FFPE-ATAC: A Highly Sensitive Method for Profiling Chromatin Accessibility in Formalin-Fixed Paraffin-Embedded Samples
Ram Prakash Yadav, Ram Prakash Yadav, Vamsi Krishna Polavarapu, Vamsi Krishna Polavarapu, Pengwei Xing, Pengwei Xing, Xingqi Chen, Xingqi Chen
chromatin accessibility
formalin-fixed paraffin-embedded (FFPE) sample
in vitro transcription
T7-Tn5-mediated transposition
Abstract
In basic and translational cancer research, the majority of biopsies are stored in formalin-fixed paraffin-embedded (FFPE) samples. Chromatin accessibility reflects the degree to which nuclear macromolecules can physically interact with chromatinized DNA and plays a key role in gene regulation in different physiological conditions. As such, the profiling of chromatin accessibility in archived FFPE tissue can be critical to understanding gene regulation in health and disease. Due to the high degree of DNA damage in FFPE samples, accurate mapping of chromatin accessibility in these specimens is extremely difficult. To address this issue, we recently established FFPE-ATAC, a highly sensitive method based on T7-Tn5-mediated transposition followed by in vitro transcription (IVT), to generate high-quality chromatin accessibility profiles with 500–50,000 nuclei from a single FFPE tissue section. In FFPE-ATAC, which we describe here, the T7-Tn5 adaptors are inserted into the genome after FFPE sample preparation and are unlikely to sustain the DNA breakage that occurs during reverse cross-linking of these samples. It should, therefore, remain at the ends of broken accessible chromatin sites after reverse cross-linking. IVT is then used to convert the two ends of the broken DNA fragments to RNA molecules before making sequencing libraries from the IVT RNAs and further decoding Tn5 adaptor insertion sites in the genome. Through this strategy, users can decode the flanking sequences of the accessible chromatin even if there are breaks between adjacent pairs of T7-T5 adaptor insertion sites. This method is applicable to dissecting chromatin profiles of a small section of the tissue sample, characterizing stage and region-specific gene regulation and disease-associated chromatin regulation in FFPE tissues. © 2022 The Authors. Current Protocols published by Wiley Periodicals LLC.
Basic Protocol 1 : Nuclei isolation from FFPE tissue samples
Basic Protocol 2 : T7-Tn5 transposase tagmentation, reverse-crosslinking, and in vitro transcription
Basic Protocol 3 : Preparation of libraries for high-throughput sequencing
INTRODUCTION
Understanding chromatin structure is critical to improving our understanding of the molecular mechanisms of human disease (Corces et al., 2018; Lu et al., 2022; Qu et al., 2017). Chromatin accessibility reflects the degree to which nuclear macromolecules can physically contact chromatinized DNA and plays a key role in gene regulation in different physiological conditions (Klemm, Shipony, & Greenleaf, 2019). Thus, decoding landscapes of chromatin regulatory elements in human disease is of critical importance for potential preclinical diagnosis and treatment (Corces et al., 2018; Qu et al., 2017).
A series of chromatin structure profiling technologies, including chromatin immunoprecipitation (ChIP) (Johnson, Mortazavi, Myers, & Wold, 2007), formaldehyde-assisted isolation of regulatory elements (FAIRE) (Giresi, Kim, McDaniell, Iyer, & Lieb, 2007), micrococcal nuclease (MNase) assays of chromatin (Zaret, 2005), cleavage under targets and release using nuclease (CUT&RUN) (Skene, Henikoff, & Henikoff, 2018), cleavage under targets and tagmentation (CUT&Tag) (Kaya-Okur et al., 2019), and many others (Bartlett, Dileep, Henikoff, & Gilbert, 2021; Carter et al., 2019; Handa et al., 2020; Harada et al., 2019; Skene et al., 2018; Skene & Henikoff, 2017; Wang et al., 2019) have been used to generate large-scale chromatin profiles, in both basic research and preclinical studies (Cejas et al., 2016; Corces et al., 2018; Jin et al., 2015; Landt et al., 2012). In addition, recently developed technologies, such as the assay for transposase-accessible chromatin by sequencing (ATAC-seq) (Buenrostro, Giresi, Zaba, Chang, & Greenleaf, 2013) and DNase I hypersensitivity sequencing (DNase-seq) (Jin et al., 2015), allow the profiling of chromatin accessibility in cells and frozen tissues with high sensitivity (Corces et al., 2017).
Formalin fixation followed by paraffin embedding (FFPE) is a routine method for long-term preservation of most archived pathological and cancer samples (Gaffney, Riegman, Grizzle, & Watson, 2018). Due to the high degree of DNA damage in FFPE samples, however, accurate mapping of chromatin accessibility in these specimens is extremely difficult. To overcome these issues with the use of FFPE tissues, we recently established FFPE-ATAC (Zhang et al., 2022), a highly sensitive method based on T7-Tn5-mediated transposition followed by in vitro transcription (IVT), to generate high-quality chromatin accessibility profiles with 500 nuclei from a single FFPE tissue section (Fig. 1). In FFPE-ATAC, the T7-Tn5 adaptors are inserted into the genome after FFPE sample preparation and are unlikely to sustain the DNA breakage that occurs during reverse cross-linking of FFPE samples, and should, therefore, remain at the ends of broken accessible chromatin sites after reverse cross-linking. IVT is then used to convert the two ends of the broken DNA fragments to RNA molecules before making sequencing libraries from the IVT RNAs and decoding the Tn5 adaptor insertion sites in the genome. Through this strategy, users can decode the flanking sequences of the accessible chromatin even if there are breaks between adjacent pairs of T7-T5 adaptor insertion sites. FFPE-ATAC is a simple method that requires low sample input to generate a high-quality sequencing library.
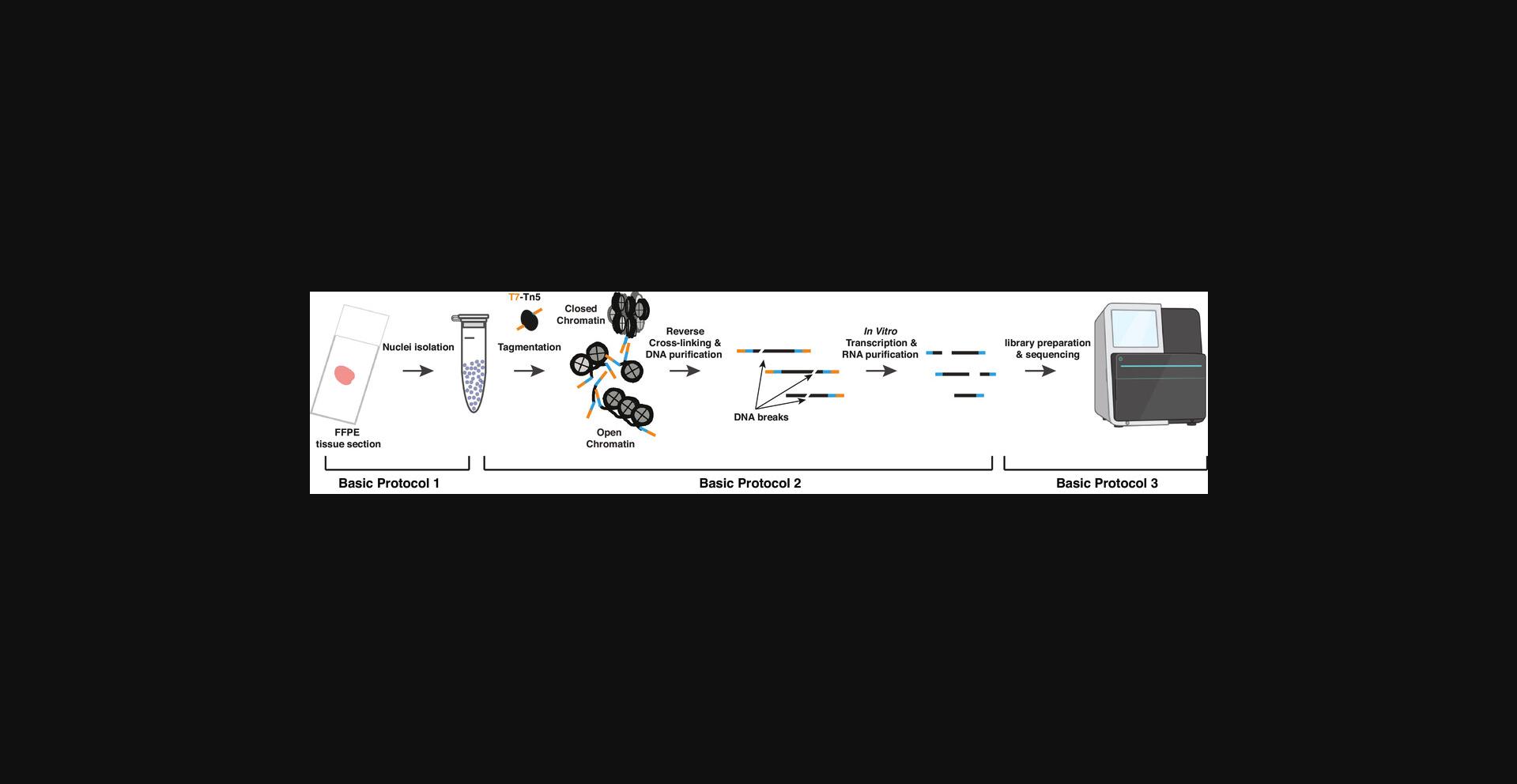
In this article, we describe the FFPE-ATAC procedure, divided into three Basic Protocols (Fig. 1). Basic Protocol 1 describes the steps for isolating nuclei from the FFPE tissue section, using microdissection and collagenase and hyaluronidase digestion. Basic Protocol 2 outlines the steps for T7-Tn5 tagmentation, reverse-crosslinking, and IVT on the intact nuclei isolated from Basic Protocol 1, followed by IVT of the RNA molecules from accessible chromatin sites. Basic Protocol 3 describes the preparation of sequencing libraries. This article does not cover the downstream analysis of sequenced libraries, which is outside the scope of this article. A description of the most up-to-date analyses and tools can be found elsewhere (Zhang et al., 2022).
