Models of Depression: Unpredictable Chronic Mild Stress in Mice
Mathieu Nollet, Mathieu Nollet
Abstract
Major depression is a complex psychiatric disorder characterized by affective, cognitive, and physiological impairments that lead to maladaptive behavior. The high lifetime prevalence of this disabling condition, coupled with limitations of existing medications, make necessary the development of improved therapeutics. This requires animal models that allow investigation of key biological correlates of the disorder. Described in this article is the unpredictable chronic mild stress mouse model that can be used to screen for antidepressant drug candidates. Originally designed for rats, this model has been adapted for mice to capitalize on the advantages of this species as an experimental model, including inter-strain variability, which permits an exploration of the contribution of genetic background; the ability to create transgenic animals; and lower cost. Thus, because it combines genetic features and socio-environmental chronic stressful events, the unpredictable chronic mild stress model in mice is a relevant and valuable paradigm to gain insight into the etiological and developmental components of major depression, as well as to identify novel treatments for this condition. © 2021 The Authors. Current Protocols published by Wiley Periodicals LLC.
Basic Protocol 1 : Unpredictable Chronic Mild Stress (UCMS) Test in Mice
Basic Protocol 2 : Assessment Of Self-Directed Activity And Anhedonia in Mice
INTRODUCTION
The unpredictable chronic mild stress (UCMS) rat model of depression was first proposed by Katz in 1982 and developed further by Papp and Willner (Willner, 2017). The model involves exposing the animal at unpredictable times over several weeks to a series of minor-intensity stressors. This results in the development of a number of behavioral alterations in a large majority of animals (some animals can be more stress-resistant), including anhedonia (loss of pleasure) and apathy. These behavioral changes, together with alterations in certain endocrine and neural variables, resemble those found in individuals suffering from major depressive disorder and, as with the clinical condition, are reversed by a wide range of antidepressant drugs with different mechanisms of action (Willner, 2017). This model is considered by many as one of the more useful animal tests for antidepressant activity. The UCMS displays face, construct, and predictive validity, and is one of the few models in which chronic, but not acute, monoaminergic antidepressant administration is effective (Belzung & Lemoine, 2011; Willner, 2017). Furthermore, the UCMS also responds to newer rapid-acting antidepressants, including the N -methyl-D-aspartate (NMDA) receptor antagonist ketamine (Ma et al., 2013; Okine et al., 2020; Fitzgerald et al., 2021).
The UCMS model has also been validated in mice (Surget & Belzung, 2009), which have several technical and practical advantages over rats as experimental animals. These include (1) inter-strain variability, which allows an examination of the contribution of genetic background, (2) the availability of transgenic animals to facilitate the identification of key genes involved in the development of these behaviors and the response to drugs, and (3) lower cost as compared to rats. Advances in transcriptomics have provided an opportunity to dissect pathophysiological mechanisms at a molecular level and offer a window onto the functional implication of gene expression changes in the UCMS (Musaelyan et al., 2020; Nollet et al., 2019). Moreover, transcriptomic analysis of clinically used and putative antidepressant responses offers a valuable window on their mechanisms of action (Hervé et al., 2017; Surget et al., 2009), but more data are needed to clarify the specific effects of different classes of drugs on the transcriptome in the UCMS model, especially for recently described rapid-onset antidepressants. The mouse version of this model also has a high translational value as it induces molecular changes that are similar to those observed in depressed patients (Hervé et al., 2017; Sibille et al., 2009).
NOTE : All protocols using live animals must first be reviewed and approved by an Institutional Animal Care and Use Committee (IACUC) or must conform to governmental regulations regarding the care and use of laboratory animals.
Basic Protocol 1: UNPREDICTABLE CHRONIC MILD STRESS (UCMS) TEST IN MICE
Mice are subjected to a schedule of mild psychosocial stressors for 9 weeks (Fig. 1) to induce physical, behavioral, biochemical, and physiological phenotypes. Once these are established, their reversal following the chronic administration of test agents can be used to predict potential antidepressant activity. Physical changes are quantified by measuring the coat state and body weight of the animal. Stressed animals display a worsening of the coat state compared to control, non-stressed animals. This change in coat state is reversed by chronic administration of antidepressants.
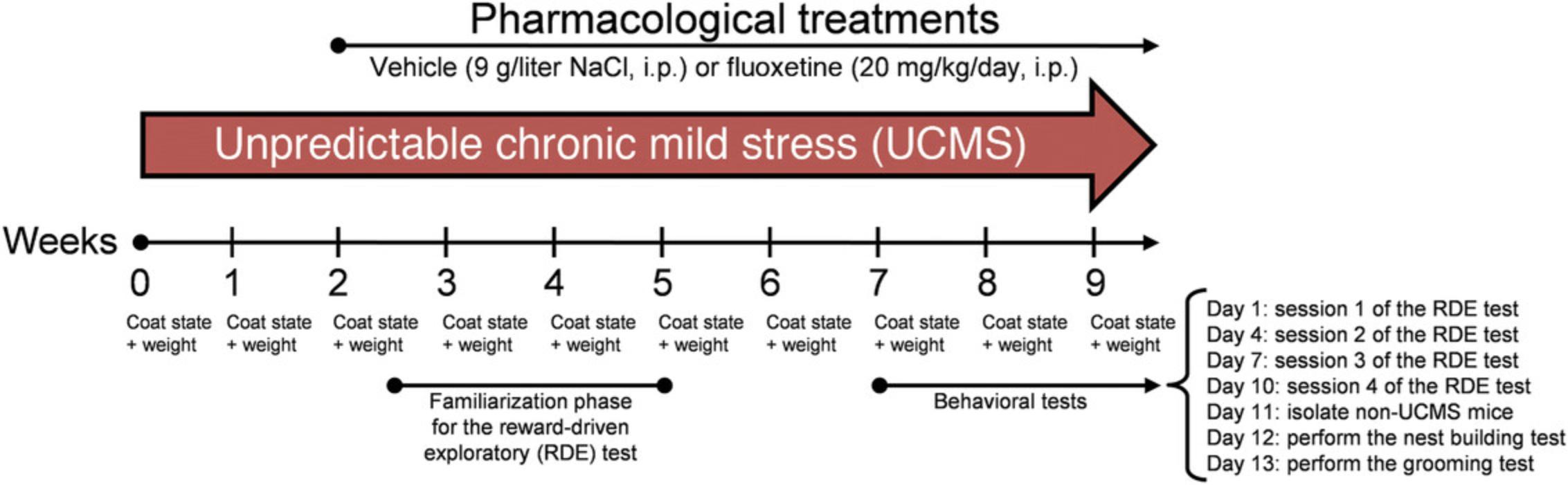
Materials
- Male BALB/c mice (n = 64), aged 8 weeks at the beginning of the experiment (e.g., Charles River)
- Food pellets and water ad libitum
- Stressors (see Table 1) including:
- Soiled sawdust of rats from the same or previous day (with feces and urine)
- Timer for changing the light/dark cycle for the stressed animals with a minimum accuracy of 30 min
- Spruce sawdust
Stressor | Description |
---|---|
Social stress | Each mouse is placed in an empty cage previously occupied by another individual. |
Cage change | Each mouse is placed in the empty cage of another individual, and then returned to its original cage. |
Sawdust change | At the beginning of the UCMS protocol, the sawdust is changed 2-3 times per 24 hr, and up to 6 times per 24 hr at the end of the UCMS regimen. It is also possible to replace clean sawdust by soiled sawdust coming from control mice. |
Without sawdust | The sawdust is removed during 1 to 6 hr. |
Damp sawdust | 125 ml water is placed in each cage (depending on the quantity of sawdust). The period during which damp sawdust is provided can range from 1 to 6 hr. |
Water stress | The sawdust in each cage is removed and replaced with ∼125 ml water at 20°C (∼1 cm of water) for 15-30 min. |
Cage tilting | The cages are tilted backwards (45 degrees) for 1-4 hr. |
Rat feces | About 60 ml of rat sawdust is deposited in each cage for a period of 1-2 hr. |
Restraint stress | The mice are kept in closed and ventilated tubes (6.5-cm length × 3.7-cm i.d.) for 15-30 min (mice have the possibility to turn themselves back into the tube). |
Cycle disturbances | Change in the light/dark cycle (e.g., complete reversal of the light/dark cycle, division of the light/dark cycle into four periods of 6 hr, or addition of one to several illumination periods of 30 min to 2 hr during the dark phase and vice versa). |
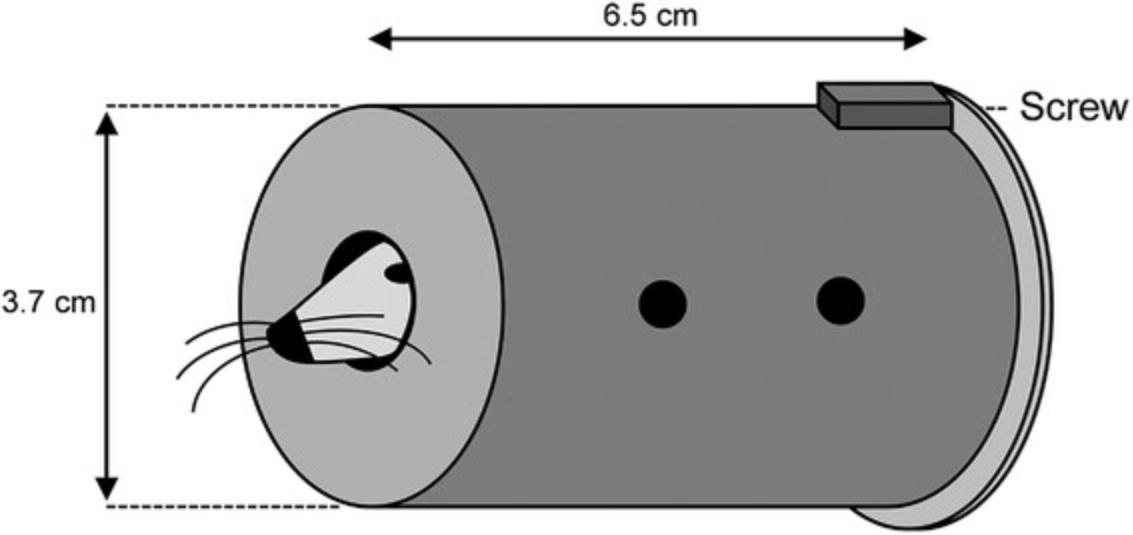
Acclimatize the animal
1.At least 1 week before initiating the protocol, place the animals in groups of four or five per cage.
