Mammalian Chromosome Analysis and Sorting by Flow Cytometry
Risani Mukhopadhyay, Risani Mukhopadhyay, DV Varshitha, DV Varshitha, William G. Telford, William G. Telford, Claire K. Sanders, Claire K. Sanders, Uttara Chakraborty, Uttara Chakraborty
Abstract
The analysis of chromosomes by flow cytometry is termed flow cytogenetics, and it involves the analysis and sorting of single mitotic chromosomes in suspension. The study of flow karyograms provides insight into chromosome number and structure to provide information on chromosomal DNA content and can enable the detection of deletions, translocations, or any forms of aneuploidy. Beyond its clinical applications, flow cytogenetics greatly contributed to the Human Genome Project through the ability to sort pure populations of chromosomes for gene mapping, cloning, and the construction of DNA libraries. Maximizing the potential of these important applications of flow cytogenetics relies on precise instrument setup and optimal sample processing, both of which impact the accuracy and quality of the data that are generated. This article is a compilation of the existing protocols that describe the stepwise methodology of accumulating, isolating, and staining metaphase chromosomes to prepare single-chromosome suspensions for flow cytometric analysis and sorting. Although the chromosome preparation protocols have remained largely unchanged, cytometer technology has advanced dramatically since these protocols were originally developed. Advances in cytometry technologies offer new and exciting approaches for understanding and monitoring chromosomal aberrations, but the hallmark of these protocols remains their simplicity in methodologies and reagent requirements and the accuracy of data resolvable to every chromosome of the cell. © 2023 The Authors. Current Protocols published by Wiley Periodicals LLC.
This article was corrected on 18 July 2023. See the end of the full text for details.
Basic Protocol 1 : Mitotic block and cell harvesting
Basic Protocol 2 : Propidium iodide isolation
Support Protocol 1 : Swelling test
Basic Protocol 3 : MgSO4 low-molecular-weight isolation
Basic Protocol 4 : Polyamine high-molecular-weight isolation
Support Protocol 2 : Molecular-weight determination of chromosomal DNA
Basic Protocol 5 : Chromosome analysis and sorting
INTRODUCTION
Cytogenetics is a field that involves the study of the morphology, functioning, and number of chromosomes under both normal and pathological conditions. The classical approaches of cytogenetics can be complemented by other technologies, such as flow cytometry, allowing for precise qualitative and quantitative analysis of chromosomes on a large scale (Callis & Hoehn, 1976). The application of flow cytometric analysis and sorting of single chromosomes for classification and purification is called flow cytogenetics. It requires the isolation of metaphase chromosomes from mitotic cells, staining with DNA dyes or fluorochrome tags in suspension, and rapid analysis in a flow cytometer (Langlois et al., 1982). The analysis of chromosomes is based on the selective inclusion of optical parameters, particularly of the signals from fluorescently labeled DNA. When plotted, the position of peaks generated on the flow karyogram is proportional to chromosomal DNA content. Additionally, dyes that preferentially bind A:T to G:C base pairs allow for further resolution of the distribution based on relative DNA content. These techniques allow for the analysis or sorting of specific chromosomes extracted from a cell population. However, due to similarity in size and relative DNA content, the peaks of some chromosomes (in humans, chromosomes 9 to 12) may overlap and cannot always be resolved (Carrano et al., 1979; Cram et al., 2002; Langlois et al., 1982). However, with advances in flow cytometry, sorting and imaging can be applied to isolate and visualize single chromosomes, creating a more specific and precise profile that allows us to distinguish between each chromosome.
Flow karyograms can be displayed in multiple ways to discriminate chromosomes. Depending on the number of parameters used, either univariate histograms for single-color analysis or bivariate density or contour plots for two-color analysis can be used to display chromosomes. A variety of DNA dyes can be used for univariate or single-color analysis. Propidium iodide (PI) has been the most commonly used as it is a specific and stoichiometric stain. In mammalian cell types, univariate flow karyotypes are commonly used in species with smaller numbers of chromosomes, for example, in the Chinese hamster, which has 10 pairs of autosomal chromosomes plus the sex chromosomes. In a histogram, the peak area is directly proportional to the number of a particular chromosome encountered, whereas the fluorescence intensity displayed by the peak position is proportional to the DNA content (Bartholdi et al., 1987; van den Engh et al., 1984). Whereas the bivariate flow karyotype takes advantage of differential staining of A:T and G:C regions labeled by the DNA dyes Hoechst 33258 and Chromomycin A3, respectively, this allows for the resolution of a greater number of chromosomes. Here, fluorescence intensity is specific to the number of A:T and G:C base pairs on a chromosome, so the position is relative to the ratio and the total size of the chromosome (van den Engh et al., 1985). Similar to the histogram, the peak areas signify the relative ratios of the chromosomes. Using both approaches, a quantitative measure of genomic stability can be obtained. New peaks are indicative of structural aberrations in chromosomes, and changes in chromosome ratio can indicate other abnormalities, such as trisomy (Dean et al., 1989; Matsson et al., 1986).
The analysis of chromosomes by flow cytometry can be followed by the sorting of individual chromosomes for downstream applications. Examples of these applications include the construction of chromosome-specific gene libraries for the Human Genome Project (Van Dilla et al., 1986) and the determination of chromosomal abnormalities like aneuploidy or structural aberrations associated with genetic diseases, which was further strengthened by the application of fabricated chromosome-specific fluorescent in situ hybridization probes (Cram et al., 2004).
In this article, we include four cytogenetics protocols published by S. Cram, C. S. Bell, and J. J. Fawcett (Cram et al., 2002) and provide updated application and instrument information for mammalian chromosome analysis and sorting. We also describe recent advances in cytometer technology (particularly lasers) and how these can be applied to chromosome analysis.
Basic Protocol 1 describes a procedure for arresting cells in metaphase and harvesting them. Basic Protocols 2 to 4 outline the different procedures to isolate these metaphase chromosomes and to prepare chromosome suspensions for analysis and sorting of single chromosomes. The protocol selection depends on the kind of analysis and downstream application of the sorted chromosomes. Additionally, the two support protocols provide confirmatory steps to ensure the efficiency of the experimental conditions and collection of the final results. Support Protocol 1 helps standardize appropriate hypertonic conditions for optimum cell swelling in Basic Protocol 2. Support Protocol 2 is used to verify the molecular weight of the chromosomes isolated using Basic Protocol 4. Lastly, improvements in instrument technology have also dramatically changed the equipment on which chromosomes are analyzed and sorted, these changes are discussed in detail in Basic Protocol 5.
Basic Protocol 1: MITOTIC BLOCK AND CELL HARVESTING
The first step in the isolation of single chromosomes is the induction of metaphase arrest. This is usually accomplished by a chemical block with Colcemid or colchicine. During mitosis, these chemicals block the formation of spindles and allow the condensed chromosomes to freely float within the cell. The following protocol outlines the arresting of cells in metaphase and the collection of these cells from monolayer cultures of primary cells/cell lines.
Materials
- Cells growing exponentially in sub-confluent culture in flasks
- 10 µg/ml Colcemid solution [in phosphate-buffered saline (PBS); Thermo Fisher, cat. no. 15212012]
- 50-ml centrifuge tubes
- Standard tabletop centrifuge
- Additional reagents and equipment for cell culture under normal growth conditions
1.Ensure cells growing exponentially in sub-confluent culture in flasks are truly sub-confluent and contain an exponentially growing monolayer of cells. Minimize the number of floating (non-viable) cells.
2.Add 10 µg/ml Colcemid solution to the flasks to a final concentration between 0.01 µg/ml and 0.1 µg/ml.
3.Incubate cells with Colcemid under normal growth conditions for 3 to 15 hr.
4.Shake off mitotic cells 2 to 3 times.
5.Recover mitotic cells by pouring off medium into a 50-ml centrifuge tube on ice.
6.Centrifuge for a normal duration at a normal speed for the cell type.
7.Completely discard the supernatant.
8.Proceed with the chosen method of chromosome isolation (see Basic Protocols 2 to 4).
Basic Protocol 2: PROPIDIUM IODIDE ISOLATION
This single-color method yields chromosomes that maintain the typically illustrated X shape. Use of the intercalating dye PI stabilizes the chromosome structure, so additional chemicals do not need to be used. Because this is a single-color (univariate) method, mammalian species with large numbers of chromosomes will have overlapping peaks that may not be fully resolvable. This protocol is therefore recommended for use in species with small numbers of chromosomes and/or chromosomes with highly variable DNA content or where not all chromosomes need to be resolved. For example, because cloned Chinese hamster cells have 11 pairs of chromosomes (10 pairs of autosomes and one pair of sex chromosomes), their karyotype can be resolved using the single-color method (as observed in Fig. 1).
