Identification and Quantification of Monosaccharides from Fungal Cell Walls and Exopolysaccharides by Gas Chromatography Coupled to Mass Spectrometry
Pierre-Guy Millette, Pierre-Guy Millette, Josée Chabot, Josée Chabot, Donald C. Sheppard, Donald C. Sheppard, François Le Mauff, François Le Mauff
Abstract
The fungal cell wall and secreted exopolysaccharides play an important role in the interactions between fungi and their environment. Despite their central role in fungal biology, ecology, and host-pathogen interactions, the composition of these polymers and their synthetic pathways are not well understood. The protocols presented in this article describe an approach to isolate fungal cell wall polysaccharides and to identify and quantify the monosaccharide composition of these polymers by gas chromatography-mass spectrometry (GC-MS). © 2023 The Authors. Current Protocols published by Wiley Periodicals LLC.
Basic Protocol : O-methyl trimethylsilyl monosaccharide derivatives composition analysis by GC-MS
Support Protocol : Fungal cell wall extraction
INTRODUCTION
Fungal cell walls are dynamic structures that play an important role at the interphase of organisms and their environment (Gow et al., 2017). They are required for cell morphology and integrity, govern macromolecule exchange, mediate adhesion to substrates, modulate host immune responses, and protect fungi from environmental and antimicrobial threats (Latgé, 2020). Polysaccharides are a major component of fungal cell walls and cell wall-associated structures like biofilm matrix (Gow & Lenardon, 2022). Despite their critical role in growth and pathogenesis, our current understanding of the structure and synthesis of fungal cell wall polysaccharides remains limited. The polysaccharide composition of cell walls of important fungal pathogens such as Rhizopus oryzae , the main etiological agent of mucormycosis, or Batrachochytrium dendrobatidis , responsible for the mass extinction of amphibians, remain largely uncharacterized (Lecointe et al., 2019). Even defining the composition of the cell walls and biofilms of the top two medically relevant fungi, Candida albicans and Aspergillus fumigatus , remain areas of ongoing research (Le Mauff & Sheppard, 2023).
A key step in expanding our understanding of the role of fungal polysaccharides in cell wall functions is to define their composition. Chromatography techniques are the most complete analytical tools developed for this purpose. They offer a full characterization of the monosaccharide content as well as their substitution and require only a few milligrams of material. However, glycan chromatography requires unique expertise that is often not available in all institutions or fee for service analytical platforms. The protocols presented in this article describe the extraction of cell wall polysaccharides and the analysis of their raw composition by gas chromatography coupled to mass spectrometry (GC-MS). The process transforms fungal biomass into a fungal cell wall preparation by physical crushing and organic solvent washes. Extracted polymers are then hydrolyzed into monosaccharides, which are derivatized into O-methyl-trimethylsilyl (TMS) derivatives. Based on the difference in boiling point of each TMS derivative, their separation is then allowed using a temperature gradient established in the gas chromatograph. Comparison of the chromatogram generated from these preparations to calibration curves generated with monosaccharide standards can then be used to identify and quantify the monosaccharide composition of the cell wall preparation.
This technique constitutes the first step in identifying and characterizing the polysaccharides that make up the fungal cell wall. Other complementary approaches to characterize cell wall polysaccharides include the determination of the monosaccharide linkages by analysis of partially methylated alditol acetate derivatives by GC-MS or analysis of partially hydrolyzed polysaccharides by mass spectrometry. Both techniques can complement the information provided by the monosaccharide composition and contribute to the elucidation of the different cell wall polysaccharide structures.
CAUTION : All chemical reactions must be run in a suitable fume hood with efficient ventilation and adequate personal protective equipment. Many of the reactions in this article are either highly exothermic or performed at high temperature; therefore, safety glasses, reagent-impermeable protective gloves and thermal protective gloves should be worn.
CAUTION : Manipulation of live fungal organism should be performed using appropriate biosafety level precautions. Follow all appropriate guidelines and regulations for the use and handling of pathogenic microorganisms.