STRATEGIC PLANNING
To avoid cross contamination, wipe and clean the work surface with 70% v/v ethanol. For Basic Protocol 1, take an FFPE block prepared using standard methods and cut one 10–20-μm thick FFPE tissue section using a microtome (Zhang et al., 2022). The tissue section can be stored at 4°C for short-term use and −20°C for long-term use. To get good sections with no tissue breakage, place pre-soaked tissue paper with sterilized water on top of the tissue block at 4°C before sectioning it.
Glassware, 1× PBS (PH 7.4), and water should be autoclaved and stored at room temperature. Before starting the experiments, prepare a working dilution of collagenase (6 mg/ml) and hyaluronidase (600 units/ml) in 1× PBS with 0.5 mM CaCl2, and pass through a 0.22-μm filter to remove any impurities. Keep on ice, then prepare the collagenase and hyaluronidase cocktail as described in REAGENTS AND SOLUTIONS.
If the isolated nuclei from the FFPE tissue sections have been stored at 4°C for 1–2 weeks, count them again with a cell counter just before starting the experiment. For Basic Protocols 2 and 3, prepare 2× dialysis buffer, 2× TD buffer, 2× reverse crosslinking buffer, and Tn5/T7-Tn5 transposase assembly buffers (see REAGENTS AND SOLUTIONS), and store these reagents at −20°C.
Users should ensure they have obtained all the necessary permissions and ethical approvals for work with human tissue samples.
Basic Protocol 1: NUCLEI ISOLATION FROM FFPE TISSUE SAMPLES
This protocol describes the procedure to isolate intact nuclei from FFPE tissue sections. It involves removal of the paraffin from the FFPE section with xylene, hydration of the tissue section with a series of different concentrations of ethanol (EtOH), mechanical tissue microdissection, enzymatic digestion with a collagenase and hyaluronidase mix, disaggregation of tissue to obtain single-nuclei suspension, and filtration to remove extracellular matrices. The isolated nuclei can then be stored at 4°C before continuing with Basic Protocol 2.
Materials
-
FFPE tissue sections (10–20 µm thick, see Strategic Planning)
-
Xylene (HistoLab, cat. no. 02070)
-
Ethanol (VWR, cat. no. VWRC20816.552)
-
Sterilized water
-
1× PBS, pH 7.4 (Thermo Fisher Scientific, cat. no 10010023)
-
1 M CaCl2 (Alfa Aesar, cat. no. J63122)
-
Collagenase (Sigma-Aldrich, cat. no. C9263)
-
Hyaluronidase (Merck Millipore, cat. no. HX0154-1)
-
NST buffer (see recipe)
-
Sodium azide (Merck Millipore, cat. no. 26628-22-8)
-
Hoechst (Thermo Fisher Scientific, cat. no 33342)
-
FBS (Thermo Fisher Scientific, cat. no. 10108-105)
-
BSA (Miltenyi Biotech MACS, cat. no. 130-091-376)
-
RNase A (Thermo Fisher Scientific, cat. no. EN0531)
-
Ampicillin (Serva, cat. no. 69-52-3)
-
IGEPAL CA-630 (Sigma-Aldrich, cat. no. 13021-50)
-
5 M NaCl (Thermo Fisher Scientific, Invitrogen, cat. no. AM9759)
-
1 M Tris·HCl, pH 7.5 (Thermo Fisher Scientific, cat. no. 15567-027)
-
1 M Tris·HCl pH 8 (Thermo Fisher Scientific, cat. no. 15568-025)
-
1 M MgCl2 (Thermo Fisher Scientific, Invitrogen, cat. no. AM9530G)
-
Nuclease-free water (Invitrogen, cat. no. AM9932)
-
Nail polish
-
Microtome (Leica, Histo Core MULTICUT semi-automated rotary microtome)
-
50-ml Falcon tubes (Sarstedt, cat. no. 62.559.001)
-
Sterilized glass 100-mm Petri plate
-
21G needles (BD Microlance, cat. no. ND432)
-
1.5-ml Lo-bind tubes (Sarstedt, cat. no. 72.706.600)
-
27G needles (BD Microlance, cat. no. 302200)
-
1-ml syringe (BD Microlance, cat. no. 309628)
-
30-μm filter (Miltenyi Biotech MACS, cat. no. 130-098-458)
-
Cell counter (Life Technologies, cat. no. AMQAF1000)
-
Cellspin I (Tharmac, cat. no. 105-12-EL)
-
Glass slides
-
Stereomicroscope
-
Incubator
-
SlowFade™ Gold Antifade Mountant (Invitrogen, cat. no: S36936)
Isolation of nuclei from FFPE tissue sections
1.For deparaffinization and rehydration, place the FFPE section (10–20 μm thick) in a 50-ml Falcon tube filled with 2 ml xylene. Repeat 3 times, each for 5 min.
2.Transfer the FFPE section to a 50-ml Falcon tube with 2 ml of 100% EtOH for 5 min, and repeat once.
3.Transfer the tissue section sequentially into another 50-ml Falcon tube containing 96%, 70%, 50%, and 30% EtOH, one per chamber, each for 5 min. Finally, incubate the tissue in sterile water for 5 min.
4.After rehydration, transfer the tissue to a 50-ml Falcon tube with 2 ml PBS containing 0.5 mM CaCl2. Then, transfer the section to a sterilized glass Petri plate for tissue microdissection. Dissect the section into small pieces with two 21G needles under a stereomicroscope.
5.Collect the fragmented tissue in a 1.5-ml tube and centrifuge at 3000 × g for 10 min at room temperature.
6.Discard supernatant. Resuspend the pellet with 1 ml of collagenase and hyaluronidase cocktail (see REAGENTS AND SOLUTIONS) and incubate for 16 hr at 37°C.
7.After incubation, dilute the enzymatic mix by adding 400 μl NST buffer. Centrifuge the mixture at 3000 × g for 10 min at room temperature.
8.Aspirate the supernatant, resuspend the pellet in 800 μl of NST buffer, containing 10% fetal bovine serum (FBS) and 0.1% DNase-free RNase A, and pass through a 27G needle syringe into 1.5-ml Eppendorf tube at least 30 times for disaggregation. Centrifuge the mixture at 3000 × g for 10 min at room temperature. Aspirate and discard the supernatant.
9.Resuspend the nuclei pellet with 800 μl of NST buffer and filter twice using a 30-µm filter into a 1.5-ml Eppendorf tube. Centrifuge at 3000 × g for 10 min at room temperature. Finally, resuspend the nuclei pellet in 500 μl PBS containing 0.05 μg of sodium azide.
