Electron Microscopy of Cells Grown on Polyacrylamide Hydrogels
Frederik Helmprobst, Frederik Helmprobst, Anna Hupfer, Anna Hupfer, Matthias Lauth, Matthias Lauth, Axel Pagenstecher, Axel Pagenstecher
Abstract
The composition of the cell culture environment profoundly affects cultured cells. Standard cell culture equipment such as plastic and glass provide extremely stiff surfaces compared to physiological cell environments (i.e., tissue). A growing body of evidence documents the artificial behavior and morphology of cells cultured on supraphysiologically stiff surfaces, such as glass (elastic modulus ca. 70,000 MPA) or plastic (e.g., polystyrol ca. 3300 MPA). Therefore, polymer-based hydrogels are increasingly employed as more physiologically appropriate (<100 kPA) supports for 2D or 3D culture. Since multiple properties that influence the cultured cells may be easily adjusted, hydrogels have become versatile tools for studying cells in a more native in vitro environment. Polyacrylamide-based hydrogels can be used as culture substrates for a broad variety of adherent cells and are easy to handle in most downstream biological assays, such as immunohistochemistry or molecular biology methods. We faced, however, serious difficulties with processing high stiffness polyacrylamide-based hydrogels for electron microscopy. To overcome this problem, we developed a simple protocol for embedding and processing cells grown on high stiffness polyacrylamide hydrogels that do not require modifications of routine embedding protocols. © 2022 The Authors. Current Protocols published by Wiley Periodicals LLC.
Basic Protocol : Embedding of polyacrylamide-based hydrogels for transmission electron microscopy
Alternate Protocol 1 : Procedure for detached hydrogels
Alternate Protocol 2 : Procedure for attached hydrogels
INTRODUCTION
The extracellular environment can significantly alter cellular behavior, function, and morphology via a broad variety of signals, including oxygen pressure, osmolarity, pH, and composition of extracellular matrix proteins or surface proteins of neighboring cells. Accordingly, in recent years the rigidity of cell culture substrates has been increasingly scrutinized for its effects on cultured cells (Blaschke et al., 2020; Janmey, Fletcher, & Reinhart-King, 2020; Mih et al., 2011; Pandamooz et al., 2020; Pelham & Wang, 1997; Wells, 2008). Together, these investigations demonstrated the profound effects of substrate rigidity on in vitro cell biology. Since most natural tissues are significantly less rigid than the commonly used tissue culture substrates made from glass or plastic, different biomaterials have been developed to resemble the physiological cell environment more closely (Caliari, 2016). One class of such biomaterial, hydrogels, is composed of swollen polymeric networks that mimic the extracellular protein matrix of soft tissue and support cell adhesion (Tibbitt & Anseth, 2009). Hydrogels have become versatile tools for studying cells in 2D or 3D cell culture systems because many relevant parameters such as the polymeric components, protein composition, or material stiffness may be independently fine-tuned (Caliari, 2016; Liu et al., 2020; Ruedinger, Lavrentieva, Blume, Pepelanova, & Scheper, 2015; Zhao, Xu, Zhu, Wang, & Chi, 2019). In addition, hydrogels are easy to handle in a variety of downstream applications, like live cell imaging (Sigaut, Bianchi, von Bilderling, & Pietrasanta, 2021) and other downstream analytical approaches such as immunohistochemistry and molecular biology assays (Caliari, 2016; Huerta-López & Alegre-Cebollada, 2021; Lachowski et al., 2021; Park, Huh, & Kang, 2021).
Despite the expanding application of hydrogels in cell biology, transmission electron microscopy (TEM) has rarely been employed in these investigations (Macrí-Pellizzeri et al., 2015). This fact is somewhat intriguing since TEM remains the morphological technique with the finest spatial resolution and is widely used to visualize fine structural features in cell biology (Ravi, Leung, & Zeev-Ben-Mordehai, 2020; Richert-Pöggeler, Franzke, Hipp, & Kleespies, 2019; Winey, Meehl, O'Toole, & Giddings, 2014).
In a recent study (Hupfer et al., 2021), we used polyacrylamide-based hydrogels with different stiffness factors (0.2 kPA and 50 kPA) on glass coverslips to analyze the ultrastructure of NIH3T3 cells. We correlated these to metabolic changes and morphological characteristics as observed by light microscopy. While the preparation of the low stiffness polyacrylamide hydrogels (0.2 kPA) posed no problems for electron microscopy, we observed extreme shrinkage and induration of stiff polyacrylamide hydrogels (30 kPA and 50 kPA) in the course of dehydration. Subsequently, the Epon compound, an epoxy resin used in electron microscopy embedding to obtain excellent preservation of the ultrastructural morphology of biological samples, did not infiltrate the high stiffness polyacrylamide gel. The dehydrated and Epon-embedded high stiffness gels initially were extremely hard and damaged the diamond knife used for sectioning. In addition, as soon as the sections touched the water bath behind the knife's edge, the polyacrylamide gel quickly reabsorbed water and swelled, softening so much that ultrathin sectioning became impossible. Consequently, ultrastructural analysis of cells grown on high stiffness gels was impossible. This behavior was observed with both self-made 30 kPA (Cretu, Castagnino, & Assoian, 2010) and commercially available 50 kPA SoftslipTM gels. Similar obstacles have been described earlier for hydroxyethyl methacrylate-based hydrogels (Toselli, Faris, Oliver, Wedel, & Franzblau, 1983). Here, we describe two easy protocols for preparing sections from cells grown on polyacrylamide hydrogels for light microscopy and ultrastructural investigation. Our protocols have the advantage of utilizing a routine embedding protocol for electron microscopy and avoiding the use of additional chemicals, as described for hydroxyethylmethacrylate-based gels (Toselli et al., 1983). Because of their simplicity, our protocols can be adopted in every electron microscopy facility. In addition, the method described might help solve similar problems when using high stiffness hydrogels based on different compounds.