Basic Protocol: O-METHYL TRIMETHYLSILYL MONOSACCHARIDE DERIVATIVES COMPOSITION ANALYSIS BY GC-MS
Materials
-
D-(+)-chiro-inositol (MilliporeSigma 74137 or equivalent)
-
ddH2O
-
L-(+)-arabinose (MilliporeSigma A3256 or equivalent)
-
L-rhamnose (MilliporeSigma W373011 or equivalent)
-
L-(-)-fucose (MilliporeSigma F2252 or equivalent)
-
D-(+)-xylose (Bioshop XYL001 or equivalent)
-
D-(+)-mannose (Bioshop MAN510 or equivalent)
-
D-(+)-galactose (Bioshop GAL500 or equivalent)
-
D-(+)-glucose (MilliporeSigma G8270 or equivalent)
-
D-glucuronic acid (MilliporeSigma G5269 or equivalent)
-
N -acetylglucosamine (Bioshop ACG604 or equivalent)
-
N -acetylgalactosamine (Bioshop AGL888 or equivalent)
-
Fungal cell wall or exopolysaccharides (see Support Protocol)
-
Hydrochloric acid (HCl) (FisherScientific 351280 or equivalent)
-
Trifluoroacetic acid (TFA) (MilliporeSigma T6508 or equivalent)
-
3 M hydrogen chloride in methanol solution (MilliporeSigma 90964 or equivalent)
-
Methanol (FisherScientific AC176840010 or equivalent)
-
Anhydrous pyridine (MilliporeSigma 270970 or equivalent)
-
Acetic anhydride (MilliporeSigma 320102 or equivalent)
-
TriSil-HTP (FisherSceintific TS-48999 or equivalent)
-
Cyclohexane (FisherScientific AC279590010 or equivalent)
-
Helium, 99.999% purity (Vitalaire SG230402 or equivalent)
-
Single channel pipettes (20-, 200- and 1000-µl)
-
Sure-stop vials (ThermoFisher C5000-592W or equivalent)
-
Sample concentrator (Bibby SBHCONC/1 or equivalent)
-
PTFE-coated needles (ColeParmer OF-36620-99 or equivalent)
-
Spatula
-
Analytical precision balance (Precisa ES225SM-DR or equivalent)
-
2 ovens (ThermoScientific HeraTherm Oven 51028121 or equivalent)
-
Autosampler (Agilent Technologies 7650A or equivalent)
-
Wash vials (VWR CA76514-766 or equivalent)
-
GC-MS (Agilent Technologies GC7890B and MS Ensemble 5977B)
-
Capillary column CP-Sil-5CB (Agilent Technologies CP7441 or equivalent)
-
Agilent Extraction Source XtrEI350 (Agilent Technologies G3870-67720 or equivalent)
-
Agilent GC-MS enhanced Masshunter software (version B.07.06 build 7.06.0.2704 or equivalent)
-
Agilent Masshunter Qualitative Analysis Navigator (version B.08.00 build 8.0.8208.38 or equivalent)
-
Agilent Masshunter Quantitative Analysis (version B.09.00 build 9.0.647.0 or equivalent)
-
10-µL fixed needle ALS syringe, 23-26s/42/cone, PTFE-tip plunger (Agilent Technologies 5181-3354 or equivalent)
Preparation of the internal standard
1.Make a solution of 10 mM D-(+)-chiro-inositol in ddH2O.
Preparation of the calibration sets
2.Make a solution of each calibration monosaccharide (arabinose, rhamnose, fucose, xylose, mannose, galactose, glucose, glucuronic acid, N -acetylgalactosamine and N -acetylgalactosamine) at 100 mM in ddH2O.
3.Mix all the calibration monosaccharide solutions together to obtain a calibrant mix at 10 mM.
4.Prepare two calibration sets by pipetting 10 µl, 50 µl, 100 µl, 250 µl and 500 µl calibrant mix in sure-stop vials.
5.Add 10 µl of 10 mM D-(+)-chiro-inositol to all sure-stop vials.
6.Evaporate the two calibration sets using the sample concentrator equipped with one PTFE-coated needle per sample.
Preparation of the cell wall samples
7.Add ∼0.5 mg of fungal cell wall sample to each of 2 sure-stop vials using a spatula and an analytical precision balance.
8.Add 10 µl of 10 mM D-(+)-chiro-inositol to all sure-stop vials.
