Generation of Tumor Spheroids to Evaluate T Cell and NK Cell Cytotoxicity
Alessandro Poggi, Alessandro Poggi
Abstract
Tumor cell spheroids are one of the three-dimensional (3D) culture systems that can be used to study the features of tumor cells in a more physiologic condition. Indeed, this 3D culture system has been validated in preclinical studies to select anticancer drugs. Tumor cell spheroids can be obtained through employing different procedures, and here I describe in detail how to obtain spheroids from tumor cell lines of colorectal carcinoma (CRC). I analyze the procedures employed to evaluate the phenotype and growth of tumor cells in this 3D culture system. Also, interaction with immune cells is considered. Indeed, it is relevant to define whether the antitumor effects exerted by different cytotoxic lymphocyte subsets are similar when tumor cells are used either as cells adherent to a plastic substrate or floating spheroids. To obtain optimal results in this complex system, some parameters must be considered, such as those related to poorly defined experimental variables including biological and biophysical features of tumor cell lines and the quality (purity and time to use after generation) of antitumor effector lymphocyte subsets. Also, I describe in detail the methods to generate these lymphocyte subsets and to characterize their cytotoxic potential and effectiveness in killing tumor cells. Human natural killer cells and T lymphocytes expressing the γδ T cell receptor (in particular Vδ2 T cells) are considered among the cytotoxic lymphocyte populations. Eventually, it should be possible to obtain reliable and feasible results to study the molecular mechanisms involved in recognition and killing of CRC spheroids exerted by effector lymphocytes. © 2022 The Authors. Current Protocols published by Wiley Periodicals LLC.
Basic Protocol 1 : Generation of tumor cell spheroids
Basic Protocol 2 : Evaluation of ATP content of spheroids
Basic Protocol 3 : Evaluation of antigen expression by cell spheroids
Basic Protocol 4 : Generation of effector lymphocytes
Basic Protocol 5 : Coculture of spheroids and lymphocytes
Basic Protocol 6 : Evaluation of lymphocyte cytotoxicity using crystal violet staining
INTRODUCTION
The development of human tumors is not easily reproducible in animal models, which are elegant but in some instances extremely artificial (Hahn et al., 2021; Ito, Takahashi, & Ito, 2018; Lee et al., 2021; Martinov et al., 2021). Some in vitro three-dimensional (3D) culture systems have been proposed and validated by the European Union Reference Laboratories for Alternatives to Animal Testing to substitute or reduce the number of animals sacrificed to analyze in preclinical studies of the effectiveness of drugs (Anderson, Dutertre, Ginhoux, & Murphy, 2021; Huang et al., 2020; Mestas & Hughes, 2004; Vacca, Chiossone, Mingari, & Moretta, 2019). Furthermore, in the era of immune checkpoint inhibitors used to release the brake on the immune system to better recognize tumor cells, it is essential that the function of effector lymphocytes can be assessed against a mass-structured tumor, which mimics the in vivo tumor appearance (De Santis et al., 2021; Nishino, Ramaiya, Hatabu, & Hodi, 2017; Siozopoulou et al., 2021; Xiao et al., 2020; Zhou, Xu, Shibata, Saloura, & Uppaluri, 2021). In this context, tumor spheroids are reminiscent of the small tumor cell clusters that represent the first stage of cancer development, and this experimental approach allows one to detect whether immune cells show a different behavior when challenged with floating nonadherent tumor cells as in conventional cytotoxic assays or a tumor mass (Bonci et al., 2009; Di Mascolo et al., 2019; Elsner & Dressel, 2020; Goldberg, Sherwood, & Clayberger, 1999; Sargenti et al., 2020; Varesano, Zocchi, & Poggi, 2018). Indeed, it is conceivable that immune cells will interact with the periphery of the tumor spheroids, and therefore only a fraction of immune cells will be able to penetrate the tumor mass (Di Mascolo et al., 2019; Sargenti et al., 2020; Varesano et al., 2018). Thus, these 3D models can be useful to study the behavior and the molecular mechanisms by which different effector lymphocytes exert their cytolytic activity against a tumor mass (Courau et al., 2019; Dangles-Marie et al., 2003; Di Mascolo et al., 2019; Sargenti et al., 2020; Sherman, Gitschier, & Rossi, 2018; Varesano et al., 2018). Herein, I describe protocols to generate spheroids and different antitumor effector lymphocytes, which can be mixed together to evaluate cytolytic activity. Additional protocols are provided for image analysis of the reduction of spheroid size (Di Mascolo et al., 2019; Sargenti et al., 2020; Varesano et al., 2018) and evaluation of vitality of tumor cells after their adhesion to appropriate plasticware.
STRATEGIC PLANNING
Evaluation of the cytotoxicity of immune cells against tumor cell spheroids requires that the two cell populations used in the experiments are ready and in good condition. Furthermore, if different immune cells are used in cytotoxic experiments, the user should be skilled in generating effector cell populations from different subsets, and the cell's cytolytic activity should be tested in conventional cytotoxicity assays before using them with spheroids. A schematic detailing relevant planning steps is shown in Figure 1, which depicts when to start and how to maintain the cultures of the different players used in the protocols.
