High-Throughput Gene Replacement in Aspergillus fumigatus
Can Zhao, Can Zhao, Marcin G. Fraczek, Marcin G. Fraczek, Lauren Dineen, Lauren Dineen, Ressa Lebedinec, Ressa Lebedinec, Juliane Macheleidt, Juliane Macheleidt, Thorsten Heinekamp, Thorsten Heinekamp, Daniela Delneri, Daniela Delneri, Paul Bowyer, Paul Bowyer, Axel A. Brakhage, Axel A. Brakhage, Michael Bromley, Michael Bromley
Abstract
Aspergillus fumigatus is a human pathogen and the principal etiologic agent of invasive and chronic aspergillosis leading to several hundreds of thousands of deaths every year. Very few antifungals are available to treat infections caused by A. fumigatus , and resistance is developing to those we have. Our understanding of the molecular mechanisms that drive pathogenicity and drug resistance have been hampered by the lack of large mutant collections, which limits our ability to perform functional genomics analysis. Here we present a high-throughput gene knockout method that combines a highly reproducible fusion PCR method to enable generation of gene replacement cassettes with a multiwell format transformation procedure. This process can be used to generate 96 null mutants within 5 days by a single person at a cost of less than £18 ($24) per mutant and is being employed in our laboratory to generate a barcoded genome-wide knockout library in A. fumigatus. © 2019 The Authors.
INTRODUCTION
Aspergillus fumigatus is the primary etiologic agent of invasive aspergillosis, a disease that primarily affects individuals who are immunocompromised, and causes 200,000 life-threatening infections annually (Brown et al., 2012). A. fumigatus also causes chronic diseases in the ostensibly immunocompetent population which affects around 3 million individuals and leads to ∼400,000 deaths (http://www.gaffi.org/why/fungal-disease-frequency). About 20 million have lifelong conditions caused by an immune hyperactivity to A. fumigatus.
A deeper insight of the pathogen and host factors at the molecular level is vastly important for us to be able to overcome the burden of such diseases; however, our understanding is actually very limited. Genome-wide knockout libraries have been used to great effect to establish an in-depth understanding of microbial functional genomics.
In this protocol, we describe a high-throughput generic process for the generation of gene replacement cassettes by adapting the aforementioned PCR approach to incorporate common-fusion sequences that improve reproducibility of amplification. In addition, our cassette generation method allows for the inclusion of randomly generated barcodes that can support the use of competitive fitness profiling methods. We also describe how replacement cassettes can be used to generate null mutants in A. fumigatus in a multiwell format in a rapid and inexpensive way. The fusion PCR and transformation procedures are integrated here into a single protocol that allows one person to generate 96 knockout mutants in <5 days. This protocol was established to facilitate the generation of an A. fumigatus genome-wide knockout library, which is an ongoing project in our laboratory. With a few modifications, this method is also applicable to other filamentous fungal species, such as Aspergillus niger or Aspergillus nidulans.
Basic Protocol 1: PCR AMPLIFICATION OF GENE KNOCKOUT CASSETTES
A PCR-based fragment fusion approach, herein described as PCR fusion, has been described previously to facilitate gene knockout in the filamentous fungus A. nidulans (Szewczyk et al., 2006). In this protocol we describe an adaptation of the previously described method to permit high-throughput gene knockout.
For gene replacement in filamentous fungi, PCR fusion–based generation of gene knockout cassettes initially involves amplification of three separate DNA fragments (Fig. 1A): (1) an ∼1-kb fragment replicating the region immediately upstream of the gene that is to be replaced, (2) an ∼1-kb fragment immediately downstream of the gene that is to be replaced, and (3) a fragment that incorporates a selectable marker cassette and a randomly generated genetic barcode. These three fragments are subsequently fused (Fig. 1B). This fusion is possible as the primers used to amplify the selectable marker incorporate sequences that are complementary to sequences present within the primers used to amplify each of the flanks. To enhance the specificity of the fusion PCR, two nested primers are used that anneal at the extremities of the upstream and downstream flanks.
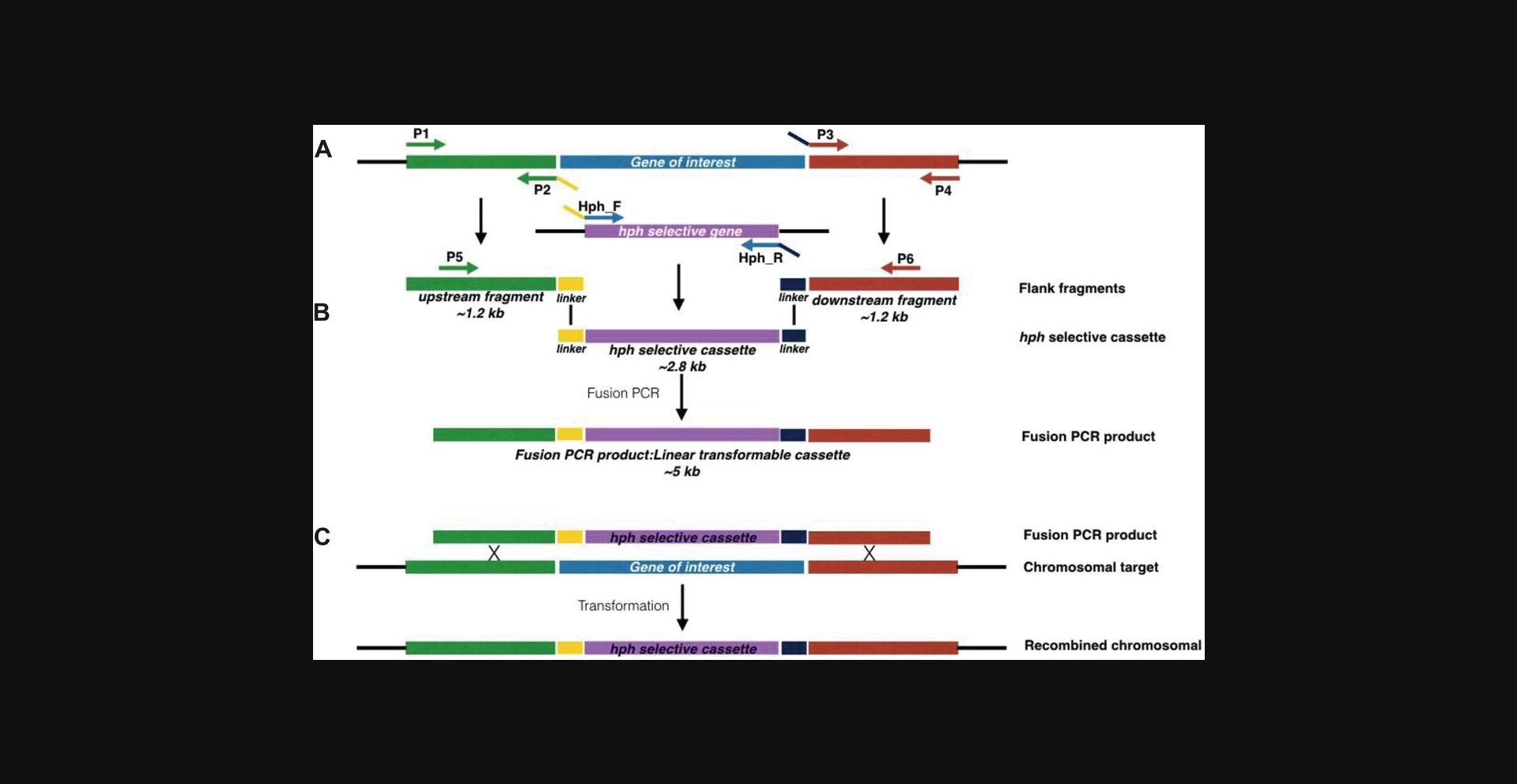
For the purposes of this protocol, the primers used to amplify these regions are designated P1 and P2 for amplification of the upstream fragment, P3 and P4 for amplification of the downstream fragment, and hph_F and hph_R for amplification of the selectable marker cassette (in this case the hygromycin B phosphotransferase gene [hph] driven by the A. nidulans gdpA promoter), with P5 and P6 being the designations for the nested primers. In each case, primers P1 to P6 will differ depending on the gene that is to be targeted. However, in order to enhance the reproducibility of the fusion PCR method, we have introduced common linker sequences at the flanks of the selectable marker cassette and on primers P2 and P3 (see yellow and navy blue linkers shown on hph_F and hph_R in Fig. 1A). As the linkers used for fusion PCR are common for all knockout cassettes, only one set of hph primers is required for hph cassette amplification. The use of this common linker sequence coupled with the high-throughput primer design and PCR amplification procedure are the key highlights of this protocol. Our combination of primer design principles and fusion PCR conditions will give nearly identical results on any modern thermal cycler.