Hydrolysis
9.Preheat oven 1 to 100°C.
10.Preheat oven 2 to 110°C.
11.Add 250 µl of 6 M HCl to one of your sample vials and to one calibration set.
12.Cap the vials.
13.Incubate at 100°C for 4 hr.
14.Add 250 µl of 2 M TFA to the remaining sample vial and calibration set.
15.Cap the vials.
16.Incubate at 110°C for 2 hr.
17.Evaporate the acid completely using the sample concentrator.
Methanolysis
18.Dilute the 3 M hydrogen chloride solution to 1 M with methanol.
19.Add at least 100 µl of the diluted hydrogen chloride solution to all sure-stop vials.
20.Incubate overnight at 80°C in one of the ovens previously used.
Re-N-acetylation of amino sugars
21.Evaporate the hydrogen chloride solution using the sample concentrator.
22.Add 500 µl methanol to all sure-stop vials.
23.Evaporate the methanol using the sample concentrator.
24.Repeat steps 22 and 23.
25.Prepare a solution of methanol:pyridine (5:1).
26.Add 600 µl of the methanol/pyridine solution to each vial.
27.Add 150 µl acetic anhydride to each vial, cap the vials and quickly swirl to mix.
28.Incubate for 1 hr at room temperature.
Persilylation
29.Preheat oven to 110°C.
30.Evaporate the re-N -acetylation mix with the sample concentrator.
31.Wash twice all sure-stop vials with 500 µl methanol.
32.Add at least 50 µl of TriSil-HTP reagent to each sure-stop vial.
33.Incubate at 110°C for 20 min.
Sample preparation for injection into the GC-MS
34.Dry down the Tri-Sil HTP mix with the sample concentrator.
35.Wash twice all sure-stop vials with 500 µl cyclohexane.
36.Reconstitute all sure-stop vials with 1 ml cyclohexane.
37.Place all the sure-stop vials in the autosampler.
Preparation of the GC-MS
For monosaccharide analysis the gas chromatograph uses helium as a carrier gas and the Agilent CP-Sil-5CB capillary column as stationary phase. The mass spectrometer is equipped with the extraction source XtrEI350. All GC-MS parameters for settings and analysis are controlled by the MassHunter software suite.
38.Prepare a blank by adding 1 ml cyclohexane to a sure-stop vial.
39.Fill 2 wash vials with cyclohexane for the injector needle to clean pre and post sampling.
40.Tune the mass spectrometer using the EI extraction tune method furnished by the machine supplier.
41.Implement your samples information into the autosampler interface.
42.Implement the gas chromatography parameters with the following gradient:
-
Hold 120°C for 2 min.
-
120°C to 160°C at 15°C/min.
-
160°C to 195°C at 1.5°C/min.
-
195°C to 280°C at 30°C/min.
-
Hold 280°C for 1.5 min.
43.Implement MS acquisition parameters as follows:
-
Start acquisition at t = 6 min.
-
Scan fromm/z50 to 400 at a frequency of 4.1 scans/s.
-
Single ion monitoring (SIM) of 3 ions under a cycle of 8.33 Hz: m/z 204 from t = 6 min to 18.5 min, m/z 305 from t = 18.5 min to 21 min, and m/z 173 from t = 21 min to 30 min.
Injection
44.Inject 1 µl of each sample and calibrant using the ALS syringe, inserting a blank injection between each sample injection.
Support Protocol: CELL WALL PREPARATION
This Support Protocol presents the different steps required to extract the fungal cell wall from fungal biomass. This protocol can be applied to any fungal specie and in any required experimental settings. As cell walls are dynamic components of the cell, any change in the growth conditions and time of extraction can impact the composition obtained. Therefore, no specific instruction regarding the culture is given.