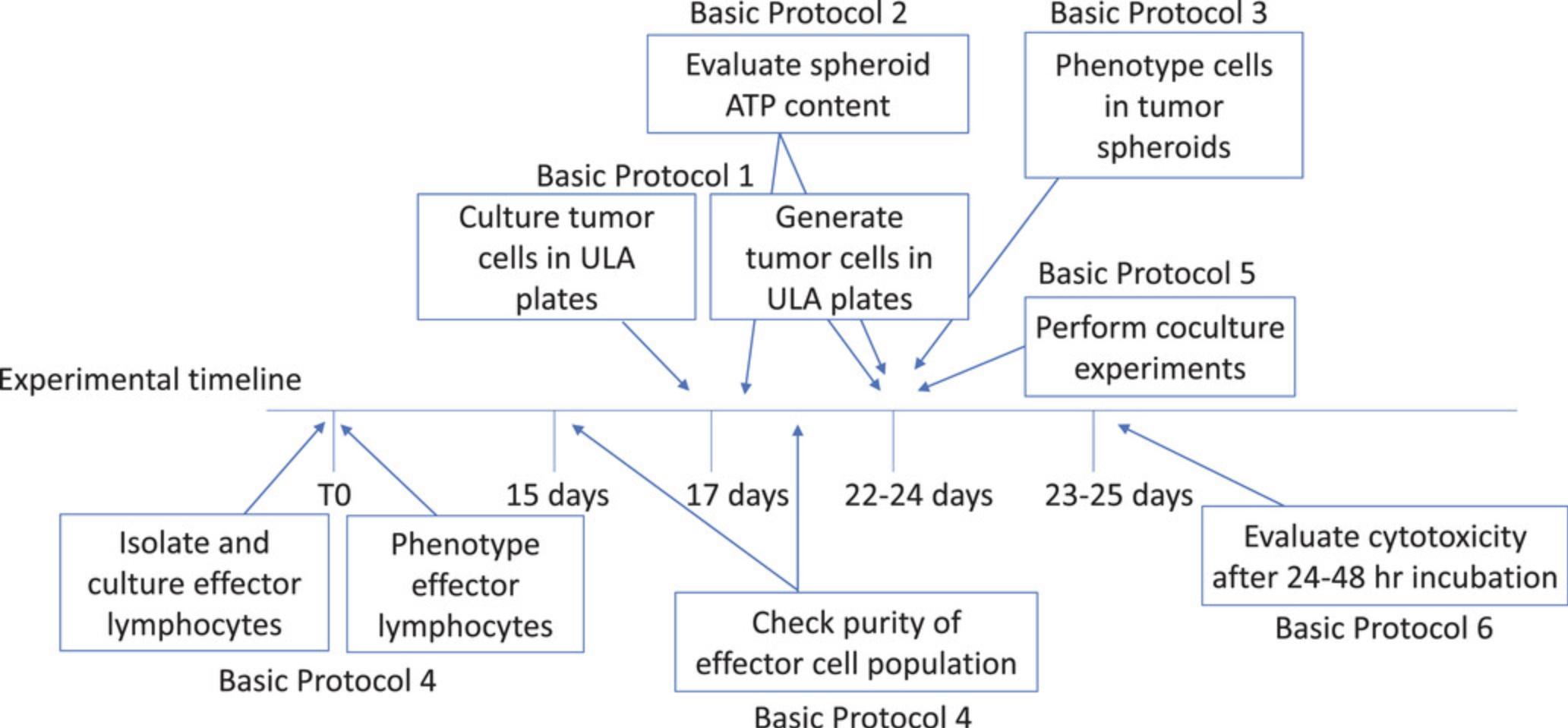
Basic Protocol 1: GENERATION OF TUMOR CELL SPHEROIDS
This protocol describes how to generate spheroids from three different colorectal carcinoma (CRC) cell lines. The protocol can be applied to any cell line independent of its histologic origin. The number of tumor cells different from those described here should be determined experimentally by serial dilution.
If it has not been determined whether a CRC tumor cell line can generate spheroids (Di Mascolo et al., 2019; Sargenti et al., 2020; Varesano et al., 2018), it is necessary to test experimentally whether this cell line can lead to spheroids. The procedure described here can be applied to any kind of tumor cell line from any tissue origin. Usually, epithelial cell lines that grow as adherent cells are able to give rise to cell aggregates if cultured in ultralow-adherent plates. However, it is not possible to know whether a given cell line can generate spheroids. In my laboratory's experience, CRC cell lines such as DLD-1 and SW-620 can form spheroids, whereas LS180 and SW-48 do not form spheroids, although all these cell lines adhere to plastic in conventional cultures (Varesano et al., 2018).
To generate spheroids, CRC cell lines (DLD-1, SW-480, and HCT-116) should be detached from the conventional plastic culture flask, petri dish, or multiwell plate if they are growing as adherent cells; otherwise the user can start directly from step 10.
Materials
-
CRC cell line:
- DLD-1, human, epithelial, adenocarcinoma colorectal Dukes’ type C (e.g., ATCC #CCL-221)
- SW-480, human, epithelial, adenocarcinoma, colorectal Dukes’ type B (e.g., ATCC #CCL-228)
- HCT-116, human, epithelial, adenocarcinoma colorectal (e.g., ATCC #CCL-247)
-
RPMI-1640 medium (e.g., Thermo Fisher Scientific, cat. no. 21875091)
-
Fetal bovine serum (FBS), heat inactivated at 56°C for 30 min (e.g., Thermo Fisher Scientific, cat. no. A3160401)
-
200 mM L-glutamine (e.g., Thermo Fisher Scientific, cat. no. 2916801)
-
100× penicillin-streptomycin (e.g., Thermo Fisher Scientific, cat. no. 15140122)
-
Dulbecco's phosphate-buffered saline (DPBS) without Ca2+ or Mg2+ (e.g., Thermo Fisher Scientific, cat. no. 14190144)
-
0.5% trypsin-EDTA, no phenol red (e.g., Thermo Fisher Scientific, cat. no. 1540054)
-
Dulbecco's modified Eagle medium/nutrient mixture F-12 (DMEM-F-12; e.g., Thermo Fisher Scientific, cat. no. 1132003)
-
Epidermal growth factor (EGF), recombinant, animal free (e.g., Peprotech, cat. no. AF-100-15)
-
37°C water bath
-
50-ml cell culture tubes (e.g., Sarstedt)
-
Centrifuge (e.g., Eppendorf 5810 R with rotor A-4-81)
-
25-cm2 culture flask
-
Cell culture incubator, 37°C, 5% CO2 (e.g., Thermo Fisher Forma Direct Heat CO2 Incubator, 184 L)
-
Microscope
-
Cell counting chamber (e.g., Biosigma, cat. no. BVS100)
-
92 × 16‒mm petri dish (e.g., Sarstedt, cat. no. 82.1473.001)
-
96-well flat-bottom plate, ultralow attachment (e.g., Corning, cat. no. CLS3474-24EA)
Detach cells
1.If DLD-1, SW-48, or HCT-116 CRC cell lines are not in culture, remove frozen vial of each CRC cell line from –80°C (or liquid nitrogen), and thaw in a 37°C water bath with gentle shaking.
2.Add vial content to a 50-ml tube, and add 20 ml complete RPMI-1640 medium (i.e., RPMI-1640 medium containing 10% [v/v] FBS, 1% [w/v] L-glutamine, and 1× penicillin-streptomycin). Centrifuge 5 min at 700 × g , room temperature. Discard supernatant and resuspend cell pellet in 20 ml RPMI-1640 medium. Centrifuge again 5 min at 700 × g , room temperature.
3.Discard supernatant and resuspend pellet with 5 to 7 ml complete RPMI-1640 medium. Transfer cell suspension to a 25-cm2 culture flask (2‒4 × 105 cells/ml), and incubate at 37°C, 5% CO2.
4.After 1 day, view cells under microscope and determine whether cells are attached to the plastic. Discard culture medium, and add 5 ml fresh complete RPMI-1640 medium. Continue to culture cells until they have covered 70% of the flask.
5.Remove cells from incubator, and discard culture supernatant. Resuspend cells in DPBS without Ca2+ or Mg2+. Gently move flask to cover and wash the whole flask. Harvest DPBS.
6.Add sufficient trypsin to culture flask to cover the surface where cells are adherent (3 ml for 25-cm2 flask, 5 ml for 75-cm2 flask), and incubate flask at 37°C for 5 to 10 min.
7.Cover flask and gently tap to detach cells from the plastic. Examine under a microscope to determine if cells are detached.