2.Individually mark the animals and separate them into two experimental groups: 32 mice will be exposed to the UCMS paradigm, while 32 mice will remain unstressed and serve as controls. House the stressed and control groups in different rooms but under the same time and temperature conditions, as noted above. Whereas the UCMS mice are housed individually in their home cage, the control mice are group-housed (four or five per cage) with environmental enrichment (shelters and tubes).
Apply stressors and assess physical condition
3.Subject the UCMS mice to stressors detailed in Table 1.Expose the animals to the stressors throughout the entire circadian period (i.e., during the dark and the light periods) and randomly (applying the different stressors in an unpredictable manner). To increase stress intensity and unpredictability, after the first week of the UCMS regimen, stressors can be combined (e.g., rat sawdust and restraint at the same time; Table 2).
Time | Condition |
---|---|
Monday morning | Social stress (9:00) + cage tilting (10:00-12:00) |
Monday afternoon | Restraint stress (14:00-14:30) + Water stress (16:30-17:00) |
Tuesday morning | Weight + coat state + sawdust change (10:00, 10:30, 11:00) |
Tuesday afternoon | Rat sawdust (14:00-16:00) + social stress (15:00-17:00) |
Wednesday morning | Damp sawdust (9:30-11:30) |
Wednesday afternoon | Light (12:00-14:00) + dark (14:00-16:00) + light (16:00-18:00) |
Thursday morning | Restraint stress (9:00-9:30) + sawdust change (10:30, 11:00) |
Thursday afternoon | Water stress (14:00-14:30) + social stress (17:00) |
Friday morning | Without sawdust (9:30-11:30) + cage tilting (10:00-11:30) |
Friday afternoon | Cage tilting (13:00-15:00) + social stress (17:00-19:00) |
Saturday (day) | Light (9:00-11:00) + dark (11:00-13:00) + light (13:00-15:00) + dark (15:00-19:00) |
Saturday (night) | Light (19:00-21:00) + dark (21:00-1:00) + light (1:00-3:00) + dark (3:00-9:00) |
Sunday (day) | Light (9:00-11:00) + dark (11:00-13:00) + light (13:00-15:00) + dark (15:00-19:00) |
Sunday (night) | Light (19:00-21:00) + dark (21:00-1:00) + light (1:00-3:00) + dark (3:00-9:00) |
- a
Example of stressors schedule during the third week with light ON at 19:00 and light ON at 07:00. To avoid sleep cycle disturbance, light change can be replaced by other stressors (Nollet et al., 2019).
4.Assess the body weight and coat state of mice weekly. Because the coat state is a function of the frequency and extent of grooming behavior, it is a measure of the animal's motivation toward self-centered activities. The coat state will vary from smooth and clear in control animals to bristling with spikes in UCMS-subjected mice that are most affected by the stressors. Assess the coat on the following seven body areas: head, neck, back, abdomen, tail, forepaws, and hindpaws (Fig. 3). Score the coat state for each area as follows: 0 (good) for smooth and shiny fur, with no tousled, spiky patches; 0.5 (moderate) for slightly fluffy fur with some spiky patches; 1 (bad) for unkempt fluffy fur with slight staining. Sum the scores for all seven body parts to obtain an overall score, with a maximum possible score of 7. Weigh the animals and monitor their overall physical condition.
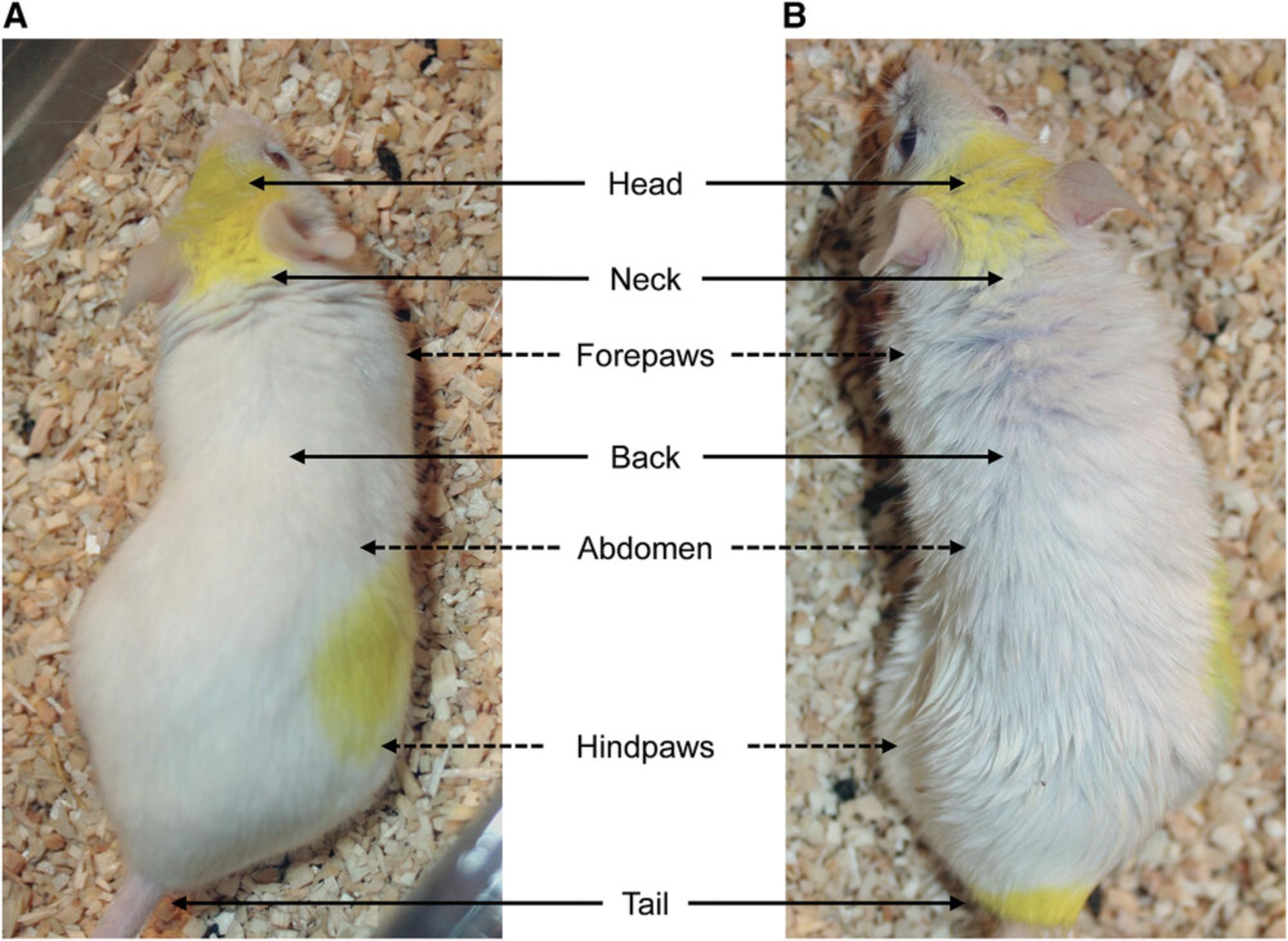
Administer the test compound
5.Further divide the 32 non-UCMS and 32 UCMS mice into two subgroups: control animals receiving vehicle (9 g/L NaCl, i.p.) and treated animals injected with antidepressant (fluoxetine, 15 mg/kg/day, i.p., or imipramine, 20 mg/kg/day, i.p.) at a volume of 10 ml/kg (16 mice per group). Administer vehicle and antidepressant to both non-UCMS and UCMS mice at a fixed time of the day (e.g., at the beginning of the dark period) for 2-4 weeks to assess the pharmacodynamic response.
Basic Protocol 2: ASSESSMENT OF SELF-DIRECTED ACTIVITY AND ANHEDONIA IN MICE
The UCMS should induce a depressive-like state. In humans, this is characterized by symptoms of apathy and anhedonia. In rodents, responses to stress and antidepressant effects can be assessed by measuring spontaneous grooming behavior (grooming test), spontaneous motivation (nest building test), and appetence for pleasurable food (cookie consumption in the reward-driven exploratory test). Unlike the weekly assessments of physical condition described in Basic Protocol 1, these tests are conducted during the final weeks of the UCMS procedure, at least 2 or 3 weeks after the beginning of the pharmacological treatments, to allow the compounds to induce their therapeutic effects (Fig. 1).
NOTE : The behavioral testing should be performed during the dark period and follow the order presented here, beginning with the test that requires a period of habituation (reward-driven exploratory test). Furthermore, behavioral tests can be stressful and can be considered as a stressor per se, hence the importance of including a non-stressed control group.