10.Count isolated nuclei using a cell counter (use 10 µl) and also take 10 µl to stain the nuclei. To stain, mix the 10 µl aliquot with 490 µl PBS containing 0.5 µl Hoechst (Stock concentration: 10 mg/ml) and incubate for 10 min at room temperature. Then, use Cellspin I to centrifuge at 600 × g for 5 min, to transfer the nuclei onto the glass slide. Air dry and place a drop of SlowFade™ Gold Antifade Mountant. Seal the edges of the glass slide with nail polish. Finally, visualize the DAPI signal under the microscope (Fig. 2). Purified nuclei can be used directly for Basic Protocol 2 or stored at 4°C for 1–2 weeks.
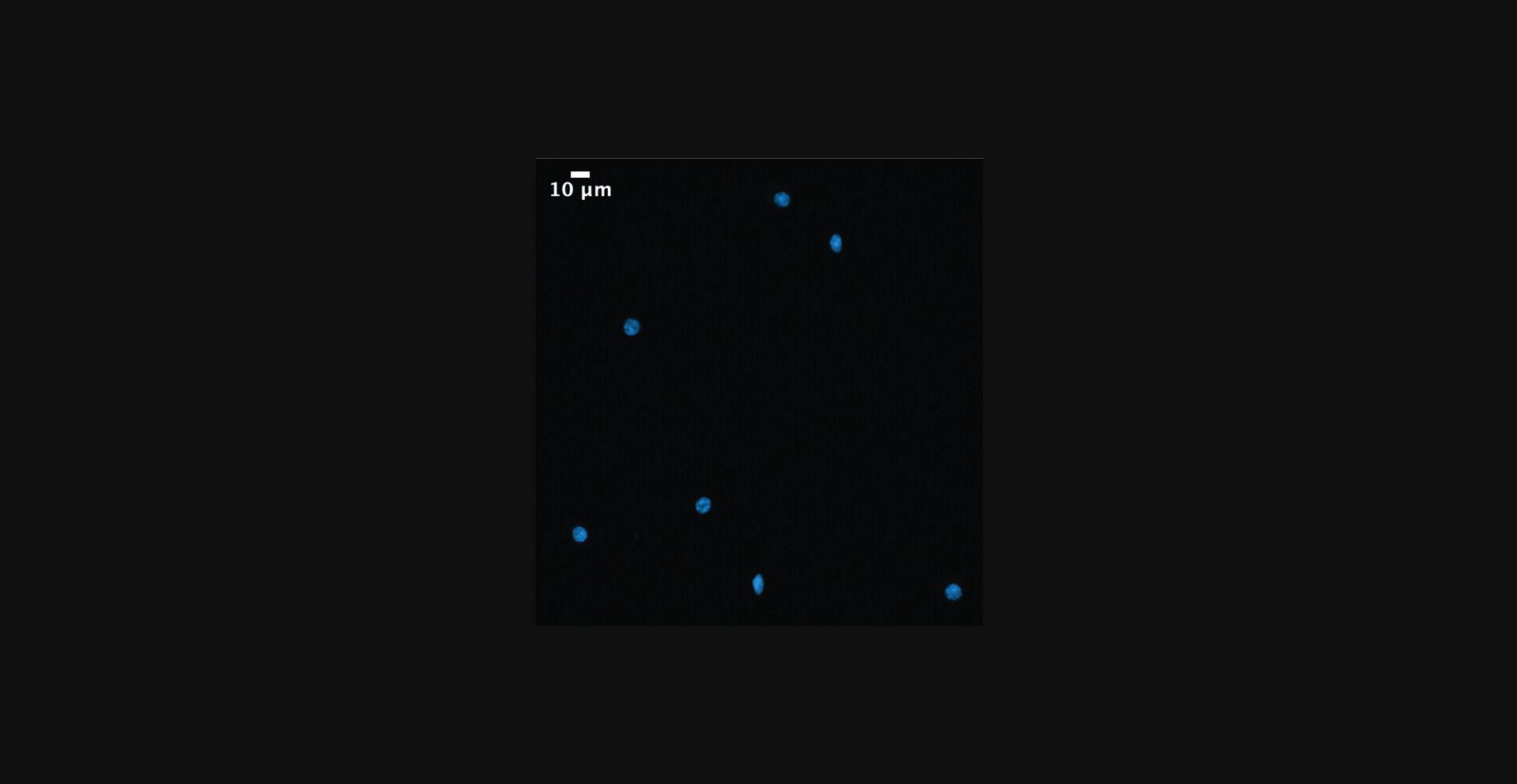
Basic Protocol 2: T7-Tn5 TRANSPOSASE TAGMENTATION, REVERSE-CROSSLINKING, AND IN VITRO TRANSCRIPTION
This protocol describes the steps from T7-Tn5 transposase tagmentation until purification of in vitro transcribed RNA. T7-Tn5 transposase is used to insert the T7 promoter sequence into the accessible regions of the chromatin from the nuclei purified in Basic Protocol 1.After tagmentation, nuclei are digested with proteinase K, followed by reverse crosslinking, and the RNA is then in vitro transcribed with T7 RNA Polymerase to produce high quality RNA molecules for library preparation for high-throughput sequencing. Purified RNA can then be stored at −80°C before continuing with Basic Protocol 3.
Materials
-
Purified nuclei (Basic Protocol 1)
-
1.5-ml Low-bind tubes (Sarstedt, cat. no. 72.706.600)
-
Proteinase K (Thermo Fisher Scientific, cat. no. EO0491)
-
Qiagen MinElute PCR purification kit (Qiagen, cat. no. 28004)
-
NEBNext high-fidelity 2× PCR master mix (New England Biolabs, cat. no. M0541S)
-
SPRIselect beads (Beckman Coulter, cat. no. B23317)
-
T7 high yield RNA synthesis kit (New England Biolabs, cat. no. E2040S)
-
Zymo RNA purification kit (Zymo Research, cat. no. R1013)
-
Nuclease-free water (Invitrogen, cat. no. AM9932)
-
1 M Tris·HCl, pH 7.5, [Invitrogen, cat. no. 15567-027]
-
IGEPAL CA-630 (Sigma-Aldrich, cat. no. 13021-50)
-
DTT (Thermo Fisher Scientific, cat. no. 20291)
-
0.5 M EDTA (Thermo Fisher Scientific, Invitrogen, cat. no. AM9260G)
-
5 M NaCl (Thermo Fisher Scientific, Invitrogen, cat. no. AM9759)
-
1 M MgCl2 (Thermo Fisher Scientific, Invitrogen, cat. no. AM9530G)
-
100% glycerol (Sigma-Aldrich, cat. no. G9012)
-
HEPES (Sigma-Aldrich, cat. no. H3375)
-
KOH (Sigma-Aldrich, cat. no. 484016)
-
Triton X-100 (Sigma-Aldrich, cat. no. T8787)
-
Tn5 transposase stock preparation (see recipe)
-
T7-Tn5 transposase stock preparation (see recipe)
-
Lysis buffer (see recipe)
-
2× TD buffer (see recipe)
-
2× reverse cross-linking buffer (see recipe)
-
40% Acrylamide:bis-acrylamide (Invitrogen, cat. no. HC2040)
-
10% Ammonium persulfate (Invitrogen, cat. no. HC2005)
-
TEMED (Invitrogen, cat. no. HC2006)
-
10× TBE Buffer (Thermo Fisher Scientific, cat. no. B52)
-
6× Loading Dye (Thermo Fisher Scientific, cat. no. R0611)
-
2× TD buffer (see recipe)
-
Human genomic DNA (Promega, cat. no. G3041)
-
NanoDrop 3000 (Thermo Fisher Scientific, cat. no. ND-3300)
-
SeaKem® LE Agarose (Lonza, cat. no. 50004)
-
ThermoMixer (Eppendorf™ F1.5 Model, cat. no. 5384000012)
-
DynaMag™-2 magnetic rack (Thermo Fisher scientific, cat. no. 12321D)
Tn5/T7-Tn5 transposase activity assay
1.Check Tn5/T7-Tn5 transposase activity. To do this, prepare the following reaction mix:
Reagents | Volume |
2× TD buffer | 10 μl |
Human genomic DNA | 50 ng |
Assembled Tn5 or T7-Tn5 (2 μM) | 1 μl |
Sterile water | Up to 20 μl/tube |
2.Incubate the mixture at 55°C for 7 min. After incubation, purify using the Qiagen MinElute PCR Purification kit (following the manufacturer's instructions) and elute in 10 μl of elution buffer. Finally, mix the solution with 2 μl of 6× loading dye and run on a 1% agarose gel to check the size of the tagmented DNA against a DNA ladder.