CAUTION: All embedding steps must be run in a suitable fume hood with efficient ventilation. Many of the reactions in this article are highly toxic; safety glasses and reagent-impermeable protective gloves should be worn.
Basic Protocol: EMBEDDING OF POLYACRYLAMIDE-BASED HYDROGELS FOR TRANSMISSION ELECTRON MICROSCOPY
This protocol can be used to embed cells grown on polyacrylamide-based hydrogels, which show limited infiltration by hydrophobic compounds such as Epon. The basic protocol describes the fixation and dehydration steps common to both alternate protocols. In our experiments, NIH3T3 cells and primary astrocytes prepared from 1- to 2-day-old newborn mice were cultured in DMEM (Invitrogen) on coverslips coated with polyacrylamide-based hydrogels and cultured under appropriate conditions (Smith, Newton, Mikulski, & Rybak, 1999; Thome et al., 2020). We used self-prepared gels described by Cretu et al. (2010) or purchased commercially available gels (SoftslipTM, Cell guidance systems, Cambridge, Great Britain). Following chemical fixation (Helmprobst, Frank, & Stigloher, 2015), cells were processed for electron microscopy (Trusal, Baker, & Guzman, 1979).
Materials
-
Cell culture medium, for example, DMEM (Gibco, ThermoFisher Scientific, Darmstadt, Germany; cat. no. 10566016)
-
Polyacrylamide-coated commercially available SoftslipTM (Matrigen Petrisoft/Softslip, Cell Guidance Systems, Cambridge, United Kingdom) or self-made polyacrylamide coated coverslips (Cretu et al., 2010)
-
Glutaraldehyde 25% (SERVA, Heidelberg, Germany, cat. no. 23114.01)
-
Sörensenbuffer (SB, see recipe)
-
Osmiumtetroxide (Chempur, Karlsruhe, Germany, cat. no. 6051) (see recipe)
-
Uranylacetate-dihydrate (Merck, Darmstadt, Germany, cat. no. 1.08473.0025)
-
Ethanol (Sigma-Aldrich, Darmstadt, cat. no. 32205)
-
Propylene oxide (SERVA, Heidelberg, Germany, cat. no. 33715)
-
Epon (SERVA, Heidelberg, Germany, cat. no. 21045)
-
Cell culture dishes (Greiner Bio-One GmbH, Frickenhausen, Germany; cat. no. 664160)
-
24-well plates (Greiner Bio-One GmbH, Frickenhausen, Germany; cat. no. 657160)
-
Silicon embedding chambers for electron microscopy (Plano GmbH, Wetzlar, Germany, cat. no. G369N)
-
Micro-graters 25 mm C-Pilot files (Pluradent, Munich, Germany, cat. no.: 45068) and Hedstroem files 25 mm (Pluradent, Munich, Germany, cat. no. 42969)
-
Wooden toothpicks
-
Incubator
-
Diamond knife (DIATOME, Schwitzerland)
-
Ultramicrotom (Ultracut E, Reichert-Jung)
Seed and fix your cells on polyacrylamide-based hydrogels
1.Seed your cells of interest on the hydrogel of choice and incubate them under appropriate conditions. It is beneficial to grow the cells to the maximum cell density desired (confluence) to increase the number of cells in the preparation. If, however, a specific experiment requires a lower cell density, the preparation can be performed with fewer cells.
2.Upon reaching the desired confluence, fix the cells with 2.5% glutaraldehyde in Sörensenbuffer (SB, see recipe) for at least an hour at 4°C.
3.Transfer the coverslips into a 24-well plate and wash the samples five times with 1 ml SB for 3 min each.
4.Postfix the cells with 2% osmiumtetroxide (OsO4) in SB for 90 min.
5.Wash 5× for 3 min with 1 ml ddH2O.
6.Prestain the samples overnight with 2% uranylacetate diluted in ddH2O.
7.Wash 5× for 3 min with 1 ml ddH2O.
8.Dehydrate the samples in ascending graded alcohol (30%, 50%, 70%, and 90%) into 100% EtOH with 1 ml at 4°C for 30 min per step on ice.