Materials
-
100 µM oligonucleotide primers, high purity, salt-free (e.g., Eurofins; see Support Protocol)
-
Molecular biology–grade water, sterile-filtered, BioReagent, suitable for cell culture (e.g. Sigma, cat. no., W3500)
-
Genomic DNA from the isotype of the host to be transformed (e.g., A. fumigatus strain MFIG000)
-
LongAmp Taq DNA polymerase (New England Biolabs, cat. no. M0323)
-
5× MyTaq reaction buffer (Bioline, cat. no. BIO-37111)
-
QIAquick 96 PCR Purification Kit (Qiagen, cat. no. 28183)
-
Plasmid pAN7-1
-
QIAquick Gel Extraction Kit (Qiagen, cat. no. 28704)
-
96-well cell culture plates, flat bottom with low-evaporation lid, polystyrene (e.g., Costar, cat. no. 3595)
-
96-well PCR plate, semi-skirted with straight edges (e.g., Starlab, cat. no. I1402-9800)
-
Mulitchannel micropipettes and pipetting reservoir
-
Aluminum seal (e.g., StarSeal Sealing Tape; Starlab, cat. no. 201906MA)
-
15-ml conical tubes (e.g., Fisher Scientific, cat. no. 11849650)
-
Thermal cycler with ramp ability for 96-well plates (e.g., Eppendorf Mastercycler nexus)
-
Additional reagents and equipment for agarose gel electrophoresis (see Current Protocols article: Voytas, 2000)
Prepare oligonucleotide primers for PCR amplification
1.Prepare 96-well plates with oligonucleotide primers needed for the subsequent PCR amplifications (see the Support Protocol for primer design).
2.To prepare a primer-premix for amplification of 96 upstream fragments, pipette 180 μl sterile water to each well of a new 96-well plate using a multichannel pipette.
3.Pipette 10 μl from each well of the stock P1 primer plate of interest to the 180 μl water, and mix by pipetting up and down.
4.Pipette 10 μl from each well of the stock P2 primer plate of interest to the 190 μl water/P1 primer mixture to bring to a total of 200 μl. Mix by pipetting up and down.
5.To prepare a primer-premix for amplification of 96 downstream fragments, repeat step 2 through 4 using stock P3 and P4 primer plates. If not proceeding immediately to step 7, seal the 96-well plates containing primer working solutions with aluminum seals.
6.To prepare a primer-premix for the nested primers, repeat step 2 through 4 using stock P5 and P6 primer plates of interest to make the working solution of primers P5 and P6 for the fusion PCR. If not proceeding immediately to step 16, seal the 96-well plates containing primer working solutions with aluminum seals.
Amplify upstream and downstream fragments
7.Prepare a master mix solution for subsequent amplification of upstream and downstream fragments, as outlined below. Keep all ingredients on ice while setting up. Mix master mix in an ice-cold 15-ml conical tube, and keep tube on ice until pipetting into the PCR plate. For pipetting, pour master mix into a reservoir to allow access of multichannel pipette.
- Per reaction (23 µl total) :
- 1 µl of 10 to 40 ng/µl A. fumigatus genomic DNA
- 1 µl of 2.5 U/µl LongAmp DNA polymerase
- 5 µl MyTaq reaction buffer
- 16 µl molecular biology–grade water
- Per 2 × 96 reactions (+5% for pipetting error; 4646 µl total) :
- 202 µl of 10 to 40 ng/µl A. fumigatus genomic DNA
- 202 µl of 2.5 U/µl LongAmp DNA polymerase
- 1010 µl MyTaq reaction buffer
- 3232 µl molecular biology–grade water
8.Set up two separate 96-well plates for PCR, one for each flanking genomic fragment (25 µl total per reaction):
- 23 µl master mix (from step 7)
- 2 µl of 5 µM primers (P1 and P2 or P3 and P4; 5 µM)
9.Amplify fragments using the following PCR parameters:
1 cycle | 2 min | 95°C | (initial denature) |
35 cycles | 30 s | 95°C | (denature) |
30 s | 55°C | (anneal) | |
90 s | 68°C | (extend) | |
1 cycle | 5 min | 68°C | (final extend) |
10.Check products by agarose gel electrophoresis by loading 2 µl of each PCR product on a 1% agarose gel.
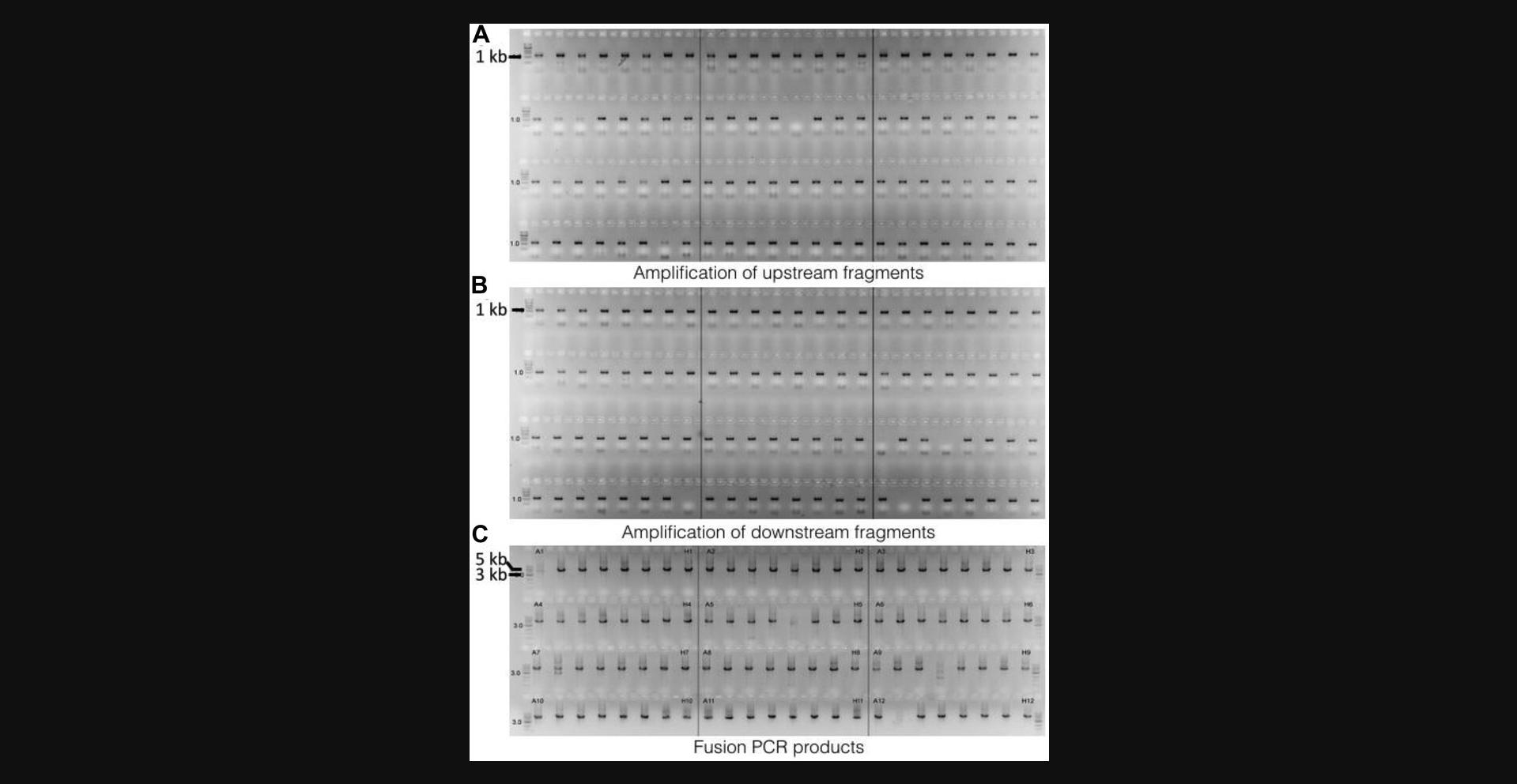
Clean PCR fragments and assess quality
11.Pool products of the upstream and downstream amplification reactions from corresponding wells, and purify using the Qiagen QIAquick 96 PCR Purification Kit. Elute purified products in a new 96-well plate.