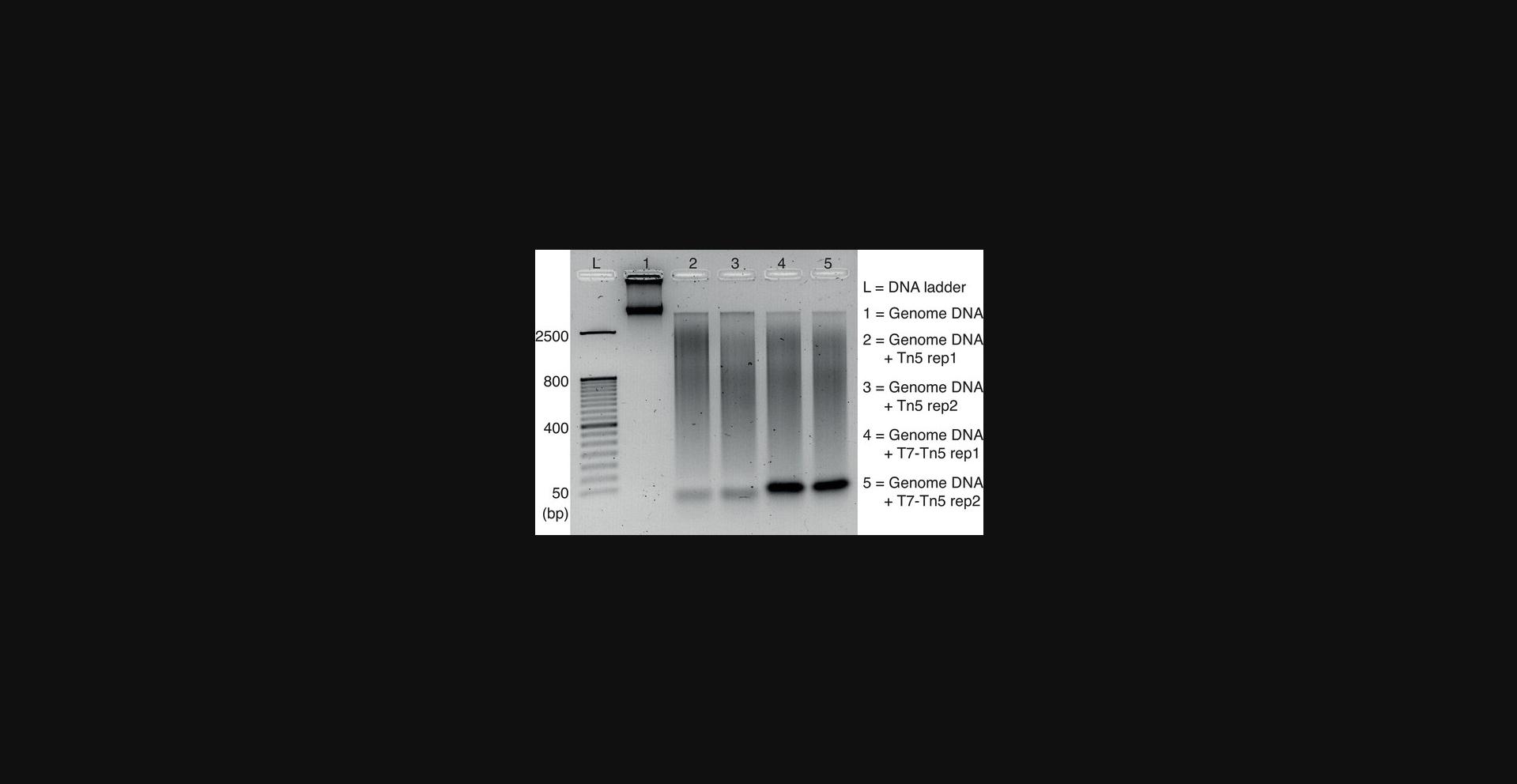
T7-Tn5 transposase tagmentation
3.After checking T7-Tn5 transposase activity, transfer the required number of isolated FFPE nuclei from Basic Protocol 1 (50,000 nuclei) to a new 1.5-ml tube and spin down the nuclei at 2800 × g for 5 min at RT. Discard the supernatant.
4.Resuspend the nuclei pellet with 50 µl lysis buffer (see recipe) and immediately centrifuge at 2800 × g for 10 min at RT. Discard the supernatant.
5.Add 50 µl of transposase master mixture (25 µl of 2× TD buffer, 22.5 µl nuclease-free water, and 2.5 µl of 2 µM T7-Tn5) to the pellet and resuspend. Incubate for 30 min at 37°C.
Reverse-crosslinking
6.After incubation, directly add 50 µl of 2× reverse cross-linking buffer and 1 µl Proteinase K to the tagmentation reaction mixture. Incubate overnight at 65°C with 1200 rpm shaking in a ThermoMixer.
7.Purify the DNA using a MinElute PCR purification kit following the manufacturer's instructions, and elute in 20 µl elution buffer.
Gap filling
8.After DNA purification, transfer the sample to a 0.2-ml PCR tube and add an equal volume (20 µl) of NEBNext high-fidelity 2× PCR master mix. Then, incubate the reaction mixture for 5 min at 72°C in a thermal cycler. Purify the sample using the Qiagen MinElute PCR Purification kit following the manufacturer's instructions, and elute in 50 µl elution buffer.
9.To purify the samples and remove fragments shorter than 150 bp, add 50 µl of SPRI beads, followed by incubation at 15 min at RT.
10.After incubation, transfer the tubes to a magnetic rack for 10 min. Wash the beads twice with 150 μl of 80% v/v ethanol, and air dry for 10 min at RT by leaving tubes on the magnetic rack.
11.Once air dry, remove the tubes from the magnetic rack, resuspend the beads in 25 µl nuclease-free water, and incubate for 10 min at RT.
12.Place the tube on the magnetic rack for 10 min and carefully transfer the supernatant to a new 1.5-ml tube. Place the tube on ice.
In vitro transcription
13.Perform in vitro transcription (IVT) with a T7 RNA synthesis kit (New England Biolabs) by mixing the following reagents: 10 µl template DNA (from Step 12), 1 µl of each ATP/CTP/UTP/GTP, 1 µl T7 mix, and 3 µl nuclease-free water.
14.Incubate the IVT mixture (20 µl) at 37°C overnight (12–16 hr). Purify the RNA using a ZYMO DNase RNA purification kit following the manufacturer's instructions and elute in 15 μl nuclease-free water.
15.Measure the RNA concentration using NanoDrop and proceed to Basic Protocol 3 or immediately store at −80°C.
Basic Protocol 3: PREPARATION OF LIBRARIES FOR HIGH-THROUGHPUT SEQUENCING
The goal of the protocol is to prepare Illumina sequencing libraries using the IVT RNA molecules from Basic Protocol 2.
Random hexamer primers are used to produce single-stranded cDNAs from the purified IVT transcripts generated in Basic Protocol 2, followed by dsDNA synthesis. A second tagmentation is performed with standard Tn5 transposase, which inserts its two DNA adaptors into the dsDNA. Each DNA sequencing library is amplified using a unique forward (i5) and reverse primer (i7) (Buenrostro et al., 2015) combination. Then, users will run a PAGE gel, isolate and purify the DNA (220–1000 bp) from the gel, and run it through a high-sensitivity DNA Bioanalyzer chip, to check DNA size distribution and quantify the DNA concentration of the DNA library.