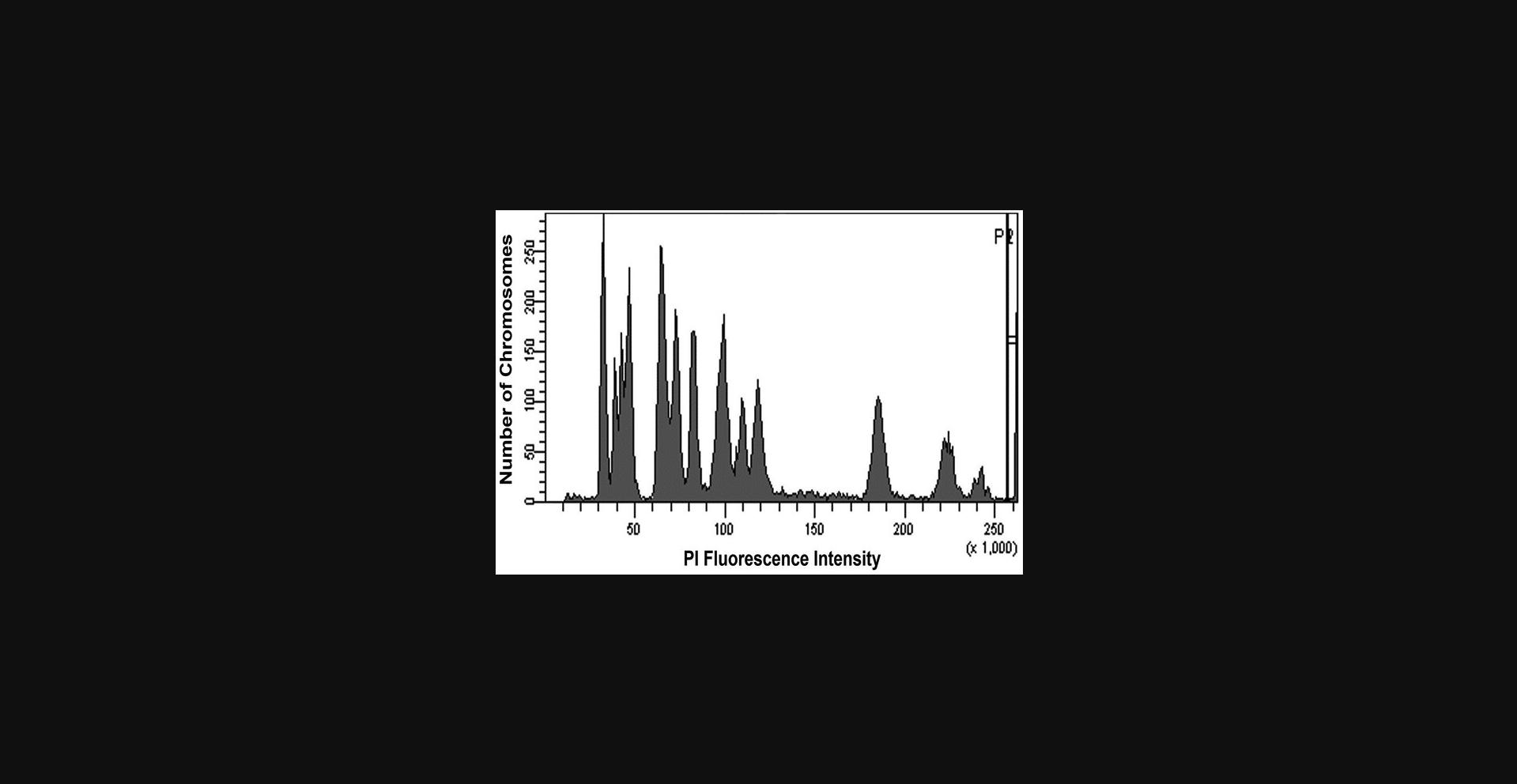
Materials
-
Cell pellets from Basic Protocol 1
-
PI solutions A and B (see recipes)
-
Glass microscope slides
-
Wax pencil or crayon
-
Coverslips
-
Fluorescence microscope
-
3-ml syringe with 22-gauge needle (1 1/2’’)
-
37°C water bath
-
35- to 60-µm mesh
Cell swelling
1.To each tube of cell pellets from Basic Protocol 1, add 0.5 ml PI solution A and resuspend the pellet.
2.Allow cells to swell for 10 min at room temperature in the dark.
3.Place 10 µl cell suspension on a glass microscope slide marked with a wax pencil or crayon and cover it with a coverslip. Observe under a fluorescence microscope.
Isolation of chromosomes
4.Add 0.25 ml PI solution B to the current suspension of 0.5 ml.
5.Incubate at room temperature in the dark for 3 min.
6.Rapidly draw up and expel the suspension 4 to 5 times using a 3-ml syringe with a 22-gauge needle, with the point of the needle against the side of the tube. Monitor under a microscope to ensure chromosomes have been released from the cell membranes.
7.Incubate the chromosome suspension at 37°C in a water bath for 30 min.
8.Filter the suspension through 35- to 60-µm mesh.
9.Store the isolated chromosomes for ≤3 weeks at 4°C until further analysis (see Basic Protocol 5).
Support Protocol 1: SWELLING TEST
It is essential to conduct the swelling test during the standardization of Basic Protocol 2 for your sample to ensure good sample preparation. This is done to establish appropriate hypotonic solution [for example, potassium chloride (KCl)] concentrations for the selected cell type. If the concentration is not optimal, the cells may swell too quickly and rupture before the chromosomes have time to increase their dispersion within the cell, or they may not swell enough, leading to incomplete lysis and cell clumping.
Additional Materials (also see Basic Protocol 2)
- Hoechst 33342 (Thermo Fisher, cat. no. 62249)
- Dulbecco's PBS (DPBS; Sigma-Aldrich, cat. no. D8537-1L)
- PI solution A containing cells to be processed (see Basic Protocol 2, step 1)
1.Make 0.5 mg/ml Hoechst 33342 stock solution in DPBS and add 12.3 μl to 1 ml of the PI solution A containing cells to be processed (final concentration 10 μM).
2.Using a wax pencil or crayon, draw parallel lines on a glass microscope slide under where the coverslip will be placed.
3.Gently add a drop or two of the cells in the solution onto the slide and carefully place a coverslip.
4.Observe under a fluorescence microscope using ultraviolet excitation.
Basic Protocol 3: MgSO4 LOW-MOLECULAR-WEIGHT ISOLATION
The magnesium sulfate (MgSO4) low-molecular-weight chromosome isolation procedure was developed using Chinese hamster ovary (CHO) cells based on the ability of MgSO4 to stabilize the chromosome (Gray et al., 1975). This procedure has also proven to be suitable for human cells (van den Engh et al., 1984).
The isolation buffer consists of KCl at a hypotonic concentration, MgSO4 as a stabilizer, HEPES buffer to maintain pH, and dithiothreitol as a reducing agent. The buffer also contains RNase, which allows for better DNA-specific staining of the isolated chromosomal preparations (Bartholdi et al., 1983). This relatively high magnesium concentration in the isolation buffer stabilizes the DNA of the isolated chromosomes, thereby allowing the Chromomycin A3 (CA3) dye to bind (Gray & Cram, 1990). The presence of MgSO4 at an optimum pH of 8.0 along with dithiothreitol reduces the number of chromosomal clumps.
The chromosomes isolated using this protocol are of low molecular weight and are appropriate for bivariate flow karyotyping. This method allows the use of DNA-binding dyes that are dependent on base composition, such as Hoechst 33258 and Chromomycin A3.
The protocol is advantageous when a small number of mitotic cells are available (Gray & Cram, 1990). However, it has been reported that high magnesium concentrations can cause chromosome contraction, resulting in decreased resolution of flow cytometric measurements. This can be overcome by the addition of sodium citrate and sodium sulfite 15 min before the analysis (Trask & van den Engh, 1990).
Materials
-
IB01 (LMW) isolation buffer (see recipe; make fresh)
-
2.5% (w/v) Triton X-100 (make fresh)
-
DNA stain: PI (Thermo Fisher, cat. no. P1304MP) for univariate analysis or Hoechst 33258 (Thermo Fisher, cat. no. H1398) and Chromomycin A3 (CA3; Sigma-Aldrich, cat. no. C2659) for bivariate analysis
-
Ethanol (to make Chromomycin A3 stock solution)
-
50-ml centrifuge tubes
-
Refrigerated centrifuge
-
22-gauge needle on 3- to 5-ml syringe
-
37°C water bath
-
Additional reagents and equipment for performing Basic Protocol 1, steps 1 to 4
Prepare cell suspension
1.Culture, incubate, and shake off mitotic cells as in Basic Protocol 1, steps 1 to 4.
2.Transfer 3 ml suspension containing mitotic cells into each 50-ml centrifuge tube.
3.Spin down the tubes for 8 min at 400 × g , 4°C.
4.Discard the supernatant and flick the bottom of each tube several times to dislodge the pellet in the remaining volume.
Cell swelling
5.Add 1 ml freshly prepared IB01 (LMW) isolation buffer and flick several times to resuspend.
6.Incubate the suspension for 10 min at room temperature.
Isolation of chromosomes
7.Add 0.1 ml of 2.5% Triton X-100 for every 1 ml of sample suspension.
8.Let stand for 10 min at room temperature.
9.Slowly force the suspension three times through a 22-gauge needle on a 3- to 5-ml syringe to disperse the metaphase chromosomes and avoid clumping.
10.Pool tubes if necessary.
11.Incubate the samples for 30 min at 37°C in a water bath.
Staining
12.Store chromosomes unstained for ≤3 weeks at 4°C or stain chromosomes with DNA stain, using PI for univariate analysis or Hoechst 33258 and Chromomycin A3 for bivariate analysis:
-
Make 1 mg/ml PI stock solution in distilled water and add 20 µl to 1.0 ml chromosomes (final concentration 20 µg/ml). Stain for ≥3 hr at room temperature before analysis to establish equilibrium.