12.Check purified products by agarose gel electrophoresis.
PCR amplify selective hph marker cassette
13.Set up PCR for amplification of the selective hph marker cassette (50 µl total per reaction):
- 2 µl of 5 µM hph_F (5 µM)
- 2 µl of 5 µM hph_R (5 µM)
- 2 µl plasmid pAN7-1 (50 ng)
- 1 µl of 2.5 U/µl LongAmp DNA polymerase (1 U/µl)
- 10 µl MyTaq reaction buffer
- 33 µl molecular biology–grade water
- hph_F:
- CCGGCTCGGTAACAGAACTA NNNNNNNNNNGCCNNNNNNNNNNCAGAACGGCGTAACCAAAAGTCAC
- hph_R:
- GTTGGAGCATATCGTTCAGAGC NNNNNNNNNNTAGNNNNNNNNNNTTCATCTTGACGACCGTTGATCTG
14.Amplify hph cassettes using the PCR parameters outlined in step 9.
15.Purify the amplified hph cassette using a Qiagen QIAquick Gel Extraction Kit. Check purified product on an agarose gel.
Perform fusion of upstream, downstream, and hph cassette fragments
16.Set up master mix solution for subsequent fusion of upstream, downstream, and hph cassette fragments:
- Per reaction (23 µl total) :
- 2 µl hph selective cassette
- 1 µl of 2.5 U/µl LongAmp DNA polymerase (2.5 U/µl)
- 5 µl MyTaq reaction buffer
- 15 µl molecular biology–grade water
- Per 96 reactions (+5% for pipetting error; 2323 µl total) :
- 202 µl hph selective cassette
- 101 µl of 2.5 U/µl LongAmp DNA polymerase (2.5 U/µl)
- 505 µl MyTaq reaction buffer
- 1515 µl molecular biology–grade water
17.Set up a fusion PCR using a 96-well PCR plate (25 µl total per reaction):
- 1 µl pooled upstream/downstream fragments
- 1 µl of 5 µM primers (P5 and P6; 5 µM)
- 23 µl master mix (from step 16)
18.Use the following PCR parameters to produce the linear transformable cassette:
1 cycle | 2 min | 95°C | (initial denature) |
35 cycles | 30 s | 95°C | (denature) |
maximum rate to | 70°C | (ramp) | |
1 s | 70°C | ||
0.1°C/s | to 55°C | ||
30 s | 55°C | (anneal) | |
0.2°C/s | to 68°C | (ramp) | |
3.5 min | 68°C | (extend) | |
1 cycle | 5 min | 68°C | (final extend) |
19.Check fusion PCR products by agarose gel electrophoresis.
20.Aliquot final fusion PCR products into two 96-well plates at 10 μl/well, and seal with aluminum seal.
Support Protocol: PRIMER DESIGN
Typically, eight primers are required to generate the linear transformable cassette for the knockout: two to amplify the 5′ flank, two to amplify the 3′ flank, two to amplify the selectable marker, and two nested primers to amplify the final product. We have optimized this procedure by introducing generic linkers; therefore, the same primer set is used for amplification of a generic hph selectable marker. Because of this, only six primers (P1 to P6) are needed for each linear transformable cassette (Fig. 1A). Primers are designed based on the general rules published by Innis and Gelfand (1990). P1, P5, P6, and P4 are 18 to 22 bp in length to have a melting temperature of ∼60°C, whereas P2 are P3 are longer due to the linker introduced.
In this protocol, we introduce a high-throughput pipeline using a novel R-script CONC@MATE together with BatchPrimer3 to achieve the rapid design of ∼60,000 primers required for the generation of the transformable cassettes.
Materials
- Computer with Internet access and the following programs:
- Aspergillus Genome Database (see Internet Resources)
- BioMart tool (see Internet Resources)
- BatchPrimer3 (see Internet Resources)
- R-Studio (see Internet Resources)
- CONC@MATE (see Internet Resources)
Design primers for upstream and downstream fragment amplifications
1.Identify the gene you wish to disrupt using the Aspergillus Genome Database (http://www.aspgd.org/).
2.Using the BioMart tool (https://fungi.ensembl.org/biomart/martview/), identify the Ensembl fungi dataset for Aspergillus fumigatus ; choose from the sequenced strains available.
3.Select “Filters,” and then select “GENE.” Then, input external references ID, or upload a file of gene IDs.
4.Select “Attributes,” “Sequences,” and then “Flank (Gene).”
5.Select the upstream flank option, and input 1200. Click the “Results” tab, and choose the option to export all results to a FASTA file.
6.Upload sequence files into BatchPrimer3 (https://probes.pw.usda.gov/batchprimer3/). Change the design settings for BatchPrimer3 as follows:
- Product size: range, 1100 to 1200
- Primer size: min, 18; opt, 20; max, 22
- Primer Tm: min, 58; opt, 60; max, 62
- Primer GC: min, 40; max, 60
7.Run BatchPrimer3.Ideally, pick primers that have 1 to 2 Gs or Cs in the last three bases (not always an option).
8.Name the primer marked left primer as xP1, where x represents the gene identity.
9.Attach the following linker to the 5′ end of the primer marked right primer to give primer xP2: TAGTTCTGTTACCGAGCCGG(X)18-22.
10.Select 1200 bp of the downstream flanking sequence, and repeat the primer design process (steps 6 and 7).
11.Name the primer marked right primer as xP4.
Design nested primers for fusion PCR
12.To design the nested primers used for fusion PCR, return to BioMart, and repeat the extraction process (steps 2 and 3). In attributes select the “Flank (Gene)” option, and input 1099 bp into the upstream and downstream option. Once again, extract the upstream and downstream flanking regions in succession.
13.Name the extracted BioMart files “upstream” and “downstream,” respectively.
14.Run the R script CONC@MATE in R-Studio using the upstream and downstream files extracted from BioMart.
15.Install the packages detailed at the start of the script by using the “Packages” tab on the R-studio interface. Click “install” and type the name of the required packages, making sure the “install from” tab has “Repository (CRAN)” selected.
16.Upload the concatenated FASTA file into BatchPrimer3, and select the same parameters as in step 6, except for the product size range, which should be 2000 to 2198 bp. Name the left primer xP5, and name the right xP6.
17.Order the primers from any qualified supplier.
Basic Protocol 2: GENERATION OF KNOCKOUTS
The host strain for our transformation procedure is MFIG000 (formally known as A1160 Δku80 pyrG+ ; Fraczek et al., 2013), an A. fumigatus strain derived from the well-characterized clinical isolate A1163 that lacks nonhomologous end joining (Δku80) and hence facilitates homologous recombination. In this protocol, we describe a high-throughput method of preparing and performing multiple transformations for generation of null mutants in A. fumigatus (Fig. 3). We suggest preparing fresh protoplasts for each transformation, as we noticed that overnight storage of protoplasts reduces transformation efficiency. Production of protoplasts can be easily scaled up to provide enough material for the desired number of transformations, and wherever possible, preparation of transformation mixture is performed in 96-well format allowing rapid processing. For the plating step, we use 6-well cell culture plates instead of the traditional 90-mm petri dishes. Using 6-well cell culture plates reduces the amount of labor involved in experimental preparation, as well as reduces use of culture medium and hygromycin B.