8.Harvest cells from flask, and place in a tube with RPMI-1640 medium containing 10% (v/v) FBS (1:4 trypsin:RPMI ratio). Centrifuge 5 to 10 min at 700 × g , room temperature.
9.Discard supernatant and resuspend cell pellet in RPMI-1640 medium containing 10% (v/v) FBS. Repeat centrifugation.
Generate tumor spheroid
10.Wash cells by centrifuging 10 min at 700 × g , room temperature, in RPMI-1640 medium (without FBS). Discard supernatant and resuspend cell pellet in fresh RPMI-1640 medium by gently shaking the bottom of the tube.
11.Wash cells again by centrifuging 10 min at 700 × g , room temperature, in RPMI-1640 medium. Discard supernatant and gently resuspend cell pellet in fresh DMEM-F12.
12.Count cells with a disposable cell counting chamber: Count 16 small squares from three nonadjacent large squares composed of the 16 small squares and calculate mean. Multiply mean by 104 (e.g., for 100 cells, cell concentration is 100 × 104 = 106). Adjust cells to desired concentration using DMEM-F12.
13.Pour cell suspension into a plastic petri dish. Using a multichannel pipette, place 100 µl cell suspension in each well of a 96-well plate for obtaining spheroids. Use care to resuspend cells each time to ensure homogenous cell seeding.
14.To each well, add 100 µl DMEM-F12 supplemented with EGF.
15.Incubate cell cultures in a 37°C, 5% CO2 humidified incubator.
16.Check cell cultures daily on a microscope.
17.At days 3, 5, and 7, analyze spheroid size and ATP content (see Basic Protocol 2) of at least four replicate wells.
Basic Protocol 2: EVALUATION OF ATP CONTENT OF SPHEROIDS
Measurement of the ATP content of spheroids is a fast and reliable method to evaluate their viability. This assay can be performed at different time points starting at the onset of spheroid generation.
Additional Materials (also see Basic Protocol 1)
-
Tumor cell spheroids (see Basic Protocol 1)
-
Triton X-100 (e.g., Sigma-Aldrich)
-
CellTiter-Glo Luminescent Cell Viability Assay (e.g., Promega, cat. no. G7570)
-
96-well OptiPlate, low-binding surface (e.g., Perkin Elmer, cat. nos. 6045580 or 6055260)
-
Luminometer (e.g., VICTOR Multilabel Plate Reader)
1.Harvest spheroids from the original plate by gently pipetting 50 to 100 µl medium from each well, and transfer to Optiplate.
2.To each well, add 100 µl DPBS without Ca2+ or Mg2+ containing 1% (v/v) Triton X-100 and CellTiter Glow reagent (concentration as indicated by the manufacturer).
3.Mix by pipetting each well; avoid making bubbles.
4.Measure luminescence immediately with a luminometer.
Basic Protocol 3: EVALUATION OF ANTIGEN EXPRESSION BY CELL SPHEROIDS
The characterization of antigen expression is essential to define whether the tumor spheroids display similar or different phenotypes to the counterpart of the same cell line cultured in conventional plasticware. This protocol describes the staining procedure for spheroids that are disaggregated to obtain a single-cell suspension. Then, cells are processed as samples of floating cells.
Additional Materials (also see Basic Protocol 1)
-
Tumor cell spheroids (see Basic Protocol 1)
-
Purified mouse IgG2β, κ isotype control antibody, clone MCP-11 (e.g., Biolegend, cat. no. 402201)
-
Anti-EPCAM antibody, clone 9C4 mouse IgG2β isotype (e.g., Biolegend, cat. no. 324202)
-
Wash medium: RMPI-1640 medium supplemented with 5% (v/v) FBS
-
Goat anti-mouse (GAM) anti-isotype-specific IgG2β cross-adsorbed secondary antibody, Alexa Fluor 647 (e.g., Thermo Fisher Scientific, cat. no. A-21242)
-
5-ml centrifuge tube
-
Flow cytometer (Cytoflex or similar)
Obtain single-cell suspension
1.Harvest 100 µl spheroids with a 100-µl pipette from three culture wells of the original plate, and place in a 5-ml tube. Add 3 ml DPBS without Ca2+ or Mg2+.
2.Centrifuge 5 to 10 min at 700 × g , room temperature.
3.Discard supernatant and gently resuspend pellet by adding 5 ml DPBS without Ca2+ or Mg2+ and gently shaking the tube. Repeat centrifugation.
4.Discard supernatant and add DPBS without Ca2+ or Mg2+. Keep at room temperature for 10 min, resuspending solution by pipetting up and down twice. Repeat centrifugation, and resuspend pellet in DPBS without Ca2+ or Mg2+.
5.Place 20 µl solution in a disposable counting chamber. Check if cells are disaggregated using a microscope and count cells.
Assess antigen expression
6.Adjust concentration to 104 to 105 cells per 100 µl in RPMI-1640 medium, and prepare two tubes with 100 µl cell suspension: one for the negative control and one for the epithelial cellular adhesion molecule (EPCAM) immunofluorescence assay.
7.To the negative control, add 20 µl of 2.5 μg/ml negative control antibody (isotype IgG2β control). To the second tube, add 20 anti-EPCAM antibody.
8.Incubate at 4°C on ice for 15 to 30 min.
9.Add 2 ml wash medium (4°C) to each tube, and centrifuge 5 to 10 min at 700 × g , 4°C. Discard supernatant and repeat this step.
10.Dry pellet by inverting tube on blotting paper. Resuspend pellet by gently tapping the tube bottom.
11.Add 10 µl of 2 μg/ml GAM anti-isotype IgG2β antibody conjugated with Alexa Fluor 647, and incubate for 15 to 30 min at 4°C on ice.
12.Add 2 ml wash medium (4°C) to each tube, and centrifuge 5 to 10 min at 700 × g , 4°C.
13.Discard supernatant and dry pellet by inverting tube on blotting paper. Resuspend pellet in 0.5 ml RPMI-1640 medium supplemented with 5% (v/v) FBS for flow cytometer analysis.
14.Analyze expression of EPCAM antigen by running samples on a flow cytometer according to the specific procedures for the instrument.
Basic Protocol 4: GENERATION OF EFFECTOR LYMPHOCYTES
This protocol describes the selection, activation, and culture expansion of immune effector cells starting from peripheral blood mononuclear cells (PBMCs). Protocols for obtaining PBMCs are available at: https://www.stemcell.com/isolating-mononuclear-cells-from-whole-blood-by-density-gradient-centrifugation.html. Vδ2+ T cells and natural killer (NK) lymphocytes are considered as effectors.
