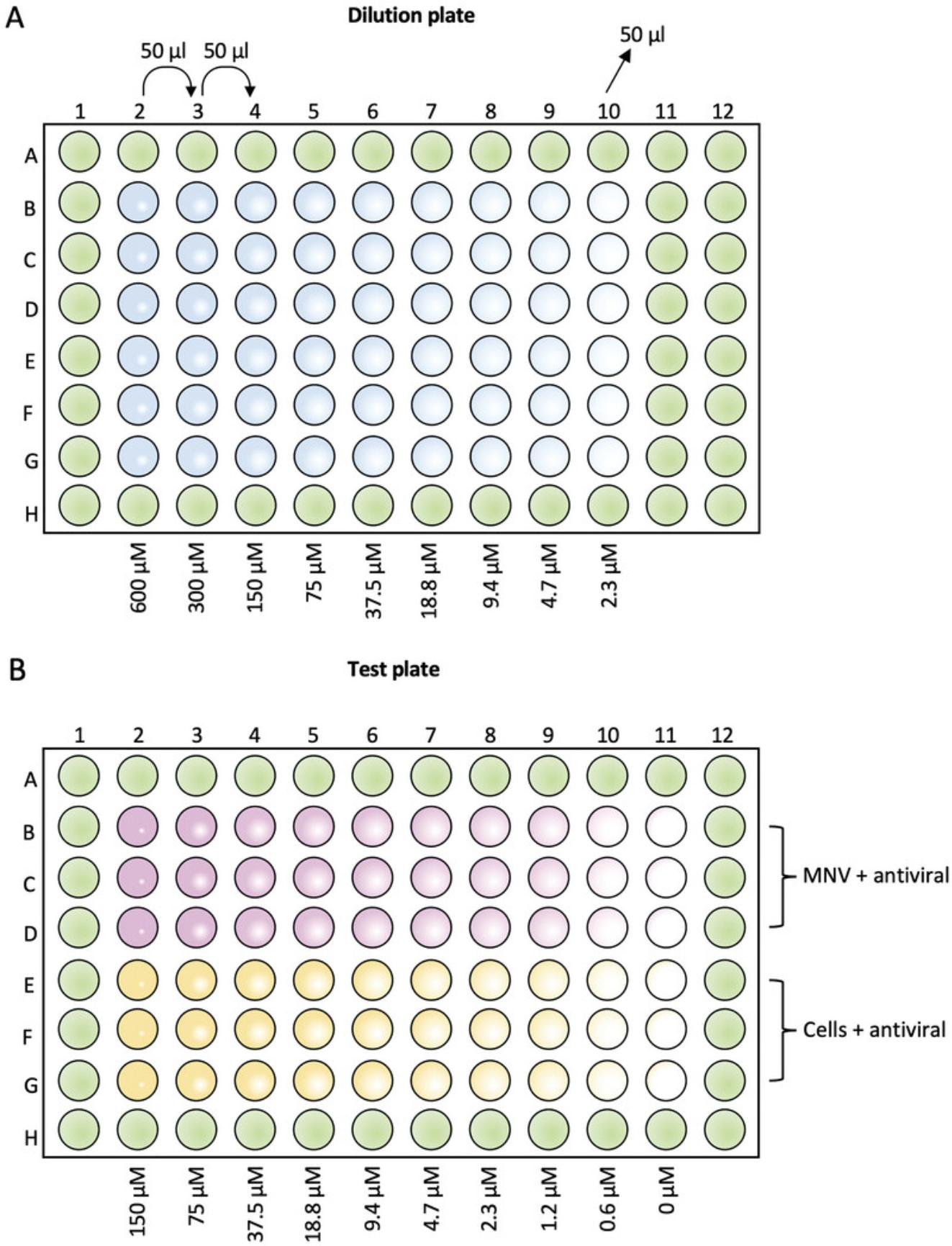
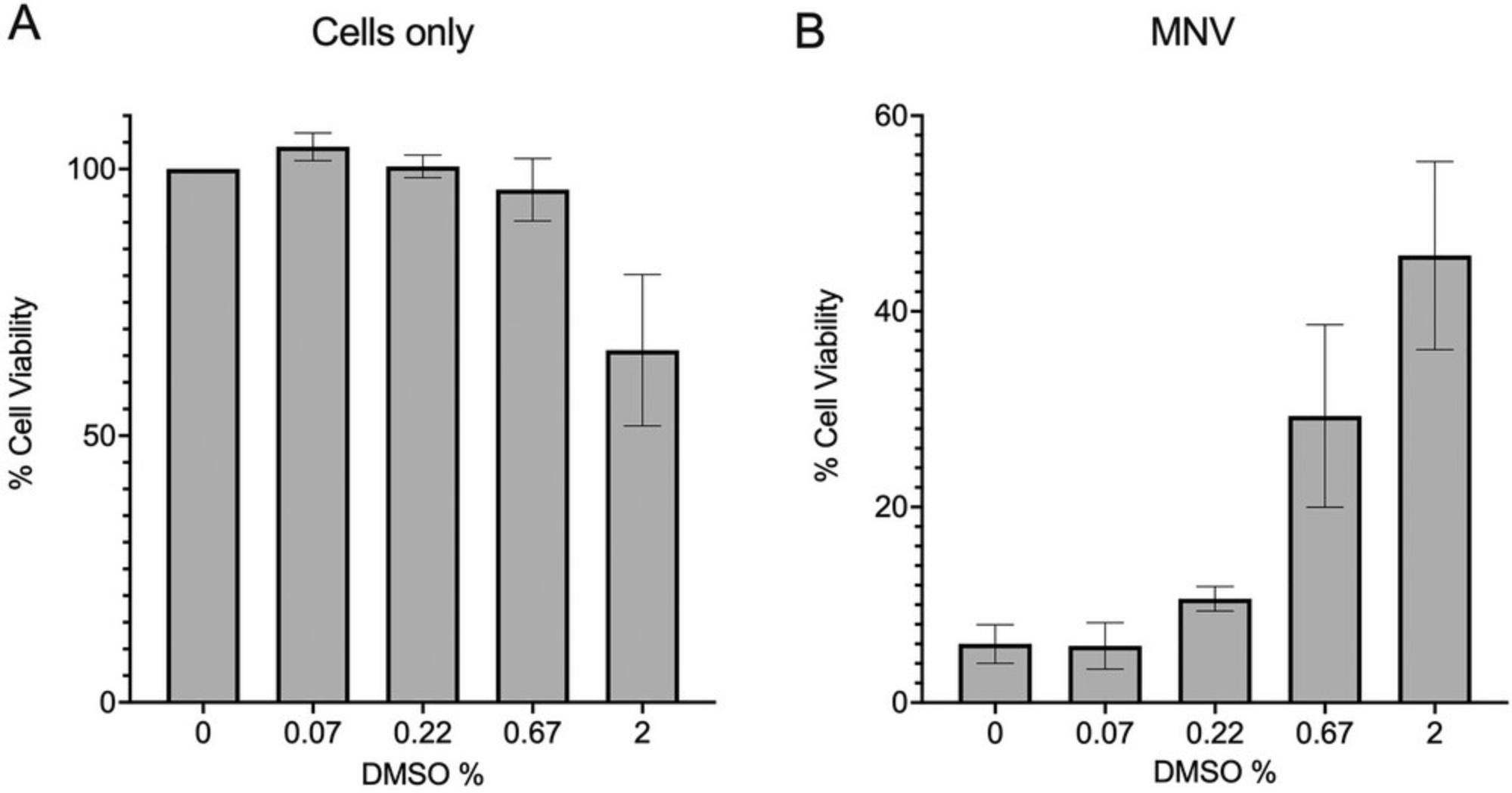
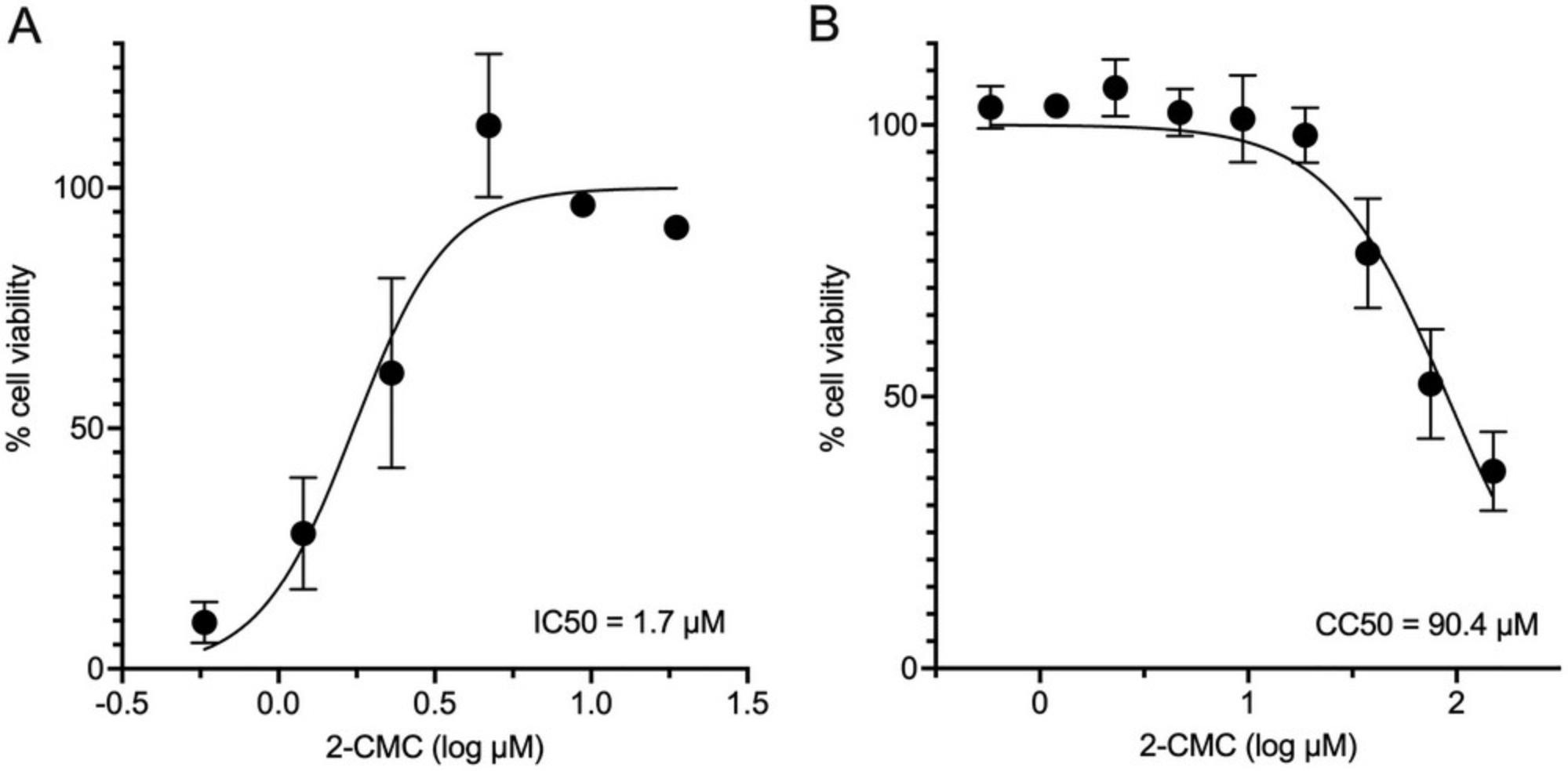
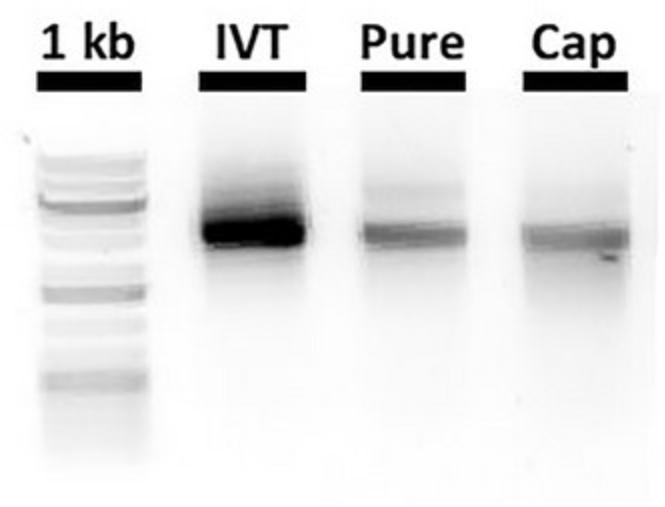
10.Run the in vitro transcribed, purified, and capped RNA samples together by gel electrophoresis (1% Agarose, 1× TAE) (Fig. 4). Run the electrophoresis at 120 V for only 10 min to avoid RNA degradation. Bands should be of equal size with decreasing intensity as the RNA becomes more dilute. Smears or multiple bands may indicate degradation at different steps but can be a result of the gel electrophoresis.