Materials
-
IVT-transcribed RNA from Basic Protocol 2
-
Random Hexamer Primer (Thermo Fisher Scientific, cat. no. SO142)
-
SMART MMLV kit (TAKARA, cat. no. 639524)
-
Nuclease-free water (Invitrogen, cat. no. AM9932)
-
RNase H (Thermo Fisher Scientific, cat. no. EN0201)
-
RNA XP beads (Beckman Coulter, cat. no. A63987)
-
NEBNext high-fidelity 2× PCR master mix (New England Biolabs, cat. no. M0541S)
-
i5 and i7 primer sets (Buenrostro et al., 2015, see Supporting Information)
-
Qiagen MinElute PCR purification kit (Qiagen, cat. no. 28004)
-
2× TD buffer (see recipe)
-
Reagents to prepare 8% acrylamide gel (see recipe)
-
6× Loading Dye (Thermo Fisher Scientific, cat. no. R0611)
-
50 bp DNA ladder (Invitrogen, cat. No. 10416014)
-
RNase Inhibitor (Thermo Fisher Scientific, cat. no. N8080119)
-
1 M Tris·HCl, pH 7.5 (Thermo Fisher Scientific, Invitrogen, cat. no. 15567-027)
-
1 M MgCl2 (Thermo Fisher Scientific, Invitrogen, cat. no. AM9530G)
-
Dimethylformamide (Sigma-Aldrich, cat. no. D4551)
-
10% SDS (Thermo Fisher Scientific, Invitrogen, cat. no. 1553-035)
-
5 M NaCl (Thermo Fisher Scientific, Invitrogen, cat. no. AM9759)
-
0.5 M EDTA (Thermo Fisher Scientific, Invitrogen, cat. no. AM9260G)
-
21G needles (BD Microlance, cat. no. ND432)
-
Crush soak buffer (see recipe)
-
Zymo ChIP DNA clean and concentrator kit (Zymo Research, cat. no. D5205)
-
40% Acrylamide:bis-acrylamide (Invitrogen, cat. no. HC2040)
-
10% Ammonium persulfate (Invitrogen, cat. no. HC2005)
-
TEMED (Invitrogen, cat. no. HC2006)
-
Human genomic DNA (Promega, cat. no. G3041)
-
Agilent High Sensitivity DNA kit (Agilent, cat. no. 5067-4626)
-
SYBRTM Gold (Invitrogen, cat. no. S11494)
-
10× TBE buffer (Thermo Fisher Scientific, cat. no. B52)
-
Costar spin-X centrifuge tube filters (Coster, cat. no. 8162)
-
Agilent 2100 Bioanalyzer Instrument (Agilent, cat. no. G2939BA)
-
Polyacrylamide gel electrophoresis Unit XCell SureLock™ Mini-Cell (Invitrogen, cat. no. EI0001)
-
DynaMaqTM-2 magnetic rack (Thermo Fisher Scientific, cat. no. 12321D)
Reverse transcription
1.Transfer 100 ng of RNA from the sample generated in Basic Protocol 2 to a fresh tube. Mix sample with 1 μl of 100 μM random hexamer primer stock and add nuclease-free water to a final volume of 11 μl. Heat the mixture at 70°C for 3 min, and immediately cool on ice.
2.Centrifuge the sample briefly, then add 4 μl of 5× First-Strand Buffer, 2 μl of dNTP Mix, 2 μl of 100 mM DTT, 0.5 µl of RNase Inhibitor (20 U/μl), and 0.5 μl SMART MMLV RT. Mix the reaction by gently pipetting up and down. Incubate the tube at 42°C for 60 min and then at 70°C for 15 min.
3.After reverse transcription, directly add 2.2 µl of 10× RNase H buffer and 0.2 µl RNase H (5 U/µl) to each reaction. Incubate the mixture at 37°C for 20 min.
4.Next, use Agent Coulter RNA XP beads to purify the cDNA reaction mixture. Add nuclease-free water to bring the sample volume up to 40 μl. Then, add 72 μl (i.e., 1.8× ratio) of Agent Coulter RNA XP beads and mix gently. Incubate the mixture at RT for 15 min.
5.After incubation, transfer the tubes to a magnetic rack and incubate for 10 min. Wash the beads twice with 200 μl of 70% v/v ethanol, keeping the tube on the magnetic rack. Air dry the beads at RT for 10 min.
6.After drying, remove the tube from the magnetic rack, resuspend the beads with 24.2 μl of elution buffer, and incubate the sample at RT for 5 min. Transfer the tube to the magnetic rack, then carefully transfer the supernatant to a fresh 0.2-ml PCR tube.
Synthesis of double-stranded DNA
7.After cDNA purification, convert single-stranded cDNAs to double-stranded DNAs (dsDNA) by adding 25 μl of NEBNext high-fidelity 2× PCR master mix and 0.8 μl of reverse primer i7.Mix gently by pipetting and perform a pre-PCR run with the following conditions on a thermocycler: 98°C for 10 s, 63°C for 30 s, 72°C for 1 min, and hold at 10°C.
8.Purify the dsDNA products with Qiagen MinElute PCR Purification kit following the manufacturer's instructions, and elute the sample in 24.5 μl of elution buffer.
Tn5 transposase tagmentation
9.Perform Tn5 transposase tagmentation of the dsDNA by adding 25 μl of 2× TD buffer and 0.5 μl of 2 μM standard Tn5 transposase. Incubate at 55°C for 7 min.
10.After incubation, purify the reaction mixture by using the Qiagen MinElute PCR Purification kit following the manufacturer's instructions and elute the samples in 24.2 μl of elution buffer.
PCR amplification
11.After purification, add 25 μl of NEBNext high-fidelity 2× PCR master mix, 0.4 μl forward (i5) primer, and 0.4 μl (i7) reverse primer (Buenrostro et al., 2015).
12.Run PCR amplification with the following cycling program: 72°C for 5 min, 20 cycles of 98°C for 10 s, 63°C for 30 s, 72°C for 1 min, and hold at 10°C.
13.After the PCR run, purify samples with the Qiagen MinElute PCR Purification kit per the manufacturer's instructions and elute in 20 μl of elution buffer.
14.Perform size selection with gel purification. To do this:
-
Mix all components necessary to prepare an 8% acrylamide gel (seerecipe) in a clean 15-ml Falcon tube.
-
Transfer the mixture to the corresponding polyacrylamide gel tray and let it solidify at room temperature for 30 min.
-
During the waiting time, prepare the DNA ladder and mix all the samples with 6× DNA loading dye.
-
Load all the samples and a 50-bp DNA ladder on the gel and run at 180 V for 15–20 min.
-
After running, transfer the gel to 1× TBE buffer containing SYBR gold (1:10,000) and stain the gel in the dark for 20 min.
-
After staining, check the gel under a gel documentation system and cut each gel lane from 220–1000 bp, using the 50-bp DNA ladder as reference.
-
Make a hole in the center of a 0.5-ml tube with a 21G needle and transfer each cut gel slice into a 0.5-ml punched tube. Place the 0.5-ml punched tube (containing the cut gel slice) inside a 2-ml Eppendorf tube. Then, centrifuge at 16,000 ×gfor 5 min and discard the 0.5-ml punched tube, leaving the small gel pieces collected in the 2-ml Eppendorf tube.
The aim of this step is to reduce the entire gel slice into small pieces.
- Into each 2-ml tube containing the gel pieces, add 300 μl of crush soak buffer and incubate the mixture at 55°C for 8 hr with 1400 rpm shaking, to dissolve DNA fragments in the buffer.
The aim of this step is to dissolve DNA fragments in the buffer.
-
After incubation, transfer the gel mixture to Costar spin-X centrifuge tubes and centrifuge for 5 min at 16,000× g.
-
Collect the solution passing through the filter and use Zymo ChIP DNA Clean and concentrator kit to purify it. Then, elute in 15 μl of elution buffer.
We follow the manufacturer's standard protocol to perform DNA purification. Please refer to the standard protocol from this kit for a detailed procedure.
Quantification of the DNA library
15.Quantify the DNA concentration and check the DNA size distribution of the FFPE-ATAC library with a high-sensitivity DNA Bioanalyzer kit, following the manufacturer's instructions.