-
Make 0.5 mg/ml Hoechst 33258 stock solution in distilled water and add 4 µl to 1.0 ml chromosomes (final concentration 2 µg/ml). Make 0.5 mg/ml Chromomycin A3 solution in ethanol and add 40 µl to 1.0 ml chromosomes (final concentration 20 µg/ml). Stain for ≥3 hr at room temperature before analysis to establish equilibrium.
Basic Protocol 4: POLYAMINE HIGH-MOLECULAR-WEIGHT ISOLATION
High-molecular-weight DNA can be isolated using a basic buffer with high pH, ranging from 9.6 to 10.5 (Blumenthal et al., 1979; Wray, 1973). However, high pH affects the recovery of acidic proteins and can result in various unwanted effects on the quality of chromosomal DNA (Trask & van den Engh, 1990). Blumenthal et al. (1979) developed the method described here to preserve the native structure of chromosomes during isolation.
This protocol utilizes a neutral buffer containing polyamines (Wallace et al., 1971). Use of polyamines such as spermidine and spermine replaces the need for divalent cations or heavy-metal chelators to stabilize the chromosomal structure and prevent nuclease activity (Wray et al., 1972).
The ability to recover high-molecular-weight DNA from chromosomes has played a huge role in developing the field of flow cytogenetics and its application in cloning (Gray et al., 1987) as well as the construction of chromosome-specific gene libraries (Van Dilla & Deaven, 1990). This method has been preferentially used for this purpose and is described here for bivariate (Hoechst 33258 and Chromomycin A3) analysis and sorting.
Materials
-
Ohnuki's hypotonic swelling buffer (see recipe)
-
IB02 (HMW) isolation buffer (see recipe; make fresh), 4°C
-
1 mg/ml PI stock solution (Thermo Fisher, cat. no. P1304MP)
-
Hoechst 33258 (Thermo Fisher, cat. no. H1398)
-
Chromomycin A3 (CA3; Sigma-Aldrich, cat. no. C2659)
-
McIlvaine's buffer, pH 7 (see recipe)
-
5 mM MgCl2
-
15-ml polypropylene centrifuge tubes
-
Vortex
-
Fluorescence microscope
-
Additional reagents and equipment for performing Basic Protocol 1
Prepare cell suspension
1.Follow Basic Protocol 1 with 106 to 107 mitotic cells per tube.
Cell swelling
2.Add 5 ml room-temperature Ohnuki's hypotonic swelling buffer per tube.
3.Transfer the cells into 15-ml polypropylene centrifuge tubes and incubate at room temperature for 70 min.
4.Centrifuge 5 min at 100 × g at room temperature.
5.Carefully aspirate the supernatant and flick the tube to dislodge the pellet in the residual volume.
Isolation of chromosomes
6.Add 1.0 ml freshly prepared cold IB02 (HMW) isolation buffer to each tube and mix gently.
7.Vortex each tube vigorously for 30 s.
8.Place the samples on ice for 10 min.
9.Combine 10 µl chromosome suspension and 0.2 µl of 1 mg/ml PI stock solution (final concentration 20 µg/ml) and observe chromosomes under a fluorescence microscope to determine the degree of dispersion and clumping.
10.If needed, repeat vortexing of the main suspension for 10 s and maintain the sample on ice while rechecking dispersion. Repeat the vortexing cycle as needed.
Staining
11.Store chromosomes unstained for ≤1 year at 4°C or stain chromosomes with Hoechst 33258 and Chromomycin A3 for ≥3 hr before analysis to establish equilibrium:
-
Make 0.5 mg/ml Hoechst stock solution in distilled water and add 4.5 µl to 1.0 ml chromosomes (final concentration 2.25 µg/ml).
-
Make 308 µg/ml Chromomycin A3 stock solution in McIlvaine's buffer, pH 7, and dilute 1:1 with 5 mM MgCl2. Add 150 µl to 1.0 ml chromosomes (final concentration 20 µg/ml).
Support Protocol 2: MOLECULAR-WEIGHT DETERMINATION OF CHROMOSOMAL DNA
To ensure that all samples have high molecular weight before sorting, each sample is checked as a precaution before long-term sorting experiments.
Materials
-
Chromosomal preparation from Basic Protocol 3 (after step 11) or Basic Protocol 4 (after step 10)
-
Nuclear lysis buffer (see recipe)
-
20% (w/v) sodium dodecyl sulfate (SDS; make fresh)
-
1.5-ml microcentrifuge tubes
1.Place 75 µl of the chromosomal preparation from Basic Protocol 3 (after step 11) or Basic Protocol 4 (after step 10) into a 1.5-ml microcentrifuge tube (assuming a chromosome concentration of about 3–4 × 107 chromosomes/ml).
2.Add 25 µl nuclear lysis buffer.
3.Add 2.5 µl of 20% SDS.
4.Wait several seconds and then check the viscosity of the sample by stirring the preparation and by drawing a small quantity into a pipet tip and then withdrawing the tip from the preparation.
Basic Protocol 5: CHROMOSOME ANALYSIS AND SORTING
After the single-chromosomal suspension is prepared, it is subjected to flow cytometric analysis. This allows us to identify the individual chromosomes, apply appropriate gating strategies, and sort the chromosomal population of interest. This processing is depicted in the schematic presented in Figure 2.
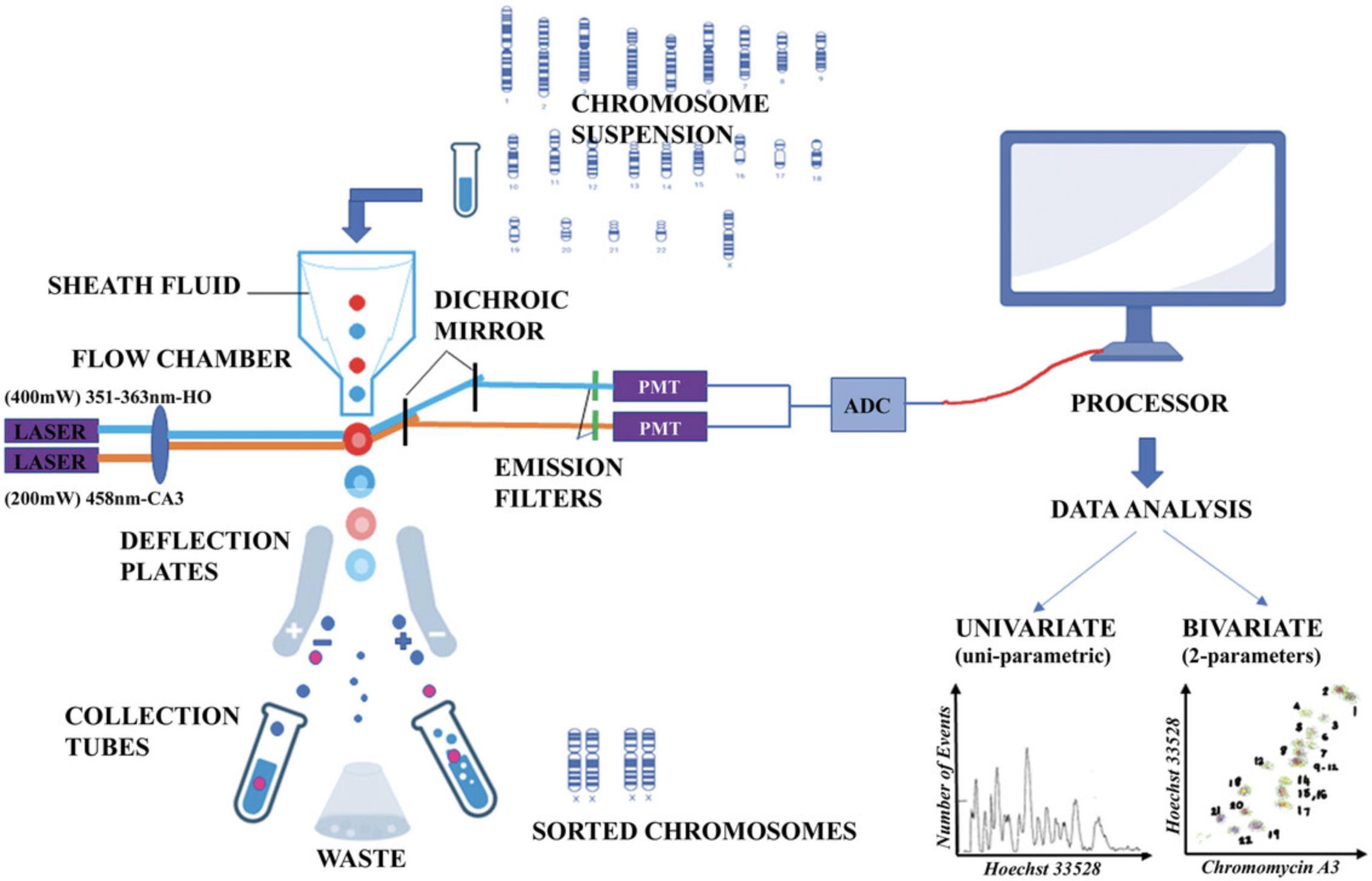
Critical Requirements for Chromosome Sorting
Univariate data acquisition
Analysis of single-labeled (univariate) chromosomes stained with PI requires a cytometer equipped with a blue-green 488-nm laser, found virtually on all instruments. Laser power should be relatively high, typically 50 mW or more, up to 200 mW. Although cytometers used for chromosome analysis have historically been jet-in-air systems, cytometers equipped with enclosed quartz cuvettes are now almost universal and may require less laser power than older systems. However, laser power should still be maximized and considered when designing a system. Univariate analysis is done with a detector equipped with a PI filter, usually ranging from ∼575 to 630 nm (orange). Typical bandpass filters including 575/26 nm, 585/42 nm, and 630/32 nm are commonly used.