Additional Materials (also see Basic Protocol)
-
Absolute ethanol (Greenfield global Inc. P016EAAN equivalent)
-
Fungal biomass
-
Chloroform (FisherScientific C606-4 or equivalent)
-
Acetone (FisherScientific A18-4 or equivalent)
-
Water bath (Montreal Biotech WBE05A11B or equivalent)
-
Miracloth (MilliporeSigma 475855-1R or equivalent)
-
50-ml conical tubes (VWR 82050-346 or equivalent)
-
Freeze drier (Labconco)
-
Biosafety cabinet
-
Tenbroeck tissue grinder (FisherScientific 06-421-40 or equivalent)
-
Table-top centrifuge (ThermoScientific Sorvall Legend RT 75004377 or equivalent)
-
Vortex
-
Nutator
-
Fume hood
1.Set the water bath to 70°C.
2.Prepare a solution of 70% ethanol.
3.Filter the biomass out of its culture medium on Miracloth.
4.Squeeze biomass in the Miracloth to remove as much culture supernatant as possible.
5.Transfer biomass to a 50-ml conical centrifuge tube.
6.Add 40 ml of 70% ethanol to fungal biomass to wash out the culture supernatant.
7.Shake vigorously.
8.Discard the ethanol supernatant.
9.Place the biomass in the freeze drier at −20°C to freeze.
10.Freeze dry the biomass.
11.Reconstitute biomass into 70% ethanol and transfer some to the tissue grinder.
12.Crush fungal biomass until only very fine particles remain.
13.Incubate the crushed biomass suspended in 70% ethanol in the water bath for 15 min.
14.Centrifuge 5 min at 2200 × g , room temperature.
15.Discard the ethanol supernatant.
16.Resuspend the pellet in 40 ml fresh 70% ethanol solution.
17.Repeat steps 13 to 16 twice.
18.Resuspend the pellet of the last ethanol wash in 40 ml of methanol:chloroform (1:1).
19.Incubate on a nutator (or shaking platform) overnight.
20.Centrifuge 5 min at 2200 × g , room temperature.
21.Discard the methanol:chloroform supernatant.
22.Resuspend the pellet in 40 ml acetone.
23.Incubate on a nutator (or shaking platform) for at least 2 hr.
24.Centrifuge 5 min at 2200 × g , room temperature.
25.Discard the acetone supernatant.
26.Dry the pellet overnight by leaving the conical tube opened in a fume hood.
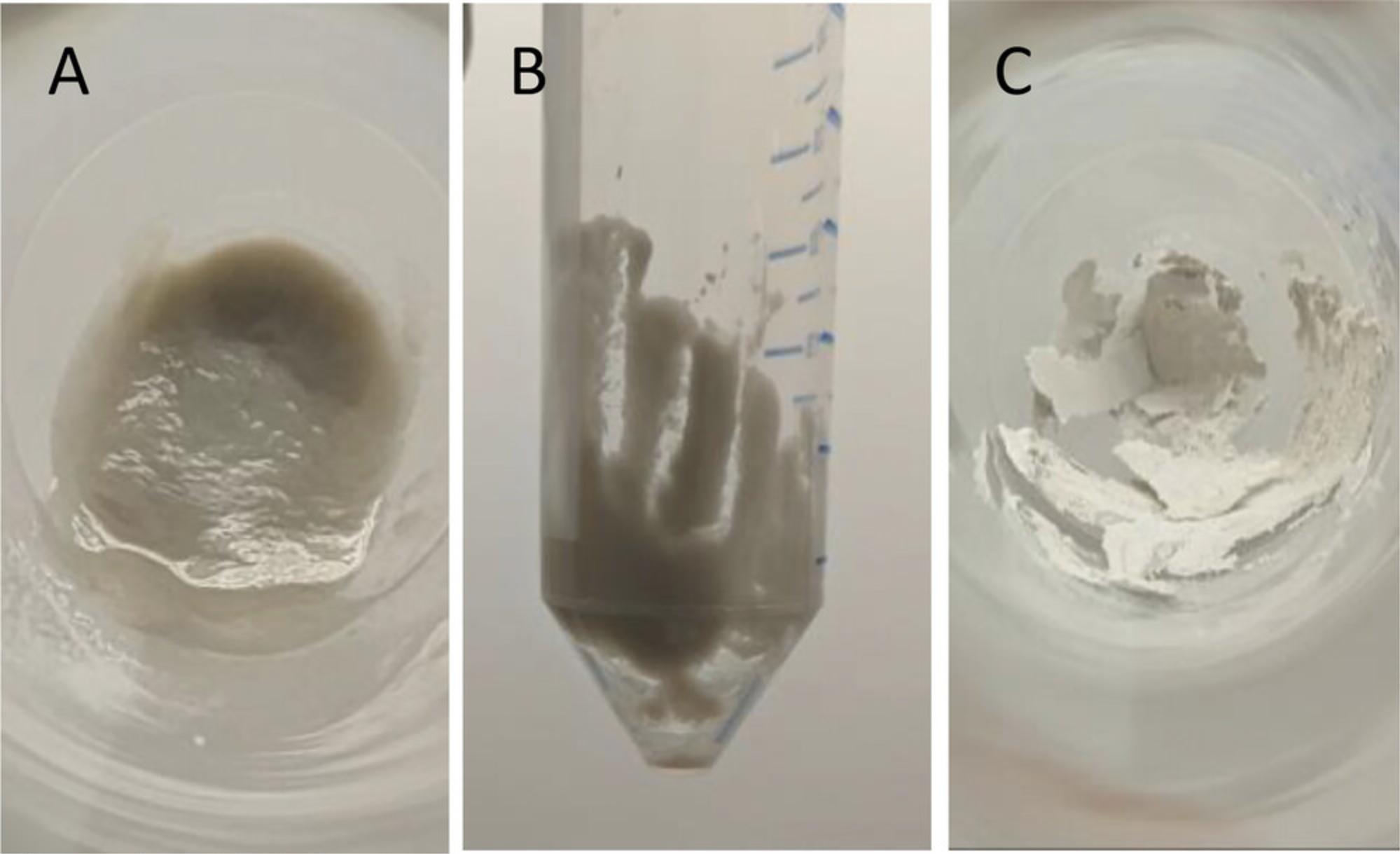
27.Break down the dry pellet by vigorously shaking the conical tube.
COMMENTARY
Background Information
