Liposome Synthesis Protocol : Synthesis of anionic and cationic unilamellar liposome nanoparticles using a thin film dispersed hydration and extrusion method.
Brijeshtiwari, James F Curtin, Julie Rose Mae Mondala, Joanna Carroll, Noah Daly, Alancasey, Alessandro Cazzolla
Abstract
Nanoparticles are the future of targeted and sustained drug delivery, yielding significant opportunities in addressing and treat challenging diseases such as cancer (Grenader et al., 2012; Luminari et al., 2010; Shoji et al., 2014). These nano vesicles can be developed into intelligent systems which can encapsulate medicinal and imaging agents, while still possessing stealth properties. Manipulating the size, surface properties, and material composition of these novel systems can help deliver drugs to specific tissues and provide controlled release therapy. Among them, lipid nanoparticles, particularly liposomes, have been identified as promising and adaptable drug vesicles. When compared to standard drug delivery systems, they have superior qualities such as site-targeting, prolonged or controlled release, drug protection from degradation and clearance, superior therapeutic efficacy, and lower toxic side effects.
Liposomes are phospholipid vesicles that form approximately spherical bilayer shapes. These spherical vesicles can contain unilamellar or multilamellar internal concentric lipid bilayers trapping aqueous space. Due to this structure, liposomal vesicles allow for a wide variety of drugs with lipophilic and hydrophilic properties to be encapsulated simultaneously with hydrophobic/lipophilic molecules being inserted into the bilayer membrane and hydrophilic molecules being entrapped in the aqueous compartments. Beginning with Gabizon et al., 1982 and Tardi et al., 1985, liposome researchers have developed a wide range of liposomal drug carriers, by primarily employing small-scale laboratory techniques. The size of liposomes has a significant role in the effective delivery of anticancer drugs to the tumour site. Liposome size has been demonstrated to affect its time in blood circulation, tumour accumulation, tumour retention and the drug release. According to data so far, a liposome diameter of below 200 nm is the ideal size to achieve efficient drug delivery especially when targeting the brain and crossing the blood brain barrier (Gao et al., 2013; Kulkarni & Feng, 2013).