Bivariate data acquisition
Analysis of Hoechst 33258 and Chromomycin A3 requires ultraviolet (320 to 365 nm) and blue (440 to 460 nm) lasers, respectively. These lasers are distinct from the usual 488-nm lasers used for PI analysis and are only available on specialized instruments. Hoechst 33258 fluorescence has historically been collected by a 420-nm long-pass filter; however, success has been reported using a blue bandpass filter (460/50 nm), and similarly, Chromomycin A3 fluorescence can be collected by a 480-nm long-pass filter or a green-to-orange bandpass filter, such as 550/50 nm (Langlois et al., 1982, Yang et al. 2011).
Instrument optimization and quality control
Instrument performance should be verified prior to analysis using uniform fluorescent microspheres excited by the lasers on the instrument. Many are available, including Spherotech Ultra microspheres, which are excited at many laser wavelengths, including ultraviolet, and are commonly used for this purpose. Not all quality-control microspheres excite using ultraviolet lasers; if using Hoechst 33258, make sure alignment particles are appropriate for this wavelength. Because chromosomes are small objects, optimization of alignment using submicron microspheres is highly recommended. Instrument alignment can be different for large objects and small ones. Optimization of a flow cytometer for chromosome analysis may require the services of a trained field service engineer informed in the specialized nature of this work.
Instrument triggering
Light scatter is the most commonly used event trigger in flow cytometry; however, because of the small size of chromosomes and the large amount of debris present in chromosome preps, this is not a reliable parameter. Instead, fluorescence should be used to trigger the instrument. As with all aspects of chromosome analysis, they should be treated as submicron objects and analyzed and sorted as all submicron objects are. Care should be taken to ensure all chromosomes are seen on the plot and are not below the threshold value.
Chromosome sorting
Physical separation of chromosomes on a cell sorter (as opposed to analysis only on a benchtop flow cytometer) has additional technical considerations. The smallest nozzle size with high sheath pressure and drop drive frequency is recommended for chromosome sorting. Nozzle sizes typically range from 70 to 130 μm; use the smallest size available.
Sheath buffer is used to hydrodynamically focus the sample stream through the instrument nozzle and laser beam. This buffer is normally PBS or similar for normal cell sorting. For cell sorting, the sheath buffer should match the final chromosome sample buffer. Sheath and sample buffers will mix during the sorting process; it is therefore critical that they be of the same composition. This maintains the chromosomes at a uniform pH and is ideal for high-pressure sorting. The refraction of light between different materials, or even two liquids with different refractive indices, lowers the resolution of chromosomes. By matching the sheath fluid to the liquid that the chromosomes are suspended in, one point of variation can be eliminated. This will require a large quantity of this buffer for a typical cell sort.
The chromosomes must be sorted into a buffer and tube compatible with subsequent methods. Polypropylene collection tubes are recommended due to their lower electrical charge accumulation, reducing the chance of droplet spatter and dispersion during the sorting process. Because physical damage is not an issue, chromosomes can be sorted into a tube with no collection buffer. However, if a specialized collection medium is needed for post-sort processing, chromosomes can be sorted directly into tubes containing a small quantity of this material. When sorting for a long period of time, the sample and the collection tubes should be maintained at 4°C. A small number of sorted chromosomes should also be collected for post-sort purity analysis. Typical sort purities should exceed 95%.
REAGENTS AND SOLUTIONS
HMW stock solution A, 10×
- 2.36 g Tris·HCl
- 0.760 g EDTA
- 5.96 g KCl
- 1.16 g NaCl
- 100 ml distilled H2O
- Filter-sterilize
- Store ≤1 month at room temperature
HMW stock solution B, 10×
- 0.190 g EGTA
- 100 ml distilled H2O
- Add concentrated NaOH dropwise to dissolve
- Filter-sterilize
- Store ≤1 month at room temperature
IB01 (LMW) isolation buffer
- 1.0 ml 100 mM MgSO4, pH 8.0
- 9.0 ml 55 mM KCl + 5.5 mM HEPES solution, pH 8.0 (should be prepared ≤1 week in advance)
- 0.5 ml 3.0 mg/ml RNase stock in 50 mM Worthington KCl at 47330 U/mg
- 0.25 ml 120 mM dithiothreitol in water (prepared and aliquoted in small amounts and frozen until use)
- Filter through a 22-µm Millipore (disposable) filter
- Prepare fresh immediately before use
IB02 (HMW) isolation buffer
- 2.5 ml 10× HMW stock solution A (see recipe)
- 2.5 ml 10× HMW stock solution B (see recipe)
- 20 ml distilled H2O
- Adjust pH to 7.2 ± 0.05 with HCl
- 25 µl 2-mercaptoethanol
- 15 mg digitonin
- Incubate at 37°C for 45 min
- 12.5 µl 0.4 M spermine tetrahydrochloride solution (see recipe)
- 12.5 µl 1.0 M spermidine trihydrochloride solution (see recipe)
- Prepare immediately before use and place on ice
LVPB (sheath fluid)
- 3.4 L distilled H2O
- 400 ml LVPB solution A (EGTA; see recipe)
- 200 ml LVPB solution B (EDTA; see recipe)
- Adjust pH to 7.2 using concentrated HCl or NaOH
- 2 ml LVPB spermine (see recipe)
- 2 ml LVPB spermidine (see recipe)
- Ensure continuous mixing with magnetic stirrer
- Prepare fresh immediately before use and keep at room temperature
LVPB solution A (EGTA)
- 1.8 L distilled H2O
- 3.8 g EGTA, anhydrous
- Begin dissolving using magnetic stirrer
- Add NaOH to dissolve EGTA
- If EGTA is not in solution after 15 min, add 1 pellet of NaOH every 5-10 min until EGTA is fully dissolved
- Add distilled H2O to make 2.0 L and mix well
- Store ≤1 month at 4°C
LVPB solution B (EDTA)
- 0.9 L distilled H2O
- 119.28 g KCl
- 47.28 g Tris·HCl, anhydrous
- 23.38 g NaCl
- 15.21 g EDTA, anhydrous
- Dissolve using magnetic stirrer
- Add distilled H2O to make 1 L and mix well
- Store ≤1 month at 4°C
LVPB spermine
- 13.93 g spermine tetrahydrochloride
- 100 ml distilled H2O
- Aliquot into 2.0-ml portions
- Store ≤1 month at −20°C and thaw just before use
LVPB spermidine
- 25.46 g spermidine trihydrochloride
- 100 ml distilled H2O
- Aliquot into 2.0-ml portions
- Store ≤1 month at −20°C and thaw just before use
McIlvaine's buffer, pH 7
- 16.47 ml 0.2 M disodium hydroxyphosphate
- 3.53 ml 0.1 M citric acid
- Prepare immediately before use
Nuclear lysis buffer
- 100 mM Tris·HCl, pH 8
- 100 mM EDTA
- 10 mM NaCl
- Store ≤1 month at 4°C
Ohnuki's hypotonic swelling buffer
- 5 ml 55 mM sodium nitrate
- 2 ml 55 mM sodium acetate
- 10 ml 55 mM KCl
- 14.1 µl 0.4 M spermine tetrahydrochloride solution (see recipe)
- 14.1 µl 1.0 M spermidine trihydrochloride solution (see recipe)
- Prepare immediately before use
PI solution A
- 75 mM KCl
- 50 µg/ml PI (Thermo Fisher, cat. no. P1304MP)
- Prepare in 10 ml distilled H2O
- Filter-sterilize
- Store ≤1 month at 4°C
PI solution B
- 75 mM KCl
- 50 µg/ml PI (Thermo Fisher, cat. no. P1304MP)
- 1% (w/v) Triton X-100 (make fresh)
- 1 mg/ml RNase
- Prepare in 10 ml distilled H2O
- Filter-sterilize
- Store ≤1 month at 4°C
Spermine tetrahydrochloride solution, 0.4 M
- 1.39 g spermine tetrahydrochloride
- 10 ml distilled H2O
- Freeze in 0.1-ml aliquots and do not refreeze after use
- Store ≤1 month at 4°C
Spermidine trihydrochloride solution, 1.0 M
- 2.54 g spermidine trihydrochloride
- 10 ml distilled H2O
- Freeze in 0.1-ml aliquots and do not refreeze after use
- Store ≤1 month at 4°C
COMMENTARY
Background Information
In the 1970s, the analysis and isolation of chromosomes using flow cytometry were a novel approach in the field of cytogenetics. The combination of flow cytometry (microfluorometry) and sorting using flow cytometers enabled the application of DNA content measurement, rapid processing, and analysis of individual metaphase chromosomes that could also be sorted. This was developed as an alternative to cytophotometric analysis and isolation of low-purity chromosomes using methods like velocity sedimentation and zonal centrifugation (Trask, 2002). Stubblefield et al. (1975) and Gray et al. (1975) independently introduced chromosomal analysis as an application of flow cytometry.