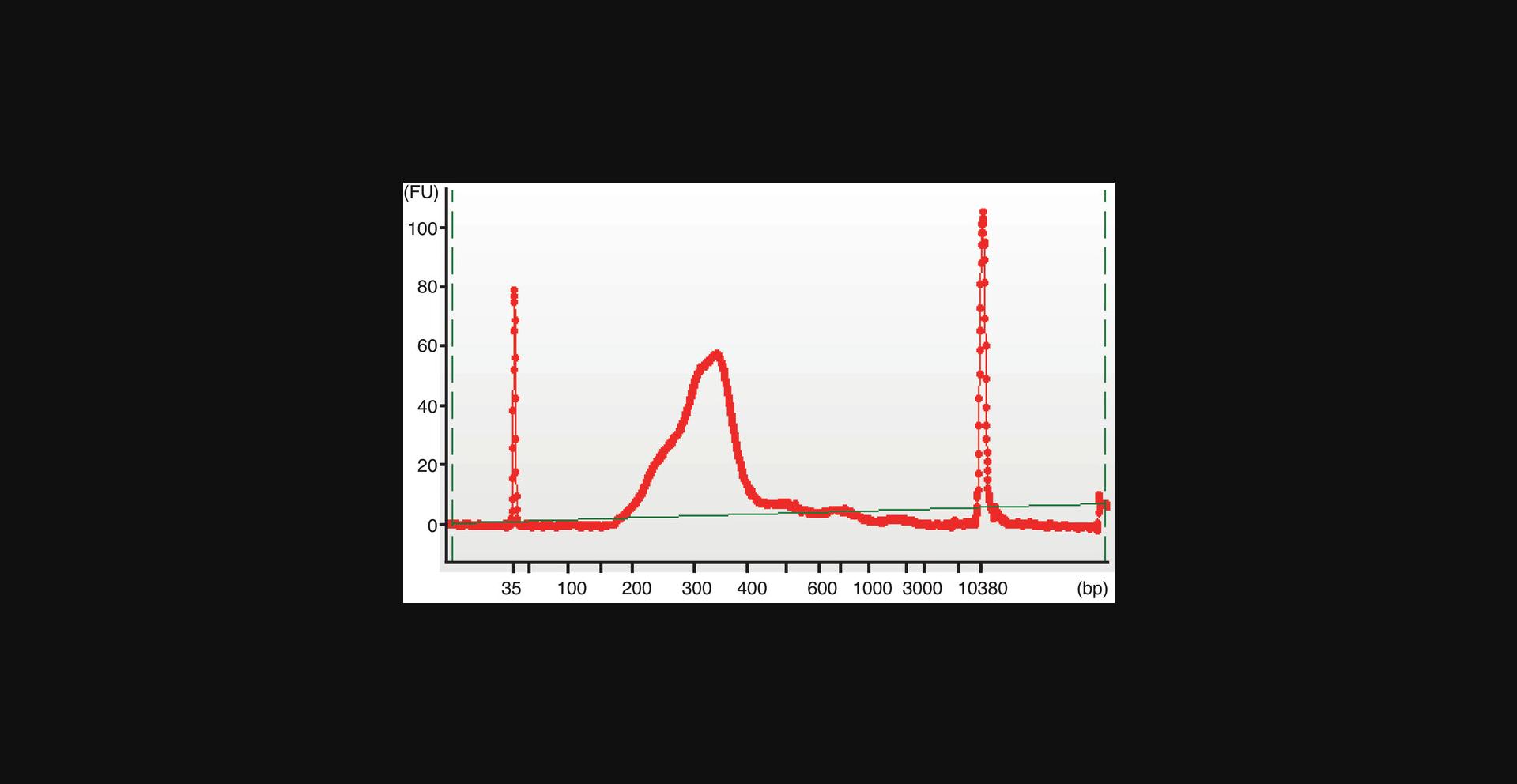
Sequencing and data analysis
16.Sequence FFPE-ATAC libraries on an Illumina sequencer to obtain at least 40 million paired-end sequencing reads (150 bp) per sequencing library.
17.Analyze FFPE-ATAC sequencing libraries following the workflow as illustrated in Figure 5.Briefly, remove adaptor sequences, align sequences to reference genome, remove sequence duplicates, and perform peak calling. All scripts are available on GitHub (https://github.com/pengweixing/FFPE-ATAC).
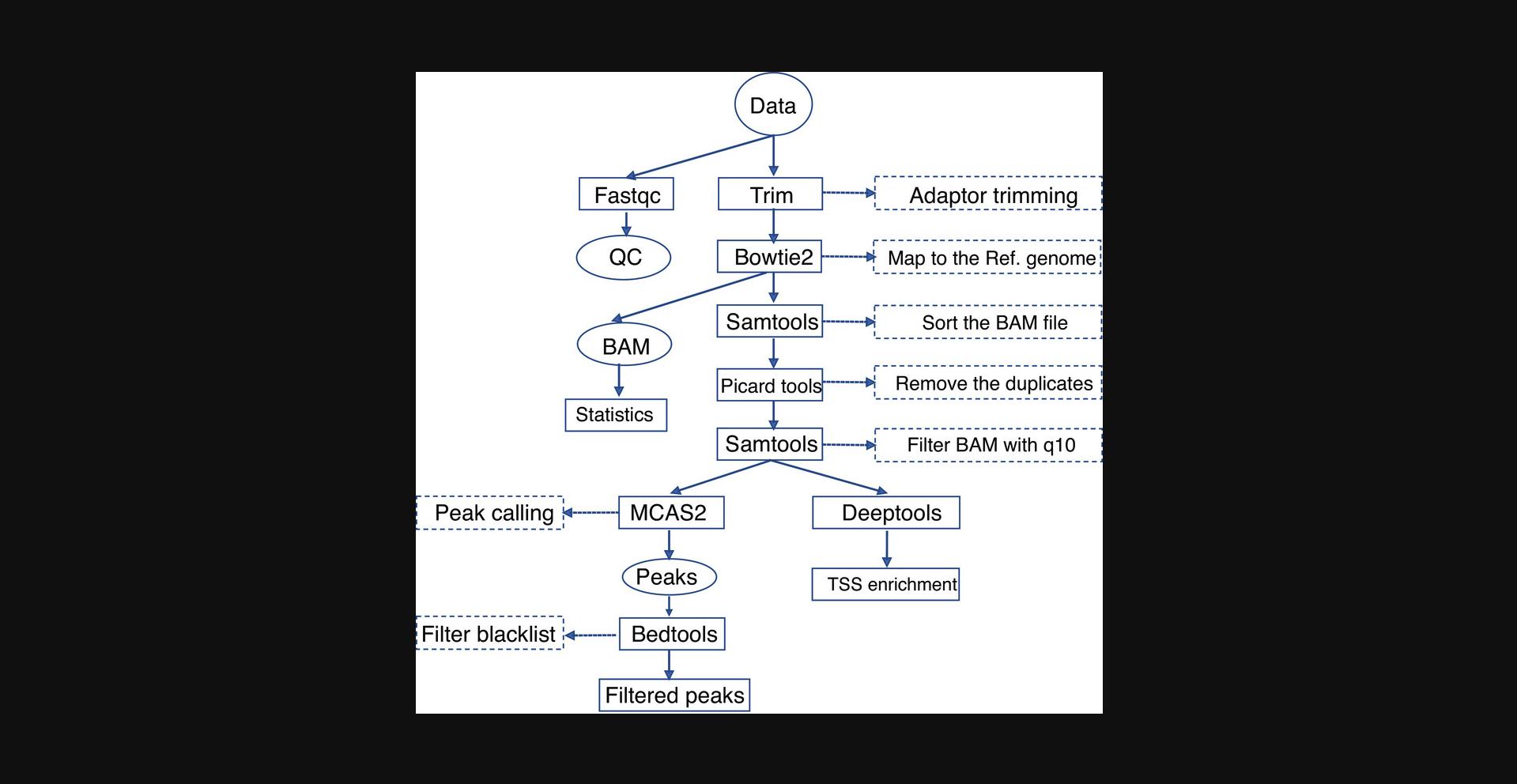
18.Visualize genome browser tracks from each individual gene locus. An example is provided in Figure 6.