This protocol aims to outline the steps involved in the synthesis of cationic and anionic liposomes with an approximate uniform size of 90 nm for targeted drug delivery in tumour cells. This method employed the use of thin-film dispersed hydration one of the most popular methods for preparing liposomes.
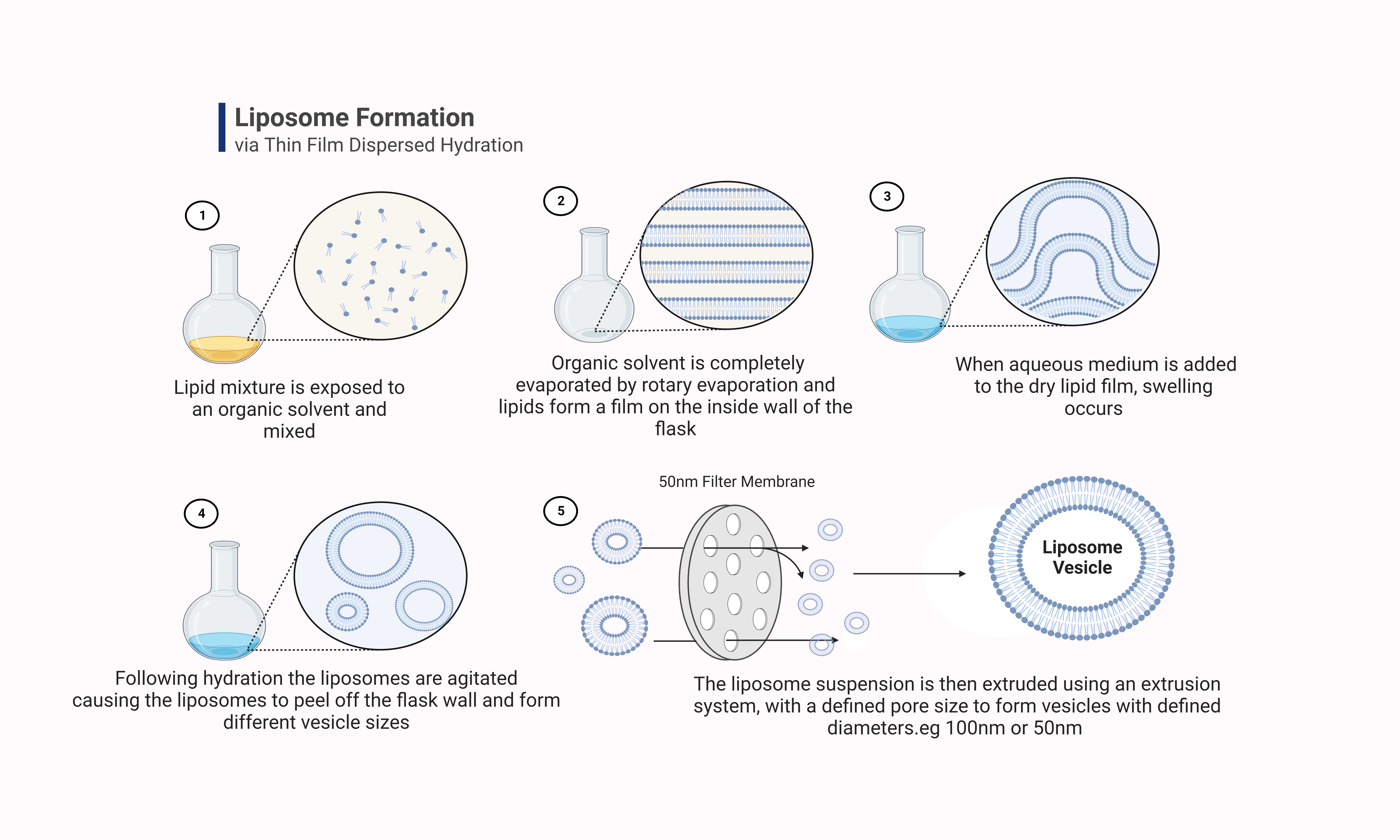
References
Gabizon, A. et al. (1982) “Liposomes as in Vivo Carriers of Adriamycin: Reduced Cardiac Uptake and Preserved Antitumor Activity in Mice,” American Association for Cancer Research , 42(11), pp. 4734–4739.
PMID: 7127308
Gao, W. et al. (2013) “Liposome-like nanostructures for drug delivery,” Journal of Materials Chemistry B , 1(48), p. 6569. DOI: 10.1039/C3TB21238F
Grenader, T. et al. (2012) “Pegylated liposomal doxorubicin/carboplatin combination in ovarian cancer, progressing on single-agent pegylated liposomal doxorubicin,” World Journal of Clinical Oncology , 3(10), p. 137. NIH [Online].
Kulkarni, S.A. and Feng, S.-S. (2013) “Effects of particle size and surface modification on cellular uptake and biodistribution of polymeric nanoparticles for drug delivery,” Pharmaceutical Research , 30(10), pp. 2512–2522. PubMed [Online].
DOI: 10.1007/s11095-012-0958-3
Luminari, S. et al. (2010) “Nonpegylated liposomal doxorubicin (Myocet™) combination (R-comp) chemotherapy in elderly patients with diffuse large B-cell lymphoma (DLBCL): Results from the phase II EUR018 trial,” Annals of Oncology , 21(7), pp. 1492–1499. ScienceDirect [Online].
Shoji, T. et al. (2014) “A phase I study of irinotecan and pegylated liposomal doxorubicin in recurrent ovarian cancer (Tohoku Gynecologic Cancer Unit 104 study),” Cancer Chemotherapy and Pharmacology , 73(5), pp. 895–901. SpringerLink [Online].
DOI: 10.1007/s00280-014-2418-8
Tardi, P., Boman, N. and Cullis, P. (1985) “Liposomal Doxorubicin,” Journal of Drug Targeting , 4(3), pp. 129–140.
Before start
Ensure that the transition temperature of the chosen lipid is known and work above this.