Materials
-
Four experimental groups of mice from the previous subdivisions in Basic Protocol 1 (non-UCMS/vehicle, non-UCMS/antidepressant, UCMS/vehicle, and UCMS/antidepressant; 16 mice per group)
-
Cookies (e.g., McVitie's Chocolate Digestives or Oreo cookies; the cookie must be appetent for mice in being somewhat crispy and sweet)
-
70% (v/v) alcohol
-
10% (w/v) sucrose solution (made with white sugar sold in conventional retail stores)
-
Apparatus containing three aligned chambers (20-cm length × 20-cm width × 20-cm height) differing only in the colors of the walls and floors (respectively, white, gray, and black for the first, second, and third chambers) and linked by two gates with a door controlled by the experimenter (Fig. 4)
-
Light dimmer and luxmeter
-
Stopwatches
-
32 individual cages for non-UCMS mice (for the nest building test and the grooming test)
-
Cotton nestlets (5 × 5 cm, 2-3 g; e.g., SERLAB, D0009, or LBS Biotech Nestlets)
-
One “spray” cage for the grooming test
-
1-L sprayer (e.g., garden hand sprayer) for the splash test
-
Lamp with red bulb
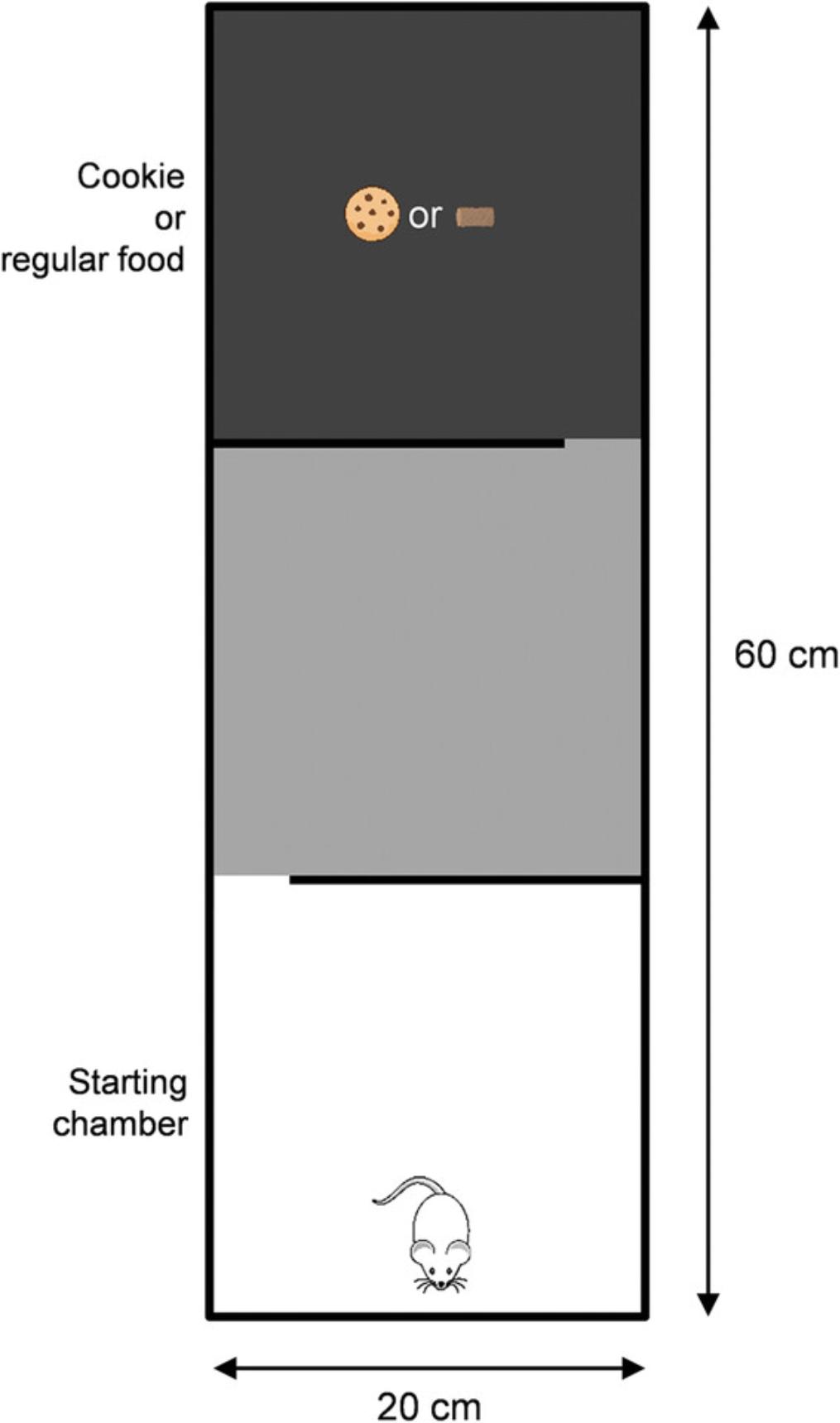
The reward-driven exploratory test
1a. Divide each experimental group (non-UCMS/vehicle, non-UCMS/antidepressant, UCMS/vehicle, and UCMS/antidepressant; sixteen mice per group) into two subgroups: mice receiving cookies and mice receiving regular food pellets (eight mice per group).
2a. Four and a half weeks before the start of the assessments, place a piece of cookie (∼2 g) in the cage of each mouse of the “cookie subgroup” every 2 days for 2.5 consecutive weeks to familiarize the animals with the palatable stimulus.
3a. One hour before the beginning of the test, retrieve all food from cages.
4a. Place a piece of cookie (∼2 cm × 2 cm) or a regular food pellet in the center of the black chamber and place the mouse at the other end of the apparatus in the white chamber (head facing opposite to the opening).
5a. During the 5-min test session, measure the time it takes (latency) for the mouse to pass through the first and the second gate (when its four legs have crossed the door) to smell the food and to chew it, and the number of times the mouse smells and chews the food.
6a. After the end of this first session, replace the mouse in its home cage.
7a. Perform this test every 3 days for each animal (i.e., four sessions of testing over 9 days).
The nest building test
1b. Because the stressed mice are already individually housed, isolate non-UCMS mice in clean individual cages for 24 hr before the test (Fig. 1).
2b. One hour before the beginning of the dark phase (active period), place a cotton nestlet in each cage.
3b. At two time points (5 and 23 hr after the beginning of the light phase), evaluate the nest quality (Deacon, 2006; Fig. 5) using the following criteria:
- Score 1: The cotton square is intact.
- Score 2: The cotton square is partially used.
- Score 3: The cotton is scattered but there is no form of nest.
- Score 4: The cotton is gathered but there is no nest (“flat nest”).
- Score 5: The cotton is gathered into a “ball” with a small passage for entry of the animal (as in an igloo; with or without roof).
The second evaluation of nest quality is performed 1 hr before the onset of the dark phase to avoid the destruction of the nest during the awakening of the mouse.
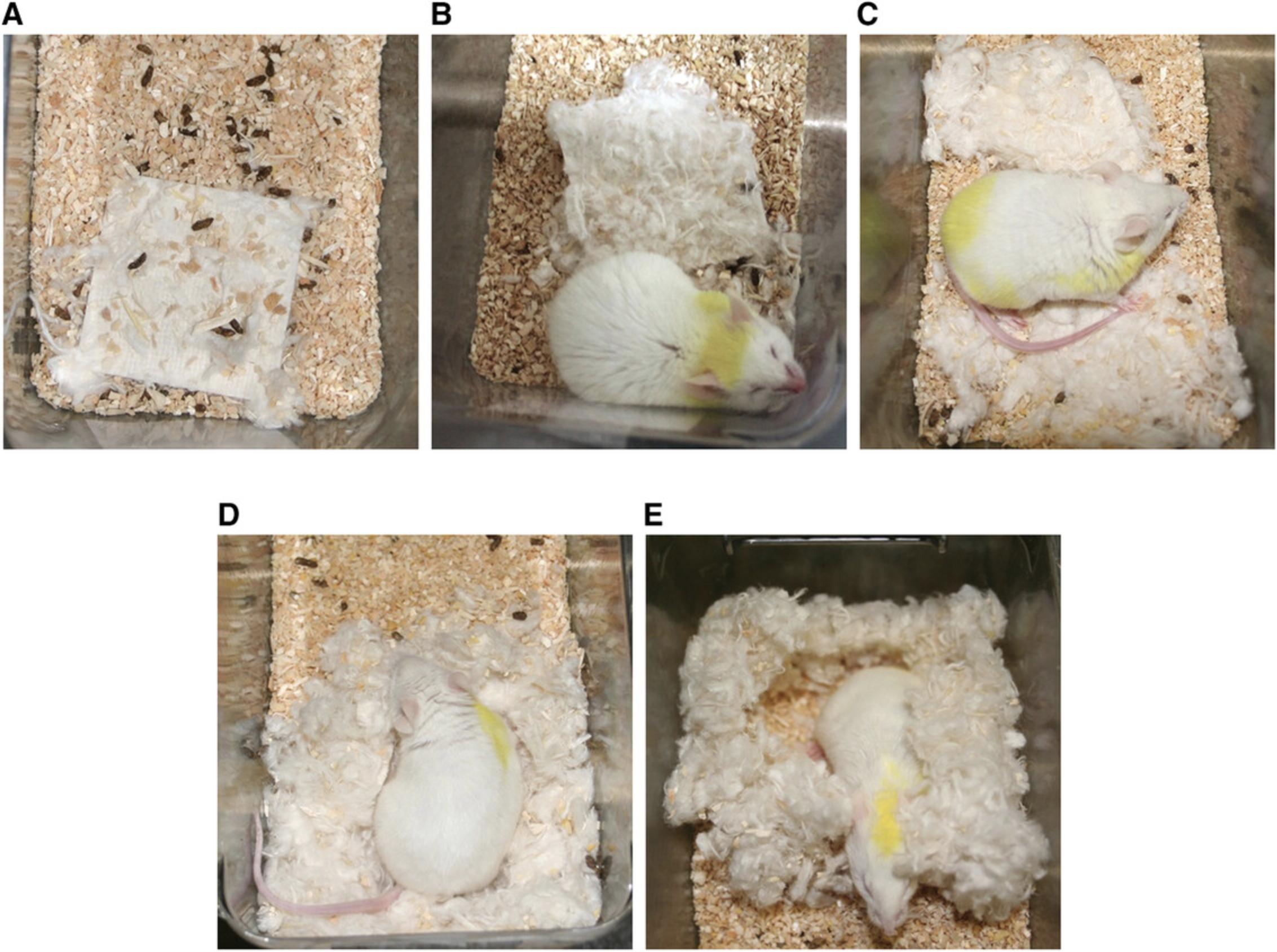
The grooming test
1c. Place a mouse in the “spray” cage.