Gas chromatography is the gold standard technology for the detection and quantification of monosaccharides (Amicucci et al., 2019). In contrast with liquid chromatography, which relies on monosaccharide polarity for separation and requires a fluorescent UV tag for detection, gas chromatography relies on the boiling point of monosaccharide derivatives. Although the derivatization of monosaccharides is required for GC analysis, GC-MS analysis of TMS derivatives permits greater chromatographic resolution and more reliable quantification. Each TMS monosaccharide produces at least 2 quantifiable peaks corresponding to the two most abundant tautomers. These tautomer peaks will always respect the same proportions corresponding to their natural abundance. In case of coelution between different monosaccharides, it is then possible to orient the quantification towards a tautomer with a greater chromatographic resolution. Alditol acetate derivatization is another common approach; however, it is less informative as only one peak can be observed per monosaccharide.
Detection of monosaccharide derivatives is most commonly performed using one of two main technologies, flame ionization detection (FID) and MS. Of these, MS is a more complete, versatile, and sensitive detector. Unlike FID, which only measures the ionization of the monosaccharides while they burn through the flame, MS provides fragmentation spectra that can be used for further confirmation of monosaccharide identity, as well as to study monosaccharide linkage analysis using partially methylated alditol acetate (PMAA) derivatives.
Although GC-MS is the gold standard technique for the determination of monosaccharide composition of polysaccharides, it should be acknowledged that this technique requires access to appropriate equipment, and that there is a significant cost to the ongoing operation and maintenance of such equipment.
Critical Parameters
Internal standard. Monosaccharide quantification is performed using normalization to an internal standard concentration; therefore, it is important to be as accurate as possible when preparing this solution and dispensing it into each vial.
Methanolysis. This step is highly sensitive to the presence of salt. Cell wall extracts prepared as per the given protocol should minimize this issue. However, it is important to consider this point when preparing other fungal polysaccharides for analysis as traces of salts from extraction or purification need to be removed prior to this step.
Drying steps. The sample concentrator allows the rapid elimination of the acids and organic solvents; however, it is important to keep the airflow to a minimum as when the samples are dried out only powder remains, which is easily aerosolizable. The risk for sample loss or cross contamination is high.
Troubleshooting
A troubleshooting guide for common problems is presented in Table 1.