Stubblefield and his colleagues analyzed ethidium bromide (EtBr)-stained chromosomes isolated from a Chinese hamster cell line using partial fractionation by zonal centrifugation on sucrose gradients. The analysis resulted in a resolvable pattern of overlapping peaks, each corresponding to the chromosomes, creating a flow-oriented karyotype (Stubblefield et al., 1975). Gray and his team were the first to isolate individual chromosomes from a Chinese hamster cell line using EtBr staining and flow cytometric analysis. Using an electronic sorter, they sorted the chromosomes based on the peaks they corresponded to on the single-color karyogram (Gray et al., 1975). Their results indicated that flow-oriented karyotyping and sorting could pave the way for a rapid method of preparing a large number of chromosomes of high purity and enhanced resolution for biochemical/biological applications and studies. For the first time, in 1976, Callis and Hoehn explored the possibility of using this method for the diagnosis of aneuploidy as an alternative to conventional cytogenetics. By comparing euploid and aneuploid samples, they were able to determine the variation in chromosomal content, but the interindividual DNA content variation was too substantial to diagnose Down's syndrome (trisomy 21) using univariate analysis (Callis & Hoehn, 1976). To alleviate this drawback, Langlois and Jensen presented a report on using two dyes (bivariate analysis) to measure the fluorescence intensities for each chromosome and create a karyogram based on both the chromosomal size and base pair composition. Their work showed that a dual-dye approach makes it possible to obtain a maximum resolution of chromosomes into individual peaks. The most commonly used DNA stain combination is Hoechst 33258 and Chromomycin A3, due to their differential nucleotide-binding affinities for A:T and G:C nucleotides, respectively, and their ability to be excited by lasers available for flow cytometry (Langlois & Jensen, 1979).
The advent of flow cytogenetics provided a robust quantitative cytochemical analysis that was statistical in nature due to the high number of events, i.e., each chromosome measured from one sample. In comparison to traditional karyotyping, this method not only generated a normal karyotyping but also provided a precise measurement of relative DNA content (measured by fluorescence), thereby allowing for the detection of structural and/or numerical chromosomal aberrations (Boschman et al., 1992; Cooke et al., 1989; Otto, 1988). However, it does require more cells than traditional karyotyping, and the chromosomes from cells in a population are mixed. Additionally, chromosomes with very similar DNA content and ratios of A:T to G:C cannot be separated. Flow-sorted chromosomes were also used for gene mapping to integrate genetic and physical chromosomal maps (Lebo, 1982), for construction of chromosome-specific recombinant DNA libraries (Korstanje et al., 2001; Van Dilla & Deaven, 1990), and for the study of chromosomal aberrations using generated chromosome painting probes (Carter, 1994). Over the years, flow analysis and sorting of metaphasic chromosomes (flow cytogenetics) have aided in the progress of human and animal chromosome characterization and genome mapping.
Accumulation of metaphase chromosomes
Before isolating chromosomes, an important goal is to maintain appropriate culture conditions to ensure that a large number of cells is available for mitotic arrest. This is accomplished by stimulating a large fraction of the cell population in culture into active proliferation. The next step is to block these cells in metaphase using anti-mitotic drugs such as Colcemid or colchicine. During mitosis, these chemicals bind to soluble tubulin, forming tubulin-colchicine complexes that prevent the elongation of microtubule polymer as these complexes bind to it (Leung et al., 2015). This in turn blocks the formation of spindles and allows the condensed chromosomes to accumulate at the metaphase plate. Without this addition, the chromosomes would be tethered to other cell structures, thereby increasing clumping and reducing resolution.
Mitotic cells among adherent cells in monolayer cultures generally round up, reducing their adhesion to the extracellular matrix, and are easily dislodged. Using a method of physically dislodging mitotic cells, generally by sharply shaking the flask, reduces the number of interphase cells in the prep. Whereas, in the case of suspension cultures or non-adherent cell lines, it is important to synchronize the culture before the Colcemid treatment to achieve a higher mitotic index. Aphidicolin inhibits DNA polymerase and can be used to block cells at the G1/S boundary and then can be washed out to allow cell cycle progression (Matherly et al., 1989). Similarly, washing the cells with serum-free medium and allowing them to incubate in that medium will cause them to accumulate in G0 (Davis et al., 2001). For these protocols, removal of aphidicolin or re-addition of medium will allow the cells to reenter the cell cycle in a synchronized fashion. A mitotic block on synchronized cells will reduce the number of cells in other cell cycle phases. Alternatively, mitotic cells can also be enriched by utilizing a velocity gradient that depends on cell size (Davis et al., 2001). Lastly, cell viability needs to be maximized in suspension cultures, as the shake selection method cannot be utilized to distinguish between live and dead cells (Gray & Cram, 1990).
Preparation of the chromosome suspension
Isolation of chromosomes from cells arrested in metaphase involves three steps: (i) cell swelling, (ii) chromosome stabilization, and, lastly, (iii) cell shearing to release the chromosomes in suspension. Cell swelling is achieved by placing the mitotic cells in a hypotonic solution; this swells the cell membrane and thereby allows the metaphase chromosomes to separate. This is followed by the stabilization of chromosomes using a buffer. The three common chromosome stabilization buffers are named after the component that acts as a stabilizing agent; these are PI, MgSO4, and polyamines (Cram et al., 2002). Table 1 shows a comparison between the isolation procedures referred to in this article.
Common name | Characteristics of the isolated chromosomes | Advantages | References |
---|---|---|---|
Propidium iodide | Slightly extended but overall excellent morphology | Protocol of choice for univariate analysis, high resolution, easily reproducible | (Bijman, 1983; Buys et al., 1982) |
Magnesium sulfate (MgSO4) | Difficult to recognize due to slight contractions | Allows dual staining, high resolution, preferable for low cell number | (van den Engh et al., 1984; van den Engh et al., 1985) |
Polyamine | Difficult to recognize due to slight contractions | Preferable for cells in suspension, high resolution, allows dual staining, maintains high molecular weight | (Blumenthal et al., 1979; Sillar & Young, 1981) |
The isolation buffers also contain RNase, which improves DNA-specific staining for the isolated chromosomal preparations (Bartholdi et al., 1983). Due to the presence of enzymes and their action on cells and chromosomal material, these buffers need to be maintained at a certain pH depending on their composition. This is supported by the microscopic examination of the preparations. At low pH, due to incomplete disruption of cells, clumps have been observed. A similar variation in chromosome preparation quality with a change in pH can be found with each of the three buffers (van den Engh et al., 1984).
Instrumentation
Although the laboratory techniques used to prepare chromosomes for flow cytometric analysis and sorting have remained unchanged for some time, the cytometric equipment used for this work has changed dramatically from the original cytometers used to develop these methods. When techniques for chromosome analysis and sorting were originally developed in the 1980s, almost all flow cytometers were cell sorters. These instruments were almost exclusively (1) large-scale cell sorters, used for both analysis and cell separation; (2) dependent on high-powered water-cooled gas lasers; (3) jet-in-air instruments, with a nozzle that projected sheath and cell streams into the open air for laser interrogation prior to droplet generation and sorting; and (4) very slow in analysis and sorting. The development of user-friendly benchtop analyzers was only beginning at this time. Both analyzers and sorters are now (1) much smaller in size while possessing much greater analytical capabilities; (2) reliant on smaller air-cooled solid-state lasers; (3) equipped with enclosed cuvette flow cells or hybrid cuvette/jet-in-air nozzles; and (4) fully digital, allowing far higher analysis and sorting rates than their earlier analog predecessors. Chromosome analysis and sorting are still possible and often enhanced by these modifications, but changes to technology need to be taken into account when choosing cytometers for this work and designing experiments.
Lasers
Single-color (univariate) chromosome analysis with PI on large cell sorters historically required a powerful argon 488-nm laser, with power levels of 100 to 300 mW. Older cytometers were primarily jet-in-air, with low optical efficiencies requiring higher power levels. Modern flow cytometers are now equipped with air-cooled diode-pumped solid-state or direct-diode 488-nm lasers. These lasers require much less maintenance and have a much longer lifespan. Modern cytometers also use enclosed quartz cuvettes with closely coupled optics for laser interrogation and signal detection, allowing lower-power lasers to be used. Benchtop cytometers typically use 488-nm lasers emitting between 20 and 50 mW; cell sorters often employ somewhat more powerful lasers, emitting between 50 and 200 mW. Although several authors have demonstrated that extremely high laser power is not necessarily required for chromosome analysis, the small size of chromosomes and the small amount of DNA dye bound to them suggests the need for a higher laser power (Frey et al., 1993; Snow & Cram, 1993). Modern benchtop systems also often install “top hat” and other beam-flattening optics that improve analysis of larger objects but can reduce beam spot power and reduce sensitivity for smaller targets.