11.One day prior to transfection, trypsinize a monolayer of Huh7mCD300lf cells and seed 2 × 105 cells per well of a 6-well plate, in cell culture medium. Cells should be ∼70% confluent at the time of transfection.
12.Prepare an RNA- and a transfection-mix:
-
For the transfection-mix, add 3.75 µl Lipofectamine MessengerMAX to 100 µl Opti-MEM and incubate at room temperature for 10 min.
-
For the RNA-mix, add 2.5 µg RNA to 100 µl Opti-MEM (always thaw and store RNA on ice to avoid degradation).
13.Combine the RNA (or DNA) and transfection mix with a slight flick and incubate at room temperature for ∼5 min (but do not exceed 30 min). Other transfection reagents may have different incubation times, so adjust accordingly if needed.
14.Meanwhile, remove the cell culture medium from the seeded 6-well and replace with 800 µl Opti-MEM.
15.Add the ∼200 µl transfection mix to the cells, drop-wise (carefully allowing the transfection mix to drop directly onto the cells instead of the more common addition of into the well wall).
16.Incubate at 37°C, 5% CO2, 95% relative humidity (RH) for 3-4 hr.
17.Replace the Opti-MEM transfection with 2 ml cell culture medium.
18.Freeze both media and cells at 2 days post-transfection at −80°C. Use cell lysate for titration by end-point dilution assay or plaques assay.
19.Passage on standard BV-2 cells or RAW246.7 cells to expand the viral stock if necessary.
Basic Protocol 4: GENERATION OF RECOMBINANT MURINE NOROVIRUS VIA CIRCULAR POLYMERASE EXTENSION REACTION (CPER)
The use and utility of recombinant virus infectious clones and replicons has revolutionized our understanding of the molecular events of the intracellular replication of many (+)-sense RNA viruses. In addition, it has informed some attributes of protein function and guided the development of antiviral compounds and therapies. Many reverse genetics systems have been employed to derive authentic full-length copies of the MNV genome in addition to mutants and to some extent the development of reporter viruses (also seeo Basic Protocol 4 and Alternate Protocol 2 in Hwang et al., 2014). The protocol described within is founded on the publication by Amarilla et al. (2021). Essentially, we describe the assembly of PCR fragments encompassing the entire genome that are circularized with a linker containing a poly-A tail, hepatitis D virus ribozyme and a CMV promoter (see Fig. 5). This assembly is then transfected into NIH3T3 cells whereupon the full-length viral genome is transcribed by the host RNA pol II enzyme before translation and replication in the cytoplasm. In NIH3T3 cells this usually yields ∼103 pfu/ml of MNV which is subsequently transferred and amplified in RAW264.7 cells to generate ∼108 pfu/ml. The advantages of this approach are: (i) rapid recovery of virus, (ii) no requirement for cloning or transformation in bacterial cultures, and (iii) gene blocks can be synthesized from sequence alone to generate the fragments required for the CPER assembly.
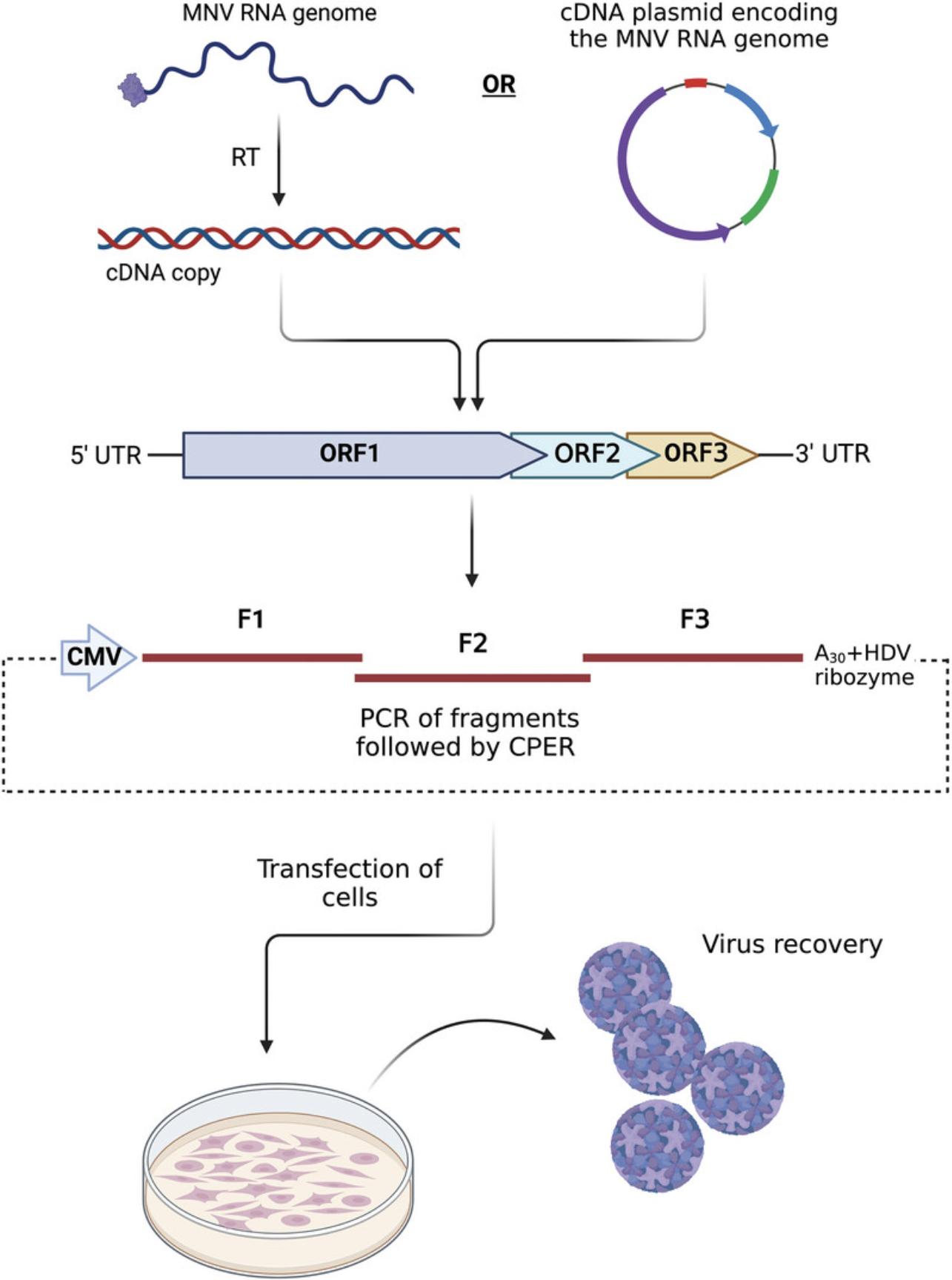
Materials
- High-fidelity PrimeSTAR GXL DNA polymerase (Takara Bio, cat. no. R050B)
- Primers (all listed as sequence 5′ to 3′):
Fragment 1 | MNV_Frag1.F | GTGAAATGAGGATGGCAACGCCATCTTC |
MNV_Frag1.R | CTGACACAGTTGACAAGTGTTTTGGGC | |
Fragment 2 | MNV_Frag2.F | GCCCAAAACACTTGTCAACTGTGTCAG |
MNV_Frag2.R | CACTCATCCTCATTCACAAAGACTGCTGAG | |
Fragment 3 | MNV_Frag3.F | CTCAGCAGTCTTTGTGAATGAGGATGAGTG |
MNV_Frag3.R | GCAGTAAGCAGAAATCATTTTCACAAAAGGTTTC | |
Linker fragment (full sequence below) | MNV_Linker.F | GAAACCTTTTGTGAAAATGATTTCTGCTTACTG |
MNV_Linker.R | GAAGATGGCGTTGCCATCCTCATTTCAC |
-
5× PrimeSTAR GXL buffer (Takara Bio, cat. no. R050B)
-
10 mM dNTP mix (Takara Bio, cat. no. R050B)
-
Full-length MNV-containing plasmid (pSPORT-T7-MNV1; received via a materials transfer agreement from Dr. Herbert Virgin)
-
Plasmid containing MNV linker sequence and HDV ribozyme (pUC19-MNV-CMV_Linker) (available from Dr. Jason Mackenzie)
-
Zymoclean gel DNA recovery kit (Zymo Research, cat. no. D4007)
-
NIH3T3 (ATCC CRL-1658) or HEK 293T (ATCC CRL-3216) cells growing in culture (low passage number works better)
-
Lipofectamine 3000 transfection reagent (Invitrogen, cat. no. L3000015)
-
Opti-MEM (Gibco, cat. no. 11058021)
-
Serum-free DMEM (Gibco, cat. no. 10567022) supplemented with 1% GlutaMAX (Gibco, cat. no. 35050061), and 1% sodium pyruvate (100 mM; Gibco, cat. no. 11360070)
-
DMEM supplemented with 4% FCS (Bovogen, cat. no. SFBS), 1% GlutaMAX, and 1% sodium pyruvate (100 mM)
-
DMEM supplemented with 10% FCS, 1% GlutaMAX, and 1% sodium pyruvate (100 mM; Gibco), referred to as complete medium
-
PCR tubes
-
Vortex
-
100-mm cell culture dish
-
15-ml conical centrifuge tubes (e.g., BD Falcon)
-
Benchtop centrifuge (Eppendorf 5424R)
-
Sequencing facility to confirm the MNV fragments (if necessary)
NOTE : All steps are carried out at room temperature. Media should be warmed to 37°C or equilibrated to room temperature.
CPER reaction
All primers are designed with a 27-33 bp overlap and approximately equal melting temperature (Tm) of 60°C. High-fidelity PrimeSTAR GXL DNA polymerase (Takara Bio) is used for all PCR amplifications.
1.PCR amplify CPER fragments in PCR tubes using primers listed in the materials list under the following conditions.
Reaction setup:
5× PrimeSTAR GXL buffer | 10 µl |
10 mM dNTP mix | 4 µl |
10 µM MNV_Frag1.F | 1 µl |
10 µM MNV_Frag1.R | 1 µl |
1 µg/µl pSPORT-T7-MNV1 | 1 µl |
PrimeSTAR GXL DNA polymerase | 1 µl |
Nuclease-free H2O | 32 µl |
Total volume | 50 µl |
Amplification cycles:
- 98°C for 30 s
- 98°C for 10 s, 55°C for 15 s, for 30 cycles
- 68°C for 3 min
- Final extension at 68°C for 10 min.
2.Purify PCR reactions by agarose gel electrophoresis and recover DNA using Zymoclean gel DNA recovery kit following the manufacturer's instructions.
3.Determine DNA concentration of each fragment using NanoDrop 2000 spectrophotometer.
4.Each CPER reaction requires 0.1 picomole of each respective cDNA fragment and the corresponding linker fragment. Use the online calculator (see Internet Resources) to calculate the amount of DNA (ng) given the size (bp) of each fragment that equates to 0.1 picomole. Using this value, calculate the volume of each fragment required for the CPER reaction.
Fragment | Size (bp) |
MNV linker | 1156 |
MNV fragment #1 | 2398 |
MNV fragment #2 | 2697 |
MNV fragment #3 | 2312 |
5.Prepare the CPER reaction by combining 0.1 picomole of each fragment and linker with PrimeSTAR GXL DNA polymerase (Takara Bio) to a total reaction volume of 50 µl.
Reaction setup:
MNV fragment #1 | 1.4 µl |
MNV fragment #2 | 2 µl |
MNV fragment #3 | 1.4 µl |
MNV linker fragment | 1 µl |
5× PrimeSTAR GXL buffer | 10 µl |
10 mM dNTP mix | 4 µl |
PrimeSTAR GXL DNA polymerase | 2 µl |
Nuclease-free H2O | 28.2 µl |
Total | 50 µl |
Cycling conditions:
- 98°C for 30 s
- 98°C for 10 s, 55°C for 30 s, for 12 cycles
- 68°C for 10 min
- Final extension at 68°C for 10 min.
Transfect NIH3T3 cells with MNV CPER reaction and passaging on RAW264.7 cells
Prepare NIH3T3 cells the day before by seeding cells into 6-well plate so that they are ∼70%-80% confluent at the time of transfection. It is important to be gentle with NIH3T3 cells as they are easily dislodged from the flask/plate. It is also possible to use HEK 293T cells instead of NIH3T3.
6.In one 1.5-ml microcentrifuge tube dilute 5 µl Lipofectamine 3000 in 120 μl Opti-MEM, vortex briefly and allow to sit for 5 min.
7.In a second 1.5-ml microcentrifuge tube combine the entire CPER reaction (50 µl DNA) with 70 μl Opti-MEM and 5 µl P3000 reagent provided with the Lipofectamine 3000 to enhance transfection. Mix well.