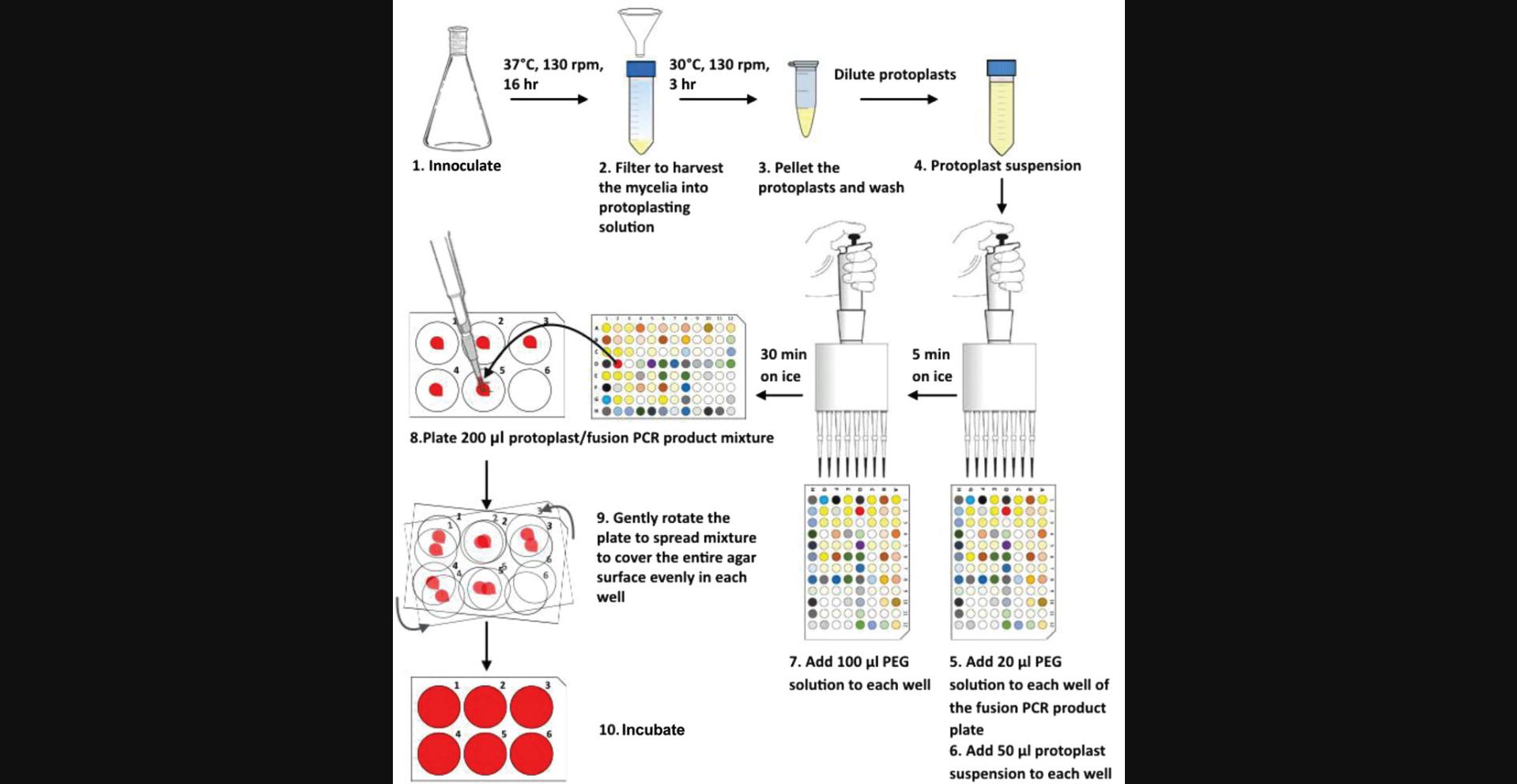
Materials
-
A. fumigatus strain MFIG000 spores (no older than 1 month)
-
Sabouraud (SAB) liquid medium (see recipe)
-
Protoplasting solution (see recipe)
-
0.6 M KCl, 50 mM CaCl2 solution (KCl/CaCl2; see recipe)
-
96-well plate containing 10 µl/well fusion PCR products (see Basic Protocol 1)
-
Polyethylene glycol (PEG) solution (see recipe)
-
6-well YPS/hygromycin B selection plates (see recipe)
-
250-ml flask, sterile
-
37°C shaking incubator
-
Foam plug for flask (50 × 38 mm; e.g., King Scientific, cat. no. FS5038)
-
Aluminum foil
-
Funnel, sterile
-
Miracloth, sterile (e.g., Millipore Sigma, cat. no. 475855)
-
Inoculating loop, sterile
-
50-ml conical tubes (e.g., Fisher Scientific, cat. no. 11819650)
-
Centrifuge (e.g., Sanyo Mistral 3000i) and microcentrifuge
-
1.5-ml microcentrifuge tubes
-
Hemocytometer (e.g., Fuchs-Rosenthal, Marienfeld-Superior)
-
Multichannel pipette
Produce transformable protoplasts
1.Inoculate 150 μl of 1 × 109 spores/ml A. fumigatus spores (MFIG000) into 50 ml SAB liquid medium in a sterile 250-ml flask, and incubate at 37°C with shaking (130 rpm) for 16 hr. Plug the top of the flask with foam, and then loosely cover with aluminum foil to allow ventilation and prevent contamination.
2.Remove flasks from the incubator, and harvest mycelia by filtration through sterile funnels with sterile Miracloth attached. Use a sterile inoculating loop to remove mycelia from the Miracloth, and transfer into a 50-ml conical tube containing 32 ml protoplasting solution.
3.Incubate tube at 30°C for 3 hr with gentle shaking (130 rpm).
4.Remove tube from shaking incubator, and for each tube, pour solution into a new tube through sterile funnels with sterile Miracloth attached to remove any residual undigested hyphal material.
5.Centrifuge tube containing protoplasts 10 min at 1800 × g , 4°C.
6.Remove tube from centrifuge. Remove supernatant and resuspend protoplasts in 2 ml KCl/CaCl2.
7.Transfer protoplast suspension from each tube into a 1.5-ml microcentrifuge tube. Centrifuge 3 min at 900 × g , 4°C. Remove supernatant and fully resuspend pellet in 1 ml ice-cold KCl/CaCl2. Repeat two additional times, and then resuspend pellet in 0.5 ml KCl/CaCl2.
8.Combine the two suspensions in one microcentrifuge tube.
9.Pellet protoplasts by centrifuging 3 min at 1800 × g , 4°C.
10.Resuspend each pellet in 1 ml KCl/CaCl2, which should give enough protoplasts for one 96-well plate worth of transformation. Make a 1000× dilution of protoplasting suspension by taking 1 μl from the suspension and mixing with 999 μl KCl/CaCl2. Count protoplasts using a hemocytometer.
11.Make a final dilution of protoplasting solution appropriate for subsequent transformation, and keep dilution on ice.
Transform
12.Defrost the 96-well plate containing 10 μl fusion PCR product per well from Basic Protocol 1.Add 20 μl room temperature PEG solution to each well using a multichannel pipette, and mix well.
13.Add 50 μl protoplast suspension from step 11 to each well, and mix by gently pipetting up and down. Incubate on ice for 30 min.
14.Add 100 μl room temperature PEG solution to each well using a multichannel pipette and mix. Incubate for 5 min on ice.
15.Using a 1000-μl micropipette, plate 200 μl protoplast/fusion PCR product mixture from each well on to the corresponding wells of the 6-well YPS/hygromycin B plates. Once plating is complete, gently rotate the 6-well selection plates to ensure the mixture is well spread and covers the entire agar surface evenly in each well.
16.Initially, incubate plates at room temperature in a sealed box for 24 hr.
17.Tape plates in stacks, and incubate at 37°C for 3 to 5 days.
18.Validate transformants, typically by streaking on selective medium.