Materials
-
PBMCs
-
RPMI-1640 medium (e.g., Thermo Fisher Scientific, cat. no. 21875091)
-
2 μM zoledronic acid (e.g., Sigma-Aldrich)
-
Interleukin 2 (IL-2), animal-free recombinant human (e.g., Peprotech, cat. no. AF-200-02)
-
Anti-Vδ2 antibody, clone γδ123R3, IgG1 isotype (e.g., Miltenyi Biotech, cat. no. 130-121-139)
-
Red blood cells, obtained from same donor as PBMCs
-
Lymphocyte cell separation medium (e.g., Cedarline, cat. no. CL5010)
-
Anti-CD16 antibody, clone REA423 (e.g., Miltenyi Biotech, cat. no. 170-078-173)
-
Anti-CD56 antibody, clone REA196 (e.g., Miltenyi Biotech, cat. no. 170-081-044)
-
Phytohemagglutinin (PHA), M form (e.g., Thermo Fisher Scientific, cat. no. 10576015)
-
96-well U-bottom plate (e.g., Sarstedt, cat. no. 83.3924)
-
37°C, 5% CO2 incubator
-
Microscope, brightfield and fluorescence
-
15-ml centrifuge tube
-
Centrifuge
-
Cell counting chamber (e.g., Biosigma, cat. no. BVS100)
-
Irradiator (γ-emitting source)
Isolate Vδ2+ T cell population
1.Dilute PBMCs to 1 × 106 cells/ml in complete RPMI-1640 medium, and place 100 µl in 96-well U-bottom plates.
2.Add 100 µl of 2 µM zoledronic acid to each well.
3.Incubate at 37°C, 5% CO2 for 24 hr.
4.Discard 100 µl medium, and add 100 µl of 60 IU IL-2.Incubate at 37°C, 5% CO2.
5.Replace 100 µl medium every 2 to 3 days until proliferation is evident by microscopic analysis.
6.Split cell cultures when the cell pellet in each well is about half the surface of the well.
7.Analyze expression of Vδ2 T cell antigen receptor on days 10, 14, and 21 by indirect immunofluorescence using an anti-Vδ2 antibody.
8.Use lymphocyte population for coculture with spheroids when the percentage of Vδ2 T cell is more than 90%.
Isolate NK cell population
9.Take 1 × 108 PBMCs in 3 ml RPMI-1640 medium at room temperature, and add 2 ml of 1 × 109 red blood cells from the same donor and 100 µl NK cell separation medium. Mix well and keep at room temperature for 15 min by gently shaking the tube every 5 min.
10.Fill a 15-ml tube with 5 ml lymphocyte cell separation medium, and layer over the 5 ml solution obtained in step 9.
11.Centrifuge 45 min at 1200 × g , room temperature, without brake.
12.Recover ring at the lymphocyte cell separation medium and RPMI-1640 medium interface containing NK cells, and transfer to another 15-ml tube. Fill tube with RPMI-1640 medium, and mix solution by pipetting up and down.
13.Centrifuge 5 to 10 min at 700 × g , room temperature. Discard supernatant and resuspend pellet in RPMI-1640 medium, filling to the top of the tube. Repeat step once more.
14.Count cells and adjust concentration to 106 cells/ml.
15.Analyze purity of NK cells isolated by direct or indirect immunofluorescence using anti-CD16 and anti-CD56 antibodies. Use NK cells only if the purity is >70% of the total population.
16.Culture NK cells at 104 cells/well with 5 µg/ml PHA (final concentration), 30 IU/ml IL-2 (final concentration), and 105 irradiated PBMCs per well (200 µl total volume).
17.Incubate plate at 37°C, 5% CO2. Analyze cell cultures daily, and replace 100 µl medium with fresh IL-2 every 3 days until proliferation is evident by microscopic analysis.
18.Split cell cultures when cell pellets in each well are about half of the well surface. Place split cells in new wells to allow expansion of cell populations.