Problem | Possible cause | Solution |
---|---|---|
No signal or low signal | Mass spectrometer is not working | Run a MS tune program to ensure that the mass spectrometer is properly working |
Derivatization failed | If no inositol is present on the chromatogram, the reaction failed; restart the experiment paying attention to the critical parameters cited above | |
If inositol is present, consider starting with more material or injecting a more concentrated TMS derivative solution | ||
Blank is not blank | Sample carryover | Dilute your sample to decrease the amount injected |
Column contamination | Run a column cleanup according to manufacturer protocol |
Understanding Results
The analysis of the results is performed in two steps. The identification of the monosaccharide present in the sample needs to be carried out first. It is important to compare the chromatograms of the samples obtained in scan and single ion monitoring (SIM) mode to that of the calibrating standards (Fig. 2). This approach will allow the detection of any potential difference in retention times that could lead to misidentification, difference in the ratio between the different monosaccharide tautomers that could lead to inaccurate quantification, or presence of monosaccharides not accounted for in the general standard list provided. In any of these three situations, the analysis of the mass spectra provided for each chromatographic peak will allow accurate identification and quantification as well as identification of potential unexpected chromatographic peaks.
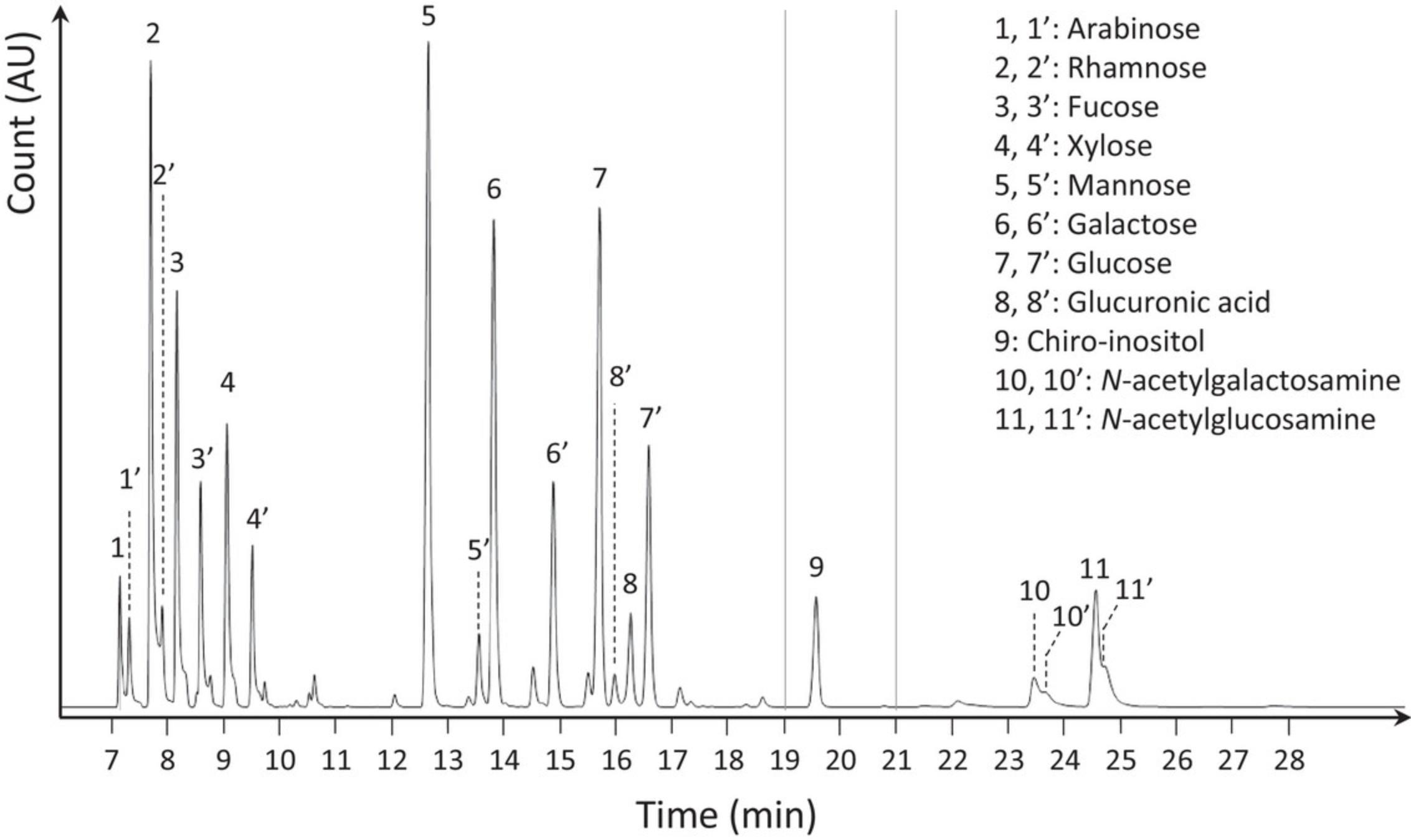
Once the monosaccharides are correctly identified, quantification can be performed. Quantification occurs in three phases. First, experimental signals need to be normalized to the signal of the internal standard in order to correct for any difference in the efficacy of the chemistry. Secondly, normalized monosaccharide signals can be compared to the calibration curves obtained with the injection of the calibration monosaccharides. Finally, once the amount of each monosaccharide present in each sample is determined, this can be normalized to the amount of biomass in each sample. This step will allow for combining the data obtained for the sample hydrolyzed with the trifluoroacetic acid providing information on hexose and pentose-based polymers, and the one hydrolyzed with the hydrochloric acid providing information on hexosamine based polymers.