REAGENTS AND SOLUTIONS
Hygromycin B stock, 200 mg/ml
- 1 g hygromycin B (e.g., Apollo Scientific)
- 5 ml deionized distilled water
- Store at 4°C for up to 1 month
KCl (0.6 M), CaCl2 (50 mM) solution (KCl/CaCl2)
- 4.47 g KCl
- 0.74 g CaCl2·2H2O
- 100 ml deionized distilled water
- Sterilize by autoclaving
- Store at room temperature indefinitely
KCl/citric acid solution
- To ∼50 ml deionized distilled water add:
- 8.2 g KCl
- 2.1 g citric acid monohydrate
- Adjust pH to 5.8 with 1.1 M KOH
- Bring volume to 100 ml with deionized distilled water
- Store at 4°C for up to 2 weeks
PEG solution
Add 400 g PEG 4000 (average molecular weight 4000) to ∼800 ml KCl/CaCl2 (see recipe). Seal the bottle, and mix by inversion. The PEG will dissolve slowly. After the PEG is fully dissolved, bring volume to 1 L with KCl/CaCl2. Sterilize by autoclaving. Store at room temperature indefinitely. Immediately before use, shake and filter sterilize using a syringe-driven 0.22-µm PVDF filter into a small sterile, clean, detergent-free vial to avoid PEG precipitate after storage.
Protoplasting solution
- 10.0 g VinoTaste Pro (Lamothe-Abiet)
- 100 ml KCl/citric acid solution (see recipe)
- Filter sterilize using a syringe-driven 0.22-µm PVDF filter
- After filtration and within 30 min before use, mix with 100 ml SAB liquid medium (see recipe)
SAB liquid medium
- 30 g SAB liquid medium powder (e.g., Oxoid)
- 1 L deionized distilled water
- Sterilize by autoclaving
- Store at room temperature for up to a few months
YPS/hygromycin B selection plates
- 20 g yeast extract
- 0.606 g Tris base
- 5.0 g bacto peptone
- 342.3 g sucrose
- 15 g agar (Technical No. 1)
- 1 L deionized distilled water
- Adjust pH to 6 with citric acid
- Sterilize by autoclaving and then cool to ∼50°C
- Add 1 ml of 200 mg/ml hygromycin B (see recipe) and mix well
- Pour 4 ml/well into sterile 6-well flat-bottom cell culture plates
- Store at 4°C for up to a few weeks
COMMENTARY
Background Information
The pathogenic fungus A. fumigatus is a leading cause of morbidity and mortality in humans. The global burden of chronic forms of aspergillosis is estimated at 3 million individuals, while 4.8 million are thought to suffer from allergic aspergillosis (Bongomin, Gago, Oladele, & Denning, 2017). A further 200,000 individuals are believed to be affected by invasive aspergillosis every year (Bongomin et al., 2017). The azole class of antifungals are the first-line therapeutics for treatment of most cases of aspergillosis (Patterson et al., 2016); however, resistance is emerging (Verweij et al., 2015). For those patients infected with a resistant isolate, mortality rates exceed 80%. In some centers, resistance rates are reaching levels that prevent use of azoles as sole primary therapeutics (Verweij et al., 2015). Novel therapeutics are therefore required for treatment. Our understanding of the molecular mechanisms that drive pathogenicity and drug resistance have been hampered by the lack of large mutant collections, which limit our ability to perform functional genomics analysis.
As no genome-wide knockout library is available in any pathogenic filamentous fungus, much of the research has been driven by hypotheses derived from model organisms that have substantially superior functional genomic resources. Current fungal genome-wide knockout libraries exist for the model nonpathogenic yeasts Saccharomyces cerevisiae , Schizosaccharomyces pombe , and the model nonpathogenic filamentous fungi Neurospora crassa. Incomplete libraries representing ∼65% and 20% of the genomes for the pathogenic yeasts Cryptococcus neoformans and Candida albicans , respectively, are also available. While these libraries have proved to be valuable for understanding certain universal aspects of fungal biology, they are unable to answer key questions relating to pathogenesis or allergy caused by A. fumigatus because: (1) none of the resources are in organisms that cause allergy; (2) significant differences are known to exist between factors that define immune responses to C. albicans and A. fumigatus (Erwig & Gow, 2016); (3) the pathogenic niche of A. fumigatus , which causes infections primarily in the lung, is significantly different from that encountered by the bloodstream infections that typify invasive candidiasis; and (4) unlike yeasts, the pathogenic success of A. fumigatus is linked with its ability to produce secondary metabolites (Heinekamp et al., 2015).
There are also more fundamental questions that remain unanswered; for example, the cohort of genes required for A. fumigatus viability is unknown. Inferences can be made from related organisms; however, as only 60% of essential genes are shared between S. cerevisiae and the related yeast C. albicans , it is unclear whether these comparisons are valid (Roemer et al., 2003). As this cohort of genes represents likely antifungal drug targets, such information is critical to inform further drug development. It is also noteworthy that the mechanisms of clinical drug resistance observed in yeasts differ significantly from that seen in filamentous fungi (Sanglard, 2016). Therefore, there is a clear need for methods that support the introduction of functional genomics in A. fumigatus.
There are significant technical hurdles in the generation of large numbers of gene replacement mutants in A. fumigatus. The efficiency of homologous recombination in A. fumigatus is much lower than in yeasts; however, previous studies have demonstrated that strains lacking nonhomologous end joining by virtue of mutations in either ku80, ku70 , or lig4 (Ishibashi, Suzuki, Ando, Takakura, & Inoue, 2006; Ninomiya, Suzuki, Ishii, & Inoue, 2004) can be used to facilitate high-efficiency homologous recombination in filamentous fungi. Even in these strains, however, effective gene replacement requires the generation of gene disruption cassettes with around 1 kb of complementary sequence flanking the gene of interest. Methods to generate cassettes rapidly and cheaply using a PCR approach to fuse two gene-flanking regions to a selectable marker have been described (Szewczyk et al., 2006). However, adoption of this technology has been limited due to difficulties in reproducibly generating cassettes for different targets. In this method we define high-throughput processes for generating large numbers of barcoded gene replacement mutants in A. fumigatus. Mutants generated in this way are suitable for use in competitive fitness profiling methods as previously described in this organism (Macdonald et al., 2019).
Critical Parameters
Basic Protocol 1 step 9 : Do not try to amplify the upstream and downstream fragments in the same PCR reaction. Although this sometimes works, we have found that there is potential for amplification between primers P1 and P4.
Basic Protocol 1 step 15 : The hph selective marker cassette must be gel purified. We noticed that normal PCR purification (e.g., using Qiagen PCR purification kit) is not sufficient and will compromise the efficiency of the fusion PCR.
Basic Protocol 1 step 16 : Do not try to run the fusion PCR with primers P1 and P4. Instead, using nested primers P5 and P6 will greatly increase the specificity of amplification and produce purer fusion PCR products with fewer extra bands.
Basic Protocol 2 step 1 : It is important to use fresh spores (harvested and stored for <1 month at 4°C), as we noticed that spores stored longer could sometimes reduce the amount of protoplasts.
Basic Protocol 2 step 3 : Higher temperatures may cause substantial lysis of protoplasts. Monitor protoplasting microscopically if needed. We suggest that conditions for protoplasting should be carefully tested when using a different strain or using a new batch of VinoTaste, as we have noticed that batch differences can affect the speed of protoplasting.
Basic Protocol 2 step 12 : The PEG solution should not be kept on ice before it is added. Cooling the solution causes the PEG to precipitate. Storage of PEG solution can also sometimes cause it to precipitate; therefore, we recommend checking the PEG solution carefully before use.
Basic Protocol 2 step 13 : It is critical to mix the protoplasts well with fusion PCR products by pipetting; otherwise, the transformation efficacy will be compromised.
Basic Protocol 2 step 17 : Do not seal the 6-well culture plates with Parafilm; sufficient aeration is required to allow conidiation. We noticed that cross contamination does not happen when using 6-well culture plates due to their design, as long as the plates are handled with care. However, it is important to seal individual petri dishes with Parafilm, should they be used, to avoid cross contamination. We noticed that sealing petri dishes can also lead to limited oxygen and prohibit conidiation; therefore, we recommend leaving the petri dishes unsealed for the first 24 hr of incubation at 37°C.