Steps
Synthesis of liposomes via thin film dispersed hydration
The thin-film dispersed hydration method can be used to prepare anionic and cationic liposomes and encapsulated liposomes with (fluorescent dyes, bio compounds or drugs).
In a round bottom pyrex flask (50 ml), add 7 mM of the chosen lipid and 3 mmol of cholesterol and dissolve in 5ml of chloroform. (if the aim is to encapsulate lipid-soluble drugs, dissolve the lipid-soluble drug during this step. Eg. Ursolic Acid 1:20 (w/w).
Stir the solution with a magnetic stirring rod for 15 minutes just above the lipid transition temperature (Tc).
Once stirring is complete, remove the stirring rod from the flask and let the chloroform solvent evaporate using a rotary evaporator at 40 ⁰C.
Note
Evaporation of the solvent should be done at a slow consistent rate until all solvent removed and flask is dry.
Further dry the flask by incubating it overnight in an oven set at just above the Tcof the chosen lipid.
Re-hydrate the lipids that have formed a thin film around the inside of the flask by adding to 2-3 mL of ultrapure water. The volume of water added to the flask depends on the desired final concentration. (If aiming to encapsulate a water-soluble drug or reporter chemical, dissolve the compound during this step. Eg. FITC or PI, 1:20 (w/w).
Stir the rehydrated solution for 30 minutes, using a magnetic stirring rod, making note again to stir the solution on a hot plate with a temperature just above the lipid Tc.
Note
The solution must be turbid when stirring is complete. If after 30 minutes of stirring the solution is not turbid, it may be necessary to manual manoeuvre the stirring of the solution. This will aid in the removal of the lipids from the side of the flask.
Once solution is finished stirring, remove the stirring rod and vortex the solution for 2 minutes.
After vortexing, store the solution at 4°C overnight. This is to prevent acid and base hydrolysis reactions taking place in lipids with ester-linked hydrocarbon chains. Temperature has a significant impact on the rate of reaction so the solution should be refrigerated to slow this process.
Method for Extrusion of Liposomes
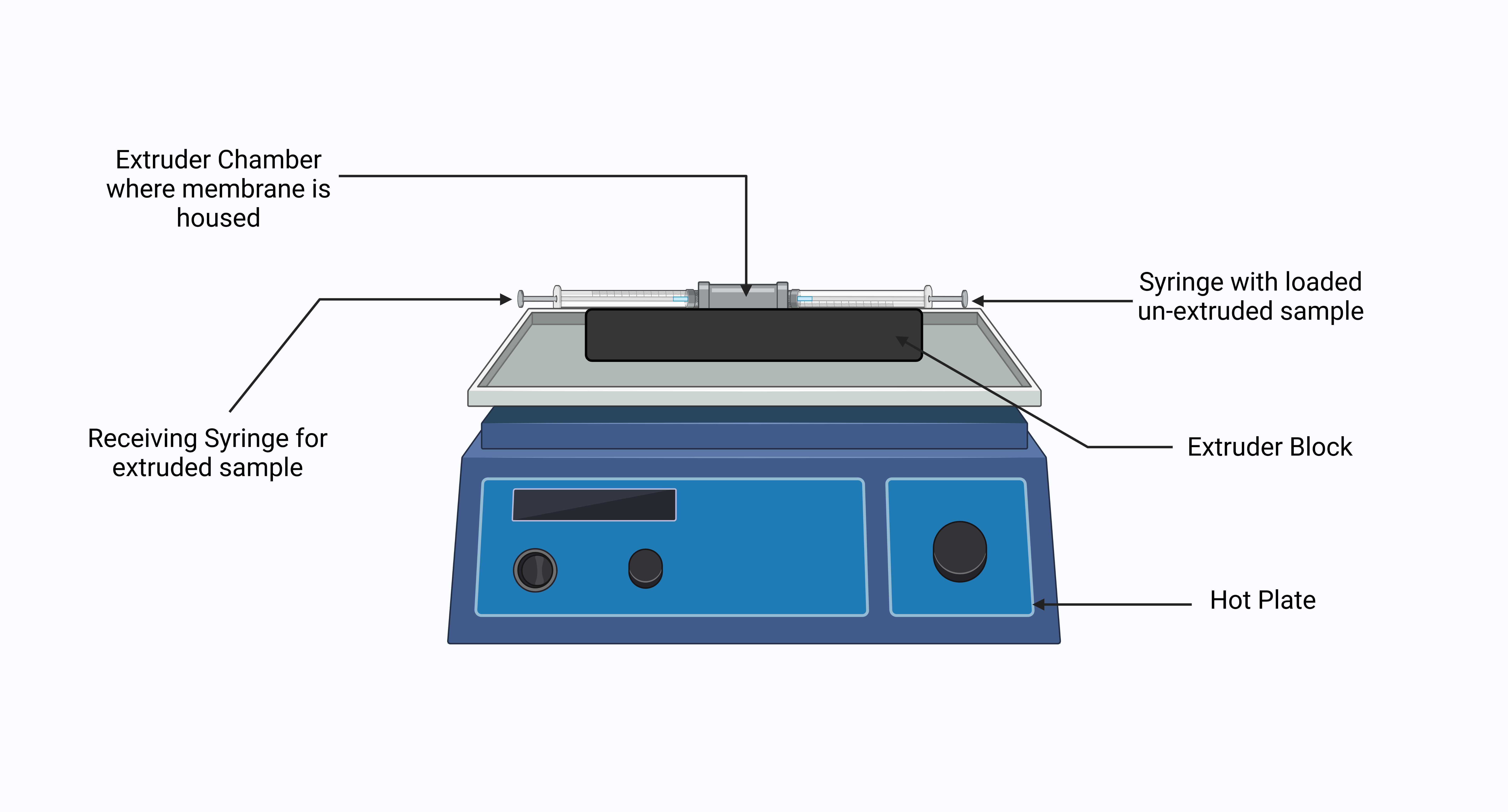