8.Add all 125 µl Lipofectamine 3000 mixture to the 1.5 ml microcentrifuge tube containing CPER DNA and mix well.
9.Incubate solutions for 15 min at room temperature while preparing cells.
10.To prepare cells, gently remove cell culture medium and replace with 1000 µl serum-free DMEM.
11.Carefully tilt 6-well plate containing NIH3T3 cells and add the transfection mix (from step 8) to the cell culture medium (from step 10). To ensure even distribution of the cDNA, gently rock the plates before placing them at 37°C with 5% CO2.
12.After 3 to 5 hr add 800 µl of DMEM supplemented with 4% FCS.
13.For the recovery of MNV the transfected cell supernatant is collected at 72 hr post-transfection (h.p.t.).
14.The collected supernatant is then clarified from cell debris by centrifugation in 15-ml centrifuge tubes for 5 min at 500 × g , room temperature. 100 μl clarified supernatant is stored at −80°C (for subsequent virus quantitation by plaque assay) while the remaining supernatant (∼1900 μl) is immediately passaged onto a 100-mm culture dish seeded to 80% confluency with RAW264.7 cells.
15.After a 1 hr inoculation of RAW264.7 cells with transfection supernatant, add an additional 10 ml DMEM supplemented with 4% FCS to each dish.
16.Monitor cells daily for cytopathic effect and harvest when CPE is observed (∼3 days).
17.Cell supernatant from the passaged RAW264.7 cell cultures was collected at 3 days post infection.
18.Clarify from cell debris by centrifugation for 5 min at 500 × g , room temperature, and store at −80°C.
Sequence of MNV linker fragment
GACATTGATTATTGACTAGTTATTAATAGTAATCAATTACGGGGTCATTAGTTCATAGCCCATATATGGAGTTCCGCGTTACATAACTTACGGTAAATGGCCCGCCTGGCTGACCGCCCAACGACCCCCGCCCATTGACGTCAATAATGACGTATGTTCCCATAGTAACGCCAATAGGGACTTTCCATTGACGTCAATGGGTGGAGTATTTACGGTAAACTGCCCACTTGGCAGTACATCAAGTGTATCATATGCCAAGTCCGCCCCCTATTGACGTCAATGACGGTAAATGGCCCGCCTGGCATTATGCCCAGTACATGACCTTACGGGACTTTCCTACTTGGCAGTACATCTACGTATTAGTCATCGCTATTACCATGGTGATGCGGTTTTGGCAGTACACCAATGGGCGTGGATAGCGGTTTGACTCACGGGGATTTCCAAGTCTCCACCCCATTGACGTCAATGGGAGTTTGTTTTGGCACCAAAATCAACGGGACTTTCCAAAATGTCGTAACAACTGCGATCGCCCGCCCCGTTGACGCAAATGGGCGGTAGGCGTGTACGGTGGGAGGTCTATATAAGCAGAGCTCGTTTAGTGAACCGGTGAAATGAGGATGGCAACGCCATCTTCGAAACCTTTTGTGAAAATGATTTCTGCTTACTGCTTTCTTTTCTTTGTGGTAGTTAGATGCATTTTAAAAAAAAAAAAAAAAAAAAAAAAAAAAAAGGGTCGGCATGGCATCTCCACCTCCTCGCGGTCCGACCTGGGCATCCGAAGGAGGACGTCGTCCACTCGGATGGCTAAGGGAGAGCCACTTTTCTCTCGATTCTCTATCGGAATCTAGGGAGCTCGGATCCAGACATGATAAGATACATTGATGAGTTTGGACAAACCACAACTAGAATGCAGTGAAAAAAATGCTTTATTTGTGAAATTTGTGATGCTATTGCTTTATTTGTAACCATTATAAGCTGCAATAAACAAGTTAACAACAACAATTGCTCGAGGGGGGGCCCGGTACCTTGAAGCTGTCCCTGATGGTCGTCATCTACCTGCCTGGACAGCATGGCCTGCAACGCGGGCATCCCGATGCCGCCGGAAGCGAGAAGAATCATAATGGGGAAGGCCATCCAGCCTCGCGTCGGCGCTTAA
Basic Protocol 5: EXPRESSION OF NOROVIRUS NS1-2 IN INSECT CELL SUSPENSION CULTURES USING A RECOMBINANT BACULOVIRUS
The expression of mammalian viral proteins in Escherichia coli is commonly undertaken. However, many viral proteins are difficult to express in E. coli and the issues of solubility and lack of post-translational modification remain. Recombinant baculovirus infection in insect cells is an effective, robust, and widely used alternative. This eukaryotic expression system has been used for norovirus virus-like particle production and is available in a range of commercial options, including the bacmid system and homologous recombination systems for recombinant virus construction. The benefits of expressing proteins through baculovirus includes the availability of the host eukaryote post-translational modification systems, such as phosphorylation, and the ability to transduce mammalian cells at high efficiency in the absence of viral replication. The baculovirus expression vector system (BEVS) is therefore a relevant system to express the potentially phosphorylated HuNV NS1-2 protein for recombinant protein production in insect cells, mammalian cells and to generate isotopically labelled proteins for NMR analysis. Herein, we describe the expression of the NS1-2 protein in insect cell suspension cultures in serum-free medium. Using this system, we and others have also expressed NS5 (VPg), NS6 (Pro), NS7 (Pol), NS6/7 (ProPol) and VP1 from GI, GII and GV noroviruses. The generation of the recombinant virus can be performed using any commercially available baculovirus system, for this protocol the FlashBac Ultra system from Oxford Expression Technologies (Oxford, UK) was utilized.
NOTE : The NS1-2 coding sequence used in this protocol for recombinant protein production and purification represents amino acids 3-249 of the NS1-2 protein and is therefore devoid of the C-terminal transmembrane region. The construct includes an N-terminal histidine and/or STREP-II tag for protein purification and with or without an NT* fusion protein (Kronqvist et al., 2017) containing an enterokinase cleavage site for removal. Typically, we express and purify 4-6 mg of NS1-2 per 50 ml of cell culture.
Additional Materials (also see Basic Protocol 1)
-
Trichoplusia ni (T. ni) insect cells (Expression Systems, cat. no. 94-002F)
-
ESF921 insect cell medium (Expression Systems, cat. no. 96-001-01)
-
0.1% trypan blue in PBS, pH 6.2 (see recipe)
-
Titred NS1-2 expressing recombinant baculovirus (Irons et al., 2018)
-
250-ml Schott bottle
-
Innova 42R incubator shaker, 1.9 cm orbit (New Brunswick Scientific)
-
Hemocytometer
-
50-ml polypropylene tube
1.Seed T. ni cells into 50-ml ESF921 medium at a final concentration of 8 × 105 cells/ml in a 250-ml Schott bottle.
2.Incubate at 27°C overnight with orbital shaking at 125 rpm.
3.Check the cell count and viability of the cells by mixing 10 µl cell suspension with 10 µl 0.1% trypan blue and loading onto a hemocytometer.
4.Infect the 50 ml cell suspension culture by pipetting the recombinant baculovirus containing the norovirus NS1-2 gene directly into the cell suspension at a multiplicity of infection (MOI) of 1.0.
5.Incubate the infected culture at 27°C for 2-4 days.
6.Collect the cells from the infected culture by centrifugation for 5 min at 500 × g , room temperature.
Support Protocol 2: ISOTOPE LABELLING OF NOROVIRUS NS1-2 IN INSECT CELLS
This protocol is to facilitate isotopic labelling of recombinant NS1-2 protein. The analysis of proteins by NMR or metabolomic mass spectrometry is benefited from the use of isotopic labels for the protein of interest. Isotopic labelling is well-established for E. coli but less so for the BEVS. This protocol is based upon the method of Sitarska et al. (Sitarska et al., 2015) for Spodoptera frugiperda cells and has been adapted to T. ni cells for labelling with either 15N or 15N/13C. On average, 73% of 14N is replaced with 15N for the NS1-2 protein of human GII.4 norovirus after isotope labelling.
Additional Materials (also see Basic Protocol 5)
- Basal ΔESF921 (all amino acid deficient modified medium, Expression Systems, cat. no. 96-276-01)
- 15N-ISOGRO medium (see recipe)
1.Make 15N-ISOGRO medium (see Reagents and Solutions) and filter into a 250-ml Schott bottle and store at 4°C.
2.Seed a 50 ml T. ni culture as in Basic Protocol 5 in ESF921 medium.
3.Incubate at 27°C for 24 hr shaking at 125 rpm.
4.Infect the 50 ml culture with the recombinant baculovirus at an MOI of 2.0 and incubate at 27°C for 16 hr.
5.Check the cell count and viability by hemocytometer as described in Basic Protocol 5, step 3.
6.Collect the infected cells by centrifugation for 5 min at 500 × g , room temperature.
7.Remove the supernatant and wash the cells by gently resuspending the cell pellet in 10 ml of basal ΔESF921 medium and recollecting the cells by centrifugation for 5 min 500 × g , room temperature.
8.Remove the basal ΔESF921 medium and gently resuspend the cell pellet in 10 ml 15N-ISOGRO medium.
9.Add the resuspended cells to 40 ml 15N-ISOGRO medium in a 250-ml Schott bottle.
10.Incubate the infected culture in 15N-ISOGRO medium at 27°C at 125 rpm and monitor daily until the cell viability drops below 80%, as described in Basic Protocol 5.
11.Harvest the infected cell pellet by centrifugation as described in Basic Protocol 5 and store at −80°C.
Support Protocol 3: PURIFICATION OF THE NOROVIRUS NS1-2 PROTEIN
This protocol describes a small-scale batch purification of the norovirus NS1-2 protein from a 50 ml T. ni suspension culture as described in Basic Protocol 4.While many laboratories will have chromatographic purification systems and these are often the methods of choice, the use of small-scale batch purifications using epitope affinity tags can be used effectively for the extraction of the NS1-2 protein from insect cells.
Additional Materials (also see Basic Protocols above)
-
NS1-2 purification buffers (see recipe)
-
cOmplete, EDTA-free protease inhibitor cocktail (Merck, cat. no. 11873580001)
-
RNAse (see recipe)
-
DNAse I
-
20-G needle and 5-ml disposable syringe
-
0.8-micron filter
-
Strep-Tactin XT 4Flow high-capacity resin (IBA Lifesciences, cat.no. 2-5030-002)
-
Gravity flow purification column (Pierce)
-
Additional reagents and equipment for SDS-PAGE and western blot analysis (Ni et al., 2006)
1.Thaw and resuspend a 50 ml T. ni cell pellet from Basic Protocol 5 in lysis buffer containing 1× protease inhibitor at ∼5 × 106–1 × 107 cells/ml.
2.Add RNase and DNase I at a final concentration of 10 µg/ml each and incubate on ice for 10 min.
3.Shear the sample by passing it through a 20-G needle five times.
4.Centrifuge the lysate 20 min at 14,000 × g , 4°C.
5.Collect the supernatant and filter through a 0.8-micron filter.
6.Add Strep-Tactin resin (0.5 ml per 20 ml) to the clarified supernatant and incubate for 1 hr at 4°C, ensuring the resin remains in suspension.
7.Load the lysate plus Strep-Tactin onto a gravity flow purification column at room temperature to collect the resin.
8.Wash the resin with 20 ml wash buffer I, followed by 20 ml wash buffer II.
9.Add 0.5 ml of elution buffer and collect the eluant.
10.Repeat the elution seven times to collect eight 0.5 ml elution fractions.
11.Analyze the fractions by SDS-PAGE and western blot analysis to confirm expression and purification.
Support Protocol 4: EXPRESSION OF NOROVIRUS NS1-2 IN MAMMALIAN CELLS BY TRANSDUCTION WITH A RECOMBINANT BACULOVIRUS
The baculovirus, Autographa californica nucleopolyhedrovirus, is unable to replicate in mammalian cells but can transduce a wide variety of cell types. Commercial vectors for the use of baculovirus in mammalian cell transduction are available. This protocol is to use a recombinant baculovirus expressing the GII.4 NS1-2 protein under the control of a constitutive CMV mammalian cell promoter to express the NS1-2 protein in HEK293T cells, thereby facilitating the analysis of this protein in a mammalian cell.
Additional Materials (also see Basic Protocols 1 and 5)
-
HEK293T mammalian cells (ATCC, cat. no. CRL-3216)
-
Dulbecco's A (Ca-free) PBS, pH 7.4 (see recipe)
-
Recombinant baculovirus stock
-
Sodium butyrate (see recipe)
-
25-cm2 tissue culture flask
1.Seed a 25-cm2 flask with 3 × 106 HEK293T cells in 5 ml DMEM-GlutaMAX-10 and incubate overnight at 37°C, 5% CO2.