Troubleshooting
See Table 1 for common problems encountered with these protocols, possible causes, and potential solutions.
Step | Problem | Possible reason | Solution |
---|---|---|---|
Basic Protocol 1 step 18 | Absence of some PCR products from the amplification of upstream and downstream fragments | Pipetting error during PCR setup; mistake in primer design | Check primer design and rerun PCR |
Basic Protocol 1 step 18 | Absence of some PCR products from fusion PCR | Mistake in PCR setup | Rerun PCR |
Hph selective cassette has been stored inappropriately | Use a new batch of Hph cassette | ||
Basic Protocol 2 step 1 | Not enough hyphae or biomass after incubation | Incubation temperature is off | Check if incubator is maintaining temperature |
Conical flask used for incubation is contaminated | Clean flask thoroughly with ethanol, soapy water, and distilled deionized water to remove any detergent or toxic materials | ||
Conidia used for inoculation is too old | Use a fresh batch of conidia | ||
Medium used is contaminated or wrongly prepared | Prepare fresh medium | ||
Basic Protocol 2 step 10 | Number of protoplasts is low after protoplasting | A new batch of VinoTaste was used; protoplasting time was too long | Batch differences exist in VinoTaste; recheck protoplasting conditions and optimize if using a new batch |
Understanding Results
Basic Protocol 1: Amplification of upstream and downstream fragments
The amplified PCR product is checked by agarose gel electrophoresis. A typical readout from a successful result should look similar to Figure 2A and 2B, where the majority of the PCR products of upstream and downstream fragments exhibit a single band at about 1.2 kb. Issues like missing bands or having bands at the wrong sizes will be experienced due to the nature of a high-throughput approach, which can be resolved by reamplification. We recommend performing agarose gel electrophoresis to check the raw product immediately after PCR and before purification and only proceeding to the purification steps if the results show successful amplification, in order to save reagents. After purification, the purified PCR products should be rechecked by agarose gel electrophoresis.
Basic Protocol 1: PCR amplification of selective hph marker cassette
Similar to the upstream and downstream fragments, a successfully amplified hph marker cassette will exhibit a single band at about 2.8 kb on the gel.
Basic Protocol 1: Fusion of upstream, downstream, and hph cassette fragments
Fusion PCR products should look similar to Figure 2C, exhibiting a major band at about 4.8 kb. There are occasionally other fragments that can be seen, apart from the major fragment that is of the desired size. In principle, these extra fragments have the potential to integrate into the genome upon transformation; however, as we are using a fungal strain deficient in nonhomologous end joining (i.e., a Δ ku80 isolate), this effect is very insignificant in practice.
Basic Protocol 2: Production of transformable protoplasts
After inoculation and overnight incubation of A. fumigatus , the biomass in the conical flask should look dense and almost fill the liquid culture medium to form a cloudy suspension. Only proceed to the protoplasting steps if the biomass looks normal; a lower yield almost always produces insufficient protoplasts and compromises transformation later on. We suggest quantifying the protoplasts before proceeding to transformation. The concentration of protoplasts should be between 1 × 108 and 1 × 109 protoplasts/ml, if the protocol is followed faithfully. We recommend stopping the experiment immediately once noticing that the protoplast count is lower than 1 × 108 per ml and repeating the steps for preparing transformable protoplasts.
Basic Protocol 2: Transformation
Transformation should be well controlled. The “no DNA” negative control plate should yield no growth after incubation, indicating there was no contamination during the procedure. The positive control should yield similar results as proven in previous experiments, indicating the transformation procedure was carried out as expected. The accrual readout from each individual, transformed strains varies. Depending on the gene that was deleted, some grow like the parental strain and some could exhibit different phenotypes that need to be analyzed on a case-by-case basis.
Time Considerations
Presented are the recommended time frames for each step of the protocol for generating one 96-well plate worth of A. fumigatus knockout mutants. To scale up the experiment, plan your experiment accordingly to improve efficacy.
Amplification of the hph cassette requires 3 hr including PCR preparation; cassettes may be amplified in advance and stored at –80°C. Purification of the hph cassette requires 1 hr. Preparation of two 96-well plates for upstream and downstream PCR takes 1 to 1.5 hr, including dilution of stock primers P1 to P4 and PCR preparation. Performing upstream and downstream PCR takes ∼2 hr. Gel electrophoresis to check upstream and downstream PCR products takes 2 hr including gel preparation (20 min for gel to cool), sample loading time (10 min per 96-well gel), and gel running time (30 min per gel at 90 V). Purification of the upstream and downstream PCR products takes 1 hr. Preparation of one 96-well plate for fusion PCR requires 40 min, including dilution of stock primers P5 and P6 and PCR preparation. The fusion PCR protocol takes ∼4 hr.
Preparation of 6-well YPS/hygromycin B selection plates (16 total) requires 2.5 to 3.5 hr, including medium preparation, autoclaving (2 to 3 hr depending on the volume and autoclave used), plate labeling (10 min), pouring (10 min), and cooling the plates (20 min). Inoculation of A. fumigatus and hyphal growth takes 16 hr (overnight). Protoplasting and protoplast purification requires 3.5 hr including protoplasting (3 hr), washing, and counting (30 min). Transformation takes 30 min, but an overnight incubation at room temperature is required before the plates can be transferred into the incubator at 37°C. Incubation takes 3 to 5 days depending on the phenotype and growth rate of the transformants.
Acknowledgments
This work was supported by a Biomedical Resources Grant UNS43895 from the Wellcome Trust and by a special grant from the Leibniz Institute for Natural Product Research and Infection Biology (HKI).
Literature Cited
- Bongomin, F., Gago, S., Oladele, R. O., & Denning, D. W. (2017). Global and multi-national prevalence of fungal diseases-estimate precision. Journal of Fungi (Basel) , 3, E57. doi: 10.3390/jof3040057.
- Brown, G. D., Denning, D. W., Gow, N. A., Levitz, S. M., Netea, M. G., & White, T. C. (2012). Hidden killers: Human fungal infections. Science Translational Medicine , 165, 165rv13. doi: 10.1126/scitranslmed.3004404.
- Erwig, L. P., & Gow, N. A. (2016). Interactions of fungal pathogens with phagocytes. Nature Reviews Microbiology , 14, 163–176. doi: 10.1038/nrmicro.2015.21.
- Fraczek, M. G., Bromley, M., Buied, A., Moore, C. B., Rajendran, R., Rautemaa, R., … Bowyer, P. (2013). The cdr1B efflux transporter is associated with non-cyp51a-mediated itraconazole resistance in Aspergillus fumigatus. Journal of Antimicrobial Chemotherapy , 68, 1486–1496. doi: 10.1093/jac/dkt075.
- Fraczek, M. G., Zhao, C., Dineen, L., Lebedinec, R., Bowyer, P., Bromley, M., & Delneri, D. (2019). Fast and reliable PCR amplification from Aspergillus fumigatus spore suspension without the need of traditional DNA extraction. Current Protocols in Microbiology , 54, e89. doi: 10.1002/cpmc.89.
- Heinekamp, T., Schmidt, H., Lapp, K., Pähtz, V., Shopova, I., Köster-Eiserfunke, N., … Brakhage, A. A. (2015). Interference of Aspergillus fumigatus with the immune response. Seminars in Immunopathology , 37, 141–152. doi: 10.1007/s00281-014-0465-1.
- Innis, M., & Gelfand, D. (1990). Optimization of PCRs. In M. J. McPherson (Ed.), PCR Protocols: A guide to methods and applications (pp. 3–12). New York: Academic Press.
- Ishibashi, K., Suzuki, K., Ando, Y., Takakura, C., & Inoue, H. (2006). Nonhomologous chromosomal integration of foreign DNA is completely dependent on MUS-53 (human Lig4 homolog) in Neurospora. Proceedings of the National Academy of Sciences of the United States of America , 103, 14871–14876. doi: 10.1073/pnas.0604477103.
- Macdonald, D., Thomson, D. D., Johns, A., Contreras Valenzuela, A., Gilsenan, J. M., Lord, K. M., … Bromley, M. J. (2019). Inducible cell fusion permits use of competitive fitness profiling in the human pathogenic fungus Aspergillus fumigatus. Antimicrobial Agents and Chemotherapy , 63, e01615–e01618. doi: 10.1128/AAC.01615-18.