19.Check purity of cultured NK cells at the desired time point by direct or indirect immunofluorescence, and use in functional experiments if the purity is >85% to 90% of the total population.
Basic Protocol 5: COCULTURE OF SPHEROIDS AND LYMPHOCYTES
Coculture of spheroids and the purified, expanded immune effector cells (Basic Protocol 4) is necessary to study the interaction between lymphocytes and tumor cells (Fig. 2). Importantly, as indicated in Strategic Planning, spheroids and immune cells should be of good condition to obtain the best results. Thus, immune cells should be prepared before tumor cell spheroids. An experimental plan should be identified beforehand, including appropriate negative and positive controls. For determining cytolytic activity of antitumor effector cells, the negative control condition is represented by spheroids cultured in the absence of immune effector cells, whereas spheroids cocultured with effector cells are the positive control. Samples should be prepared in at least triplicate, but usually six replicates are better.
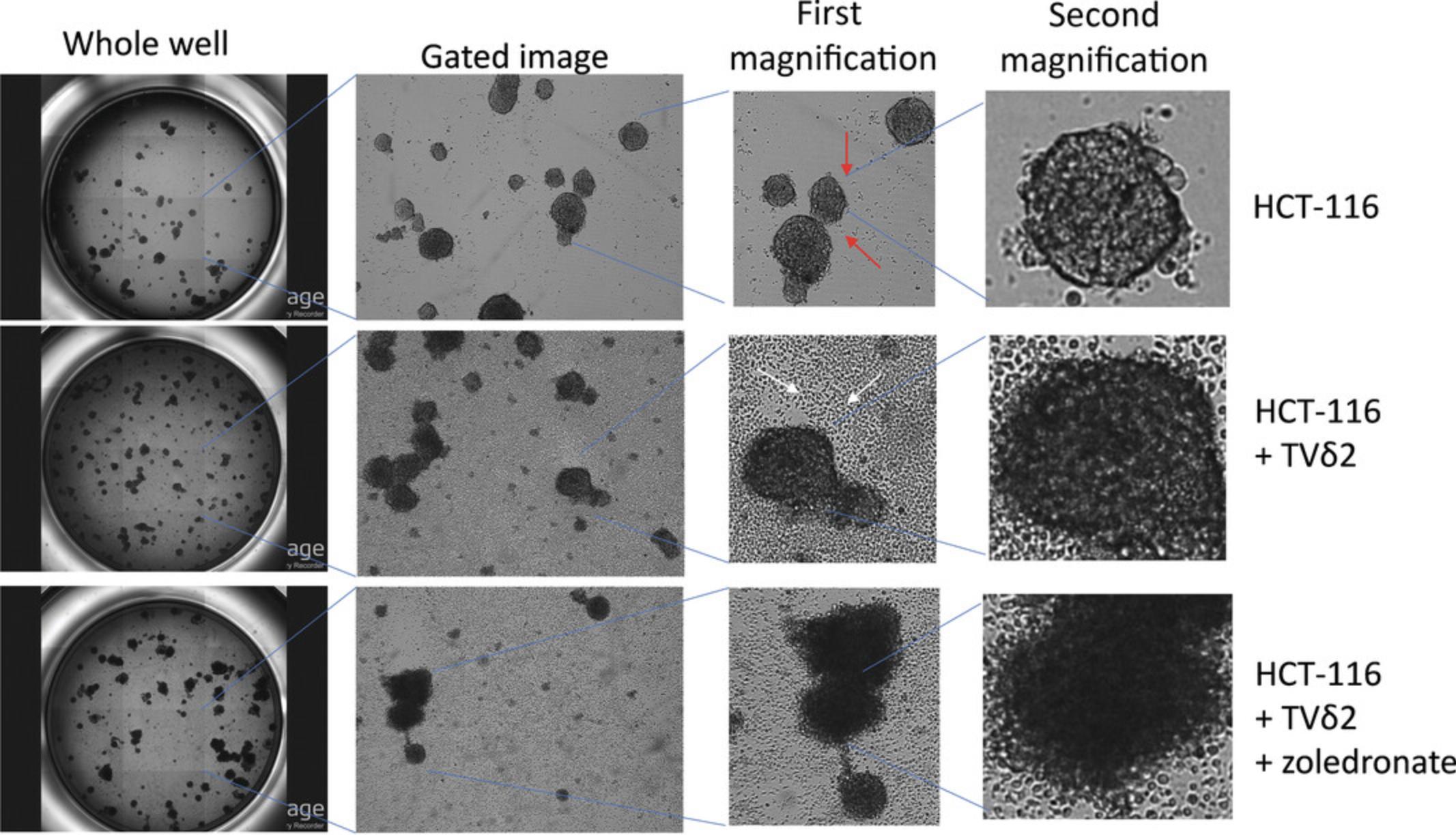
Materials
-
NK cells or Vδ2 T cells (see Basic Protocol 4)
-
RPMI-1640 medium (e.g., Thermo Fisher Scientific, cat. no. 21875091)
-
Tumor cell spheroids (see Basic Protocol 1)
-
5- to 15-ml tube
-
Centrifuge
-
Cell counting chamber (e.g., Biosigma, cat. no. BVS100)
-
37°C, 5% CO2 incubator
-
Microscope
1.Recover NK cells or Vδ2 T cells from 96-well U-bottom plates, and place in a 5- to 15-ml tube.
2.Centrifuge tubes 5 min at 700 × g , room temperature. Discard supernatant and gently resuspend cell pellets in 5 to 10 ml RPMI-1640 medium.
3.Centrifuge tubes as in step 2.
4.Resuspend cells in 5 to 10 ml RPMI-1640 medium and count cells.
5.Adjust cell concentration by diluting the original volume of each tube or by centrifuging the tubes again and resuspending cells at the appropriate cell concentration.
6.Discard 100 µl medium from each well of the spheroid plate, and add 100 µl NK cells or Vδ2 T cells.
7.Incubate plates at 37°C, 5% CO2 for 4 to 48 hr.
8.Monitor cocultures by microscopy and evaluate cytotoxicity (see Basic Protocol 6).
Basic Protocol 6: EVALUATION OF LYMPHOCYTE CYTOTOXICITY USING CRYSTAL VIOLET STAINING
This assay to evaluate cytotoxicity can be applied to both drugs and antitumor lymphocytes (Fig. 3). The basis of this assay is the ability of living tumor cells to adhere to conventional culture wells. The assay evaluates living cells directly, and thus the percentage of dying cells can be evaluated indirectly. Tumor cell spheroids are allowed to adhere to conventional culture plates, which may take several hours. Thus, the degree of cytotoxicity is determined at the end of the coculture experiments plus the time due to adhesion.
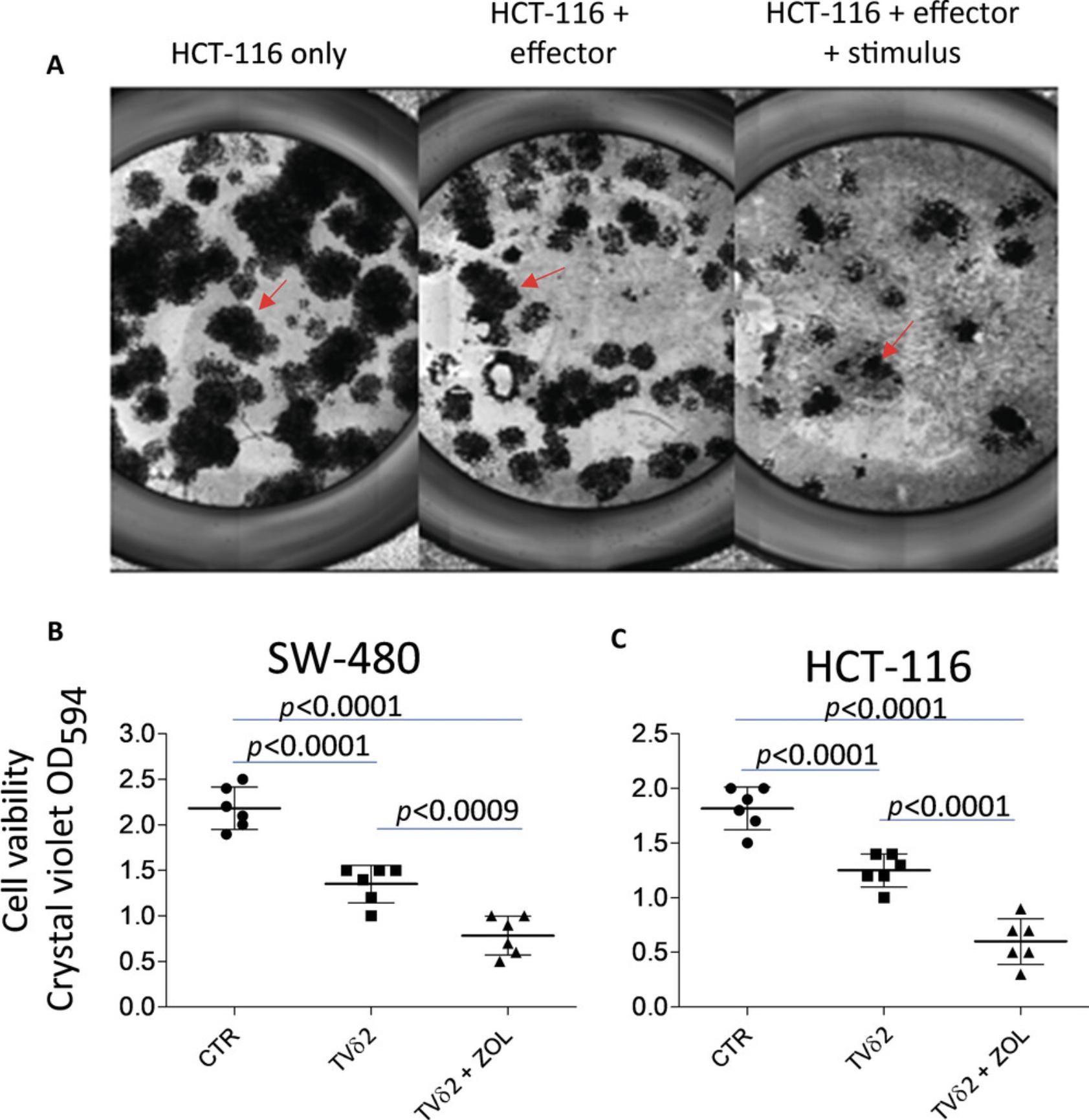
Materials
-
Cocultured cells (see Basic Protocol 5)
-
RPMI-1640 medium (e.g., Thermo Fisher Scientific, cat. no. 21875091)
-
FBS, heat inactivated (e.g., Thermo Fisher Scientific, cat. no. A3160401)
-
DPBS with Ca2+ and Mg2+ (e.g., Thermo Fisher Scientific, cat. no. 14040133)
-
70% ethanol
-
Crystal violet (see recipe)
-
Solubilizing solution (see recipe)
-
96-well culture plate, conventional
-
Cell culture incubator, 37°C, 5% CO2
-
Microscope
-
Plate shaker (optional)
-
Plate reader (e.g., VICTOR Multilabel Plate Reader)
CAUTION : Crystal violet is carcinogenic upon contact and may cause acute oral toxicity. Use appropriate personal protective equipment.