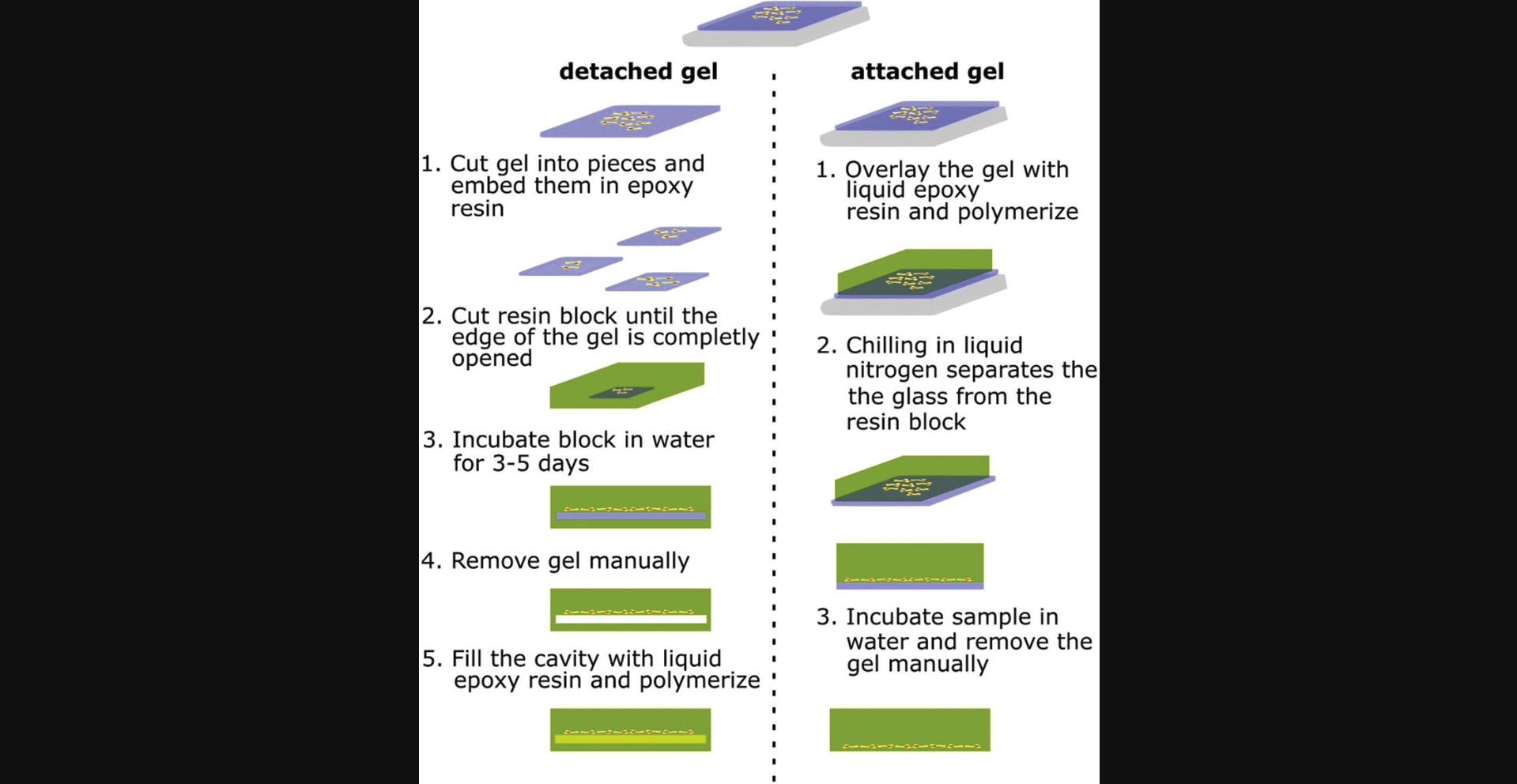
9.Transfer the samples to room temperature and wash twice with 100% EtOH.
Alternate Protocol 1: PROCEDURE FOR DETACHED HYDROGELS
This protocol describes the embedding of detached hydrogels in Epon and the steps necessary to prepare sections of high stiffness hydrogels.
Materials
- See Basic Protocol
1.Cut the detached hydrogels into pieces, transfer them to a glass vessel, and wash 3 × 30 min with 1 ml propylene oxide.
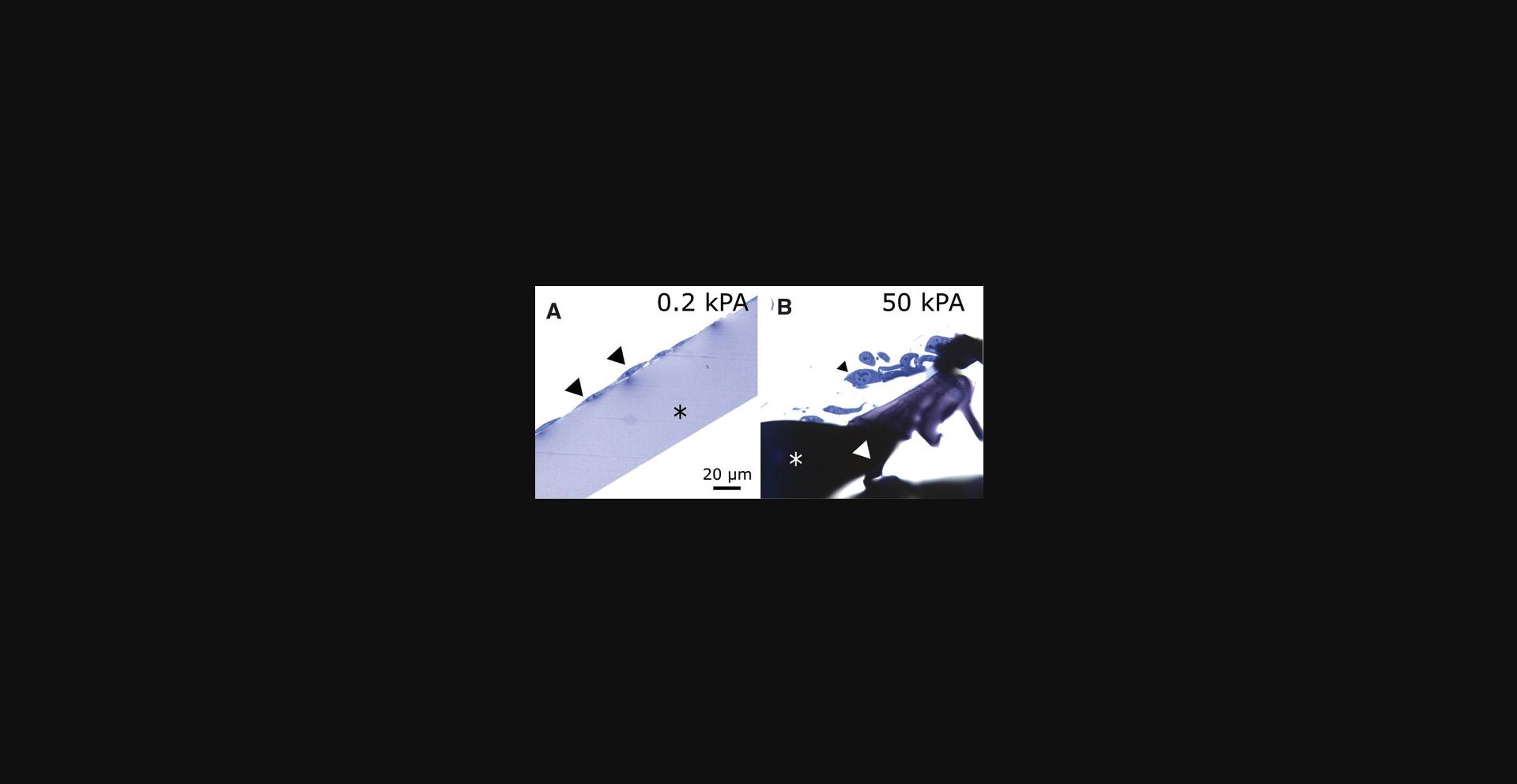
2.Incubate the samples overnight in 1 ml of a 1:1 Epon:propylene oxide solution.