2c. Spray the back of the mouse with a high-viscosity 10% sucrose solution to stimulate grooming behavior, and quickly place the mouse back into its home cage.
3c. Measure the latency to initiate the first grooming behavior, as well as the frequency and duration of grooming over a 5-min period. Start the stopwatch when the mouse is returned to its home cage, immediately after applying the sucrose solution.
COMMENTARY
Background Information
Chronic stress disrupts the overall homeostasis of the organism and contributes to the etiology of major depression in causing cognitive, behavioral, and physiological impairments (Surget & Belzung, 2009; Willner, 2017). The environmental factors triggering major depression include various psychosocial stressors (e.g., disturbed family environment, childhood sexual abuse, educational attainment, lifetime traumas, marital problems, the COVID-19 pandemic, etc.; Assari & Lankarani, 2016; Keller, Neale, & Kendler, 2007; Nelson, Klumparendt, Doebler, & Ehring, 2017; Pan et al., 2021). Moreover, stressful life events can precipitate a depressive episode in vulnerable subjects (Caspi et al., 2003; Zimmermann et al., 2011). Unlike animal models used to assess a symptom of major depression based on exposure to a relatively aversive acute stress, such as the forced swim and tail suspension tests (Pollak, Rey, & Monje, 2010), the UCMS mouse model was developed to study multiple disturbances resulting from chronic exposure to stress. This model therefore aims to reproduce a depressive-like state that emerges gradually in response to chronic stress, which is thought to be a major contributor in the development of clinical depression. Thus, the UCMS model has construct validity because, like human depression, the phenotype results from environmental and psychological stressors.
Although many of the core features of major depression, such as suicidal ideation and excessive guilt, are human prerogatives and therefore not reproducible in mice, UCMS animals display a long-lasting complex pathological syndrome resembling many of the symptoms/endophenotypes of human depression, including behavioral and cognitive alterations such as anhedonia, loss of interest, learning deficits, signs of despair, difficulty in decision making, and sleep disturbances (Surget & Belzung, 2009; Willner, 2017). These animals also display neurobiological abnormalities such as reduced levels of monoamines, immune system dysfunction, reduced hippocampal dendritic branching, increased branching in the amygdala, and decreased hippocampal neurogenesis. UCMS-subjected mice also exhibit endocrine changes and, in particular, an increase in glucocorticoid levels (Surget & Belzung, 2009; Willner, 2017). These behavioral, neurochemical, and endocrine alterations develop gradually after exposure to different stressors, indicating face validity for the model.
A key feature for an animal model of a psychiatric disorder is its ability to predict clinical efficacy for compounds designed to treat the pathological condition. This characteristic is essential to validate new targets for antidepressant action. The UCMS mouse model displays a variety of physical, behavioral, and neurobiological disturbances that are reversed, as in humans, by chronic administration of both conventional and atypical antidepressants (Surget & Belzung, 2009; Willner, 2017). Indeed, the UCMS-induced alterations can be reduced or abolished in mice by chronic treatment with a variety of conventional antidepressant drugs, including the tricyclics imipramine and desipramine, selective serotonin reuptake inhibitors (SSRIs) such as fluoxetine, and the selective noradrenaline reuptake inhibitor (SNRI) maprotiline (Yalcin, Belzung, & Surget, 2008). Other agents that have been reported to display antidepressant-like activity in UCMS animals include the opioid tramadol, which has a venlafaxine-like structure (Yalcin, Aksu, & Belzung, 2005); the corticotropin-releasing factor 1 (CRF1) receptor antagonists antalarmin and SSR125543 (Alonso et al., 2004; Ducottet, Griebel, & Belzung, 2003; Surget et al., 2008); the vasopressin 1b (V1b) receptor antagonist SSR149415 (Alonso et al., 2004; Griebel et al., 2002; Surget et al., 2008); the cannabinoid CB1 receptor antagonist rimonabant (Griebel, Stemmelin, & Scatton, 2005); the β3-adrenoreceptor agonist SR58611A (amibregon; Stemmelin et al., 2008); and the dual orexin receptor antagonist almorexant (Nollet et al., 2012). Furthermore, it is noteworthy that new pharmacological approaches, such as acute or subacute treatment with the NMDA receptor antagonist ketamine or metabotropic glutamate 2/3 receptor antagonist LY341495, can counteract the deleterious effects induced by UCMS in mice (Ma et al., 2013; Okine et al., 2020; Pałucha-Poniewiera, Podkowa, & Rafało-Ulińska, 2021). Psychoactive agents that do not display antidepressant activity in the clinic, such as pindolol, yohimbine, chlordiazepoxide, sulpiride, MK801, morphine, and 3,4-methylenedioxymethamphetamine (MDMA), are also ineffective in the UCMS model mouse (Surget & Belzung, 2009). Together, these findings substantiate the predictive validity of this model and emphasize its relevance as a screening test for antidepressants.
Critical Parameters and Troubleshooting
Stress procedure
The stressors used, which are mainly psychosocial, are mild in intensity, with the essential features being their chronic and unpredictable natures. For ethical and scientific reasons, severe stressors, such as food and water deprivation or painful stress, should not be employed. From a technical standpoint, moreover, these stressors may not parallel the etiology of human pathology, and their generalized effects on the animal's physical state and well-being might interfere with the measures of the UCMS-induced effects. Furthermore, if the nature or timing of the proposed stressors could interfere with the interpretation of the experimental results, they should be avoided. For instance, a study of UCMS-induced alterations of sleep-related parameters should not include disturbances of the light/dark cycle or any stressors during the light period (Nollet et al., 2019). Also, because the UCMS paradigm requires a large number of animals, it is possible to divide the animals into different cohorts that do not begin the UCMS regimen at the same time, with up to a 1-week lag between cohorts (Fig. 6). Although this facilitates the behavioral testing phase by minimizing the number of mice involved, it means that the animal groups are not subjected to the same stressors simultaneously, which creates an additional variable when interpreting the results, as well as a potential increase in workload. Moreover, without several experimental rooms available in which to isolate the different batches of mice, some stressors, such as cycle disturbances or predator sounds, cannot be used.

Coat state
In rodents, auto-grooming behavior is very sensitive to stress (Kalueff & Tuohimaa, 2004a, 2004b). In the UCMS model, the deterioration of mouse coat state can be related to a decrease in grooming and, in consequence, to a disturbance of self-directed behavior. The human correlate would be the poor personal hygiene displayed by many depressed individuals. The deterioration of the coat state are mainly observed on the head, the neck, and the back of the mice, as well as on the abdomen and the hindpaws. Thus, the maximum score rarely exceeds 4. However, as not all stressed mice cope with stress in the same way, a complete assessment of the coat state is still necessary to provide the most accurate measurement possible. It is important to note that the deterioration of the coat in the UCMS mice is dependent on their genetic background. Certain strains, such as BALB/c and DBA/2, are more sensitive to UCMS-induced effect on coat state (Ibarguen-Vargas, Surget, Touma, Palme, & Belzung, 2008). Assessing coat condition in mice with darker fur is more challenging than in albino animals. Moreover, the subjective nature of this measurement is a limitation, and it is recommended that two investigators, both of whom are blinded to treatment, make independent assessments of coat condition.
Body weight
Weight gain is another physical sign of the effects of chronic stress and pharmacological treatments. When using several animal cohorts that do not start the UCMS regimen at the same time/age, the body weight must be expressed relative to the initial weight of the mice before exposure to stress (baseline, week 0). Although a decline in the rate of body weight gain, along with a reversal of such an effect following chronic administration of an antidepressant, are generally observed in UCMS animals (Nollet et al., 2011; Surget et al., 2009), others have been unable to detect a difference in body weight gain between control and UCMS mice (Surget et al., 2008), even though other depression-like changes were observed. Because major depression is often associated with weight changes, the lack of a UCMS-induced effect on body weight reported by some is perplexing. It should be kept in mind, however, that human depression may be accompanied by either weight gain or weight loss, and that UCMS-induced body weight disruption appears to be dependent on the duration, timing, and type of stressors employed (Fig. 7B). Given these findings, however, changes in body weight should not be the primary endpoint in the UCMS paradigm.