1.Discard 100 µl culture supernatant from each culture well, and mix remaining 100 µl by pipetting medium up and down.
2.Transfer 100 µl cell suspension to a corresponding well of a conventional culture plate.
3.To each well of the conventional culture plate, add 100 µl RPMI-1640 medium supplemented with 10% (v/v) FBS.
4.Incubate plate at 37°C, 5% CO2 until the living cells adhere to the plate, monitoring cultures under a microscope.
5.Gently harvest the total volume (200 µl) of each well, and add 200 µl DPBS with Ca2+ and Mg2+. Gently resuspend each well, and then discard the 200 µl.
6.To each well add 200 µl of 70% ethanol, and incubate plate at 4°C for 30 min.
7.Discard the total volume of each well, and add 100 µl crystal violet. Incubate at 37°C for 30 min.
8.Discard the total volume of each well, and add 200 µl DPBS with Ca2+ and Mg2+. Discard the 200 µl from each well, and repeat this step at least three times.
9.Discard the total volume of each well, and tap plate on paper to eliminate any drop from each well. Move plate to fume hood.
10.To each well add 100 µl solubilizing solution, and put plate on a shaker or gently tap the sides to allow the elution of crystal violet from cells in each well.
11.Measure intensity of the dark blue color using a plate reader at an optical density at 594 nm (OD594; Fig. 3).
REAGENTS AND SOLUTIONS
Each commercially available reagent has a recommended storage temperature and length indicated with the datasheet of that specific reagent. Generally, most reagents used can be kept at 4°C for up to 1 year from the date of receipt. Primary and secondary antibodies are generally stable for several years at the concentrations indicated in the protocols. Cytokines and growth factors should be kept at ‒20°C or at ‒80°C at the original solution as indicated by the datasheet. When diluted these reagents can be kept at 4°C. Avoid repeated freezing and thawing, especially for cytokines at low concentration.
Crystal violet
- 0.2% (w/v) crystal violet (e.g., Millipore Sigma, cat. no. CO775)
- 2% (v/v) ethanol (e.g., Carlo Erba, cat. no. 3086472)
- Distilled water
- Store at 4°C for up to 1 year
Solubilizing solution
- 50% (v/v) ethanol (e.g., Carlo Erba, cat. no. 3086472)
- 0.1% (v/v) acetic acid (e.g., Millipore Sigma, cat. no. A6283)
- Distilled water
- Store at room temperature for up to 1 year
COMMENTARY
Background Information
The generation of spheroids can be achieved by following Basic Protocol 1 using different kinds of ultralow-adherent plates. My laboratory has chosen the indicated plasticware mainly for the relatively low cost and more importantly for ease of handling. Indeed, the use of hanging-drop plates from InSphero was not easy in our hands, and their high cost (about twice to three times ultralow-adherent plates from Corning) was prohibitive. The main disadvantage is that spheroids obtained do not display the same size, and thus cytotoxic effects may be different upon challenge with immune cells. Culture plates coated with agar can also be used, but the strong reproducibility of spheroid generation obtained with ultralow-adherent plates is a major advantage. Evaluation of ATP is the easiest assay to assess viability and growth of spheroids. Of course, evaluation of crystal violet staining is useful as well (Di Mascolo et al., 2019; Sargenti et al., 2020; Varesano et al., 2018).
Critical Parameters
Generation of spheroids (Basic Protocol 1) is optimal when tumor cells are growing well. Indeed, it is better to perform the experiment when cells are growing (50% to 70% confluence in conventional culture wells) and not when they are 100% confluent. It is important to determine in preliminary experiments the optimal number of cells to be seeded for each cell line. Usually, tumor cell spheroids can be used for cytotoxic experiments within 7 days of culture, but 5 days is optimal. It is necessary to seed the same number of cells in each well and to use a multichannel pipette.
In setting up coculture experiments of tumor spheroids and effector cells (Basic Protocol 5), a key step is to add the same number of effector cells to tumor cell spheroids to ensure good replicates. Multichannel pipettes should be used and cell solutions mixed before seeding.
For evaluation of cytotoxicity using crystal violet staining (Basic Protocol 6), it is essential that the transfer of spheroids from the original coculture plates is optimal. Ensure that all cell spheroids have been transferred. Staining should be performed with well-fixed cells, and attention should be paid during washes so as not to detach cells. Addition of solutions and washes should be performed gently using a multichannel pipette. After crystal violet washes, plates should be dried well under a sterile hood for at least 6 hr.
The efficiency of cytolytic antitumor cells is variable among different donors. Thus, it is better to perform experiments using effector cell populations from at least two donors. Furthermore, Vδ2 T cells and NK cells can display different levels of antitumor effect independent of the level of purity of the Vδ2 T cell or NK cell populations, as determined by immunofluorescence with specific antibodies.