3.Exchange the solution with 500 µl Epon and wash twice with 500 µl Epon for at least 2 hr each.
4.Transfer the samples to flat silicon embedding chambers for electron microscopy and cover the samples with Epon.
5.Let the Epon polymerize at 70°C for 48 hr.
6.Open the Epon blocks with a razor blade until the gel is reached (Fig. 3A).
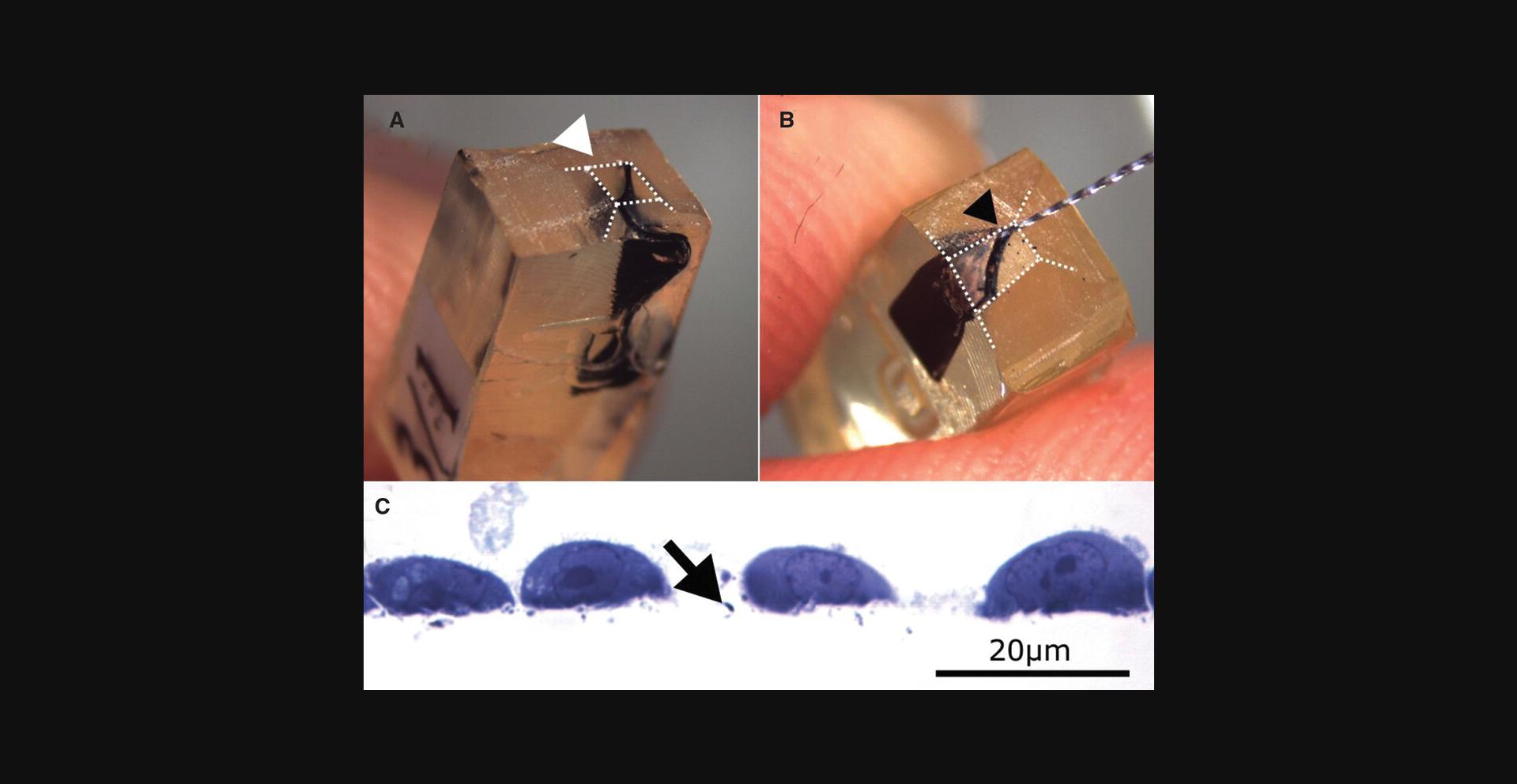
7.Incubate the opened block in water for 3 to 5 days to let the gel swell.