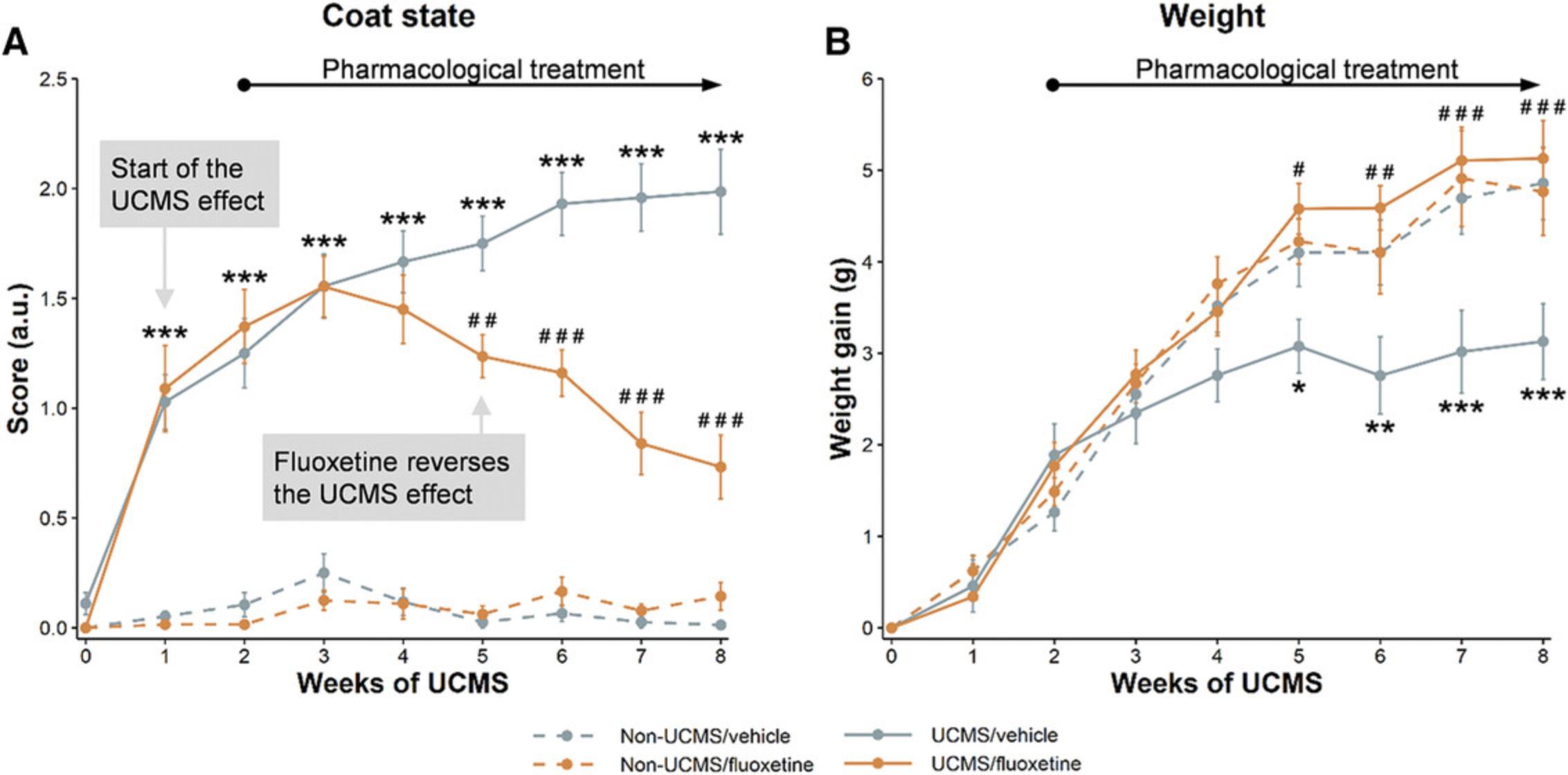
Test compound administration
In the UCMS model, compounds are usually administered at the beginning of the third week of stress exposure and maintained continuously for 4 to 7 weeks thereafter (i.e., during weeks 6 to 9 of the UCMS regimen). Acute administration of monoaminergic antidepressants is ineffective in this model of major depression, with compound-induced effects typically appearing within 2 to 4 weeks of treatment (Surget & Belzung, 2009). Intraperitoneally (i.p.; 10 to 20 mg/kg/day) or orally (p.o.; 20 to 30 mg/kg/day) administered fluoxetine or imipramine are the most widely used treatments for UCMS validation. Indeed, when the model is used to screen novel drugs for potential antidepressant activity, one of these drugs should be included as a reference agent.
When compounds are being evaluated in vivo, it is imperative to generate some preliminary pharmacokinetic data to ensure that the compound is present at the time of testing and in what amount. Increasingly, in vivo studies rely more on the measured plasma level of a compound, rather than the dose administered, to construct accurate dose-response curves. For peptides, a half-life of <1 min can be incompatible with a test procedure in which animals are assessed for a behavior or a performance phenotype 30 or 60 min after compound administration. Many compounds can, however, produce their effects via alterations in gene expression, which can be long-lasting, sometimes to the extent that their biological half-life is longer than the actual presence of the compound in the plasma. If a short-acting compound produces an effect beyond its plasma half-life, this can provide valuable information on its potential mechanism of action. Conversely, a behavioral effect of a compound that parallels its plasma half-life provides a direct cause-and-effect relationship that is proportional to the plasma concentration. If there is no pharmacokinetic information available for a given compound, this can seriously compromise the intent and outcome of the experiment.
The reward-driven exploratory test
Because anhedonia is a symptom of major depression, the reward-driven exploratory test (or cookie test) was designed to evaluate the motivation for a palatable stimulus. This is accomplished by assessing three behavioral dimensions: (1) anxiety-like state and exploration of the novel environment (latency to pass through the doors during the first session); (2) habituation to a novel environment (latency to pass through the doors over the sessions); and (3) anhedonia (latency to chew the food and the number of cookie chews versus the number of chews of the regular food pellet). By assessing the latter dimension over several sessions, it is possible to evaluate the interaction between anhedonic features and environmental habituation. This behavioral test has been validated in BALB/c mice exposed to UCMS and chronically treated with fluoxetine or the CRF1 receptor antagonist SSR125543 (Isingrini et al., 2010; Surget et al., 2011). Inasmuch as the sucrose preference test has not been successfully adapted to measure UCMS-induced anhedonia in mice (Ducottet & Belzung, 2005; Pothion, Bizot, Trovero, & Belzung, 2004), the reward-driven exploratory test appears to be a useful way to investigate the motivation for a reward. Furthermore, compared to other assays, the reward-driven exploratory test takes advantage of multiple measures and sessions to provide a more accurate analysis of UCMS-induced effects on behavior, and particularly on anhedonia. Although the data on the consumption of the regular pellets are not used as part of the statistical analysis in assessing UCMS-induced impairment of anhedonia, the regular food control is needed to establish the baseline consumption for all experimental groups and to avoid any interpretation biases. Because the use of separate control subgroups potentially decreases the statistical power of this assay, it may be possible to design the reward-driven exploratory test so that each animal is its own control. This could be accomplished by adding a control session with a regular food pellet instead of the cookie, or by placing both types of food in the black chamber, giving the animal a choice. It is noteworthy that once the data on the consumption of the regular pellets have been collected for the validation of the test in a particular experimental setup, it is not absolutely necessary to add these subgroups in the subsequent experiments. In addition, the test can also be performed under red light (Nollet et al., 2019).
Nest building test
A major advantage of the nest building test is its ease of execution and interpretation. Another advantage is that this test can, like the monitoring of coat state, be performed repeatedly (Nollet et al., 2019). This makes it possible to define more precisely the onset of any antidepressant effects. The second score, which is obtained 24 hr after the cotton squares are placed into the cage, appears to be the most relevant for evaluating nest quality, whereas the first score, which is taken 6 hr after placing the cotton squares into the cage, provides an estimate of nest building speed.
Grooming test
Unlike the coat state assay, the grooming test (or splash test) is a direct quantitative measure of the grooming behavior. The palatability and viscosity characteristics of the sucrose solution sprayed on the dorsal coat of mice cause the auto-grooming behavior. The grooming test is usually performed a single time at the end of the UCMS procedure and/or after the test compound or antidepressant administration. Repeated measures can also be performed since this test is not based on novelty (Nollet et al., 2019). Because all mice must be isolated for the test, control group-housed mice have to be singly housed in a novel home cage containing fresh sawdust 24 hr before testing (if this has not already been done before). The sawdust is changed at the same time in the cages of UCMS mice. This delay allows the animal to become familiar with a novel environment, minimizing novelty-induced behavioral changes.
Statistics
Considering the relatively small sample sizes, assumptions for parametric statistics (normality and homoscedasticity) may be invalid. Therefore, transformation using logarithms may be applied to normalize the data allowing the use of parametric tests. For repeated measures (coat state, body weight, and reward-driven exploratory test), three-way ANOVA or mixed-effects model (stress × treatment × time/session) could be used, followed by post hoc pairwise comparisons corrected for multiple testing, such as t -tests or Tukey tests (Schlenker, 2016). For non-repeated measures (nest building and grooming tests), two-way ANOVA or mixed model (stress × treatment) could be used, followed by similar post hoc tests. Alternatively, non-parametric solutions could be applied, such as three-way mixed ANOVA with aligned rank tests for repeated measures (Oliver-Rodríguez & Wang, 2015) and Kruskal-Wallis “ANOVA by ranks” H -test for the comparison of multiple independent samples. When appropriate, two independent samples can be compared using Mann-Whitney U -tests with correction for multiple testing (Shaffer, 1995).
Anticipated Results
Coat state deterioration occurs within the first 2 weeks of stress exposure. It generally begins on the neck and on the back, and then goes on to involve the head, abdomen, and hindpaws (Fig. 7A). As noted above, the deterioration is rarely observed on the other parts of the body. Any decrease in the rate of body weight gain usually takes 4 to 5 weeks of stress to become apparent (Fig. 7B). Because these measurements can be repeated, they can be used to determine the time of onset of the drug action. This typically occurs after 2 to 4 weeks of continuous, daily compound administration.
In the reward-driven exploratory test, considering the robust reduction of the latency to chew the cookie and the important increase in its consumption that are observed over the sessions for unstressed animals compared to controls on regular food, the results indicate that the drive for eating after a 1-hr food deprivation is stronger with the palatable cookie than with the regular food pellet (Isingrini et al., 2010; Surget et al., 2011). It has also been found that, during the last two sessions, the UCMS mice display (1) no significant effect on the latency to pass through the doors in all the sessions, (2) an increase in the latency to chew the cookie, and (3) a decrease in the number of chews of the cookie (Fig. 8). No UCMS-related differences were found when the regular food pellet was used, indicating that the stressors induced anhedonia. Furthermore, the UCMS-induced alterations were reversed during sessions 3 and 4 by chronic administration of fluoxetine (20 mg/kg/day, i.p.) and during the last session by daily administration of SSR125543 (20 mg/kg/day, i.p.). It is noteworthy that other parameters, such as the latency to pass through the gates or the latency to smell the food, were not affected by drug administration and did not yield relevant results.