The degree of cytotoxic effect of immune cells against CRC cell spheroids can be different from what is detected using CRC target cells as single cells in suspension. Indeed, after a 4-hr incubation the large majority of cells present in spheroids are still living. This is not the case when cytotoxicity is assessed with tumor cells as single cells. This is because, during the killing of tumor cells in a spheroid, effector cells will interact first with the outer layer of tumor cells at the surface of the spheroid and later with tumor cells inside the spheroid. Indeed, effector cells used to kill tumor cells in the spheroid should penetrate tumor cells and alter the compactness of the spheroid itself. Penetration into a tumor mass takes time, and consequently after 4 hr of incubation only a few effector cells will have encountered tumor cells. However, when used as a single-cell suspension, any single tumor cell can interact with one or more effector cells after mixing the two cell populations together. Furthermore, the degree of cytotoxic effect will mainly depend on the ratio of effector cells to target cells because the probability of an effector cell binding to a CRC cell will be greater at higher ratios. Consequently, the cytotoxic effect exerted by the same effector cells can be stronger when assessed in a coculture of single tumor cells compared with that observed with spheroids. In my experience, after 4 hr of incubation the degree of spheroid cytotoxicity is negligible using the three CRC cell lines—DLD-1, SW-480, and HCT-116—whereas an evident effect can be seen after 18 to 24 hr of incubation. It is noteworthy that the compactness of HCT-116 spheroids is lower than that of DLD-1 and SW-480 spheroids. This different physical property leads to a stronger cytotoxic effect using spheroids from HCT-116 cell lines than from DLD-1 or SW-480 cell lines. Altogether, these findings suggest that the killing activity evaluated in a 3D system may be different from what has been described previously in a setting in which epithelial cells are detached from the substrate and used as single target cells.
Troubleshooting
See Table 1 for common problems encountered when following these protocols, their possible causes, and suggested solutions.
Problem | Possible cause | Solution |
---|---|---|
No spheroid generation or cell growth | Intrinsic feature of cell line used | Use DMEM/F12 supplemented with FBS |
DMEM/F12 is not appropriate | Use another medium (e.g., RPMI-1640 medium with or without FBS) | |
Try to generate spheroids in Geltrexa or Matrigel domes or by addition of tissue-specific matrix | ||
No cytotoxic effect of effector cells or cytotoxic drugs | Effector cell population is not cytotoxic | Test effector cells in conventional cytotoxic assay before use in 3D assay |
Error in counting cells | Ensure correct cell number | |
Low effector:tumor spheroid ratio | Increase effector:tumor spheroid ratio | |
Effector cell culture too old or too young | Use effector cells of different culture age | |
Drugs used are not cytotoxic or are inactivated | Add strong stimulus to induce cytotoxicity (e.g., zoledronic acid for Vδ2 T cells, PHA for NK cells, anti-EGFR antibody cetuximab to trigger ADCC) | |
Use drugs diluted from original solution that have been aliquoted and stored at the appropriate temperature | ||
Increase dose | ||
Test drug in conventional cultures | ||
Use cells sensitive to drug described in the literature | ||
Effector cells do not grow well; too few cells are obtained | Medium is not adequate | Select best batch of FBS among several batches |
IL-2 is not good; FBS is not inactivated | Check efficiency of the reagents | |
Cell cultures are improperly split | Carefully follow cell culture protocols |
- ADCC, antibody-dependent cell-mediated cytotoxicity; DMEM, Dulbecco's modified Eagle medium; EGFR, epidermal growth factor receptor; FBS, fetal bovine serum; IL-2, interleukin 2; NK, natural killer; PHA, phytohemagglutinin.
- a For additional details, see https://www.thermofisher.com/us/en/home/life-science/cell-culture/organoids-spheroids-3d-cell-culture/extracellular-matrices-ecm/geltrex-matrix-products.html.
Understanding Results
As evaluation of the magnitude of cytotoxicity is the main focus of the protocols described here (Fig. 3), it should be noted that staining with crystal violet can evaluate viability in addition to cytotoxicity. Thus, results should be shown as the percentage of living cells standardized to control wells according to the following formula: \begin{equation*} \frac{{{\rm{Experimental;O}}{{\rm{D}}{594}}}}{{{\rm{Control;O}}{{\rm{D}}{594}}}} \times {\rm{100}} = % ;{\rm{of}};{\rm{living}};{\rm{cells,}} \end{equation*} where the experimental OD represents the OD of cocultures of spheroids and lymphocytes and the control OD represents the OD obtained in wells with spheroids only.
It is necessary to have a positive control in each experiment to ensure that all reagents are working well. In some instances, wells can be incubated with 1 N HCl to kill all cells, and in this case no cells from spheroids will adhere to the conventional adherent plate. Usually, with descending effector to target cell ratios, a progressive reduction of cytolysis (and thus progressive increase in cell viability and OD594) should be detected.
Statistical analysis
At least three (usually six) replicates for each experimental condition should be performed, and results of cytotoxicity can be analyzed using Student's t -test. The same test can be applied when several independent experiments are performed.
Time Considerations
Basic Protocol 1: Preparation of cell cultures for the generation of spheroids takes <1 hr to culture cells on day 0. It takes about 30 min to detach cells from conventional plasticware. The complexity of the experimental plan is the major determinant of time spent to execute the different protocols described. A total of 3 days is the shortest time necessary to see initial formation of spheroids. Within 5 to 7 days of culture, spheroids are well formed, and they can be used in different protocols by days 8 and 9.
Basic Protocol 2: Evaluation of ATP content takes <30 min, which includes 10 min to transfer spheroids from the original plate to the Optiplate, 2 to 5 min to add the solution for ATP evaluation, and 10 min to analyze luminescence.
Basic Protocol 3: The immunofluorescence assay takes <2 hr from the disaggregation of spheroids to analysis by fluorescence-activated cell sorting. The complexity of the experiment and thus the number of tubes to be prepared is the key element that influences the time spent performing this assay. Each tube is analyzed by a flow cytometer for 1 to 2 min in order to obtain 104 events (i.e., cells).
Basic Protocol 4: Preparation of cultures to generate NK cells takes 2 hr starting from isolated PBMCs. Culture and stimulation of Vδ2 T cells takes 20 min starting from PBMCs. The bulk population of NK cells can be obtained in 15 to 21 days, whereas a high percentage of Vδ2 T cells can be obtained within 14 days of culture.
Basic Protocol 5: Cocultures can be prepared in 30 to 60 min depending on the complexity of the experiment and the number of experimental replicates.
Basic Protocol 6: Transfer of lymphocyte-spheroid cocultures from original ultralow-adherent plates to a conventional plate takes 10 min, and the procedure to stain attached cells after the appropriate incubation takes 2 hr, including solubilization and reading of the color intensity of crystal violet.
Acknowledgments
This study has been supported by the Associazione Italiana per la Ricerca sul Cancro (AIRC) IG-21648, Compagnia del San Paolo (ROL 32567), 5×mille 2015 and 2016, and Ricerca Corrente from the Italian Ministry of Health to A.P
Author Contributions
A. Poggi : conceptualization, funding acquisition, methodology, software, supervision, writing ‒ original draft, writing ‒ review and editing.
Conflict of Interest
The author declares no conflict of interest.
Open Research
Data Availability Statement
The data that support this article are available from the author upon reasonable request.
Literature Cited
- Anderson, D. A. 3rd, Dutertre, C. A., Ginhoux, F., & Murphy, K. M. (2021). Genetic models of human and mouse dendritic cell development and function. Nature Reviews Immunology , 21(2), 101–115. doi: 10.1038/s41577-020-00413-x.