8.Remove the gel carefully with a 25-mm Hedstroem and C-Pilot files micro-grater or toothpick (Fig. 3B).
9.Refill the cavity with fresh Epon and let polymerize for 48 hr at 70°C.
10.Prepare 60-nm ultrathin sections and follow standard staining protocols for imaging samples in the electron microscope, as described (Reynolds, 1963) (Fig. 3C).
Alternate Protocol 2: PROCEDURE FOR ATTACHED HYDROGELS
Low and high stiffness hydrogels still attached to the glass coverslips are handled similarly after dehydration. The samples were embedded as described elsewhere (Trusal et al., 1979).
Materials
- See Basic Protocol
1.Transfer the glass slides to an appropriate glass vessel with the cells on top and wash 3 × 30 min with propylene oxide.
2.Incubate the samples overnight in a 1:1 Epon:propylene oxide solution.
3.Exchange the solution with Epon and wash twice with Epon for at least 2 hr each.
4.Transfer the glass slide with the cell layer facing downwards on top of an Epon-filled flat silicon embedding chamber and let polymerize for 48 hr and 70°C.
5.Remove the sample from the silicon embedding chamber and remove the glass slide by freezing the Epon block in liquid nitrogen.
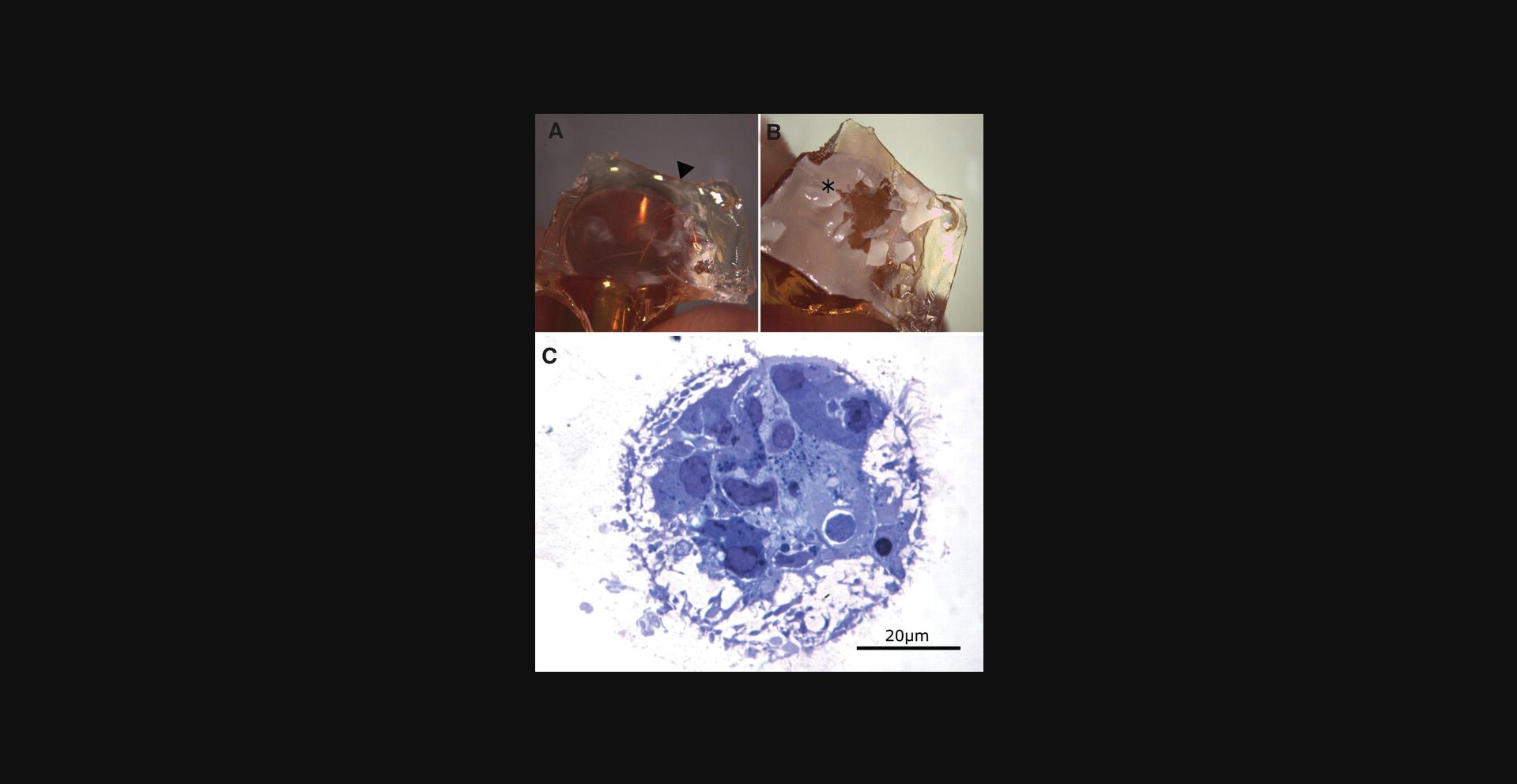
6.Incubate in water (we found 10 min sufficient to swell and soften the gel, then remove the high stiffness hydrogel with a wooden toothpick (Fig. 4B).
7.Prepare ultrathin sections and follow standard staining protocols for imaging samples in the electron microscope, as described previously (Reynolds, 1963).
REAGENTS AND SOLUTIONS
Use ddH2O for all preparations. Use appropriate precautions (fume hood, personal protective equipment, and glass containers) when handling toxic reagents.