2.Remove medium and wash the cell monolayer with 2 ml of Dulbecco's A (Ca-free) PBS, pH 7.4.
3.Dilute recombinant baculovirus inoculum by adding 600 µl virus to 2.4 ml of Dulbecco's A PBS pH 7.4 (1:4 ratio) to dilute the insect cell medium.
4.Add 3 ml of the diluted inoculum to the HEK293T cells.
5.Incubate at room temperature for 8 hr to allow the baculovirus to transduce the cells.
6.Remove the inoculum and wash the cells once with 2 ml Dulbecco's A PBS pH 7.4.
7.Add fresh DMEM-GlutaMAX-10 containing 5 mM sodium butyrate to the cells and incubate at 37°C for 48 hr.
8.Wash the cells with 5 ml of Dulbecco's A PBS then detach into 5 ml of fresh Dulbecco's A PBS using a cell scraper.
9.Collect the cells by centrifugation for 5 min at 300 × g , room temperature, remove the Dulbecco's A PBS and use immediately or store the pellet at −80°C.
Basic Protocol 6: INFECTION OF ENTEROIDS IN TRANSWELL INSERTS WITH MURINE NOROVIRUS
This procedure describes the process of infecting mouse small intestinal enteroids with MNV. This protocol offers an ex vivo infection system to interrogate MNV infection in primary epithelial cells, without any immune, stromal, or mesenchymal cellular contributors. Intestinal tuft cells are the exclusive epithelial cell type which expresses the MNV receptor CD300lf. However, CD300lf is expressed apically on tuft cells, while enteroids grown in 3D culture grow with their apical surface facing inward. When enteroids are polarized on a transwell filter, their apical surface becomes exposed to enable viral entry (Strine et al., 2022). Tuft cells can be robustly infected by both MNVCW3 and MNVCR6 in enteroids in vitro (Strine et al., 2022).
Additional Materials (also see Basic Protocols 1 and 4)
-
Matrigel (Corning basement membrane matrix, cat. no. 354234 or 356231), thawed on ice and aliquoted in small volumes and stored at −20°C
-
1× PBS, pH 7.2 (Gibco, cat. no. 20012027)
-
DMEM-10 (see recipe)
-
50% L-WRN conditioned medium (see Support Protocol 5, step 9 or 10)
-
Y27632 dihydrochloride (ROCK inhibitor) (Tocris, cat. no. 1254)
-
IntestiCult organoid growth medium (Mouse, STEMCELL Technologies, cat. no. 06005)
-
Recombinant murine IL-4 (Peprotech, cat. no. 214-14)
-
Transwell inserts (Costar, 6.5 mm insert, 0.4 μm pore polyester membrane, cat. no. 3470)
-
24-well clear TC-treated plates (Corning, cat. no. 3524)
-
37°C water bath
Pre-coat the Transwell inserts
1.Place one Transwell insert per one well of a 24-well plate.
2.Add 100 μl of 10% Matrigel solution in sterile 1× PBS to each Transwell insert.
3.Incubate at 37°C for at least 20 min.
Dissociate the 3D enteroid cultures
4.Dissociate 3D enteroids gently in Matrigel bubbles (see Support Protocol 7, steps 1-6).
5.Manually disrupt the enteroids by pipetting up and down to produce a single-cell suspension.
6.Neutralize the dissociation reaction with ∼10 ml DMEM-10.
7.Pellet the cells by centrifuging 5 min at 500 × g , 4°C, and aspirate the supernatant without disturbing the pellet.
8.Resuspend the cells in 50% L-WRN conditioned medium supplemented with 10 mM Y27632 (see Support Protocol 5, step 9 or 10).
Plate the single-cell suspension on the Transwell inserts
9.Aspirate Matrigel/PBS solution from the pre-incubated Transwell inserts.
10.Add 100 μl resuspended cells into the upper (top) Transwell chamber and add 600 μl 50% L-WRN conditioned medium with 10 mM Y27362 to the lower compartment beneath the insert.
11.The next day, replace the medium in the upper and lower compartments with fresh mouse IntestiCult organoid growth medium supplemented with 50 ng/ml rIL-4.
Differentiating the enteroids on the Transwell inserts
12.Replace the medium every 1-2 days.
13.Maintain the culture for 14 days before infection.
Infect the enteroids with MNV
14.On day 14, infect the enteroids apically (i.e., adding virus to the upper chamber) by adding 100 μl L-WRN conditioned medium containing MNVCR6 or MNVCW3 at the desired multiplicity of infection (MOI).
15.Incubate the virus on the enteroids for 1 hr at 37°C to facilitate viral binding.
16.After 1 hr, wash off any unbound virus using 100 μl pre-warmed 1× PBS in the apical chamber.
17.After three washes, add fresh L-WRN conditioned medium to the apical chamber.
18.Harvest the virus by collecting supernatant from the upper chamber of the Transwell insert at desired timepoints.
Support Protocol 5: PREPARATION OF CONDITIONED MEDIUM FOR ENTEROID CULTURE
Additional Materials (also see Basic Protocols above)
-
L-WRN cells (available from ATCC, CRL-3276)
-
L-cell medium (see recipe)
-
0.05% Trypsin-EDTA (Gibco, cat. no. 25300054)
-
G418 (Gibco, cat. no. 10131035)
-
Hygromycin (InvivoGen, cat. no. ant-hg-5)
-
Primary culture medium (see recipe)
-
150-cm2 tissue culture treated vented flasks (e.g., Corning)
-
0.22-micron filter system (Millipore Stericup)
-
50-ml conical centrifuge tube (e.g., Falcon)
NOTE : This protocol is for small scale preparation and has been adapted from (Miyoshi & Stappenbeck, 2013).
1.Grow L-WRN cells in L-cell medium (see recipe) at 37°C in a 150-cm2 flask.
2.When confluent, trypsinize the cells using 0.05% Trypsin-EDTA and incubate at 37°C until they detach (∼1-2 min).
3.Once detached, neutralize with L-cell medium and re-plate them at ∼1–2 × 106/flask in 150-cm2 flasks containing 500 μg/ml G418 and 2 mM hygromycin in L-cell medium. Incubate at 37°C.
4.When cells are overly confluent (∼2-3 days), wash the flasks twice with 20 ml 1× PBS and once with 10 ml primary culture medium (see recipe).
5.Add 25 ml fresh primary culture medium to each flask and incubate at 37°C.
6.Every 24 hr, collect the culture medium (“L-WRN conditioned medium”). Store these fractions at 4°C.
7.Pool all the L-WRN conditioned medium fractions and clarify them by centrifugation for 5 min at 500 × g , 4°C. Collect the medium, leaving the pelleted cellular debris behind.
8.Filter the L-WRN conditioned medium through a 0.22-micron filter.
9.Dilute the L-WRN conditioned medium with an equal volume of fresh primary culture medium, generating 50% L-WRN conditioned medium.
10.Aliquot the 50% L-WRN conditioned medium stocks in 50-ml falcon tubes and store at −80°C until further use.
Support Protocol 6: ISOLATION OF CRYPTS FOR ENTEROID GENERATION
Additional Materials (also see Basic Protocols above and Support Protocol 5)
-
Mouse of desired genotype
-
Crypt culture medium (see recipe)
-
Collagenase type I (Invitrogen, cat. no. 17100-017)
-
Gentamicin (Sigma, cat. no. G1397)
-
Surgical scissors
-
Forceps
-
Wide bore syringe
-
40-micron cell strainer (e.g., Fisher)
1.Sacrifice the mouse according to the Institutional Animal Care and Use Committee protocol. Using surgical scissors and forceps, cut open the mouse abdominal cavity and harvest the distal small intestine (∼10 cm), gently clearing it of fat and connective tissue (Fig. 6).
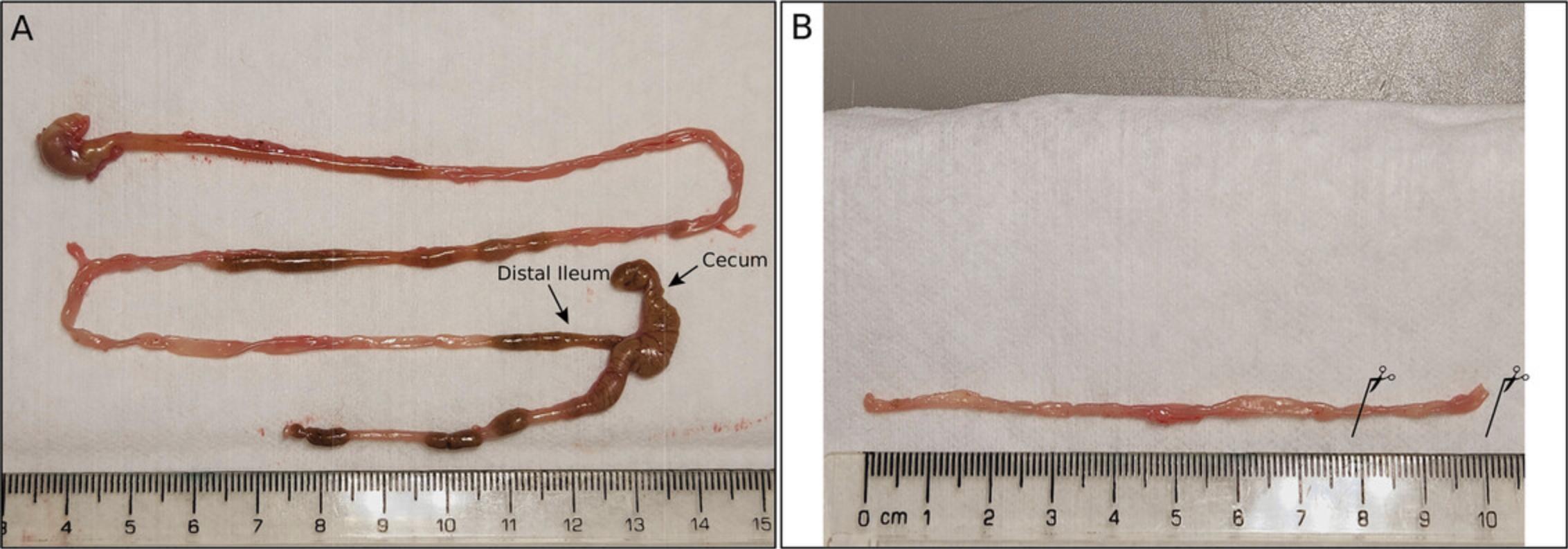
2.Using a wide bore blunt syringe or P1000 pipet tip, flush the tissue with cold 1× PBS to remove any contents.
3.Cut the tissue longitudinally and vortex 15 s in cold 1× PBS.
4.Mince the tissue and transfer it in a 15-ml conical tube in 1-2 ml crypt culture medium (see recipe) supplemented with 2 mg/ml collagenase type I and 50 μM gentamicin. Incubate at 37°C for 30 min.
5.After 30 min, filter the isolated crypts through a 40-micron cell strainer into a 50-ml conical tube. Add 10 ml crypt culture medium and centrifuge 5 min at 500 × g , 4°C.
6.Aspirate the supernatant without disturbing the pellet and add another 10 ml crypt culture medium. Gently resuspend the pellet, transfer to a 15-ml conical tube, and centrifuge 5 min at 500 × g , 4°C.
7.Aspirate the supernatant and resuspend the purified crypts in one drop of 30 μl of Matrigel per well of a 24-well tissue plate.
8.Invert the plate and incubate it ∼10 min at 37°C to polymerize the Matrigel.
9.Once the Matrigel is solidified, return the plate to its correct orientation, and add 600 μl 50% L-WRN conditioned medium with 10 mM Y27632 per well of a 24-well plate.
Support Protocol 7: ENTEROID CULTURE PASSAGING AND MAINTENANCE
Additional Materials (also see Basic Protocols above)
- PBS-EDTA (see recipe)
- 0.05% trypsin-EDTA (Gibco, cat. no. 25300054)
1.Aspirate the culture medium from each well.
2.Dissociate 3D enteroids gently in Matrigel bubbles by adding 500 μl PBS-EDTA to each well of the 24-well plate containing Matrigel enteroid bubbles.
3.Manually scratch and triturate the enteroid bubbles gently by pipetting up and down ∼5 times.
4.Pool the enteroids and transfer them to a single 15-ml conical tube and centrifuge for 5 min at 500 × g , 4°C.
5.Aspirate the PBS-EDTA without disturbing the pellet and add 200 μl of pre-warmed (37°C) 0.05% Trypsin-EDTA per 8 dissociated bubbles (e.g., from 8 wells with 30 μl enteroids in one Matrigel bubble in each one).
6.Incubate the cells in a 37°C water bath for 90 s.
7.Manually dissociate the enteroids by gently pipetting up and down.
8.Neutralize the dissociation reaction with 10 ml DMEM-10.