When designing a custom system, a good general rule is to use the highest laser power levels available from the manufacturer. For solid-state lasers, in the hundreds-of-milliwatts range, higher power can often be produced in a module of physical size equal to that of lower-power modules, so producing 100 more milliwatts from a small laser is usually possible. Solid-state 488-nm lasers ranging from 200 to 300 mW are now available and may improve results, and even lasers in the 50-to-100 mW range may be sufficient if the laser and detection optics are good. If the system being used can only employ lower-power laser modules, test the system carefully with good chromosomal preparations. Standard analyzer and sorter systems may only use lower-power lasers, with higher-power options not available. The choices available for custom instrumentation are not very plentiful (see the “Nozzle and flow cell design” section below). When acquiring a new system or modifying an old one, always notify the manufacturer of your intended application.
Bivariate chromosome analysis with Hoechst 33258 and Chromomycin A3 requires an ultraviolet laser (320 to 365 nm) and a blue laser (440 to 460 nm), respectively. Historically, these wavelengths were generated using argon-ion lasers, which could produce these laser lines if the laser optics were modified. Modern multi-laser cytometers employ frequency-tripled Nd:YVO4 solid-state ultraviolet lasers; these units are increasing in power and are now available at levels approaching those of gas lasers. Blue solid-state lasers are now available both as direct diodes (440 to 450 nm) and as diode-pump solid-state units (457 nm). These modules can be quite powerful. Unfortunately, whereas ultraviolet lasers are common (if expensive) options on high-end analyzers and sorters, blue lasers have few applications and are not a common laser option. At this writing, BD Biosciences is one of the few manufacturers that will produce custom analyzer and sorter configurations with ultraviolet and blue solid-state lasers. For both lasers, somewhat higher power levels (100 to 200 mW) are recommended, although lower levels may be effective with efficient signal collection optics.
Laser beam focusing and shaping
Chromosome analysis requires a laser beam with a tightly focused beam profile, both to concentrate energy on the sample stream and to minimize the analysis of multiple chromosomes as a single event (coincidence). Conventional commercial cytometers may need to have their beam shaping and focusing modified for optimal chromosome analysis and sorting. The instrument manufacturer may be able to make these modifications and improve laser focusing for instruments dedicated to chromosome work. Very small quality-control beads (0.5 to 1 μm) should be used for instrument alignment rather than the larger particles typically used; optimal laser alignment for larger objects may not be the same as for smaller ones.
Nozzle and flow cell design
Older instruments for chromosome analysis and sorting used jet-in-air nozzles, where both laser interrogation and droplet generation/sorting occurred in a cell stream projected into the open air. Older cell sorters usually had interchangeable nozzles with varying sample stream diameters, and the smallest size (usually 50 μm) in diameter was usually used. Using small nozzle diameters in theory reduced the required laser spot size, allowing higher laser power and signal resolution. It also allowed higher sort rates. The disadvantages of smaller nozzle sizes are increased clogging and the possibility of lower purity due to closer chromosome spacing in the stream. Modern analyzers use enclosed quartz cuvettes, which can be more efficiently coupled to signal collection optics. Modern sorters almost all use hybrid cuvette systems, where objects are analyzed in a quartz cuvette but then projected into the air for droplet generation and sorting. Chromosomes have been successfully analyzed and sorted using both of these systems (Picot et al., 2012). In theory, chromosome resolution may actually improve with a cuvette system. However, flexibility in nozzle and stream diameters on cell sorters is now more restricted than in older systems. Modern instruments normally have a minimum stream diameter of 70 μm, with larger diameters like 100 μm often standard and non-changeable on user-friendly sorters. Larger stream diameters reduce the laser cross-section and can reduce small object resolution. Use the smallest nozzle diameter possible. Keep in mind that smaller nozzles are more prone to clogging. If the chromosome preparation quality is good, clogging should be minimal with these submicron objects.
Instrument selection
Chromosome analysis and sorting have been traditionally done on a few large-scale cytometer platforms, including the DakoCytomation (now Beckman-Coulter) MoFlo, the Cytopeia (now BD Biosciences) Influx, and the BD FACSVantage. These systems are no longer manufactured. Modern cell sorters may not be equipped with the necessary lasers and optics for good chromosome analysis and sorting. It is critical to discuss the conditions required for chromosome analysis with the manufacturer prior to purchase. As an example, BD Biosciences analyzers and sorters can be equipped with custom high-power lasers and modified optics for chromosome analysis and sorting under their Special Order Research Product (SORP) program. Other manufacturers may also be able to install high-power lasers within the same space as the usual lower-power versions. Some instrument customizations will likely be necessary for high-resolution chromosome detection, especially for bivariate analysis. Post-installation modifications to beam focusing and other instrument elements may also be available to improve the detection of small particles like chromosomes. These are also cytometry systems available designed for submicron object analysis; these changes might be beneficial for chromosome analysis as well. It is an unfortunate situation that the large number of more economical cytometry systems available today has reduced the options for custom systems.
Critical Parameters
Cell swelling
Monitoring of cell swelling (Support Protocol 1) is critical for the success of chromosome preparation and subsequent analysis. A microscope equipped with fluorescent optics should be available for this monitoring.
Chromosome sorting
For chromosome separation (as opposed to analysis only; Basic Protocol 5), the sheath buffer should match the chromosome suspension solution. Mixing of chromosome sample and sheath buffer will occur during the sorting process, causing possible chromosome structural changes and damage during separation. A large quantity of this buffer will need to be prepared prior to sorting. For the best resolution, the sheath buffer needs to match the chromosome suspension solution.
Sample temperature
Keep samples at the temperatures indicated in the procedures at all times. Failure to maintain samples at 4°C can reduce chromosome yield and alter the structure.
Chromosome storage
Chromosomes do not need to be analyzed immediately after isolation as they are stabilized by the buffers and dyes. Chromosomes isolated using Basic Protocol 2 can be stored up to 3 weeks at 4°C, whereas chromosomes isolated using Basic Protocol 4 can be stored up to 1 year at 4°C; this is because the isolation buffer contains EGTA or EDTA, which maintain the integrity of the DNA.
Troubleshooting
The possible causes of errors in different protocol steps that may contribute to incorrect data interpretation or inefficient sorting are listed in Table 2.
Step | Problem | Possible cause | Solution |
---|---|---|---|
Preparation of chromosomal suspension | Not achieving a suspension of single chromosomes | Nuclei, chromosome clumps, or single chromatids |
Optimal lysing conditions are required to obtain a high proportion of individual chromosomes and negligible contaminants. An ideal sample concentration is 3 × 107 chromosomes/ml. Pure chromosomes will have an appropriate ratio of A:T to G:C. |
Chromosome clumps | Optimize cell swelling to ensure that cells are not lysing prematurely. See Support Protocol 1. Maintain chromosome suspensions at 4°C. | ||
Nuclear and cellular debris | A volume of 5 ml of the chromosome yield can be segregated into a 15-ml tube and subjected to repeated centrifugation for 3 min at 30 × g, at 4°C, to pellet the nuclei. The obtained clear supernatant is vortexed and re-centrifuged. | ||
Integrity | Yield not intact | After every isolation, the sample can be either stored at 4°C or processed. In the case of processing a stored sample, an extra 45 s of centrifugation is required. | |
Sorting of chromosomes | Chromosome resolution | Instrumentation |
The choice of nozzle size and stream diameter can change the ability to obtain highly resolved chromosome populations. A smaller nozzle diameter is preferred for this. Higher laser powers may be needed for maximum resolution. Ensure laser and emission filter wavelengths are ideally suited to the selected fluorochromes. |
Understanding Results
The data are obtained in the form of univariate and bivariate flow karyograms depending on the number of parameters used to discriminate among the chromosomes. Histograms of different expression profiles can be overlaid to allow readers to grasp the differential expression in a pattern. Bivariate graphs or plots can display the same data using different formats, including dot plots, contour plots, or density plots. These displays can be smoothened further using analysis software where one can apply outlier contour plots or density plots with color codes and also introduce newer display formats like outlier zebra plots, which are a combination display of contour with outlying dots. Table 3 highlights the differences between the two forms of data acquisition and their impact on the analysis of karyograms.
Parameter | Univariate | Bivariate |
---|---|---|
Dye used | Chromosomes are stained with a single fluorescent DNA dye like propidium iodide (PI), ethidium bromide (EtBr), Hoechst 33258 (HO), or Chromomycin A3 (CA3) | Chromosomes are stained with two fluorescent DNA dyes, generally CA3 (binds to GC-rich regions) and HO (binds to AT-rich regions) |
DNA base | Not specific for PI or EtBr. For HO or CA3, it is useful with a constant (or nearly) base ratio of A:T to G:C for all types of chromosomes | Chromosomes of species with differing DNA base compositions can be analyzed better using bivariate flow karyotype data. Hence, it is useful in distinguishing human chromosomes that are larger in size, with a higher HO/CA3 ratio, from hamster chromosomes in isolated human-hamster hybrid cells. Chromosomes within a species also have variable base ratios of A:T to G:C, which allows further resolution of the karyotype. |
Resolving ability |
Intercalating fluorescent dyes like PI bind stoichiometrically and specifically to double-stranded nucleic acid. Chromosomes with DNA content differing by <0.5% (∼1 fg DNA) to 2% (5 fg DNA) can be resolved as separate peaks in the flow karyotypes. It is important to have significant differences in the content of DNA. |
The flow karyotype is characterized by the mean of the fixed HO/CA3 ratio of a band of peaks that fall as a single diagonal. The chromosomes that differ in the base composition form peaks that fall off the diagonal. These are better resolved in bivariate than univariate analysis. |
Feasibility | Practical when a single laser is available for analysis. It is important to have a low coefficient of variation. Particularly useful in species with small numbers of chromosomes. | Useful in species with larger numbers of chromosomes. It is used to purify large human chromosomes to produce chromosome gene maps or recombinant DNA libraries. |
Figure 3 provides an example of how chromosomal aberrations can be detected using flow cytometric analysis and can further be sorted for downstream applications.