Sörensenbuffer
SB buffer should be fresh when used. For SB preparation, titrate solution A with solution B until a pH of 7.45 is reached. The SB can be stored for up to two weeks at 4°C.
Solution A
- 17.8 g NaHPO4 × 2H2O
- 1000 ml ddH2O
- Store at 4°C for up to 6 months.
Solution B
- 4.08 g KH2PO4
- 300 ml ddH2O
- Store at 4°C for up to 6 months.
4% Stock Osmium tetroxide (OsO4) solution
- A stock solution of 4% OsO4 in water is prepared under a fume hood as described, with minor modification (Cold Spring Harbor Protocols, 2010).
It is crucial to wear goggles and nitrile gloves, as OsO4 is highly toxic and skin contact must be avoided. Used OsO4 solution is collected in a glass bottle, neutralized with ascorbic acid or vegetable oil, and disposed of appropriately.
- Clean the inner side of a brown wide-necked glass bottle with a ground-glass stopper and the outside of the glass vial containing the OsO4 with acetone and afterward with 100% ethanol to remove traces of lipids.
- Place the bottle into a styrofoam bucket and fill 25 ml of distilled water into the glass bottle.
- Submerge the glass vial containing 1 g of OsO4 in the water and break the glass vial with a metal rod.
- Incubate at 4°C until the OsO4 is completely dissolved.
- Aliquot in 1 ml portions and enwrap with parafilm.
- Freeze aliquots and store in a sealed glass vessel at −20°C.
COMMENTARY
Background Information
In a recent study, we used polyacrylamide-based hydrogels of different stiffness factors on glass coverslips to study metabolic changes and ultrastructural morphological characteristics of NIH3T3 cells (Hupfer et al., 2021). Using established standard protocols for chemical fixation and Epon embedding of cells and tissues, we could not investigate cells grown on high stiffness gels because the gel became extremely hard and brittle and destroyed the diamond knife used for ultrathin sectioning. Surprisingly, very few publications discuss embedding polyacrylamide-based hydrogels for electron microscopy (Macrí-Pellizzeri et al., 2015). One group used hydroxyethyl methacrylate-based hydrogels and developed a protocol in which additional chemicals and reagents were used (Toselli et al., 1983). We developed these easy-to-follow protocols to prepare low and high stiffness hydrogels with materials comparable to routine embedding methods. Our protocols might also work for other hydrophilic high stiffness hydrogels that are impermeable by Epon after dehydration.
Critical Parameters and Troubleshooting
The protocols describe a simple and easy method for embedding low and high stiffness hydrogels. Nevertheless, there are some critical parameters to consider. During all steps, it is necessary to change all solutions very carefully because some types of cells might be washed away from the gel surface. In addition, the dehydration steps must be performed carefully to avoid drying artifacts.
When removing the rehydrated high stiffness hydrogel with micro graters (Fig. 3B), it is extremely important to be very gentle and not remove hard Epon parts. If the high stiffness hydrogel remains hard, additional incubation in water should be performed. Rehydrated hydrogel is soft and can easily be removed without exertion. Otherwise, when Epon is removed, artifacts and damaged cells are later visible, which can lead to a misinterpretation of results.