Place a 100nm polycarbonate membrane into the extruder chamber. For this protocol, we have used an Avanti mini extruder system (see steps on how to do this on the Avanti website: https://avantilipids.com/divisions/equipment-products/mini-extruder-assembly-instructions). https://avantilipids.com/divisions/equipment-products/mini-extruder-assembly-instructions). The system allows large vesicles to be reduced to unilamellar vesicles by extrusion through defined pore membrane sizes in an efficient manner. The protocol can be adapted for other mini extruder systems which should be used in accordance with the manufacturer’s guidelines.
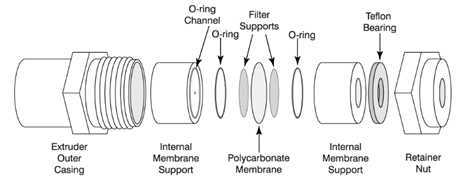
Once chamber is sealed closed, position the extruder chamber into the extruder block (See Figure 2 ).
With the syringe, draw up 1ml of the freshly vortexed liposome solution.
Position the syringes into the extruder block as seen in (See Figure 2 ) and passage the liposome solution through the 100nm polycarbonate membrane 7 times. Be sure that the syringes are fully inside the block.
Once the liposome solution has been brought down to 100nm, open the extruder chamber and replace the 100nm membrane with a 50nm polycarbonate membrane.
Rinse the system with ultrapure water prior to the 50nm extrusion. Once flushed, repeat the extrusion process.
Store extruded liposomes in 4°C to maintain liposome stability.
Characterization of Synthesized Liposomes
Liposome properties, such as particle size, poly dispersity index (PDI) and zeta potential (ZP) are important parameters to study when synthesising liposomes for drug delivery. Dynamic Light Scattering (DLS) is a commonly used equipment for determining the particle size, PDI and ZP of liposome solutions. Studying the morphology of synthesised liposomes is also a helpful characterization step and be done using Scanning electron microscope (SEM).
DLS - Determines the average particle size of the liposomes and the polydispersity of the vesicle size.
Zeta Potential – Determines the average surface charge on the liposome vesicles.
Scanning electron microscope (SEM) – Used to observe the liposomes morphology.
HPLC – Used to analyse the separation and quantification of the lipid components in the liposome formulations.
NMR - Can be used to investigate if the liposomes are unilamellar or multilamellar as they provide different NMR resolution.