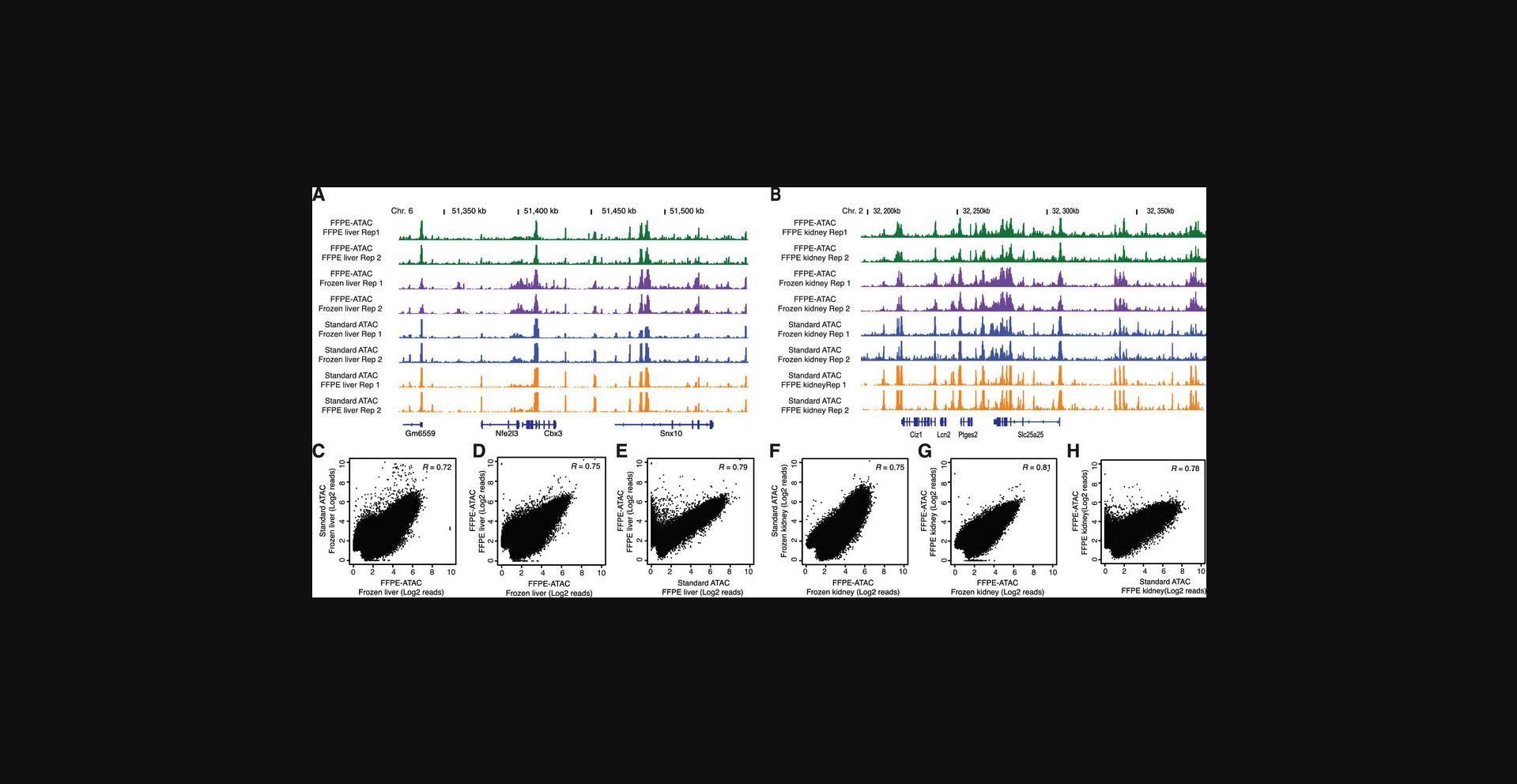
REAGENTS AND SOLUTIONS
Use sterilized double-distilled water for all recipes. Unless otherwise indicated, all preparations should be made and used fresh.
2× reverse cross-linking buffer
- 1 ml of 1 M Tris·Cl, pH 8.0
- 0.04 ml of 0.5 M EDTA
- 2 ml of 10% SDS
- 0.8 ml of 5 M NaCl
- 6.16 ml of sterilized water
2× TD buffer
- 200 μl of 1M Tris·HCl, pH 7.5
- 100 μl of 1 M MgCl2
- 2 ml Dimethyl formamide (100%)
- 7.7 ml sterile water
8% acrylamide gel
- 2 ml of 40% Acrylamide:bis-acrylamide
- 1 ml of 10× TBE buffer
- 50 μl of 10% Ammonium persulfate
- 10 μl TEMED
- 6.94 ml sterile water.
Collagenase and hyaluronidase cocktail
- 500 μl collagenase (6 mg/ml)
- 500 μl hyaluronidase (600 units/ml)
- 100 μg ampicillin
- 50 μg sodium azide
Crush soak buffer
- 2 ml of 5 M NaCl
- 0.04 ml of 0.5 M EDTA
- 1 ml of 10% SDS
- 16.96 ml sterile water
Lysis buffer
- 10 mM Tris·HCl at pH 7.4
- 10 mM NaCl
- 3 mM MgCl2
- 0.1% IGEPAL CA-360
NST Buffer
- 292 μl of 5 M NaCl
- 100 μl of 1 M Tris·HCl, pH 7.8
- 10 μl of 1 M CaCl2
- 210 μl of 1 M MgCl2
- 50 μl of 10% BSA
- 200 μl of 10% IGEPAL CA-630
- 9.138 ml sterile water
2× Dialysis Buffer
- 2 ml of 0.5 M HEPES(K+), pH 7.2
- 400 μl of 5 M NaCl
- 4 μl of 0.5 M EDTA
- 40 μl of 0.5 M DTT
- 200 μl of 10% Triton X-100
- 2 ml of 100% Glycerol
- 5.356 ml sterile water
Standard Tn5 transposase
- 1.Prepare stock solutions of the following oligos at 100 μM:
Name | Oligonucleotides sequence |
Tn5MErev | 5′-[phos]CTGTCTCTTATACACATCT-3′ |
Tn5ME-A | 5′ TCGTCGGCAGCGTCAGATGTGTATAAGAGACAG-3′ |
Tn5ME-B | 5′ -GTCTCGTGGGCTCGGAGATGTGTATAAGAGACAG-3′ |
- 2.Mix equimolar amounts of Tn5ME-A/Tn5MErev and Tn5ME-B/Tn5MErev in PCR tubes and perform adapter annealing by subjecting the oligos to 95°C for 5 min, followed by slow ramping to 25°C with −0.1°C/s ramping rate.
- 3.Mix the following reagents and incubate at 25°C for 1 hr:
- 2 μl Tn5MErev/Tn5ME-A
- 2 μl Tn5MErev/Tn5ME-B
- 20 μl of 100% glycerol,
- 15.24 μl of 2× dialysis buffer,
- 2.15 μl of Tn5 transposase (46.55 μM)
- 8.61 μl sterile water
- 4.After incubation, store T7-Tn5 transposase at −20°C for long-term use.
T7-Tn5 transposase
- 1.Prepare stock solutions of the following oligos at 100 μM.
Name | Oligonucleotides sequence |
Tn5MErev | 5′-[phos]CTGTCTCTTATACACATCT-3′ |
T7-Tn5ME | 5′-CATGAGATTAATACGACTCACTATAGGGAGAAGATGTGTATAAGAGACAG-3′ |
- 2.Mix equimolar amounts of T7-Tn5ME/Tn5Merev and perform adapter annealing by subjecting the oligos to 95°C for 5 min followed by slow ramping to 25°C with a −0.1°C/s ramping rate.
- 3.Mix the following reagents and incubate at 25°C for 1 hr:
- 4 μl T7-Tn5ME/Tn5MErev
- 20 μl of 100% glycerol,
- 15.24 μl of 2× dialysis buffer,
- 2.15 μl Tn5 transposase (46.55 μM)
- 8.61 μl sterile water
- 4.After incubation, store T7-Tn5 transposase at −20°C for long-term use.
COMMENTARY
Background Information
In basic and translational cancer research, the majority of biopsies are archived in the form of FFPE tissues. Importantly, profiling chromatin accessibility in archived FFPE tissue could be extremely useful for translational cancer research. Chromatin accessibility reflects the degree to which nuclear macromolecules can contact chromatinized DNA, and plays a key role in gene regulation in different physiological conditions (Klemm et al., 2019). As such, profiling of chromatin accessibility in archived FFPE tissue can be critical to understanding gene regulation in health and disease.