Understanding Results
After staining, prepared ultrathin sections (Reynolds, 1963) can be imaged with an electron microscope. The low stiffness hydrogel is still visible in the electron microscope (Fig. 5A), while the high stiffness gel is missing having been completely removed (Fig. 5B). When the hydrogel was removed with appropriate prudence, the cell membrane facing the hydrogel remained intact (Fig. 5C).
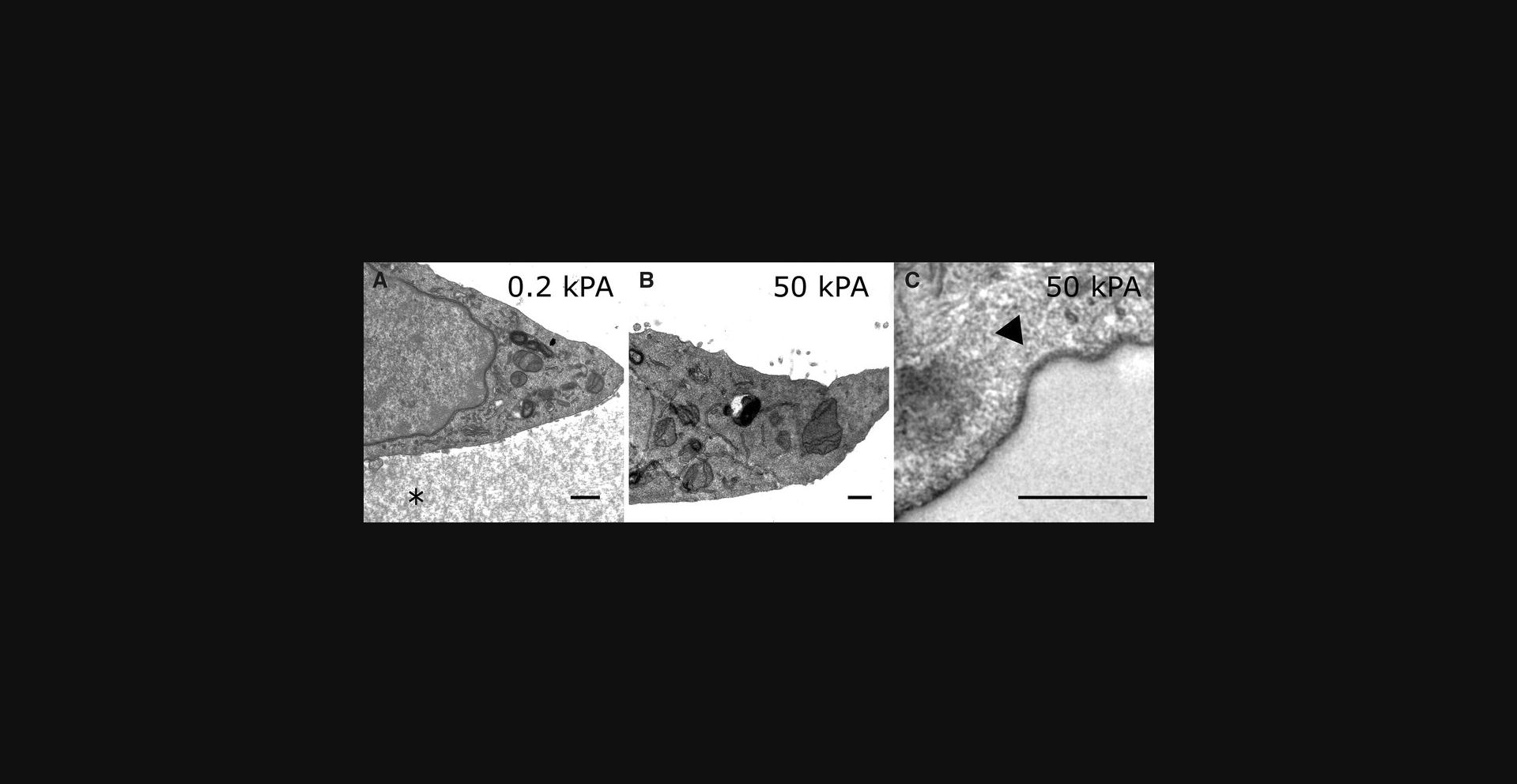
Time Considerations
The time to consider depends strikingly on the behavior of the hydrogels during the dehydration step and the stiffness of the gel. While low and attached high stiffness hydrogels can be embedded within 5 days, at least 8 days are needed for detached high stiffness gels. The latter can be split up into 3 days for fixation and infiltration, 2 days for Epon polymerization, 1 day to swell and remove the hydrogel, and 2 additional days for the polymerization of refilled Epon. The time to consider for each of the steps mentioned in the protocol can be found in Table S1. Please note that certain steps imply considerable hands-on time; thus, the total for these steps depends on the number of samples processed in parallel.
Acknowledgments
We are very thankful to Silvia Stanek and Ginette Bortolussi for their technical support.
Open access funding enabled and organized by Projekt DEAL.
Author Contributions
Frederik Helmprobst : Conceptualization, methodology, data curation, original draft preparation, visualization, and project administration; Anna Hupfer : Draft review and editing; Matthias Lauth : Draft review and editing, supervision, and project administration; Axel Pagenstecher : Conceptualization, resources, draft review and editing, supervision, and project administration. All authors have read and agreed to the published version of the manuscript.
Conflict of Interest
The authors have no conflict of interest to declare.
Open Research
Data Availability Statement
Data sharing is not applicable to this article as no new data were created or analyzed in this study. All generated data are included in the previously published paper:
Hupfer, A., Brichkina, A., Koeniger, A., Keber, C., Denkert, C., Pfefferle, P., … Lauth, M. (2021). Matrix stiffness drives stromal autophagy and promotes formation of a protumorigenic niche. Proceedings of the National Academy of Sciences of the United States of America , 118 , e2105367118, doi:10.1073/ pnas.2105367118.
Supporting Information
Filename | Description |
---|---|
cpz1524-sup-0001-SupMat.docx13.1 KB | Supporting Information |
Please note: The publisher is not responsible for the content or functionality of any supporting information supplied by the authors. Any queries (other than missing content) should be directed to the corresponding author for the article.
Literature Cited
- Blaschke, S. J., Demir, S., König, A., Abraham, J.-A., Vay, S. U., Rabenstein, M., … Rueger, M. A. (2020). Substrate elasticity exerts functional effects on primary microglia. Frontiers in Cellular Neuroscience , 14, 590500. doi: 10.3389/fncel.2020.590500
- Caliari. (2016). Review: Hydrogels. Ecologia Austral , 26, 72–82.
- Cold Spring Harbor Protocols. (2010). Osmium tetroxide stock solution (2%). doi: 10.1101/pdb.rec12107.