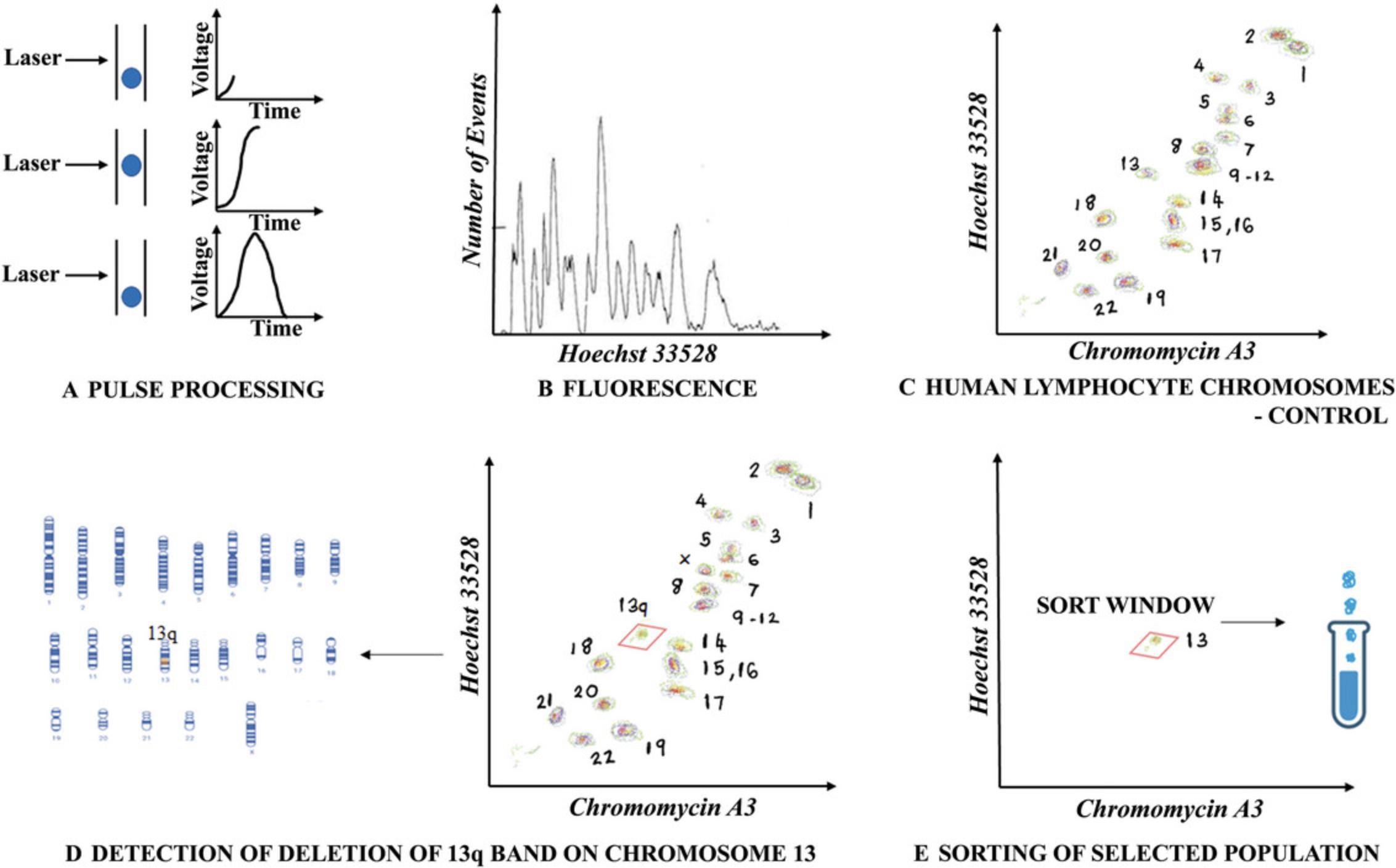
Time Considerations
From the isolation of metaphase chromosomes for preparing single-chromosome suspensions (Basic Protocol 1) to their analysis and chromosome sorting (Basic Protocol 5), the complete procedure might take up to a few days. Therefore, the experiment needs to be carefully planned and organized. Even though the protocols listed here are not complex or difficult to perform, they require a certain level of expertise in execution. Table 4 lists how long it will take to perform the protocols described in this article.
Protocol name | Time consideration | Points to be noted |
---|---|---|
Basic Protocol 1: Mitotic block and cell harvesting | Once the culture is sub-confluent, it takes 3-15 hr for the Colcemid to act and recover the cells | It may take several days to optimize the incubation time depending on the cell type of choice and its growth rate |
Basic Protocol 2: Propidium iodide isolation | The whole procedure takes a maximum of 2 hr to complete | It must be performed immediately after the cells have been mitotically blocked |
Basic Protocol 3: MgSO4 low-molecular-weight isolation | The whole procedure takes a maximum of 2 hr to complete | It must be performed immediately after the cells have been mitotically blocked |
Basic Protocol 4: Polyamine high-molecular-weight isolation | The whole procedure takes a maximum of 6 hr to complete | It must be performed immediately after the cells have been mitotically blocked |
Basic Protocol 5: Chromosome analysis and sorting | The analysis and sorting rate depends on the concentration of chromosomes. The total time required is determined by the number of chromosomes that need to be analyzed or sorted. |
Alignment of the components of the flow cytometer is time consuming and needs to be done by trained specialists regularly. Problems with the instrument may cause delays. A low fluidic flow rate should be used to minimize sample diameter and maximize resolution. |
Acknowledgments
WGT is supported by intramural research funds from the National Cancer Institute and the National Institutes of Health. CKS is supported by the DOE EERE Bioenergy Technologies Office and LANL Laboratory Directed Research and Development funds. UC is supported by intramural funding from the Manipal Academy of Higher Education.
Author Contributions
Risani Mukhopadhyay : Data curation, investigation, methodology, resources, visualization, writing – original draft, writing – review and editing; DV Varshitha : Data curation, investigation, methodology, resources, visualization, writing – original draft, writing – review and editing; William G. Telford : Conceptualization, data curation, formal analysis, investigation, methodology, resources, supervision, validation, visualization, writing – original draft, writing – review and editing; Claire K. Sanders : Conceptualization, data curation, formal analysis, methodology, resources, supervision, validation, visualization, writing – original draft, writing – review and editing; Uttara Chakraborty : Formal analysis, investigation, project administration, supervision, writing – original draft, writing – review and editing.
Conflict of Interest
WGT is an editorial board member for the cytometry section of Current Protocols but played no role in the editorial review of this article. No remuneration was received for this work.
Open Research
Data Availability Statement
Data sharing is not applicable to this article as no new data were created or analyzed. The figures presented in this article contain data of historical interest only and are not available for further analysis.
Literature Cited
- Bartholdi, M. F., Ray, F. A., Cram, L. S., & Kraemer, P. M. (1987). Karyotype instability of Chinese hamster cells during in vivo tumor progression. Somatic Cell and Molecular Genetics , 13(1), 10. https://doi.org/10.1007/bf02422294
- Bartholdi, M. F., Sinclair, D. C., & Cram, L. S. (1983). Chromosome analysis by high illumination flow cytometry. Cytometry , 3(6), 395–401. https://doi.org/10.1002/cyto.990030602
- Bijman, J. T. (1983). Optimization of mammalian chromosome suspension preparations employed in a flow cytometric analysis. Cytometry , 3(5), 354–358. https://doi.org/10.1002/cyto.990030509
- Blumenthal, A. B., Dieden, J. D., Kapp, L. N., & Sedat, J. W. (1979). Rapid isolation of metaphase chromosomes containing high molecular weight DNA. The Journal of Cell Biology , 81(1), 255–259. https://doi.org/10.1083/jcb.81.1.255
- Boschman, G. A., Manders, E. M., Rens, W., Slater, R., & Aten, J. A. (1992). Semi-automated detection of aberrant chromosomes in bivariate flow karyotypes. Cytometry , 13(5), 469–477. https://doi.org/10.1002/cyto.990130504
- Buys, C. H., Koerts, T., & Aten, J. A. (1982). Well-identifiable human chromosomes isolated from mitotic fibroblasts by a new method. Human Genetics , 61(2), 157–159. https://doi.org/10.1007/BF00274208
- Callis, J., & Hoehn, H. (1976). Flow-fluorometric diagnosis of euploid and aneuploid human lymphocytes. American Journal of Human Genetics , 28(6), 577–584.