Data are often expressed as a fraction of total polysaccharides or sample amount. However, as for all relative quantification methods it is important to remain aware these findings can be misleading; if one monosaccharide decreases, by default all the others will increase and vice versa. It is therefore preferable to present data using absolute quantification.
Time Considerations
The identification of the monosaccharide content of cell wall preparations is a process requiring two days of intermittent work due to the multiple steps requiring solvent evaporation. Once the TMS derivatives are prepared, they will only be stable for a few days. In the case of a large analytical set, an immediate injection is recommended as each analysis will take ∼45 min.
Acknowledgments
This work is supported by CFI-MSI GlycoNET Integrated Services, MI4 and CIHR grant FDN159902.
Author Contributions
Pierre-Guy Millette : Methodology; Josée Chabot : Project administration, Resources, Writing review and editing; Donald C. Sheppard : Funding acquisition, Writing review and editing; François Le Mauff : Conceptualization, Methodology, Writing original draft, Writing review and editing.
Conflict of Interest
The authors declare no conflict of interest.
Open Research
Data Availability Statement
Data are available upon request by email.
Literature Cited
- Amicucci, M. J., Galermo, A. G., Nandita, E., Vo, T. T. T., Liu, Y., Lee, M., Xu, G., & Lebrilla, C. B. (2019). A rapid-throughput adaptable method for determining the monosaccharide composition of polysaccharides. International Journal of Mass Spectrometry , 438, 22–28. https://doi.org/10.1016/j.ijms.2018.12.009
- Gow, N. A. R., Latge, J. P., & Munro, C. A. (2017). The fungal cell wall: Structure, biosynthesis, and function. Microbiology Spectrum , 5(3), https://doi.org/10.1128/microbiolspec.funk-0035–2016
- Gow, N. A. R., & Lenardon, M. D. (2023). Architecture of the dynamic fungal cell wall. Nature Reviews Microbiology , 21, 248–259. https://doi.org/10.1038/s41579-022-00796-9
- Latgé, J.-P. (2020). The fungal cell wall: An armour and a weapon for human fungal pathogens (Vol. 425). Springer Nature.
- le Mauff, F., & Sheppard, D. C. (2023). Understanding Aspergillus fumigatus galactosaminogalactan biosynthesis: A few questions remain. The Cell Surface , 9, 100095. https://doi.org/10.1016/j.tcsw.2023.100095
- Lecointe, K., Cornu, M., Leroy, J., Coulon, P., & Sendid, B. (2019). Polysaccharides cell wall architecture of mucorales. Frontiers in Microbiology , 10, 469. https://doi.org/10.3389/fmicb.2019.00469