- Cretu, A., Castagnino, P., & Assoian, R. (2010). Studying the effects of matrix stiffness on cellular function using acrylamide-based hydrogels. Journal of Visualized Experiments , 42, 2089. doi: 10.3791/2089
- Helmprobst, F., Frank, M., & Stigloher, C. (2015). Presynaptic architecture of the larval zebrafish neuromuscular junction. Journal of Comparative Neurology , 523, 1984–1997. doi: 10.1002/cne.23775
- Huerta-López, C., & Alegre-Cebollada, J. (2021). Protein hydrogels: The Swiss army knife for enhanced mechanical and bioactive properties of biomaterials. Nanomaterials (Basel) , 11(7), 1656. doi: 10.3390/nano11071656
- Hupfer, A., Brichkina, A., Koeniger, A., Keber, C., Denkert, C., Pfefferle, P., … Lauth, M. (2021). Matrix stiffness drives stromal autophagy and promotes formation of a protumorigenic niche. Proceedings of the National Academy of Sciences , 118, e2105367118. doi: 10.1073/pnas.2105367118
- Janmey, P. A., Fletcher, D. A., & Reinhart-King, C. A. (2020). Stiffness sensing by cells. Physiological Reviews , 100, 695–724. doi: 10.1152/physrev.00013.2019
- Lachowski, D., Matellan, C., Cortes, E., Saiani, A., Miller, A. F., & Del Río Hernández, A. E. (2021). Self-assembling polypeptide hydrogels as a platform to recapitulate the tumor microenvironment. Cancers , 13, 3286. doi: 10.3390/cancers13133286
- Liu, H., Li, M., Liu, S., Jia, P., Guo, X., Feng, S., … Xu, F. (2020). Spatially modulated stiffness on hydrogels for soft and stretchable integrated electronics. Materials Horizons , 7, 203–213. doi: 10.1039/C9MH01211G
- Macrí-Pellizzeri, L., Sancho, A., Iglesias-García, O., Simón-Yarza, A. M., Soriano-Navarro, M., González-Granero, S., … Prosper, F. (2015). Substrate stiffness and composition specifically direct differentiation of induced pluripotent stem cells. Tissue Engineering - Part A , 21, 1633–1641. doi: 10.1089/ten.tea.2014.0251
- Mih, J. D., Sharif, A. S., Liu, F., Marinkovic, A., Symer, M. M., & Tschumperlin, D. J. (2011). A multiwell platform for studying stiffness-dependent cell biology. PLoS One , 6, e19929. doi: 10.1371/journal.pone.0019929
- Pandamooz, S., Jafari, A., Salehi, M. S., Jurek, B., Ahmadiani, A., Safari, A., … Dargahi, L. (2020). Substrate stiffness affects the morphology and gene expression of epidermal neural crest stem cells in a short-term culture. Biotechnology and Bioengineering , 117, 305–317. doi: 10.1002/bit.27208
- Park, Y., Huh, K. M., & Kang, S. W. (2021). Applications of biomaterials in 3d cell culture and contributions of 3d cell culture to drug development and basic biomedical research. International Journal of Molecular Sciences , 22(5), 2491. doi: 10.3390/ijms22052491
- Pelham, R. J., & Wang, Y. L. (1997). Cell locomotion and focal adhesions are regulated by substrate flexibility. Proceedings of the National Academy of Sciences of the United States of America , 94, 13661–13665. doi: 10.1073/pnas.94.25.13661
- Ravi, R. T., Leung, M. R., & Zeev-Ben-Mordehai, T. (2020). Looking back and looking forward: Contributions of electron microscopy to the structural cell biology of gametes and fertilization. Open Biology , 10, 200186. doi: 10.1098/rsob.200186
- Reynolds, E. S. (1963). The use of lead citrate stain at high pH in electron microscopy. Journal of Cell Biology , 17, 208. doi: 10.1083/jcb.17.1.208
- Richert-Pöggeler, K. R., Franzke, K., Hipp, K., & Kleespies, R. G. (2019). Electron microscopy methods for virus diagnosis and high resolution analysis of viruses. Frontiers in Microbiology , 0, 3255. doi: 10.3389/fmicb.2018.03255
- Ruedinger, F., Lavrentieva, A., Blume, C., Pepelanova, I., & Scheper, T. (2015). Hydrogels for 3D mammalian cell culture: A starting guide for laboratory practice. Applied Microbiology and Biotechnology , 99, 623–636. doi: 10.1007/s00253-014-6253-y
- Sigaut, L., Bianchi, M., von Bilderling, C., & Pietrasanta, L. I. (2021). Correlation of cellular traction forces and dissociation kinetics of adhesive protein zyxin revealed by multi-parametric live cell microscopy. PLoS One , 16, e0251411. doi: 10.1371/journal.pone.0251411
- Smith, M. R., Newton, D. L., Mikulski, S. M., & Rybak, S. M. (1999). Cell cycle-related differences in susceptibility of NIH/3T3 cells to ribonucleases. Experimental Cell Research , 247, 220–232. doi: 10.1006/excr.1998.4317
- Thome, I., Lacle, R., Voß, A., Bortolussi, G., Pantazis, G., Schmidt, A., … Pagenstecher, A. (2020). Neoplastic cells are the major source of MT-MMPs in IDH1-mutant glioma, thus enhancing tumor-cell intrinsic brain infiltration. Cancers , 12, 2456. doi: 10.3390/cancers12092456
- Tibbitt, M. W., & Anseth, K. S. (2009). Hydrogels as extracellular matrix mimics for 3D cell culture. Biotechnology and Bioengineering , 103, 655–663. doi: 10.1002/bit.22361
- Toselli, P., Faris, B., Oliver, P., Wedel, N., & Franzblau, C. (1983). Preservation of ultrastructure of cells cultured on protein—hydroxyethylmethacrylate hydrogels. Journal of Ultrastructure Research , 83, 220–231. doi: 10.1016/S0022-5320(83)90079-5
- Trusal, L. R., Baker, C. J., & Guzman, A. W. (1979). Transmission and scanning electron microscopy of cell monolayers grown on polymethylpentene coverslips. Biotechnic and Histochemistry , 54, 77–83. doi: 10.3109/10520297909112638
- Wells, R. G. (2008). The role of matrix stiffness in regulating cell behavior. Hepatology (Baltimore, Md.) , 47, 1394–1400. doi: 10.1002/hep.22193
- Winey, M., Meehl, J. B., O'Toole, E. T., & Giddings, T. H. Jr. (2014). Conventional transmission electron microscopy. Molecular Biology of the Cell , 25, 319. doi: 10.1091/mbc.e12-12-0863
- Zhao, H., Xu, K., Zhu, P., Wang, C., & Chi, Q. (2019). Smart hydrogels with high tunability of stiffness as a biomimetic cell carrier. Cell Biology International , 43, 84–97. doi: 10.1002/cbin.11091