9.Pellet the cells by centrifuging 5 min at 500 × g , 4°C, and aspirate the supernatant without disturbing the pellet.
10.Resuspend the cells in one drop of 30 μl Matrigel per well of a 24-well plate and grown in 50% L-WRN conditioned medium.
Basic Protocol 7: QUANTIFICATION OF MURINE NOROVIRUS-INDUCED DIARRHEA USING NEONATAL MOUSE INFECTIONS
This procedure outlines the quantification of MNV-induced diarrhea using the neonatal mouse model. We describe the inoculation methodology and disease scoring system. This protocol can be used with neonatal mice 3-6 days post birth (P2-P5) with no significant difference in intestinal disease outcomes, but diarrhea severity is reduced in mice infected at P7 or later (Peiper et al., 2023). The oral gavage method has been validated using BALB/c mice (Roth et al., 2020) while the intragastric inoculation method has been validated in both BALB/c (Roth et al., 2020) and C57BL/6J neonatal mice (Peiper et al., 2023). Both inoculation methods can deliver up to 40 µl of volume to the pups. Disease outcomes can be observed 24-72 hr post-infection (hpi). Depending on the application, infection doses of 107 to 108 are useful. Fecal scoring can be performed at multiple time points using the same neonatal mouse, but the mice do not defecate at every timepoint, representing a limitation of this readout. Colon content scores can be used to overcome this limitation, although the mouse must be euthanized to acquire a colon content score (Helm et al., 2022). This model is useful for a wide range of applications including disease kinetics of MNV strains (Peiper et al., 2023; Roth et al., 2020), identification of virulence determinants and host determinants of protection (Helm et al., 2022), and efficacy of small molecules and therapeutics.
Additional Materials (also see Basic Protocol 1 and 6)
-
P0-P5 neonatal mice (BALB/c or C57BL/6J)
-
1.5-2 ml Eppendorf tubes
-
22-G × 25-mm plastic feeding tubes (Instech, cat. no. FTP-22-25-50)
-
1-ml insulin syringes (Fisher Scientific, cat. no. 14955462)
Oral gavage inoculation of neonatal mice
It is recommended that only BALB/c pups are used with this method since the relatively small size of C57BL/6J mice make them vulnerable to gavage-induced trauma. In our experience, ∼20% of C57BL/6J pups inoculated at P3-P5 succumb to oral gavage independent of infection, a confounding variable not observed in BALB/c pups.
1.Make the desired virus inoculum in Eppendorf tubes in a volume of 10-40 µl per mouse. Additional volume should be made for loss of inoculum in the tip of the syringe.
2.Attach 22-G plastic feeding tube to the end of syringe and remove any air bubbles. The same tube can be used between each mouse as long as the tube filter remains clear.
3.Insert feeding tube into the mouth of each pup and thread down to the esophagus. Make sure to insert slowly to avoid punctures in the esophagus.
4.Deliver up to 40 µl to each mouse.
5.Carefully withdraw the tube.
6.Observe the mouse for 5 min to monitor for any signs of distress before returning it to the cage. If signs of distress such as difficulty breathing or lethargy are observed, euthanize the mouse per IACUC protocol.
Scoring intestinal disease in fecal samples
7.At desired time points, palpate the abdomen of each mouse to induce defecation.
8.Assess feces based on color and consistency according to a 6-point scale (Fig. 7): 0, no defecation; 1, firm, orange, does not smear; 2, pasty, orange, or mixed color, does not smear; 3, orange or yellow, semi-liquid and smears; 4, yellow, liquid, and smears; 5, clear, liquid, and smears.
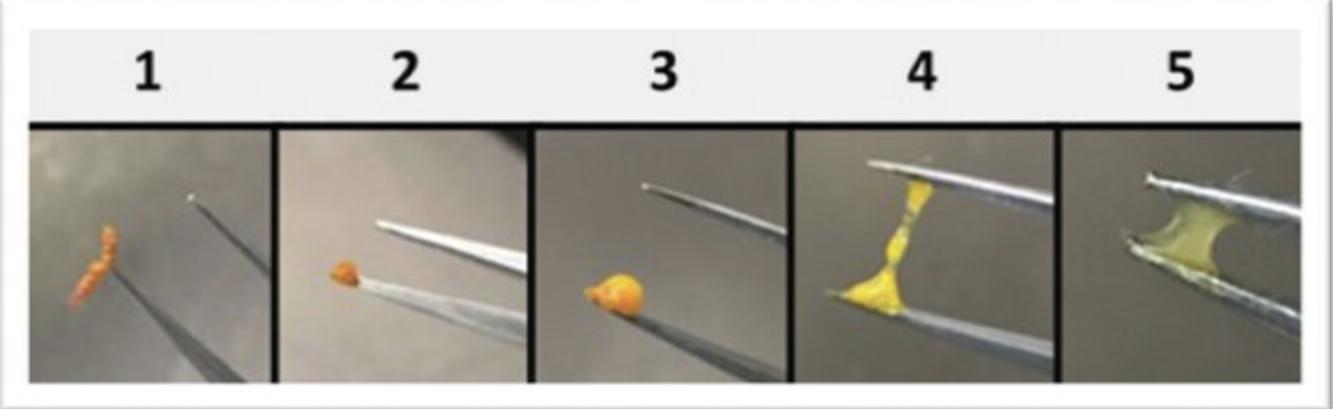
9.Disease severity is reported as the average fecal score per condition, excluding any scores of 0. Disease incidence is reported at the percentage of mice per condition with a fecal score of 3 or higher. For statistical purposes and to control for inter-litter heterogeneity, at least 10 neonates from 3 separate litters should be used per condition tested.
Alternate Protocol 1: INTRAGASTRIC INOCULATION OF NEONATAL MICE
Additional Materials (also see Basic Protocol 7)
- 30-G (0.3-mm × 25-mm) needles (BD, cat. no. 305128)
NOTE : This method can be used in BALB/c and C57BL/6J mice up to P7.After P7, the milk spot disappears and intragastric delivery is no longer possible.
1.Make desired inoculum in Eppendorf tubes in a volume of 10-40 µl per mouse. Additional volume should be made for loss of inoculum in the tip of the syringe.
2.Attach 30-G needle to the end of syringe and remove any air bubbles. The same needle can be used between each mouse if no blood is present on the tip of the needle.
3.Restrain the neonatal mouse so the milk spot becomes clear and close to the surface. The milk spot in the stomach is visible through the skin on the abdominal wall. It should appear in the upper right quadrant of the abdomen. It is recommended to do inoculations when the milk spot is clearly visible to ensure accurate injections (Fig. 8).
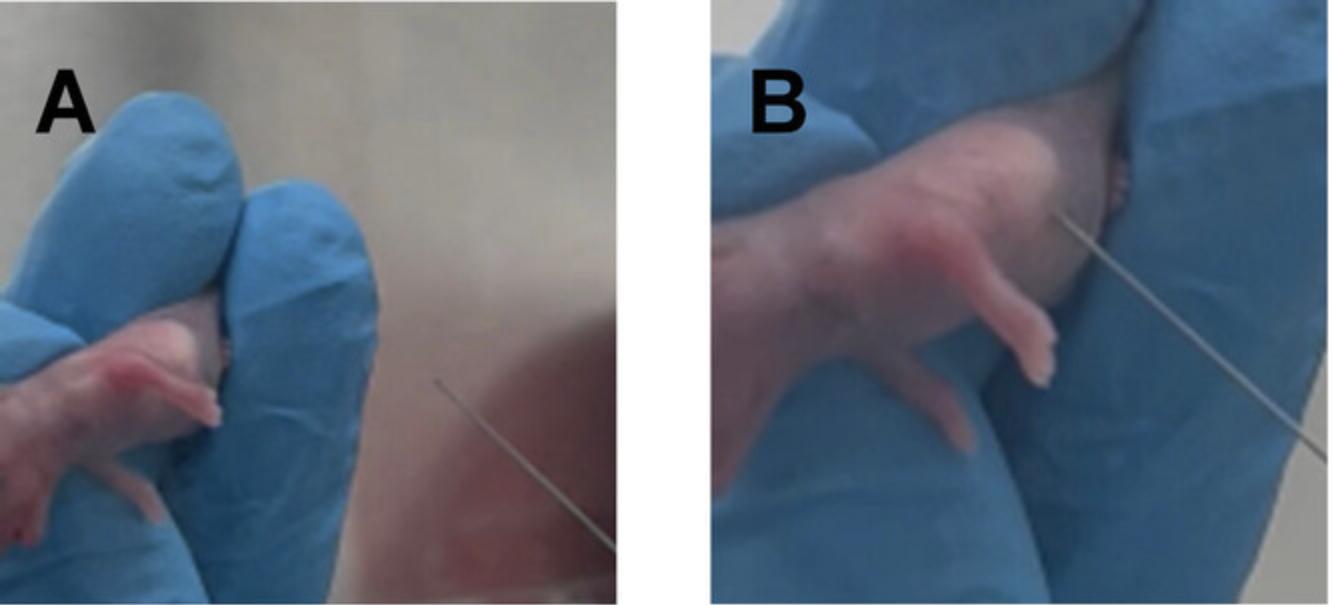
4.Insert the needle parallel to the stomach so that the needle pierces the stomach lengthwise. If the needle is inserted properly, the stomach wall will indent and then bounce back when the stomach is pierced (Fig. 8).
5.Deliver up to 40 µl to each mouse.
6.Carefully withdraw the needle. Minimal flowback is expected.
7.Observe the neonate for 5 min to monitor for any signs of distress before returning it to the cage. If signs of distress are observed, euthanize the mouse per IACUC protocol.
Alternate Protocol 2: SCORING COLON CONTENTS
1.At the desired timepoint, euthanize the mouse according to the guidelines of the home institution and IACUC protocol.
2.Briefly dip the mouse carcass into 70% ethanol. Using forceps and scissors, cut both the skin and peritoneum longitudinally along the midline of the abdomen being careful not to cut the intestines.
3.Locate the junction of the anus and colon and cut the colon as close to the anus as possible.
4.Carefully remove the colon without tearing it until you locate the cecum and then cut at the cecum.
5.Using the scissors in a closed position, squeeze out the contents of the colon by running the closed scissors across the colon.
6.Score the contents according to the scale in Figure 7.
REAGENTS AND SOLUTIONS
15N-ISOGRO medium
- 200 ml basal ΔESF921 (all amino acid deficient modified medium, Expression Systems, cat. no. 96-276-01)
- 2 g 15N-ISOGRO powder (Merck, cat. no. 606871)
- 2 g D-glucose (56 mM final)
- Stir 15 min at room temperature
- Add:
- 400 µl 10 mg/ml L-tryptophan-15N (100 µM final; see recipe)
- 167 µl 300 mg/ml 15N-NH4Cl (5 mM final; see recipe)
- 200 µl 5 mg/ml L-cycloserine (50 µM final; see recipe)
- Stir 1 min at room temperature
- Sterilize using a 0.22-micron filter (Thermo Fisher Scientific, cat. no. 596-4520)
- Store up to 1 month at 4°C
15N-NH4Cl, 300 mg/ml
- 250 mg 15N-NH4Cl
- 833 µl in milliQ H2O
- Sterilize using a 0.22-micron filter
- Store at −20°C
Cell culture medium
- 500 ml DMEM, low glucose (Gibco, cat. no. 41965062)
- Add:
- 10% (v/v) FBS (Bovogen, cat. no. SFBS)
- 1% (v/v) penicillin/streptomycin solution (Sigma-Aldrich, cat. no. P0781)
- 1% (v/v) L-glutamine (C C Pro, cat. no. Z-10-M)
- 1% (v/v) non-essential amino acids (Capricorn Scientific, cat. no. NEAA-B)
- Store up to 6 months at 4°C
Crypt culture medium
- DMEM/F12 (Gibco, cat. no. 11320033)
- 15 mM HEPES (Gibco, cat. no. 15630080)
- 200 mM L-glutamine (Gibco, 25030081)
- 10% (v/v) FBS, heat inactivated (Corning, cat. no. 35-010-CV)
- 1% (v/v) penicillin/streptomycin solution (Gibco, cat. no. 15070063)
- Store up to 1 year at 4°C
L-Cycloserine, 5 mg/ml
- 10 mg L-cycloserine (Merck, cat.no. C1159)
- 2 ml in milliQ H2O
- Filter sterilize using a 0.22-micron filter
- Store at −20°C
DMEM-10
- DMEM (Gibco, cat. no. 11965092)
- 10% FBS heat inactivated (Corning, cat. no. 35-010-CV)
- Store up to 1 year at 4°C
DMEM-GlutaMAX-10
- DMEM, high glucose, GlutaMAX (Thermo Fisher Scientific, cat. no. 10566-016)
- 10% (v/v) FBS (ThermoFisher Scientific, cat. no. 10091-148)
- Store at 4°C
Dulbecco's A (Ca-free) PBS, pH 7.4
- PBS tablet (Oxoid, cat. no. BR0014G)
- 100 ml milliQ H2O
- Sterilize using a 0.22-micron filter
- Store aliquots at −20°C
L-cell medium
- High glucose DMEM (Gibco, cat. no. 11965092)
- 1% (v/v) penicillin/streptomycin solution (Gibco, cat. no. 15070063)
- 10% (v/v) FBS, heat inactivated (Corning, cat. no. 35-010-CV)
- Store up to 1 year at 4°C
NS1-2 purification buffers
Sterilize all buffers using a 0.22-micron filter and store at 4°C before use. The Elution Buffer can be aliquoted and stored at −20°C.