- Ninomiya, Y., Suzuki, K., Ishii, C., & Inoue, H. (2004). Highly efficient gene replacements in Neurospora strains deficient for nonhomologous end-joining. Proceedings of the National Academy of Sciences of the United States of America , 101, 12248–12253. doi: 10.1073/pnas.0402780101.
- Patterson, T. F., Thompson, G. R., III, Denning, D. W., Fishman, J. A., Hadley, S., Herbrecht, R., … Bennett, J. E. (2016). Practice guidelines for the diagnosis and management of aspergillosis: 2016 update by the Infectious Diseases Society of America. Clinical Infectious Diseases , 63, e1–e60. doi: 10.1093/cid/ciw326.
- Roemer, T., Jiang, B., Davison, J., Ketela, T., Veillette, K., Breton, A., … Bussey, H. (2003). Large-scale essential gene identification in Candida albicans and applications to antifungal drug discovery. Molecular Microbiology , 50, 167–181. doi: 10.1046/j.1365-2958.2003.03697.x.
- Sanglard, D. (2016). Emerging threats in antifungal-resistant fungal pathogens. Frontiers in Medicine (Lausanne) , 3, 11.
- Szewczyk, E., Nayak, T., Oakley, C. E., Edgerton, H., Xiong, Y., Taheri-Talesh, N., … Oakley, B. R. (2006). Fusion PCR and gene targeting in Aspergillus nidulans. Nature Protocols , 1, 3111–3120. doi: 10.1038/nprot.2006.405.
- Verweij, P. E., Ananda-Rajah, M., Andes, D., Arendrup, M. C., Brüggemann, R. J., Chowdhary, A., … Donnelly, P. (2015). International expert opinion on the management of infection caused by azole-resistant Aspergillus fumigatus. Drug Resistance Updates , 21–22, 30–40. doi: 10.1016/j.drup.2015.08.001.
- Voytas, D. (2000). Agarose gel electrophoresis. Current Protocols in Molecular Biology , 51, 2.5A.1–2.5A.9. doi: 10.1002/0471142727.mb0205as51.
Key Reference
- Szewczyk et al. (2006). See above.
Describes a rapid and inexpensive method for generating cassettes for individual targets using a PCR approach to fuse two gene-flanking regions to a selectable marker.
Internet Resources
Global Action Fund for Fungal Infections (GAFFI).
Aspergillus Genome Database.
BatchPrimer3.
BioMart tool.
R-Studio.
CONC@MATE.
Citing Literature
Number of times cited according to CrossRef: 30
- Víctor Coca-Ruiz, Nuria Cabrera-Gómez, Isidro G. Collado, Josefina Aleu, Improved Protoplast Production Protocol for Fungal Transformations Mediated by CRISPR/Cas9 in Botrytis cinerea Non-Sporulating Isolates, Plants, 10.3390/plants13131754, 13 , 13, (1754), (2024).
- Soukaina Timouma, Laura Natalia Balarezo-Cisneros, Jean-Marc Schwartz, Daniela Delneri, Development of a genome-scale metabolic model for the lager hybrid yeast S. pastorianus to understand the evolution of metabolic pathways in industrial settings , mSystems, 10.1128/msystems.00429-24, 9 , 6, (2024).
- I. S. R. Storer, L. E. Sastré-Velásquez, T. Easter, B. Mertens, A. Dallemulle, M. Bottery, R. Tank, M. Offterdinger, M. J. Bromley, N. van Rhijn, F. Gsaller, Shining a light on the impact of antifungals on Aspergillus fumigatus subcellular dynamics through fluorescence imaging , Antimicrobial Agents and Chemotherapy, 10.1128/aac.00803-24, (2024).
- Xiao Gong, Heng Zhang, Wenxu Cheng, Zhangxuan He, Tianyan Ma, Tian Chen, Yi Sun, Aspergillus fumigatus ctf1 –a novel zinc finger transcription factor involved in azole resistance , Mycology, 10.1080/21501203.2024.2342521, (1-14), (2024).
- Camila Figueiredo Pinzan, Clara Valero, Patrícia Alves de Castro, Jefferson Luiz da Silva, Kayleigh Earle, Hong Liu, Maria Augusta Crivelente Horta, Olaf Kniemeyer, Thomas Krüger, Annica Pschibul, Derya Nur Cömert, Thorsten Heinekamp, Axel A. Brakhage, Jacob L. Steenwyk, Matthew E. Mead, Nico Hermsdorf, Scott G. Filler, Nathalia Gonsales da Rosa-Garzon, Endrews Delbaje, Michael J. Bromley, Hamilton Cabral, Camila Diehl, Claudia B. Angeli, Giuseppe Palmisano, Ashraf S. Ibrahim, David C. Rinker, Thomas J. C. Sauters, Karin Steffen, Adiyantara Gumilang, Antonis Rokas, Sara Gago, Thaila F. dos Reis, Gustavo H. Goldman, Aspergillus fumigatus conidial surface-associated proteome reveals factors for fungal evasion and host immunity modulation, Nature Microbiology, 10.1038/s41564-024-01782-y, 9 , 10, (2710-2726), (2024).
- Norman van Rhijn, Can Zhao, Narjes Al-Furaiji, Isabelle S. R. Storer, Clara Valero, Sara Gago, Harry Chown, Clara Baldin, Rachael-Fortune Grant, Hajer Bin Shuraym, Lia Ivanova, Olaf Kniemeyer, Thomas Krüger, Elaine Bignell, Gustavo H. Goldman, Jorge Amich, Daniela Delneri, Paul Bowyer, Axel A. Brakhage, Hubertus Haas, Michael J. Bromley, Functional analysis of the Aspergillus fumigatus kinome identifies a druggable DYRK kinase that regulates septal plugging, Nature Communications, 10.1038/s41467-024-48592-8, 15 , 1, (2024).
- Katharina Steinert, Anna K. Atanasoff-Kardjalieff, Elias Messner, Markus Gorfer, Eva-Maria Niehaus, Hans-Ulrich Humpf, Lena Studt-Reinhold, Svetlana A. Kalinina, Tools to make Stachybotrys chartarum genetically amendable: Key to unlocking cryptic biosynthetic gene clusters, Fungal Genetics and Biology, 10.1016/j.fgb.2024.103892, 172 , (103892), (2024).
- Marketa Samalova, Patricia Flamant, Rémi Beau, Mike Bromley, Maryse Moya-Nilges, Thierry Fontaine, Jean-Paul Latgé, Isabelle Mouyna, The New GPI-Anchored Protein, SwgA, Is Involved in Nitrogen Metabolism in the Pathogenic Filamentous Fungus Aspergillus fumigatus, Journal of Fungi, 10.3390/jof9020256, 9 , 2, (256), (2023).
- Qiang Ding, Chao Ye, Microbial cell factories based on filamentous bacteria, yeasts, and fungi, Microbial Cell Factories, 10.1186/s12934-023-02025-1, 22 , 1, (2023).
- Jufang Tan, Heng Zhang, Yi Sun, Lujuan Gao, Afu-Emi1 Contributes to Stress Adaptation and Voriconazole Susceptibility in Aspergillus fumigatus, Microbiology Spectrum, 10.1128/spectrum.00956-23, 11 , 3, (2023).
- Tim J.H. Baltussen, Norman van Rhijn, Jordy P.M. Coolen, Jan Dijksterhuis, Paul E. Verweij, Michael J. Bromley, Willem J.G. Melchers, The C 2 H 2 transcription factor SltA is required for germination and hyphal development in Aspergillus fumigatus , mSphere, 10.1128/msphere.00076-23, 8 , 4, (2023).