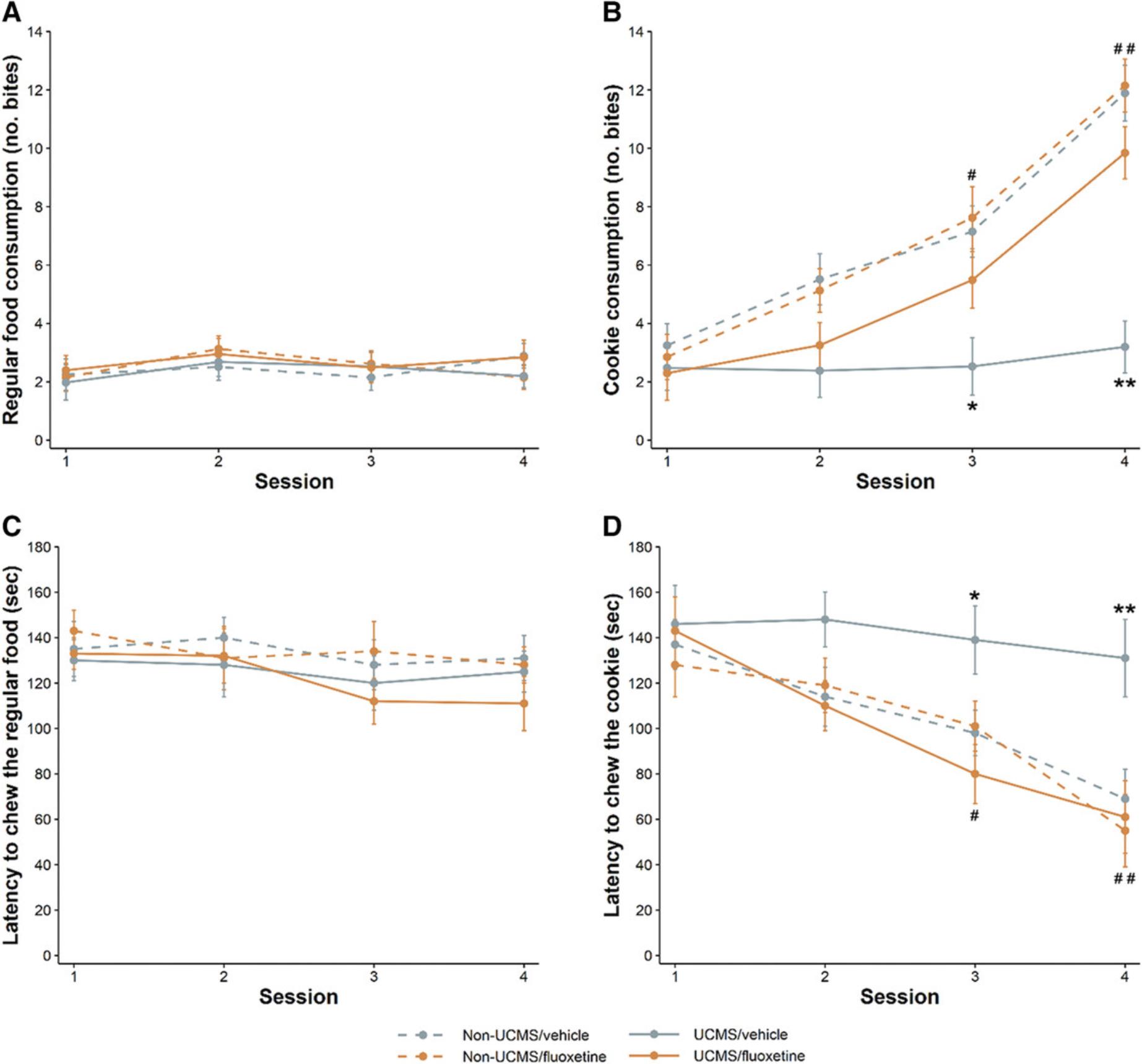
The UCMS mice typically display a decrease in nest quality (Nollet et al., 2019), a behavior that is counteracted by antidepressant treatment (Fig. 9A). In the grooming test, the latency to groom is increased (Fig. 9B) and its duration and frequency are reduced (Fig. 9C and D) in the UCMS mice. That is, the stressed animals most consistently exhibit a decrease in grooming behavior, which is restored by the chronic administration of an antidepressant.
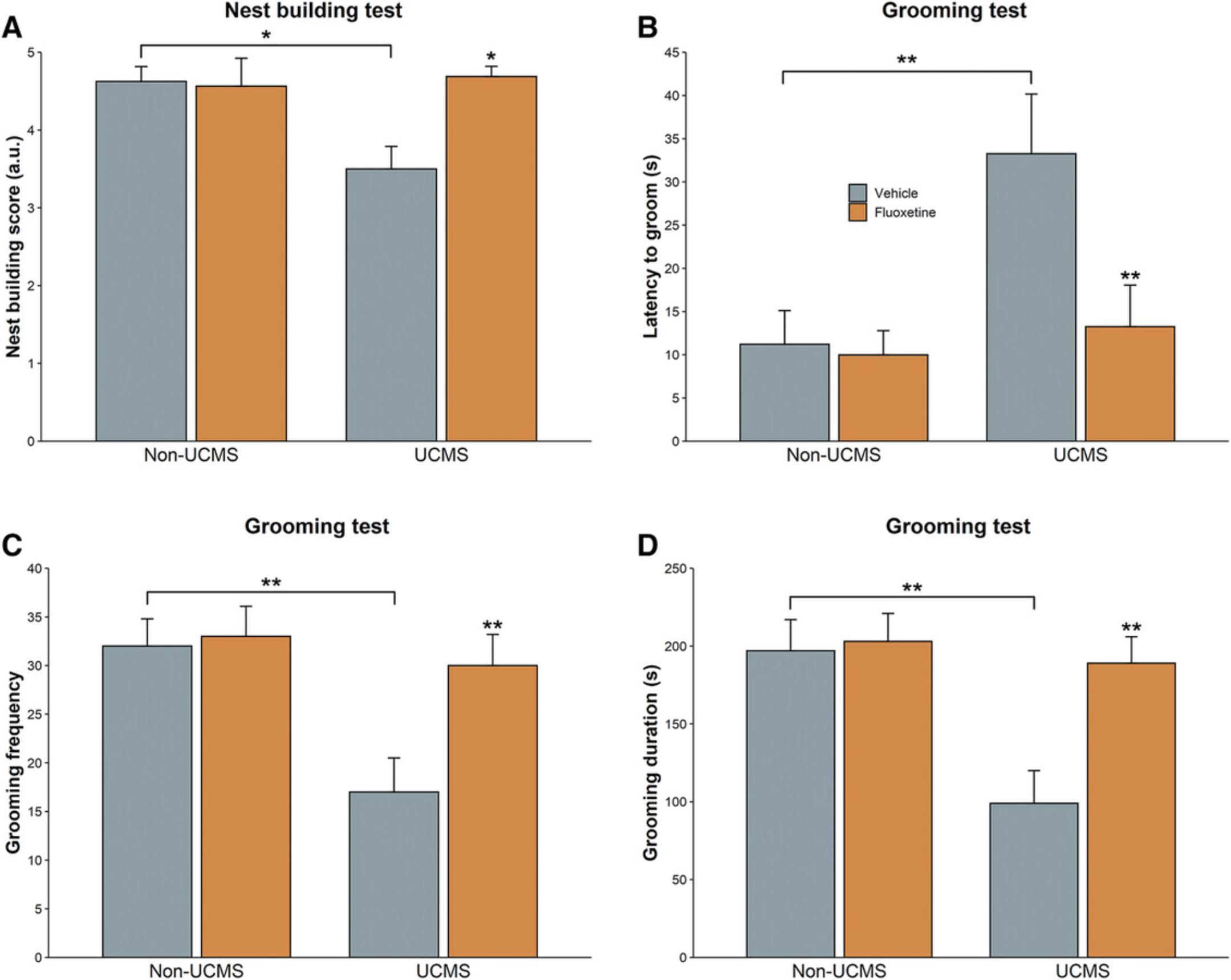
Other well-established assays, such as the novelty-suppressed feeding, forced swim, and tail suspension tests (Pollak et al., 2010) to assess behavioral despair or the open field, elevated plus maze, and light-dark tests (Ennaceur & Chazot, 2016) to assess anxiety, can also be performed at the end of the UCMS procedure to provide additional insight. The same applies to biochemical measures (e.g., levels of corticosterone or pro-inflammatory cytokines) and physiological measures.
Antidepressive compounds, such as ketamine, whose mechanisms of action are based on the blockade of glutamatergic receptor and show rapid onset effects in human, are also able to acutely reverse UCMS-induced changes in mice. Although no data are available yet regarding the effect of ketamine administration on UCMS-related alterations of coat state or on the results of the reward-driven exploratory test, nest building test, or grooming test, a single dose of this rapid-acting NMDA receptor is able to counteract the deleterious effects of the UCMS in the tail suspension test, the forced swim test, and the open field test (Fitzgerald et al., 2021; Ma et al., 2013). Furthermore, prophylactic treatment with ketamine can prevent the development of UCMS-induced depressive-like phenotype (Okine et al., 2020).
Results obtained from a UCMS regimen are dependent on many parameters, which may explain the variability observed between laboratories (and sometimes between protocols within a laboratory). Indeed, UCMS procedures often vary in terms of duration, stressors, unpredictability, species, strains, and treatments. Inter-strain variability (Ducottet & Belzung, 2005; Ibarguen-Vargas et al., 2008; Pothion et al., 2004) is a critical issue in optimizing the effectiveness of the UCMS paradigm. We use the BALB/c mouse strain because it displays high stress vulnerability. There can also be variability in the response to UCMS among mice in the same procedure, as some animals are more resilient and others are more vulnerable. However, when using inbred strains of mice, variability is typically low. Furthermore, despite the higher prevalence of major depression among women, a limited number of UCMS studies have been conducted in female mice, which have been found to display greater variability that is attributed to factors such as estrus cycle and stress susceptibility (Franceschelli, Herchick, Thelen, Papadopoulou-Daifoti, & Pitychoutis, 2014; Prevot et al., 2019). However, this variability could be explained by the lack of standardization between UCMS protocols (Mineur, Belzung, & Crusio, 2006). When using standardized models, and notwithstanding the sex-related differential sensitivity and coping mechanisms in regard to chronic stress, female mice seem to be more susceptible to UCMS than males (Guilloux, Seney, Edgar, & Sibille, 2011; Shepard, Page, & Coutellier, 2016; Stanley et al., 2014). Interestingly, the rapid-onset antidepressant ketamine seems to have differential effects in male and female mice subjected to UCMS (Fitzgerald et al., 2021; Okine et al., 2020). Overall, these concerns highlight the importance of standardizing the procedure in each laboratory to ensure consistent and reproducible results, making UCMS models all the more reliable and robust (Willner, 2016).