- Base Buffer :
- 50 mM NaH2PO4.2H2O pH8
- 300 mM NaCl
- Make up to 1 L and autoclave
- Lysis Buffer :
- Base Buffer
- 10% glycerol
- 1% Triton X-100.
- Wash Buffer I :
- Base Buffer
- 10% glycerol
- 0.05% Triton X-100
- Wash Buffer II :
- Base Buffer
- 10% glycerol
- Elution Buffer :
- Base Buffer
- 50 mM biotin
- Adjust pH to 8.0 with 10 M NaOH
PBS, pH 6.2
- 1 mM Na2HPO4
- 10.5 mM KH2PO4
- 140 mM NaCl
- 40 mM KCl
- Adjust pH to 6.2 using HCl
- Store at room temperature
PBS-EDTA
- 1× PBS, pH 7.2 (Gibco, cat. no. 20012027)
- 5 mM EDTA (AmericanBio, cat. no. AB00502)
- Store up to 1 year at 4°C
Primary culture medium
- Advanced DMEM/F-12 (Gibco, cat. no. 12634010)
- 200 mM L-glutamine (Gibco, cat. no. 25030081)
- 1% (v/v) penicillin/streptomycin solution (Gibco, cat. no. 15070063)
- 4% FBS, heat inactivated (Corning, cat. no. 35-010-CV)
- Store up to 1 year at 4°C
RNase
- RNase A (10 mg/ml final) (Sigma, cat. no. R4875)
- 20 mM Tris pH 7.5 (Current Protocols, 2006)
- 1 mM MgCl2 (Current Protocols, 2006)
- 50% (v/v) glycerol
- Store at −20°C
Sodium butyrate
- 5 mM sodium butyrate
- DMEM GlutaMAX medium (Thermofisher Scientific, cat. no. 10566-024)
- 10% FBS
- Sterilize using a 0.22-micron filter and use immediately
L-Tryptophan-15N, 10 mg/ml
- 20 mg L-Tryptophan-15N (Merck, cat. no. 609064)
- 2 ml in milliQ H2O
- Sterilize using a 0.22-micron filter
- Store at −80°C
COMMENTARY
MNV antiviral efficacy determination
Large scale screening of putative antivirals against human norovirus remains challenging. Herein, we use MNV as a model to illustrate a laboratory scale system utilizing the measurement of ATP to determine cell health, and the utility of indirect measurements of virus replication to observe unexpected effects that compound carriers such as DMSO can have on norovirus infection. Specifically, we present a method for indirectly assessing cell viability in the presence of MNV and antiviral compounds using CellTiter-Glo (Promega, WI, USA). The regent lyses cells to release ATP which is then converted to a stable luminescent signal proportional to the health of the cells at the time of harvest. The sensitivity and linear range of CellTiter-Glo allow for the detection of subtle changes in the cells or virus in response to an antiviral, that may go undetected with other reagents. The reagent does not require fixing or washing of cells, thereby significantly limiting loss of cells that lift off the plate during infection. This protocol generates both CC50 and EC50 values in technical triplicate on one 96-well plate. This is achieved by seeding plates with an MNV permissible cell line (Haga et al., 2016; Lingemann, 2020; Orchard et al., 2016; Wobus et al., 2004), treating with a dilution series of the antiviral, and infecting with MNV. The plate is incubated for 24 hr, until 90% of virally infected control cells are dead, following this CellTiter-Glo reagent is added. Limitations of using CellTiter-Glo include the potential cost compared to other indirect cell viability reagents. This limitation can be mitigated as the “one-solution” method is simple and quick, includes non-adherent cells, and due to the reagent's sensitivity could be adapted to a 1536 well plate assay. Another potential limitation of CellTiter-Glo, is that the reagent measures ATP as an indicator of cell metabolism and therefore health. Activation of immune cells and potentially viral infection also have the ability to increase ATP levels within cells. Therefore, before antiviral screening, a viral infection comparing the TCID50 generated using CellTitre-Glo to the titer calculated by plaque assay would need to be performed to confirm there is no to minimal effect between assays. Finally, because the values generated using this method are an indirect measure of viral replication, and potentially some antivirals tested may interact with the reagent itself, positive results should be confirmed using a direct measure of replication.
Baculovirus expression of NS1-2
Despite progress in the development of cell culture systems for human norovirus (Ettayebi et al., 2016; Ettayebi et al., 2021; Jones et al., 2015; Van Dycke et al., 2019), much is yet to be discovered regarding the function of individual proteins during viral replication. Some norovirus proteins remain challenging to express and analyze, therefore a potent eukaryotic system is needed to further our understanding of virus-host interactions and to advance antiviral drug discovery. Norovirus NS1-2, the N-terminal non-structural protein present in ORF1, contains both an inherently disordered region (IDR) and a transmembrane domain. Much is still to be determined regarding the function of this protein, posttranslational modifications, and its potential cleavage products. Recombinant baculovirus infection in insect cells is an effective, robust, and widely used eukaryotic expression system that has been used for norovirus virus-like particle production and is available in a range of commercial options. The benefits of expressing proteins through baculovirus includes the availability of the host eukaryote post-translational modification systems, such as phosphorylation, and the ability to transduce mammalian cells at high efficiency in the absence of viral replication.
MNV infection of intestinal enteroids
MNV was initially shown to infect immune cells in cell culture (Wobus et al., 2004). While the acute MNV-1 strain (and MNV-1 clone MNV.CW3) also infects immune cells in vivo (Grau et al., 2017; Van Winkle et al., 2018), persistent MNV strains, including MNV.CR6, infect intestinal tuft cells, a feature critical for virus persistence (Strine et al., 2022; Wilen et al., 2018). Tuft cells are rare epithelial cells located in the gut and lung that exhibit functions akin to those of immune cells. They mediate important interkingdom interactions between the host and helminths, protists, viruses, and bacteria and have diverse and critical functions in immunity, inflammation, and tumorigenesis (Strine & Wilen, 2022). To study the interaction of MNV with tuft cells, we have turned to intestinal enteroids, non-transformed biopsy-derived small intestinal organoids. Murine enteroids recapitulate the mouse intestinal epithelium but lack hematopoietic and stromal compartments. To generate an in vitro enteroid culture system that supports tuft cell development, intestinal stem cells are isolated from the small intestinal epithelium of adult mice, propagated, and differentiated in the presence of recombinant interleukin-4 to stimulate tuft cell expansion. Since the viral receptor CD300lf is expressed on the apical surface of tuft cells, which are facing inward in the three-dimensional (3D) enteroid structure, the receptor is made accessible by plating enteroids-derived cells in 2D and polarizing the monolayer in transwells. This in vitro system supports MNV infection of tuft cells from the apical surface. Contrary to in vivo infections, both acute and persistent strains of MNV similarly infect tuft cells in this culture model (Strine et al., 2022). This model enables the study of MNV interaction with murine tuft cells.
Neonatal infections with MNV
Human noroviruses are a leading cause of severe childhood diarrhea worldwide. However, very little is known about the pathogenic mechanisms underlying norovirus diarrhea, principally because of the lack of tractable small animal models. Mice are a versatile small animal model for MNV and the MNV model has greatly facilitated progress in understanding host-norovirus interactions and norovirus strain variability (Karst & Wobus, 2015; Wobus et al., 2006). However, the model remains limited because immunocompetent adult mice do not develop diarrhea upon MNV infection, and mice lack the emetic reflex. While diarrhea was observed in MNV-infected interferon-deficient adult mice, these hosts also develop systemic pathology and lethality, confounding translatability of findings to human norovirus disease. Thus, the mechanistic basis of norovirus diarrhea has remained unclear. Recently, we developed a norovirus small animal disease model in wild-type mice by demonstrating that MNV-1 causes self-resolving diarrhea in neonatal C57Bl6 mice (Roth et al., 2020). We have now extended these studies to Balb/c mice and another MNV strain, WU23, that was isolated from a diarrhetic mouse (Peiper et al., 2023). These models mirror key features of human norovirus disease, such as acute self-resolving diarrhea and weight loss, and thus enable studies to understand the mechanism of norovirus diarrhea.
Critical Parameters and Troubleshooting
MNV antiviral efficacy determination (see Table 5)
- The health and quality of the cells (passage number below 20 for RAW264.7 cells) plated into the 96-well plate will determine the reproducibility of results.
- It is important to know the DMSO sensitivity of the cells. Different cell lines have differing sensitivities, and this affects the interpretation of results.
- Be careful to avoid ATP contamination.
- MNV control infection should show >90% death.
Problem | Possible cause | Solution |
---|---|---|
High luminescent reading for all wells | Bacterial contamination of CellTiter Glo | Use a fresh aliquot stored at −20°C |
Luminescence signal of MNV infected and cells-only controls are similar | MNV infection of cells has not progressed enough | Extend the time course of infection |
MNV qRT-PCR protocol
Follow the critical parameters previously outlined (Hwang et al., 2014), additionally:
- All plastic consumables including tips and mixing tubes should be RNase-DNase-free for all steps of this protocol.
- Using commercial DNAse and Rnase free H2O dedicated for primer and probe resuspension is highly recommended.
- Setting up separate, dedicated areas for different processes in the qPCR workflow, e.g., RNA extraction, standard preparation, master mix preparation with its own set of pipettors, tips, and microcentrifuge tubes helps to avoid cross-contamination.
- Never use autoclaved materials, such as filter-tips or tubes, as the autoclave can be a source for nucleic acid contamination.
- The DNA and RNA PCR standard should be aliquoted in single-use aliquots and stored accordingly.
- When pipetting the qPCR plates, it is recommended to pipette the samples first, then the standard (from the lowest concentration to the highest) and finally the negative controls.
MNV reverse genetics protocol (see Table 6)
- Cells used should be in a healthy growing state and with a reasonable passage number.
- Work swiftly if possible and always keep the RNA on ice.
- To verify successful transfection, the RNA concentration should be determined. However, this cannot readily be measured by a NanoDrop spectrophotometer after the capping reaction without an extra purification step. It is therefore recommended to determine the RNA concentration after purification and estimate the RNA concentration after capping based on the RNA-control gel.
- If the RNA control gel shows a smear due to partial digestion it is better to start over as it will have great impact on viral yields
Problem | Possible cause | Solution |
---|---|---|
Purified linearized DNA shows lower band | DNA degradation, possibly due to high temperature and/or poor handling | If only very faint, typically can be ignored as this should not affect IVT; if prominent, perform linearization and purification again working faster and ensuring DNA is kept cool |
RNA gel shows smears in bands | RNA degradation | If capped product and/or others are smeared, clean the electrophoresis chamber with a solution containing NaOH and run another gel; if capped end product is as expected, continue with transfection |
MNV CPER protocol (see Table 7)
- The design and quality of the primers are one of the key issues for the CPER reaction. Ensure that there is at least a 25-35 bp overlap with the primers and that the annealing temperatures are close to equal.
- Ensure a high-fidelity DNA polymerase is used.
- We found that the CMV promoter worked in the linker and the use of EF1a did not result in the recovery of infectious virus.
- All cells used for transfection should be highly transfectable (i.e., NIH3T3 or HEK 293T) and in a healthy growing state.
- We have found that for virus amplification after transfer of the transfection supernatant that the RAW264.7 cells should be a of a low passage number i.e. , no greater than 20 passages.
Problem | Possible cause | Solution |
---|---|---|
No infectious virus is recovered | Primers do not amplify fragments | Check that the overlapping areas of the primers have the same melting temperature (we suggest ∼55°C) |
Purity of DNA fragments is low | Re-purify the fragments after gel extraction until 260/280 and 260/230 ratios are optimal for DNA | |
CPER ligation did not occur | Check the CPER ligation reaction by gel electrophoresis, you should see the complete ligated product | |
Transfect immediately after the CPER reaction is complete, i.e., do not freeze CPER reaction (it is ok to freeze the fragments though) | ||
No CPE after 3 days | Tranfection efficiency is low | Keep monitoring the transfected cells for several additional days (up to 7 days) to allow for virus to spread through culture |
Baculovirus expression of NS1-2 (see Table 8)
- The overall quality of the T. ni insect cells will determine the success of the baculovirus infection and the production of high-quality protein. Routinely count cells and check viability during cell maintenance. Keep cell viability above 90%, discard cells if they drop below this. To maintain exponential growth of T. ni cells and to prevent clumping when they reach a high density, split cells every 2 days at 8 × 105 cells/ml. Cells can be split every 3 days at 5 × 105 cells/ml but this can increase the likelihood of cell aggregation.