- Bonci, F., Zabogli, E., Conti, F., Merico, A., Freer, G., Bendinelli, M., & Pistello, M. (2009). A novel method for producing target cells and assessing cytotoxic T lymphocyte activity in outbred hosts. BMC Biotechnology , 9, 18. doi: 10.1186/1472-6750-9-18.
- Courau, T., Bonnereau, J., Chicoteau, J., Bottois, H., Remark, R., Assante Miranda, L., … Le Bourhis, L. (2019). Cocultures of human colorectal tumor spheroids with immune cells reveal the therapeutic potential of MICA/B and NKG2A targeting for cancer treatment. Journal for ImmunoTherapy of Cancer , 7, 74. doi: 10.1186/s40425-019-0553-9.
- Dangles-Marie, V., Richon, S., Behi, M. E., Echchakir, H., Dorothée, G., Thiery, J., … Mami-Chouaib, F. (2003). A three-dimensional tumor cell defect in activating autologous CTLs is associated with inefficient antigen presentation correlated with heat shock protein-70 down-regulation. Cancer Research , 63, 3682–3687.
- De Santis, F., Fucà, G., Schadendorf, D., Mantovani, A., Magnani, L., Lisanti, M., … Di Nicola, M. (2021). Anticancer innovative therapy congress: Highlights from the 10th anniversary edition. Cytokine & Growth Factor Reviews, 59, 1‒8. doi: 10.1016/j.cytogfr.2021.02.001.
- Di Mascolo, D., Varesano, S., Benelli, R., Mollica, H., Salis, A., Zocchi, M. R., … Poggi, A. (2019). Cancers nanoformulated zoledronic acid boosts the Vδ2 T cell immunotherapeutic potential in colorectal cancer. Cancers , 12(1), 104. doi: 10.3390/cancers12010104.
- Elsner, L., & Dressel, R. (2020). 51Cr-release to monitor NK cell cytotoxicity. Methods in Enzymology , 631, 497–512. doi: 10.1016/bs.mie.2019.05.037.
- Goldberg, J. E., Sherwood, S. W., & Clayberger, C. (1999). A novel method for measuring CTL and NK cell-mediated cytotoxicity using annexin V and two-color flow cytometry. Journal of Immunological Methods , 224(1-2), 1–9. doi: 10.1016/s0022-1759(98)00038-6.
- Hahn, W. C., Bader, J. S., Braun, T. P., Califano, A., Clemons, P. A., Druker, B. J., … Gerhard, D. S. (2021). An expanded universe of cancer targets. Cell , 184(5), 1142–1155. doi: 10.1016/j.cell.2021.02.020.
- Huang, L., Bockorny, B., Paul, I., Akshinthala, D., Frappart, P. O., Gandarilla, O., … Muthuswamy, S. K. (2020). PDX-derived organoids model in vivo drug response and secrete biomarkers. JCI Insight , 5(21), e135544. doi: 10.1172/jci.insight.135544.
- Ito, R., Takahashi, T., & Ito, M. (2018). Humanized mouse models: Application to human diseases. Journal of Cellular Physiology , 233(5), 3723–3728. doi: 10.1002/jcp.26045.
- Lee, M. W., Miljanic, M., Triplett, T., Ramirez, C., Aung, K. L., Eckhardt, S. G., & Capasso, A. (2021). Current methods in translational cancer research. Cancer and Metastasis Reviews , 40, 7–30. doi: 10.1007/s10555-020-09931-5.
- Martinov, T., McKenna, K. M., Tan, W. H., Collins, E. J., Kehret, A. R., Linton, J. D., … Rongvaux, A. (2021). Building the next generation of humanized hemato-lymphoid system mice. Frontiers in Immunology , 12, 643852. doi: 10.3389/fimmu.2021.643852.
- Mestas, J., & Hughes, C. C. (2004). Of mice and not men: Differences between mouse and human immunology. Journal of Immunology , 172(5), 2731–2738. doi: 10.4049/jimmunol.172.5.2731.
- Nishino, M., Ramaiya, N. H., Hatabu, H., & Hodi, F. S. (2017). Monitoring immune-checkpoint blockade: Response evaluation and biomarker development. Nature Reviews Clinical Oncology , 14(11), 655–668. doi: 10.1038/nrclinonc.2017.88.
- Sargenti, A., Musmeci, F., Bacchi, F., Delprete, C., Cristaldi, D. A., Cannas, F., … Poggi, A. (2020). Physical characterization of colorectal cancer spheroids and evaluation of NK cell infiltration through a flow-based analysis. Frontiers in Immunology , 11, 564887. doi: 10.3389/fimmu.2020.564887.
- Sherman, H., Gitschier, H. J., & Rossi, A. E. (2018). A novel three-dimensional immune oncology model for high-throughput testing of tumoricidal activity. Frontiers in Immunology , 9, 857. doi: 10.3389/fimmu.2018.00857.
- Siozopoulou, V., Domen, A., Zwaenepoel, K., Van Beeck, A., Smits, E., Pauwels, P., & Marcq, E. (2021). Immune checkpoint inhibitory therapy in sarcomas: Is there light at the end of the tunnel? Cancers , 13(2), 360. doi: 10.3390/cancers13020360.
- Vacca, P., Chiossone, L., Mingari, M. C., & Moretta, L. (2019). Heterogeneity of NK cells and other innate lymphoid cells in human and murine decidua. Frontiers in Immunology , 10, 170. doi: 10.3389/fimmu.2019.00170.
- Varesano, S., Zocchi, M. R., & Poggi, A. (2018). Zoledronate triggers Vδ2 T cells to destroy and kill spheroids of colon carcinoma: Quantitative image analysis of three-dimensional cultures. Frontiers in Immunology , 9, 998. doi: 10.3389/fimmu.2018.00998.
- Xiao, Q., Nobre, A., Piñeiro, P., Berciano-Guerrero, M. Á., Alba, E., Cobo, M., … Barragán, I. (2020). Genetic and epigenetic biomarkers of immune checkpoint blockade response. Journal of Clinical Medicine , 9(1), 286. doi: 10.3390/jcm9010286.
- Zhou, L., Xu, N., Shibata, H., Saloura, V., & Uppaluri, R. (2021). Epigenetic modulation of immunotherapy and implications in head and neck cancer. Cancer and Metastasis Reviews , 40(1), 141–152. doi: 10.1007/s10555-020-09944-0.
Citing Literature
Number of times cited according to CrossRef: 1
- Shih‐Han Wang, Te‐An Lee, Chun‐Tse Kuo, Yun‐Ju Lai, Chia‐Wei Li, γδ T cells in 3D culture: The potential use in the therapeutics development, Clinical and Translational Discovery, 10.1002/ctd2.75, 2 , 2, (2022).