Time Considerations
The UCMS protocol is very time-consuming. A typical experiment in which mice are chronically administered a conventional antidepressant requires between 6 and 9 weeks to complete. This involves the time to adapt to the laboratory conditions (1 week) and the application of stressors, including the following phases: the drug-free period (2 weeks), the pharmacological treatment phase (3 to 4 weeks), and the potential behavioral testing period (1 week). As the UCMS must be continued until the animals are culled, extra time might be planned to, for example, perform intracardiac perfusion or microdissections if other assays are being conducted.
Author Contributions
Mathieu Nollet : Supervision, validation, writing–original draft, writing–review and editing.
Conflict of Interest
The author declares no conflict of interest.
Open Research
Data Availability Statement
Data sharing is not applicable to this article as no new data were created or analyzed in this study.
Literature Cited
- Alonso, R., Griebel, G., Pavone, G., Stemmelin, J., le, F. G., & Soubrie, P. (2004). Blockade of CRF(1) or V(1b) receptors reverses stress-induced suppression of neurogenesis in a mouse model of depression. Molecular Psychiatry , 9, 278–286, 224. doi: 10.1038/sj.mp.4001464.
- Assari, S., & Lankarani, M. M. (2016). Stressful life events and risk of depression 25 years later: Race and gender differences. Frontiers in Public Health , 4, 49. doi: 10.3389/fpubh.2016.00049.
- Belzung, C., & Lemoine, M. (2011). Criteria of validity for animal models of psychiatric disorders: Focus on anxiety disorders and depression. Biology of Mood & Anxiety Disorders, 1, 9.
- Caspi, A., Sugden, K., Moffitt, T. E., Taylor, A., Craig, I. W., Harrington, H., … Poulton, R. (2003). Influence of life stress on depression: Moderation by a polymorphism in the 5-HTT gene. Science , 301, 386–389. doi: 10.1126/science.1083968.
- Deacon, R. M. (2006). Assessing nest building in mice. Nature Protocols , 1, 1117–1119. doi: 10.1038/nprot.2006.170.
- Ducottet, C., & Belzung, C. (2005). Correlations between behaviours in the elevated plus-maze and sensitivity to unpredictable subchronic mild stress: Evidence from inbred strains of mice. Behavioural Brain Research , 156, 153–162. doi: 10.1016/j.bbr.2004.05.018.
- Ducottet, C., Griebel, G., & Belzung, C. (2003). Effects of the selective nonpeptide corticotropin-releasing factor receptor 1 antagonist antalarmin in the chronic mild stress model of depression in mice. Progress in Neuro-Psychopharmacology & Biological Psychiatry, 27, 625–631.
- Ennaceur, A., & Chazot, P. L. (2016). Preclinical animal anxiety research—flaws and prejudices. Pharmacology Research & Perspectives, 4, e00223.
- Fitzgerald, P. J., Kounelis-Wuillaume, S. K., Gheidi, A., Morrow, J. D., Spencer-Segal, J. L., & Watson, B. O. (2021). Sex- and stress-dependent effects of a single injection of ketamine on open field and forced swim behavior. Stress (Amsterdam, Netherlands). Advance online publication. doi: 10.1080/10253890.2021.1871600.
- Franceschelli, A., Herchick, S., Thelen, C., Papadopoulou-Daifoti, Z., & Pitychoutis, P. M. (2014). Sex differences in the chronic mild stress model of depression. Behavioural Pharmacology , 25, 372–383. doi: 10.1097/FBP.0000000000000062.
- Guilloux, J.-P., Seney, M., Edgar, N., & Sibille, E. (2011). Integrated behavioral z -scoring increases the sensitivity and reliability of behavioral phenotyping in mice: Relevance to emotionality and sex. Journal of Neuroscience Methods , 197, 21–31. doi: 10.1016/j.jneumeth.2011.01.019.
- Griebel, G., Simiand, J., Serradeil-Le, G. C., Wagnon, J., Pascal, M., Scatton, B., … Soubrie, P. (2002). Anxiolytic- and anti-depressant-like effects of the non-peptide vasopressin V1b receptor antagonist, SSR149415, suggest an innovative approach for the treatment of stress-related disorders. Proceedings of the National Academy of Sciences of the United States of America , 99, 6370–6375. doi: 10.1073/pnas.092012099.
- Griebel, G., Stemmelin, J., & Scatton, B. (2005). Effects of the cannabinoid CB1 receptor antagonist rimonabant in models of emotional reactivity in rodents. Biological Psychiatry , 57, 261–267. doi: 10.1016/j.biopsych.2004.10.032.
- Hervé, M., Bergon, A., Le Guisquet, A.-M., Leman, S., Consoloni, J.-L., Fernandez-Nunez, N., … Ibrahim, E. C. (2017). Translational identification of transcriptional signatures of major depression and antidepressant response. Frontiers in Molecular Neuroscience , 10, 248. doi: 10.3389/fnmol.2017.00248.
- Ibarguen-Vargas, Y., Surget, A., Touma, C., Palme, R., & Belzung, C. (2008). Multifaceted strain-specific effects in a mouse model of depression and of antidepressant reversal. Psychoneuroendocrinology , 33, 1357–1368. doi: 10.1016/j.psyneuen.2008.07.010.
- Isingrini, E., Camus, V., Le Guisquet, A. M., Pingaud, M., Devers, S., & Belzung, C. (2010). Association between repeated unpredictable chronic mild stress (UCMS) procedures with a high fat diet: A model of fluoxetine resistance in mice. Plos One , 5, e10404. doi: 10.1371/journal.pone.0010404.
- Kalueff, A. V., & Tuohimaa, P. (2004a). Contrasting grooming phenotypes in C57Bl/6 and 129S1/SvImJ mice. Brain Research , 1028, 75–82. doi: 10.1016/j.brainres.2004.09.001.
- Kalueff, A. V., & Tuohimaa, P. (2004b). Grooming analysis algorithm for neurobehavioural stress research. Brain Research. Brain Research Protocols , 13, 151–158. doi: 10.1016/j.brainresprot.2004.04.002.
- Keller, M. C., Neale, M. C., & Kendler, K. S. (2007). Association of different adverse life events with distinct patterns of depressive symptoms. American Journal of Psychiatry , 164, 1521–1529. doi: 10.1176/appi.ajp.2007.06091564.
- Ma, X.-C., Dang, Y.-H., Jia, M., Ma, R., Wang, F., Wu, J., … Hashimoto, K. (2013). Long-lasting antidepressant action of ketamine, but not glycogen synthase kinase-3 inhibitor SB216763, in the chronic mild stress model of mice. Plos One , 8, e56053. doi: 10.1371/journal.pone.0056053.
- Mineur, Y. S., Belzung, C., & Crusio, W. E. (2006). Effects of unpredictable chronic mild stress on anxiety and depression-like behavior in mice. Behavioural Brain Research , 175, 43–50. doi: 10.1016/j.bbr.2006.07.029.
- Musaelyan, K., Yildizoglu, S., Bozeman, J., Du Preez, A., Egeland, M., Zunszain, P. A., … Thuret, S. (2020). Chronic stress induces significant gene expression changes in the prefrontal cortex alongside alterations in adult hippocampal neurogenesis. Brain Communications , 2, fcaa153. doi: 10.1093/braincomms/fcaa153.
- Nelson, J., Klumparendt, A., Doebler, P., & Ehring, T. (2017). Childhood maltreatment and characteristics of adult depression: Meta-analysis. British Journal of Psychiatry , 210, 96–104. doi: 10.1192/bjp.bp.115.180752.
- Nollet, M., Gaillard, P., Minier, F., Tanti, A., Belzung, C., & Leman, S. (2011). Activation of orexin neurons in dorsomedial/perifornical hypothalamus and antidepressant reversal in a rodent model of depression. Neuropharmacology , 61, 336–346. doi: 10.1016/j.neuropharm.2011.04.022.
- Nollet, M., Gaillard, P., Tanti, A., Girault, V., Belzung, C., & Leman, S. (2012). Neurogenesis-independent antidepressant-like effects on behavior and stress axis response of a dual orexin receptor antagonist in a rodent model of depression. Neuropsychopharmacology , 3, 2210–2221. doi: 10.1038/npp.2012.70.
- Nollet, M., Hicks, H., McCarthy, A. P., Wu, H., Möller-Levet, C. S., Laing, E. E., … Winsky-Sommerer, R. (2019). REM sleep's unique associations with corticosterone regulation, apoptotic pathways, and behavior in chronic stress in mice. Proceedings of the National Academy of Sciences of the United States of America , 116, 2733–2742. doi: 10.1073/pnas.1816456116.
- Okine, T., Shepard, R., Lemanski, E., & Coutellier, L. (2020). Sex differences in the sustained effects of ketamine on resilience to chronic stress. Frontiers in Behavioral Neuroscience , 14, 581360. doi: 10.3389/fnbeh.2020.581360.
- Oliver-Rodríguez, J. C., & Wang, X. T. (2015). Non-parametric three-way mixed ANOVA with aligned rank tests. British Journal of Mathematical and Statistical Psychology , 68, 23–42. doi: 10.1111/bmsp.12031.
- Pałucha-Poniewiera, A., Podkowa, K., & Rafało-Ulińska, A. (2021). The group II mGlu receptor antagonist, LY341495 induces a rapid antidepressant-like effect and enhances the effect of ketamine in the chronic unpredictable mild stress model of depression in C57BL/6J mice. Progress in Neuro-Psychopharmacology & Biological Psychiatry, 109, 110239.