- To generate good quality protein, a time course needs to be performed prior to expression to determine the best time to harvest for when the most protein is expressed and before the state of the infected cells begins to affect protein quality.
- To reduce viscosity of the cell lysate, include RNAse and DNAse, shear with a 20-G needle and filter through a 0.8-micron filter, this will prevent the solution from blocking on the gravity flow column.
Problem | Possible cause | Solution |
---|---|---|
No protein expressed | Titer of baculovirus too low | Plaque assay inoculum to determine titer; re-amplify the inoculum through Sf21 insect cells |
Multiple NS1-2 protein bands detected after purification | Baculovirus infection allowed to progress for too long or too high an m.o.i. was used | Optimize the point of harvest by time course; determine cell viability before harvest, optimum viability at harvest should be ∼50%-70% |
MNV infection of intestinal enteroids (see Table 9)
- Generating a single cell suspension prior to plating on the transwell is essential for an optimal monolayer.
- A second wash step during crypt isolation is important to remove mesenchymal cells.
- Differentiation with 50 ng/ml rIL-4 is critical for tuft cell differentiation and infection.
- General tips:
Pre-wet (using medium or FBS) pipette tips before manipulating intestinal pieces or crypts to prevent them from sticking to the wall of the pipettes. Make sure to remove the mesentery/membrane around the intestine before processing as it may be difficult to spin it out later in the process. Use gentle dissociation reagents to avoid too much damage to the intestine. When incubating with the dissociation medium to generate the single cell suspension, the next steps need to be done as quickly as possible, as the cells will start to clump together. Usually, monolayers (transwell) can reach 100% confluency within ∼3 days, but if the attachment is poor, this may take longer. Monolayers with good attachments can last at least 3 weeks, with proper maintenance (i.e., change of medium every 2 days).
Problem | Possible cause | Solution |
---|---|---|
Poor recovery of enteroids from mice | Sub-optimal crypt dissociation; if cells are not treated long enough with collagenase, the crypts will not dissociate, and if cells are treated too long, cell viability will be poor | Examine the cells under the microscope every 10 min during the 30 min dissociation period to ensure optimal dissociation; adjust incubation time accordingly |
Inadequate monolayer on transwells | Transwell was not properly coated or plated with single-cell suspension | Pre-coat entire transwell surface evenly; pre-wet pipette tips, ensure cells are in a single cell suspension before adding to transwell; use lobular rather than spheroidal enteroids to create single cell suspension |
Neonatal infections with MNV (see Table 10)
One of the most important factors for observing intestinal disease is the age of the mice at initial infection. MNV intestinal disease is highly age restricted with ∼80% incidence of disease at 48 hpi when mice are infected at P3-P5 with incidence dropping to ∼30% by P7 (Peiper et al., 2023). The infection dose is another major determinant in the development of intestinal disease; 80% mice infected with 1 × 107 TCID50 units of MNV develop intestinal disease at 48 hpi, but only 40% of mice develop disease when infected with a one log lower dose (Roth et al., 2020).
Problem | Possible cause | Solution |
---|---|---|
The neonatal mice do not defecate when abdomen is palpated | The neonatal mice are not eating as they are too ill to eat | Check the weights of the mice; if the weights are decreasing, virus dose likely needs to be decreased |
The neonatal mouse has defecated recently, and colon is empty, or the stool is too proximal in the colon | Each mouse can be palpated up to 3 times to induce defecation; after 3 attempts, the mouse needs to be returned to the dam, and palpation can be repeated in 1-2 hr |
Time Considerations
MNV antiviral efficacy determination
The whole assay will take two days. Allow ∼1.5 hours for setting up inhibitor dilutions and addition of virus. Harvesting of the plate takes ∼30 min with another 30 min to analyze the data.
MNV qRT-PCR protocol
Time for the RNA extraction depends on the number of samples and the method used. For a TRIzol extraction using the column-based spin protocol, this can be done within 30 min for a set ∼10 samples. Preparation of the standard dilutions and aliquoting takes ∼1 hr and lasts for many qRT-PCR runs. Pipetting the qRT-PCR requires ∼30 min for a 96-well plate if the workspace is setup. The qRT-PCR run takes ∼1 hr 15 min depending on the cycler.
MNV reverse genetics protocol
Plasmid linearization and purification takes ∼3 hr. This can be prepared in advance and stored at −20°C (day 1). The generation of in vitro transcribed RNA, purification, and capping takes ∼5 hr (day 2), storage at −80°C. The initial transfection of Huh7mCD300lf cells takes ∼1 hr but requires a medium change after 4 hr (day 3). Amplification of MNV from transfection of Huh7mCD300lf cells takes another 48 hr for recombinant wild type and maybe 96 hr for viruses with mutations leading to reduced fitness.
MNV CPER protocol
The PCR amplification of the linker and fragments will take ∼2.5 hr and an additional 1 hr for purification and gel analysis. The CPER reaction itself takes ∼90 min and the transfection ∼30 min. The initial transfection of NIH3T3 cells occurs for 3 days and the amplification of MNV in RAW264.7 cells takes another 48-72 hr.
Baculovirus expression of NS1-2
The baculovirus protein expression in insect cells will generally take two to three days, determined by a prior time-course. The harvest and subsequent protein purification can take ∼3 hr.
MNV infection of intestinal enteroids
Crypt isolation takes several hours depending on how many mice/genetic backgrounds are being used. Enteroid passaging requires several hours every 3-5 days depending on how dense the cells are seeded and the growth rate. Virus infection itself is less than one hour.
Neonatal infections with MNV
The time commitment for infecting neonatal mice depends on the number of pups to be infected but takes ∼1 hr. The total time for the experiment depends on the experimental design but is typically 3 days. The kinetics of intestinal disease are determined by the viral dose at initial infection. With an infection of 1 × 107 TCID50 units of MNV, disease starts to develop by 24 hpi. Peak intestinal disease is observed at 48 hpi with a disease plateauing at 72 hpi and fully resolving within 96 hpi.
Acknowledgments
C.E.W. was supported by NIH R21 AI 154647, S.M.K. by NIH R01AI162970 and R01AI141478, C.B.W. by NIH R01 AI148467, S.T. by the DFG research unit VIROCARB (FOR2327, TA1093-2-2), A.M.P. by NIH T32AI007110, and E.W.H. by NIH F30AI154834. Research in the lab of V.W. was funded by the New Zealand Ministry for Business Innovation and Employment, grant number UOOX1904. J.M.M. was funded by the National Health and Medical Research Council of Australia, grant number 1123135, and the Miller Foundation.
Author Contributions
Christiane E. Wobus : Conceptualization, funding acquisition, writing – original draft, writing – review and editing; Amy M. Peiper : Writing – original draft, writing – review and editing; Alice M. McSweeney : Writing – original draft, writing – review and editing; Vivienne L. Young : Writing – original draft, writing – review and editing; Maryna Chaika : Writing – original draft, writing – review and editing; Miranda Sophie Lane : Writing – original draft, writing – review and editing; Marit Lingemann : Writing – original draft, writing – review and editing; Joshua M. Deerain : Writing – original draft; Madison S. Strine : Writing – original draft, writing – review and editing; Mia Madel Alfajaro : Writing – original draft, writing – review and editing; Emily W. Helm : Writing – original draft, writing – review and editing; Stephanie M. Karst : Funding acquisition, writing – original draft, writing – review and editing; Jason M. Mackenzie : Funding acquisition, writing – original draft, writing – review and editing; Stefan Taube : Funding acquisition, writing – original draft, writing – review and editing; Vernon K. Ward : Funding acquisition, writing – original draft, writing – review and editing; Craig B. Wilen : Funding acquisition, writing – original draft, writing – review and editing.
Conflict of Interest
The funding sources of all authors had no conflicting interests with the protocols and research described within, nor did they have a role in protocol design and the collection, analysis, and interpretation of data.
Open Research
Data Availability Statement
Data sharing is not applicable to this article as no new data were created or analyzed in this study.
Literature Cited
- Amarilla, A. A., Sng, J. D. J., Parry, R., Deerain, J. M., Potter, J. R., Setoh, Y. X., Rawle, D. J., Le, T. T., Modhiran, N., Wang, X., Peng, N. Y. G., Torres, F. J., Pyke, A., Harrison, J. J., Freney, M. E., Liang, B., McMillan, C. L. D., Cheung, S. T. M., Guevara, D., … Khromykh, A. A. (2021). A versatile reverse genetics platform for SARS-CoV-2 and other positive-strand RNA viruses. Nature Communications , 12(1), 3431. https://doi.org/10.1038/s41467-021-23779-5
- Arias, A., Urena, L., Thorne, L., Yunus, M. A., & Goodfellow, I. (2012). Reverse genetics mediated recovery of infectious murine norovirus. Journal of Visualized Experiments: JoVE , (64), 4145. https://doi.org/10.3791/4145
- Condreay, J. P., Witherspoon, S. M., Clay, W. C., & Kost, T. A. (1999). Transient and stable gene expression in mammalian cells transduced with a recombinant baculovirus vector. Proceedings of the National Academy of Sciences of the United States of America , 96(1), 127–132. https://doi.org/10.1073/pnas.96.1.127
- Current Protocols. (1994). Extraction and Precipitation of DNA. Current Protocols in Human Genetics , 00, A.3C.1–A.3C.4. https://doi.org/10.1002/0471142905.hga03cs00
- Current Protocols. (2006). Commonly Used Reagents. Current Protocols in Microbiology , 00, A.2A.1–A.2A.15. https://doi.org/10.1002/9780471729259.mca02as00
- Duizer, E., Schwab, K. J., Neill, F. H., Atmar, R. L., Koopmans, M. P., & Estes, M. K. (2004). Laboratory efforts to cultivate noroviruses. Journal of General Virology , 85(Pt 1), 79–87. https://doi.org/10.1099/vir.0.19478-0
- Estes, M. K., Ettayebi, K., Tenge, V. R., Murakami, K., Karandikar, U., Lin, S. C., Ayyar, B. V., Cortes-Penfield, N. W., Haga, K., Neill, F. H., Opekun, A. R., Broughman, J. R., Zeng, X. L., Blutt, S. E., Crawford, S. E., Ramani, S., Graham, D. Y., & Atmar, R. L. (2019). Human norovirus cultivation in nontransformed stem cell-derived human intestinal enteroid cultures: Success and challenges. Viruses , 11(7), 683. https://doi.org/10.3390/v11070638
- Ettayebi, K., Crawford, S. E., Murakami, K., Broughman, J. R., Karandikar, U., Tenge, V. R., Neill, F. H., Blutt, S. E., Zeng, X. L., Qu, L., Kou, B., Opekun, A. R., Burrin, D., Graham, D. Y., Ramani, S., Atmar, R. L., & Estes, M. K. (2016). Replication of human noroviruses in stem cell-derived human enteroids. Science , 353(6306), 1387–1393. https://doi.org/10.1126/science.aaf5211
- Ettayebi, K., Tenge, V. R., Cortes-Penfield, N. W., Crawford, S. E., Neill, F. H., Zeng, X. L., Yu, X., Ayyar, B. V., Burrin, D., Ramani, S., Atmar, R. L., & Estes, M. K. (2021). New insights and enhanced human norovirus cultivation in human intestinal enteroids. mSphere , 6(1), e01136–20. https://doi.org/10.1128/mSphere.01136-20