- Carrano, A. V., Gray, J. W., Langlois, R. G., Burkhart-Schultz, K. J., & van Dilla, M. A. (1979). Measurement and purification of human chromosomes by flow cytometry and sorting. Proceedings of the National Academy of Sciences of the United States of America , 76(3), 1382–1384. https://doi.org/10.1073/pnas.76.3.1382
- Carter, N. P. (1994). Cytogenetic analysis by chromosome painting. Cytometry , 18(1), 2–10. https://doi.org/10.1002/cyto.990180103
- Cooke, A., Tolmie, J. L., Colgan, J. M., Greig, C. M., & Connor, J. M. (1989). Detection of an unbalanced translocation (4;14) in a mildly retarded father and son by flow cytometry. Human Genetics , 83(1), 83–87. https://doi.org/10.1007/BF00274155
- Cram, L. S., Bell, C. S., & Fawcett, J. J. (2002). Chromosome sorting and genomics. Methods in Cell Science: An Official Journal of the Society for In Vitro Biology , 24(1-3), 27–35. https://doi.org/10.1023/a:1024108923475
- Cram, L. S., Gray, J. W., & Carter, N. P. (2004). Cytometry and genetics. Cytometry Part A: The journal of the International Society for Analytical Cytology , 58(1), 33–36. https://doi.org/10.1002/cyto.a.20008
- Davis, P. K., Ho, A., & Dowdy, S. F. (2001). Biological methods for cell-cycle synchronization of mammalian cells. Biotechniques , 30, 1322–1331. https://doi.org/10.2144/01306rv01
- Dean, P. N., Kolla, S., & van Dilla, M. A. (1989). Analysis of bivariate flow karyotypes. Cytometry , 10(2), 109–123. https://doi.org/10.1002/cyto.990100202
- Fawcett, J. J., Longmire, J. L., Martin, J. C., Deaven, L. L., & Cram, L. S. (1992). Large-scale chromosome sorting. Methods in Cell Biology , 42, 319–330. https://doi.org/10.1016/s0091-679x(08)61082-1
- Frey, T., Stokdijk, W., & Hoffman, R. A. (1993). Bivariate flow karyotyping with air-cooled lasers. Cytometry (Suppl. 6) , 71.
- Gray, J. W., Carrano, A. V., Steinmetz, L. L., van Dilla, M. A., Moore, D. H. N., Mayall, B. H., & Mendelsohn, M. L. (1975). Chromosome measurement and sorting by flow systems. Proceedings of the National Academy of Sciences of the United States of America , 72(4), 1231–1234. https://doi.org/10.1073/pnas.72.4.1231
- Gray, J. W., & Cram, L. S. (1990). Flow karyotyping and chromosome sorting. In M. R. Melamed & M. L. Mendelsohn (Eds.), Flow cytometry and sorting (pp. 503–529). Wiley-Liss.
- Gray, J. W., Dean, P. N., Fuscoe, J. C., Peters, D. C., Trask, B. J., van den Engh, G. J., & van Dilla, M. A. (1987). High-speed chromosome sorting. Science , 238, 323–329. https://doi.org/10.1126/science.2443974
- Korstanje, R., Gillissen, G. F., den Bieman, M. G., Versteeg, S. A., van Oost, B., Fox, R. R., van Lith, H. A., & van Zutphen, L. F. (2001). Mapping of rabbit chromosome 1 markers generated from a microsatellite-enriched chromosome-specific library. Animal Genetics , 32(5), 308–312. https://doi.org/10.1046/j.1365-2052.2001.00783.x
- Langlois, R. G., & Jensen, R. H. (1979). Interactions between pairs of DNA-specific fluorescent stains bound to mammalian cells. The Journal of Histochemistry and Cytochemistry: Official Journal of the Histochemistry Society , 27(1), 72–79. https://doi.org/10.1177/27.1.86583
- Langlois, R. G., Yu, L. C., Gray, J. W., & Carrano, A. V. (1982). Quantitative karyotyping of human chromosomes by dual beam flow cytometry. Proceedings of the National Academy of Sciences of the United States of America , 79(24), 7876–7880. https://doi.org/10.1073/pnas.79.24.7876
- Lebo, R. V. (1982). Chromosome sorting and DNA sequence localization. Cytometry , 3(3), 145–154. https://doi.org/10.1002/cyto.990030302
- Leung, Y. Y., Yao Hui, L. L., & Kraus, V. B. (2015). Colchicine–Update on mechanisms of action and therapeutic uses. Seminars in Arthritis and Rheumatism , 45(3), 341–350. https://doi.org/10.1016/j.semarthrit.2015.06.013
- Matherly, L. H., Schuetz, J. D., Westin, E., & Goldman, I. D. (1989). A method for synchronization of cultured cells with aphidicolin: Application to the large-scale synchronization of L1210 cells and the study of the cell cycle regulation of thymidylate synthase and dihydrofolate reductase. Analytical Biochemistry , 182, 338–345. https://doi.org/10.1016/0003-2697(89)90605-2
- Matsson, P., Annerén, G., & Gustavsson, I. (1986). Flow cytometric karyotyping of mammals, using blood lymphocytes: Detection and analysis of chromosomal abnormalities. Hereditas , 104(1), 49–54. https://doi.org/10.1111/j.1601-5223.1986.tb00516.x
- Otto, F. J. (1988). Assessment of persisting chromosome aberrations by flow karyotyping of cloned Chinese hamster cells. Zeitschrift fur Naturforschung. C , Journal of Biosciences , 43(11-12), 948–954. https://doi.org/10.1515/znc-1988-11-1223
- Picot, J., Guerin, C. L., le van Kim, C., & Boulanger, C. M. (2012). Flow cytometry: Retrospective, fundamentals and recent instrumentation. Cytotechnology , 64(2), 109–130. https://doi.org/10.1007/s10616-011-9415-0
- Sillar, R., & Young, B. D. (1981). A new method for the preparation of metaphase chromosomes for flow analysis. Journal of Histochemistry and Cytochemistry , 29(1), 74–78. https://doi.org/10.1177/29.1.6162882
- Snow, C., & Cram, L. S. (1993). The suitability of air-cooled helium cadmium (HeCad) lasers for two color analysis and sorting of human chromosomes. Cytometry (Suppl. 6) , 20.
- Stubblefield, E., Cram, S., & Deaven, L. L. (1975). Flow microfluorometric analysis of isolated Chinese hamster chromosomes. Experimental Cell Research , 94(2), 464–468. https://doi.org/10.1016/0014-4827(75)90519-4
- Trask, B., & van den Engh, G. (1990). Chromosome and nuclei isolation with the MgSO4 procedure. Methods in Cell Biology , 33, 363–367. https://doi.org/10.1016/s0091-679x(08)60539-7
- Trask, B. J. (2002). Human cytogenetics: 46 chromosomes, 46 years and counting. Nature Reviews Genetics , 3(10), 769–778. https://doi.org/10.1038/nrg905
- van den Engh, G., Trask, B., Cram, S., & Bartholdi, M. (1984). Preparation of chromosome suspensions for flow cytometry. Cytometry , 5(2), 108–117. https://doi.org/10.1002/cyto.990050203
- van den Engh, G. J., Trask, B. J., Gray, J. W., Langlois, R. G., & Yu, L. C. (1985). Preparation and bivariate analysis of suspensions of human chromosomes. Cytometry , 6(2), 92–100. https://doi.org/10.1002/cyto.990060203
- van Dilla, M. A., & Deaven, L. L. (1990). Construction of gene libraries for each human chromosome. Cytometry , 11(1), 208–218. https://doi.org/10.1002/cyto.990110124
- van Dilla, M. A., Deaven, L. L., Albright, K. L., Allen, N. A., Aubuchon, M. R., Bartholdi, M. F., Brown, N. C., Campbell, E. W., Carrano, A. V., Clark, L. M., Cram, L. S., Crawford, B. D., Fuscoe, J. C., Gray, J. W., Hildebrand, C. E., Jackson, P. J., Jett, J. H., Longmire, J. L., Lozes, C. R., … Trask, B. J. (1986). Human chromosome–specific DNA libraries: Construction and availability. Nature Biotechnology , 4(6), 537–552. https://doi.org/10.1038/nbt0686-537
- Wallace, P. G., Hewish, D. R., Venning, M. M., & Burgoyne, L. A. (1971). Multiple forms of mammalian deoxyribonucleic acid polymerase. An attempt to relate their interactions with nuclei and free deoxyribonucleic acid in vitro with their possible functions in vivo. The Biochemical Journal , 125(1), 47–54. https://doi.org/10.1042/bj1250047
- Wray, W. (1973). Isolation of metaphase chromosomes, mitotic apparatus, and nuclei. Methods in Cell Biology , 6, 283–306. https://doi.org/10.1016/s0091-679x(08)60053-9
- Wray, W., Stubblefield, E., & Humphrey, R. (1972). Mammalian metaphase chromosomes with high molecular weight DNA isolated at pH 10.5. Nature: New Biology , 238(86), 237–238. https://doi.org/10.1038/newbio238237a0
- Yang, H., Chen, X., & Wong, W. H. (2011). Completely phased genome sequencing through chromosome sorting. Proceedings of the National Academy of Sciences of the United States of America , 108(1), 12–17. https://doi.org/10.1073/pnas.1016725108
Corrections
In this publication, the following correction has been made:
The affiliation of the authors, Risani Mukhopadhyay and Uttara Chakraborty has been corrected.
“Manipal Institute of Regenerative Medicine, Manipal Academy of Higher Education, Yelahanka, Bengaluru, India” has been replaced with the correct address.
The current version online now includes these corrections and may be considered the authoritative version of record.