- Shuang Deng, Joonhoon Kim, Kyle R. Pomraning, Yuqian Gao, James E. Evans, Beth A. Hofstad, Ziyu Dai, Bobbie-Jo Webb-Robertson, Samantha M. Powell, Irina V. Novikova, Nathalie Munoz, Young-Mo Kim, Marie Swita, Ana L. Robles, Teresa Lemmon, Rylan D. Duong, Carrie Nicora, Kristin E. Burnum-Johnson, Jon Magnuson, Identification of a specific exporter that enables high production of aconitic acid in Aspergillus pseudoterreus, Metabolic Engineering, 10.1016/j.ymben.2023.09.011, 80 , (163-172), (2023).
- Vimalraj Ponnusamy, Meenakshisundaram Sankaranarayanan, Targeted gene manipulation of Leloir pathway genes for the constitutive expression of β-galactosidase and its transgalactosylation product galacto-oligosaccharides from Kluyveromyces lactis GG799 and knockout strains, Enzyme and Microbial Technology, 10.1016/j.enzmictec.2023.110263, 169 , (110263), (2023).
- Johanna Rhodes, Alireza Abdolrasouli, Katie Dunne, Thomas R. Sewell, Yuyi Zhang, Eloise Ballard, Amelie P. Brackin, Norman van Rhijn, Harry Chown, Alexandra Tsitsopoulou, Raquel B. Posso, Sanjay H. Chotirmall, Noel G. McElvaney, Philip G. Murphy, Alida Fe Talento, Julie Renwick, Paul S. Dyer, Adrien Szekely, Paul Bowyer, Michael J. Bromley, Elizabeth M. Johnson, P. Lewis White, Adilia Warris, Richard C. Barton, Silke Schelenz, Thomas R. Rogers, Darius Armstrong-James, Matthew C. Fisher, Population genomics confirms acquisition of drug-resistant Aspergillus fumigatus infection by humans from the environment, Nature Microbiology, 10.1038/s41564-022-01091-2, 7 , 5, (663-674), (2022).
- Paul Bowyer, Andrew Currin, Daniela Delneri, Marcin G. Fraczek, Telomere-to-telomere genome sequence of the model mould pathogen Aspergillus fumigatus, Nature Communications, 10.1038/s41467-022-32924-7, 13 , 1, (2022).
- Takanori Furukawa, Norman van Rhijn, Harry Chown, Johanna Rhodes, Narjes Alfuraiji, Rachael Fortune-Grant, Elaine Bignell, Matthew C. Fisher, Michael Bromley, Exploring a novel genomic safe-haven site in the human pathogenic mould Aspergillus fumigatus, Fungal Genetics and Biology, 10.1016/j.fgb.2022.103702, 161 , (103702), (2022).
- Clara Valero, Ana Cristina Colabardini, Patrícia Alves de Castro, Lilian Pereira Silva, Laure Nicolas Annick Ries, Lakhansing Pardeshi, Fang Wang, Marina Campos Rocha, Iran Malavazi, Roberto Nascimento Silva, Celso Martins, Patrícia Domingos, Cristina Pereira-Silva, Michael J. Bromley, Koon Ho Wong, Gustavo H. Goldman, Aspergillus Fumigatus ZnfA, a Novel Zinc Finger Transcription Factor Involved in Calcium Metabolism and Caspofungin Tolerance, Frontiers in Fungal Biology, 10.3389/ffunb.2021.689900, 2 , (2021).
- Devanshi Khokhani, Cristobal Carrera Carriel, Shivangi Vayla, Thomas B. Irving, Christina Stonoha-Arther, Nancy P. Keller, Jean-Michel Ané, Deciphering the Chitin Code in Plant Symbiosis, Defense, and Microbial Networks, Annual Review of Microbiology, 10.1146/annurev-micro-051921-114809, 75 , 1, (583-607), (2021).
- Caitlin H. Kowalski, Kaesi A. Morelli, Jason E. Stajich, Carey D. Nadell, Robert A. Cramer, A Heterogeneously Expressed Gene Family Modulates the Biofilm Architecture and Hypoxic Growth of Aspergillus fumigatus , mBio, 10.1128/mBio.03579-20, 12 , 1, (2021).
- Zhonghua Liu, Shriya Raj, Norman van Rhijn, Marcin Fraczek, Jean-Philippe Michel, Odile Sismeiro, Rachel Legendre, Hugo Varet, Thierry Fontaine, Michael Bromley, Jean-Paul Latgé, Functional Genomic and Biochemical Analysis Reveals Pleiotropic Effect of Congo Red on Aspergillus fumigatus, mBio, 10.1128/mBio.00863-21, 12 , 3, (2021).
- Matthew E Mead, Jacob L Steenwyk, Lilian P Silva, Patrícia A de Castro, Nauman Saeed, Falk Hillmann, Gustavo H Goldman, Antonis Rokas, An evolutionary genomic approach reveals both conserved and species-specific genetic elements related to human disease in closely related Aspergillus fungi , Genetics, 10.1093/genetics/iyab066, 218 , 2, (2021).
- Ivey A. Geoghegan, Malcolm Stratford, Mike Bromley, David B. Archer, Simon V. Avery, Weak Acid Resistance A (WarA), a Novel Transcription Factor Required for Regulation of Weak-Acid Resistance and Spore-Spore Heterogeneity in Aspergillus niger, mSphere, 10.1128/mSphere.00685-19, 5 , 1, (2020).
- Clara Valero, Ana Cristina Colabardini, Jéssica Chiaratto, Lakhansing Pardeshi, Patrícia Alves de Castro, Jaire Alves Ferreira Filho, Lilian Pereira Silva, Marina Campos Rocha, Iran Malavazi, Jonas Henrique Costa, Taícia Fill, Mário Henrique Barros, Sarah Sze Wah Wong, Vishukumar Aimanianda, Koon Ho Wong, Gustavo H. Goldman, Aspergillus fumigatus Transcription Factors Involved in the Caspofungin Paradoxical Effect, mBio, 10.1128/mBio.00816-20, 11 , 3, (2020).
- Ye-Eun Son, Hee-Soo Park, Genetic Manipulation and Transformation Methods for Aspergillus spp. , Mycobiology, 10.1080/12298093.2020.1838115, 49 , 2, (95-104), (2020).
- Mengyao Niu, Breanne N. Steffan, Gregory J. Fischer, Nandhitha Venkatesh, Nicholas L. Raffa, Molly A. Wettstein, Jin Woo Bok, Claudio Greco, Can Zhao, Erwin Berthier, Ernst Oliw, David Beebe, Michael Bromley, Nancy P. Keller, Fungal oxylipins direct programmed developmental switches in filamentous fungi, Nature Communications, 10.1038/s41467-020-18999-0, 11 , 1, (2020).
- Norman van Rhijn, Takanori Furukawa, Can Zhao, Bethany L. McCann, Elaine Bignell, Michael J. Bromley, Development of a marker-free mutagenesis system using CRISPR-Cas9 in the pathogenic mould Aspergillus fumigatus, Fungal Genetics and Biology, 10.1016/j.fgb.2020.103479, 145 , (103479), (2020).
- Roland Martzy, Astrid R. Mach-Aigner, The Potential of Synthetic Biology for Trichoderma reesei, Trichoderma reesei, 10.1007/978-1-0716-1048-0_3, (45-54), (2020).
- Marketa Samalova, Paul Carr, Mike Bromley, Michael Blatzer, Maryse Moya-Nilges, Jean-Paul Latgé, Isabelle Mouyna, GPI Anchored Proteins in Aspergillus fumigatus and Cell Wall Morphogenesis, 10.1007/82_2020_207, (2020).
- Benjamin P. Thornton, Anna Johns, Reem Al-Shidhani, Sandra Álvarez-Carretero, Isabelle S. R. Storer, Michael J. Bromley, Lydia Tabernero, Identification of Functional and Druggable Sites in Aspergillus fumigatus Essential Phosphatases by Virtual Screening, International Journal of Molecular Sciences, 10.3390/ijms20184636, 20 , 18, (4636), (2019).
- Marcin G. Fraczek, Can Zhao, Lauren Dineen, Ressa Lebedinec, Paul Bowyer, Michael Bromley, Daniela Delneri, Fast and Reliable PCR Amplification from Aspergillus fumigatus Spore Suspension Without Traditional DNA Extraction, Current Protocols in Microbiology, 10.1002/cpmc.89, 54 , 1, (2019).