- Farkas, T., Fey, B., Keller, G., Martella, V., & Egyed, L. (2012). Molecular detection of murine noroviruses in laboratory and wild mice. Veterinary Microbiology , 160(3-4), 463–467. https://doi.org/10.1016/j.vetmic.2012.06.002
- Fung, K. L., Kapoor, K., Pixley, J. N., Talbert, D. J., Kwit, A. D., Ambudkar, S. V., & Gottesman, M. M. (2016). Using the BacMam baculovirus system to study expression and function of recombinant efflux drug transporters in polarized epithelial cell monolayers. Drug Metabolism and Disposition , 44(2), 180–188. https://doi.org/10.1124/dmd.115.066506
- Gonzalez-Hernandez, M. B., Liu, T., Payne, H. C., Stencel-Baerenwald, J. E., Ikizler, M., Yagita, H., Dermody, T. S., Williams, I. R., & Wobus, C. E. (2014). Efficient norovirus and reovirus replication in the mouse intestine requires microfold (M) cells. Journal of Virology , 88(12), 6934–6943. https://doi.org/10.1128/JVI.00204-14
- Grau, K. R., Roth, A. N., Zhu, S., Hernandez, A., Colliou, N., DiVita, B. B., Philip, D. T., Riffe, C., Giasson, B., Wallet, S. M., Mohamadzadeh, M., & Karst, S. M. (2017). The major targets of acute norovirus infection are immune cells in the gut-associated lymphoid tissue. Nature Microbiology , 2(12), 1586–1591. https://doi.org/10.1038/s41564-017-0057-7
- Haga, K., Fujimoto, A., Takai-Todaka, R., Miki, M., Doan, Y. H., Murakami, K., Yokoyama, M., Murata, K., Nakanishi, A., & Katayama, K. (2016). Functional receptor molecules CD300lf and CD300ld within the CD300 family enable murine noroviruses to infect cells. Proceedings of the National Academy of Sciences of the United States of America , 113(41), E6248–E6255. https://doi.org/10.1073/pnas.1605575113
- Helm, E. W., Peiper, A. M., Phillips, M., Williams, C. G., Sherman, M. B., Kelley, T., Smith, H. Q., Jacobs, S. O., Shah, D., Tatum, S. M., Iyer, N., Grodzki, M., Morales Aparicio, J. C., Kennedy, E. A., Manzi, M. S., Baldridge, M. T., Smith, T. J., & Karst, S. M. (2022). Environmentally-triggered contraction of the norovirus virion determines diarrheagenic potential. Frontiers in Immunology , 13, 1043746. https://doi.org/10.3389/fimmu.2022.1043746
- Hsu, C. S., Ho, Y. C., Wang, K. C., & Hu, Y. C. (2004). Investigation of optimal transduction conditions for baculovirus-mediated gene delivery into mammalian cells. Biotechnology and Bioengineering , 88(1), 42–51. https://doi.org/10.1002/bit.20213
- Hwang, S., Alhatlani, B., Arias, A., Caddy, S. L., Christodoulou, C., Cunha, J. B., Emmott, E., Gonzalez-Hernandez, M., Kolawole, A., Lu, J., Rippinger, C., Sorgeloos, F., Thorne, L., Vashist, S., Goodfellow, I., & Wobus, C. E. (2014). Murine norovirus: Propagation, quantification, and genetic manipulation. Current Protocols in Microbiology , 33, 15K 12 11–61. https://doi.org/10.1002/9780471729259.mc15k02s33
- Irons, S. L., Chambers, A. C., Lissina, O., King, L. A., & Possee, R. D. (2018). Protein production using the baculovirus expression system. Current Protocols in Protein Science , 91, 5 5 1–5 5 22. https://doi.org/10.1002/cpps.45
- Jones, M. K., Grau, K. R., Costantini, V., Kolawole, A. O., de Graaf, M., Freiden, P., Graves, C. L., Koopmans, M., Wallet, S. M., Tibbetts, S. A., Schultz-Cherry, S., Wobus, C. E., Vinje, J., & Karst, S. M. (2015). Human norovirus culture in B cells. Nature Protocols , 10(12), 1939–1947. https://doi.org/10.1038/nprot.2015.121
- Karst, S. M., & Wobus, C. E. (2015). Viruses in rodent colonies: Lessons learned from murine noroviruses. Annual Review of Virology , 2(1), 525–548. https://doi.org/10.1146/annurev-virology-100114-055204
- Karst, S. M., Wobus, C. E., Lay, M., Davidson, J., & Virgin, H. W. IV. (2003). STAT1-dependent innate immunity to a Norwalk-like virus. Science , 299(5612), 1575–1578. https://doi.org/10.1126/science.1077905
- Kronqvist, N., Sarr, M., Lindqvist, A., Nordling, K., Otikovs, M., Venturi, L., Pioselli, B., Purhonen, P., Landreh, M., Biverstal, H., Toleikis, Z., Sjoberg, L., Robinson, C. V., Pelizzi, N., Jornvall, H., Hebert, H., Jaudzems, K., Curstedt, T., Rising, A., & Johansson, J. (2017). Efficient protein production inspired by how spiders make silk. Nature Communications , 8, 15504. https://doi.org/10.1038/ncomms15504
- Kwon, M., & Firestein, B. L. (2013). DNA transfection: Calcium phosphate method. Methods in Molecular Biology , 1018, 107–110. https://doi.org/10.1007/978-1-62703-444-9_10
- Lei, C., Yang, J., Hu, J., & Sun, X. (2021). On the calculation of TCID(50) for quantitation of virus infectivity. Virologica Sinica , 36(1), 141–144. https://doi.org/10.1007/s12250-020-00230-5
- Lingemann, M. (2020). New tools to study murine norovirus-host interactions. University of Lubeck.
- Liu, H., & Naismith, J. H. (2008). An efficient one-step site-directed deletion, insertion, single and multiple-site plasmid mutagenesis protocol. BMC Biotechnology [Electronic Resource] , 8, 91. https://doi.org/10.1186/1472-6750-8-91
- Miyoshi, H., & Stappenbeck, T. S. (2013). In vitro expansion and genetic modification of gastrointestinal stem cells in spheroid culture. Nature Protocols , 8(12), 2471–2482. https://doi.org/10.1038/nprot.2013.153
- Moon, C., VanDussen, K., Miyoshi, H., & Stappenbeck, T. (2014). Development of a primary mouse intestinal epithelial cell monolayer culture system to evaluate factors that modulate IgA transcytosis. Mucosal Immunology , 7(4), P818–828. https://doi.org/10.1038/mi.2013.98
- Ni, D., Xu, P., & Gallagher, S. (2016). Immunoblotting and immunodetection. Current Protocols in Molecular Biology , 114, 10.8.1–10.8.37. https://doi.org/10.1002/0471142727.mb1008s114
- Nice, T. J., Strong, D. W., McCune, B. T., Pohl, C. S., & Virgin, H. W. (2013). A single-amino-acid change in murine norovirus NS1/2 is sufficient for colonic tropism and persistence. Journal of Virology , 87(1), 327–334. https://doi.org/10.1128/JVI.01864-12
- Orchard, R. C., Wilen, C. B., Doench, J. G., Baldridge, M. T., McCune, B. T., Lee, Y. C., Lee, S., Pruett-Miller, S. M., Nelson, C. A., Fremont, D. H., & Virgin, H. W. (2016). Discovery of a proteinaceous cellular receptor for a norovirus. Science , 353(6302), 933–936. https://doi.org/10.1126/science.aaf1220
- Peiper, A. M., Helm, E. W., Nguyen, Q., Phillips, M., Williams, C. G., Shah, D., Tatum, S., Iyer, N., Grodzki, M., Eurell, L. B., Nasir, A., Baldridge, M. T., & Karst, S. M. (2023). Infection of neonatal mice with the murine norovirus strain WU23 is a robust model to study norovirus pathogenesis. Lab Animal , 52(6), 119–129. https://doi.org/10.1038/s41684-023-01166-5
- Roth, A. N., Helm, E. W., Mirabelli, C., Kirsche, E., Smith, J. C., Eurell, L. B., Ghosh, S., Altan-Bonnet, N., Wobus, C. E., & Karst, S. M. (2020). Norovirus infection causes acute self-resolving diarrhea in wild-type neonatal mice. Nature Communications , 11(1), 2968. https://doi.org/10.1038/s41467-020-16798-1
- Sitarska, A., Skora, L., Klopp, J., Roest, S., Fernandez, C., Shrestha, B., & Gossert, A. D. (2015). Affordable uniform isotope labeling with (2)H, (13)C and (15)N in insect cells. Journal of Biomolecular NMR , 62(2), 191–197. https://doi.org/10.1007/s10858-015-9935-6
- Smith, D. B., McFadden, N., Blundell, R. J., Meredith, A., & Simmonds, P. (2012). Diversity of murine norovirus in wild-rodent populations: Species-specific associations suggest an ancient divergence. Journal of General Virology , 93(Pt 2), 259–266. https://doi.org/10.1099/vir.0.036392-0
- Strine, M. S., Alfajaro, M. M., Graziano, V. R., Song, J., Hsieh, L. L., Hill, R., Guo, J., VanDussen, K. L., Orchard, R. C., Baldridge, M. T., Lee, S., & Wilen, C. B. (2022). Tuft-cell-intrinsic and -extrinsic mediators of norovirus tropism regulate viral immunity. Cell Reports , 41(6), 111593. https://doi.org/10.1016/j.celrep.2022.111593
- Strine, M. S., & Wilen, C. B. (2022). Tuft cells are key mediators of interkingdom interactions at mucosal barrier surfaces. Plos Pathogens , 18(3), e1010318. https://doi.org/10.1371/journal.ppat.1010318
- Taube, S., Perry, J. W., Yetming, K., Patel, S. P., Auble, H., Shu, L., Nawar, H. F., Lee, C. H., Connell, T. D., Shayman, J. A., & Wobus, C. E. (2009). Ganglioside-linked terminal sialic acid moieties on murine macrophages function as attachment receptors for murine noroviruses. Journal of Virology , 83(9), 4092–4101. https://doi.org/10.1128/JVI.02245-08
- Thackray, L. B., Wobus, C. E., Chachu, K. A., Liu, B., Alegre, E. R., Henderson, K. S., Kelley, S. T., & Virgin, H. W. IV. (2007). Murine noroviruses comprising a single genogroup exhibit biological diversity despite limited sequence divergence. Journal of Virology , 81(19), 10460–10473. https://doi.org/10.1128/JVI.00783-07
- van Dycke, J., Ny, A., Conceicao-Neto, N., Maes, J., Hosmillo, M., Cuvry, A., Goodfellow, I., Nogueira, T. C., Verbeken, E., Matthijnssens, J., de Witte, P., Neyts, J., & Rocha-Pereira, J. (2019). A robust human norovirus replication model in zebrafish larvae. Plos Pathogens , 15(9), e1008009. https://doi.org/10.1371/journal.ppat.1008009
- van Winkle, J. A., Robinson, B. A., Peters, A. M., Li, L., Nouboussi, R. V., Mack, M., & Nice, T. J. (2018). Persistence of systemic murine norovirus is maintained by inflammatory recruitment of susceptible myeloid cells. Cell Host & Microbe, 24(5), 665–676 e664. https://doi.org/10.1016/j.chom.2018.10.003
- Voytas, D. (2000). Agarose Gel Electrophoresis. Current Protocols in Molecular Biology , 51, 2.5A.1–2.5A.9. https://doi.org/10.1002/0471142727.mb0205as51
- Ward, V. K., McCormick, C. J., Clarke, I. N., Salim, O., Wobus, C. E., Thackray, L. B., Virgin, H. W. T., & Lambden, P. R. (2007). Recovery of infectious murine norovirus using pol II-driven expression of full-length cDNA. Proceedings of the National Academy of Sciences of the United States of America , 104(26), 11050–11055. https://doi.org/10.1073/pnas.0700336104
- Wilen, C. B., Lee, S., Hsieh, L. L., Orchard, R. C., Desai, C., Hykes, B. L. Jr., McAllaster, M. R., Balce, D. R., Feehley, T., Brestoff, J. R., Hickey, C. A., Yokoyama, C. C., Wang, Y. T., MacDuff, D. A., Kreamalmayer, D., Howitt, M. R., Neil, J. A., Cadwell, K., Allen, P. M., … Virgin, H. W. (2018). Tropism for tuft cells determines immune promotion of norovirus pathogenesis. Science , 360(6385), 204–208. https://doi.org/10.1126/science.aar3799
- Wobus, C. E., & Green, K. Y. (2020). Caliciviridae: The viruses and their replication. In D. M. K. Peter, M. Howley, & Sean Whelan (Eds.), Fields virology: Emerging viruses ( 7th ed., Vol. 1, pp. 129–169). Wolters Kluwer.
- Wobus, C. E., Karst, S. M., Thackray, L. B., Chang, K. O., Sosnovtsev, S. V., Belliot, G., Krug, A., Mackenzie, J. M., Green, K. Y., & Virgin, H. W. (2004). Replication of norovirus in cell culture reveals a tropism for dendritic cells and macrophages. Plos Biology , 2(12), e432. https://doi.org/10.1371/journal.pbio.0020432
- Wobus, C. E., Thackray, L. B., & Virgin, H. W. T. (2006). Murine norovirus: A model system to study norovirus biology and pathogenesis. Journal of Virology , 80(11), 5104–5112. https://doi.org/10.1128/JVI.02346-05
- Zufferey, R., Dull, T., Mandel, R. J., Bukovsky, A., Quiroz, D., Naldini, L., & Trono, D. (1998). Self-inactivating lentivirus vector for safe and efficient in vivo gene delivery. Journal of Virology , 72(12), 9873–9880. https://doi.org/10.1128/JVI.72.12.9873-9880.1998
Internet Resources
Lentivector Toolbox from the Trono lab outlining a protocol for the lentivirus vector generation.
Online calculator to calculate the amount of DNA given the size of each fragment.