- Pan, K.-Y., Kok, A. A. L., Eikelenboom, M., Horsfall, M., Jörg, F., Luteijn, R. A., … Penninx, B. W. J.H. (2021). The mental health impact of the COVID-19 pandemic on people with and without depressive, anxiety, or obsessive-compulsive disorders: A longitudinal study of three Dutch case-control cohorts. Lancet Psychiatry , 8, 121–129. doi: 10.1016/S2215-0366(20)30491-0.
- Pollak, D. D., Rey, C. E., & Monje, F. J. (2010). Rodent models in depression research: Classical strategies and new directions. Annals of Medicine , 42, 252–264. doi: 10.3109/07853891003769957.
- Pothion, S., Bizot, J. C., Trovero, F., & Belzung, C. (2004). Strain differences in sucrose preference and in the consequences of unpredictable chronic mild stress. Behavioural Brain Research , 155, 135–146. doi: 10.1016/j.bbr.2004.04.008.
- Prevot, T. D., Misquitta, K. A., Fee, C., Newton, D. F., Chatterjee, D., Nikolova, Y. S., … Banasr, M. (2019). Residual avoidance: A new, consistent and repeatable readout of chronic stress-induced conflict anxiety reversible by antidepressant treatment. Neuropharmacology , 153, 98–110. doi: 10.1016/j.neuropharm.2019.05.005.
- Ruff, J. S., Suchy, A. K., Hugentobler, S. A., Sosa, M. M., Schwartz, B. L., Morrison, L. C., … Potts, W. K. (2013). Human-relevant levels of added sugar consumption increase female mortality and lower male fitness in mice. Nature Communications , 4, 2245. doi: 10.1038/ncomms3245.
- Schlenker, E. (2016). Tips and tricks for successful application of statistical methods to biological data. Methods in Molecular Biology , 1366, 271–285. doi: 10.1007/978-1-4939-3127-9_21.
- Shaffer, J. P. (1995). Multiple hypothesis testing. Annual Review of Psychology , 46, 561–584. doi: 10.1146/annurev.ps.46.020195.003021.
- Shepard, R., Page, C. E., & Coutellier, L. (2016). Sensitivity of the prefrontal GABAergic system to chronic stress in male and female mice: Relevance for sex differences in stress-related disorders. Neuroscience , 332, 1–12. doi: 10.1016/j.neuroscience.2016.06.038.
- Sibille, E., Wang, Y., Joeyen-Waldorf, J., Gaiteri, C., Surget, A., Oh, S., … Lewis, D. A. (2009). A molecular signature of depression in the amygdala. American Journal of Psychiatry , 166, 1011–1024. doi: 10.1176/appi.ajp.2009.08121760.
- Stanley, S. C., Brooks, S. D., Butcher, J. T., d'Audiffret, A. C., Frisbee, S. J., & Frisbee, J. C. (2014). Protective effect of sex on chronic stress- and depressive behavior-induced vascular dysfunction in BALB/cJ mice. Journal of Applied Physiology , 117, 959–970. doi: 10.1152/japplphysiol.00537.2014.
- Stemmelin, J., Cohen, C., Terranova, J. P., Lopez-Grancha, M., Pichat, P., Bergis, O., … Griebel, G. (2008). Stimulation of the beta3-adrenoceptor as a novel treatment strategy for anxiety and depressive disorders. Neuropsychopharmacology , 33, 574–587. doi: 10.1038/sj.npp.1301424.
- Surget, A., & Belzung, C. (2009). Unpredictable chronic mild stress in mice. In A. V. Kalueff & J. L. LaPorte (Eds.), Experimental animal models in neurobehavioral research (pp. 79–112). Nova Science Publishers.
- Surget, A., Saxe, M., Leman, S., Ibarguen-Vargas, Y., Chalon, S., Griebel, G., … Belzung, C. (2008). Drug-dependent requirement of hippocampal neurogenesis in a model of depression and of antidepressant reversal. Biological Psychiatry , 64, 293–301. doi: 10.1016/j.biopsych.2008.02.022.
- Surget, A., Wang, Y., Leman, S., Ibarguen-Vargas, Y., Edgar, N., Griebel, G., … Sibille, E. (2009). Corticolimbic transcriptome changes are state-dependent and region-specific in a rodent model of depression and of antidepressant reversal. Neuropsychopharmacology , 34, 1363–1380. doi: 10.1038/npp.2008.76.
- Surget, A., Tanti, A., Leonardo, E. D., Laugeray, A., Rainer, Q., Touma, C., … Belzung, C. (2011). Antidepressants recruit new neurons to improve stress response regulation. Molecular Psychiatry , 16, 1177–1188. doi: 10.1038/mp.2011.48.
- Willner, P. (2016). Reliability of the chronic mild stress model of depression: A user survey. Neurobiology of Stress , 6, 68–77. doi: 10.1016/j.ynstr.2016.08.001.
- Willner, P. (2017). The chronic mild stress (CMS) model of depression: History, evaluation and usage. Neurobiology of Stress , 6, 78–93. doi: 10.1016/j.ynstr.2016.08.002.
- Yalcin, I., Aksu, F., & Belzung, C. (2005). Effects of desipramine and tramadol in a chronic mild stress model in mice are altered by yohimbine but not by pindolol. European Journal of Pharmacology , 514, 165–174. doi: 10.1016/j.ejphar.2005.03.029.
- Yalcin, I., Belzung, C., & Surget, A. (2008). Mouse strain differences in the unpredictable chronic mild stress: A four-antidepressant survey. Behavioural Brain Research , 193, 140–143. doi: 10.1016/j.bbr.2008.04.021.
- Zimmermann, P., Brückl, T., Nocon, A., Pfister, H., Binder, E. B., Uhr, M., … Ising, M. (2011). Interaction of FKBP5 gene variants and adverse life events in predicting depression onset: Results from a 10-year prospective community study. American Journal of Psychiatry , 168, 1107–1116. doi: 10.1176/appi.ajp.2011.10111577.
Citing Literature
Number of times cited according to CrossRef: 11
- María Salud García-Gutiérrez, Daniela Navarro, Amaya Austrich-Olivares, Jorge Manzanares, Unveiling behavioral and molecular neuroadaptations related to the antidepressant action of cannabidiol in the unpredictable chronic mild stress model, Frontiers in Pharmacology, 10.3389/fphar.2023.1171646, 14 , (2023).
- Canye Li, Zuanjun Su, Zhicong Chen, Jinming Cao, Xiufeng Liu, Feng Xu, Lactobacillus reuteri strain 8008 attenuated the aggravation of depressive-like behavior induced by CUMS in high-fat diet-fed mice through regulating the gut microbiota, Frontiers in Pharmacology, 10.3389/fphar.2023.1149185, 14 , (2023).
- Hwei-Ee Tan, The microbiota-gut-brain axis in stress and depression, Frontiers in Neuroscience, 10.3389/fnins.2023.1151478, 17 , (2023).
- Astrocytes as Context for the Involvement of Myelin and Nodes of Ranvier in the Pathophysiology of Depression and Stress-Related Disorders, Journal of Psychiatry and Brain Science, 10.20900/jpbs.20230001, (2023).
- Jianping Wang, Yujiao Men, Zeyu Wang, Polydatin Alleviates Chronic Stress-Induced Depressive and Anxiety-like Behaviors in a Mouse Model, ACS Chemical Neuroscience, 10.1021/acschemneuro.2c00758, 14 , 5, (977-987), (2023).
- Anna Rafało-Ulińska, Bartłomiej Pochwat, Paulina Misztak, Ryszard Bugno, Agata Kryczyk-Poprawa, Włodzimierz Opoka, Bożena Muszyńska, Ewa Poleszak, Gabriel Nowak, Bernadeta Szewczyk, Zinc Deficiency Blunts the Effectiveness of Antidepressants in the Olfactory Bulbectomy Model of Depression in Rats, Nutrients, 10.3390/nu14132746, 14 , 13, (2746), (2022).
- Charinya Khamphukdee, Ibrahim Turkmani, Yutthana Chotritthirong, Yaowared Chulikhit, Chantana Boonyarat, Nazim Sekeroglu, Artur M. S. Silva, Orawan Monthakantirat, Anake Kijjoa, Effects of the Bark Resin Extract of Garcinia nigrolineata on Chronic Stress-Induced Memory Deficit in Mice Model and the In Vitro Monoamine Oxidases and β-Amyloid Aggregation Inhibitory Activities of Its Prenylated Xanthone Constituents, Molecules, 10.3390/molecules27093014, 27 , 9, (3014), (2022).
- Dan Liu, Haohao Hu, Yuchuan Hong, Qian Xiao, Jie Tu, Sugar Beverage Habitation Relieves Chronic Stress-Induced Anxiety-like Behavior but Elicits Compulsive Eating Phenotype via vLSGAD2 Neurons, International Journal of Molecular Sciences, 10.3390/ijms24010661, 24 , 1, (661), (2022).
- Dmitrii D. Markov, Sucrose Preference Test as a Measure of Anhedonic Behavior in a Chronic Unpredictable Mild Stress Model of Depression: Outstanding Issues, Brain Sciences, 10.3390/brainsci12101287, 12 , 10, (1287), (2022).
- Junni Yan, Fuping Zhang, Le Niu, Xiaonan Wang, Xinxin Lu, Chaoyue Ma, Chencheng Zhang, Jinggui Song, Zhaohui Zhang, High-frequency repetitive transcranial magnetic stimulation mitigates depression-like behaviors in CUMS-induced rats via FGF2/FGFR1/p-ERK signaling pathway, Brain Research Bulletin, 10.1016/j.brainresbull.2022.02.020, 183 , (94-103), (2022).
- Jorge Mendoza, Noëmi Billon, Guillaume Vanotti, Viviane Pallage, Chronobiology of Mood States: Introducing Circadian Animal Models, Psychiatric Vulnerability, Mood, and Anxiety Disorders, 10.1007/978-1-0716-2748-8_10, (185-199), (2022).