During FFPE sample preparation and long-term storage, chromatin structure is preserved (Cejas et al., 2016; Fanelli et al., 2010; Jin et al., 2015). However, because of the high degree of DNA damage in FFPE tissue samples, it is almost impossible to apply currently available highly sensitive chromatin accessibility technologies to decode the chromatin accessibility of these samples (Chin et al., 2020). To overcome these issues in FFPE tissues, we recently established FFPE-ATAC (Zhang et al., 2022), a highly sensitive method based on T7-Tn5-mediated transposition followed by in vitro transcription (IVT), to generate high-quality chromatin accessibility profiles with 500–50,000 nuclei from a single FFPE tissue section. FPE-ATAC is a simple method that requires low sample input to generate a high-quality sequencing library. Profiling chromatin accessibility in FFPE tissues from pathological collections allows researchers better insight into human disease by integrating epigenetic regulation and gene expression with clinicopathological records and discovering new potential clinical markers. This protocol will enable researchers to perform chromatin and epigenetic studies in clinically derived FFPE samples.
Critical Parameters
Tissue sectioning and tissue microdissection
With this protocol, one 10–20 µm-thick section provides a good number of nuclei. Indeed, 500,000 – 1,000,000 nuclei can be obtained from one mouse liver or kidney FFPE tissue section (20 μm thick, with an area of 1 cm × 1 cm). To err on the side of caution, we always recommend using 20 µm-thick sections for nuclei isolation. On the other hand, we have also observed that very fine microdissection of tissue under a stereomicroscope yields approximately 3 to 4 times more nuclei than random tissue microdissection, so users should empirically determine what works best for them in their laboratory.
Quality of nuclei
Generally, isolated nuclei form clumps during storage at 4°C. To obtain a single nuclei suspension, it is critical to remove the clumps via filtration (30 µm) and count again, to know exactly the number of nuclei present in the suspension before the T7-Tn5 transposase tagmentation.
Enzymatic activity
Due to repetitive use and freeze/thaw cycles, the enzymatic activity of the Tn5/T7-Tn5 transposase assembly decreases over time, so it is critical to check its enzymatic activity for proper tagmentation.
Bead purification
It is critical to not over-dry the SPRI beads or Agent Coulter RNA XP beads after ethanol washes in bead-based purification; otherwise, the DNA/cDNA will not be eluted properly.
Troubleshooting
Assessing nuclei quality
Nuclei quality is of critical importance for FFPE-ATAC. Insufficient enzyme digestion could introduce debris to the isolated nuclei prep but can be alleviated by increasing the concentration of collagenase and hyaluronidase, or by increasing digestion time. Due to the different composition of tissues derived from different organs, this process needs to be optimized separately, either by adjusting enzyme concentration or digestion time, to get an optimum number of nuclei (10,000–1,000,000).
The size of the nucleus in mammalian cells ranges from 6 to 10 µm. If the thickness of the tissue sections is thinner than the diameter of the nuclei, it is possible to obtain nuclei that are not full-size in those sections. Thus, we recommend cutting thicker sections (>20 µm) to avoid non-intact nuclei and obtain as many full-size nuclei as possible.
RNA yield from in vitro transcription (IVT)
If too few RNA molecules are generated after the IVT reaction, this can be resolved by increasing IVT time (16–20 hr).
Contamination of sequencing reads from bacterial sequences
We have observed that a proportion of sequencing reads could not be mapped to the mammalian genome but could, instead, be mapped to bacterial genomes. One possible explanation is the bacteria in the FFPE block begin to grow during digestion with the collagenase and hyaluronidase cocktail. Such bacterial growth can be inhibited by adding sodium azide and ampicillin to the enzyme cocktail.
Understanding Results
A typical sequencing result on FFPE-ATAC libraries produced following the protocols described here will have over 85% of reads mapped to a reference genome (mouse or human genome). To get sufficient sequencing coverage of accessible chromatin sites, 40 million paired-end Illumina sequencing reads are needed for each sequencing library. Since the starting materials for T7-Tn5 tagmentation is isolated nuclei, the percentage of sequencing reads from mitochondria is limited to <5%. PCR duplicates are often observed in the sequencing libraries, and the duplication rate in sequencing libraries is ∼15% to 20%. In this protocol, 20 PCR cycles are used to amplify accessible chromatin sites; however, we have used 15 PCR cycles and still obtained sufficient PCR product and a lower PCR duplication rate in the sequencing libraries.
We found that FFPE-ATAC results in accessible chromatin profiles that are very similar to those derived from standard ATAC-seq done on frozen samples (Fig. 6), revealing the versatility of FFPE-ATAC-seq in capturing all the accessible regions. Comparing with standard ATAC-seq, however, FFPE-ATAC is more labor-intensive and 4 working days are needed.
Time Considerations
FFPE-ATAC-seq was designed to take place over 4 consecutive days, regardless of the number of samples being generated. The workflow is organized as follows:
- Day 1
Tissue processing (3 hr) plus overnight enzymatic digestion (16 hr)
- Day 2
Single nuclei suspension preparation and tagmentation (3–4 hr), plus overnight reverse crosslinking (12 hr)
- Day 3
DNA purification and gap filing (2–3 hr), plus overnight in vitro transcription (16 hr)
- Day 4
DNA library preparation and bioanalyzer (6–8 hr), plus mini seq run (overnight)
Breaks can be made (1) after the preparation of the single nuclei suspension, (2) after gap filling and DNA purification, (3) after purification of in vitro -transcribed RNA, or (4) after purification of the DNA library PCR reaction mixture. After each overnight incubation, samples can always be stored long term at −20°C or short term at 4°C. With the materials listed in this article, experienced users should be able to comfortably process 8 samples simultaneously.
Acknowledgments
This protocol is derived from our previous publication (Zhang et al., 2022). We want to thank the contributions of all Chen Lab members who were involved in this work. We want to thank the Protein Science Facility at Karolinska Institute for providing purified Tn5. Our funding sources include the Swedish Research Council (VR-2016-06794, VR-201702074 to X.C.); Åke Wibergs stiftelse (M20-0007 to X.C.); Beijer Foundation (to X.C.); Jeassons Foundation (to X.C.); Petrus och Augusta Hedlunds Stiftelse (to X.C.); Göran Gustafsson's prize for younger researchers (to X.C.); Vleugel Foundation (to X.C.); Linnéstiftelsen for medicinsk forskning (to X.C.); and the Swedish Cancer Society (CAN 2021-1449, 22 0491 JIA to X.C.).
Author Contributions
Ram Yadav : Methodology and draft writing; Vamsikrishna Polavarapu : Methodology; Pengwei Xing : Software, Visualization; Xingqi Chen : Conceptualization, Funding acquisition, Methodology, Draft writing.
Conflict of Interest
Authors (X.C., V.K.P) have filed patent applications related to FFPE-ATAC. A Swedish Provisional Application was filed on 28 June 2021.
Open Research
Data Availability Statement
Data sharing is not applicable to this article as no new data were created or analyzed in this study.
Supporting Information
Filename | Description |
---|---|
cpz1535-sup-0001-TableS1.docx20.3 KB | TableS1 |
Please note: The publisher is not responsible for the content or functionality of any supporting information supplied by the authors. Any queries (other than missing content) should be directed to the corresponding author for the article.
Literature Cited
- Bartlett, D. A., Dileep, V., Henikoff, S., & Gilbert, D. M. (2021). High throughput genome-wide single cell protein: DNA binding site mapping by targeted insertion of promoters (TIP-seq). bioRxiv , 2021.03.17.435909. doi: 10.1101/2021.03.17.435909
- Buenrostro, J. D., Giresi, P. G., Zaba, L. C., Chang, H. Y., & Greenleaf, W. J. (2013). Transposition of native chromatin for fast and sensitive epigenomic profiling of open chromatin, DNA-binding proteins and nucleosome position. Nature Methods , 10, 1213–1218. doi: 10.1038/nmeth.2688
- Buenrostro, J. D., Wu, B., Litzenburger, U. M., Ruff, D., Gonzales, M. L., Snyder, M. P., … Greenleaf, W. J. (2015). Single-cell chromatin accessibility reveals principles of regulatory variation. Nature , 523, 486–490. doi: 10.1038/nature14590
- Carter, B., Ku, W. L., Kang, J. Y., Hu, G. Q., Perrie, J., Tang, Q. S., & Zhao, K. J. (2019). Mapping histone modifications in low cell number and single cells using antibody-guided chromatin tagmentation (ACT-seq). Nature Communications , 10, 3747. doi: 10.1038/s41467-019-11559-1
- Cejas, P., Li, L., O'Neill, N. K., Duarte, M., Rao, P., Bowden, M., … Long, H. W. (2016). Chromatin immunoprecipitation from fixed clinical tissues reveals tumor-specific enhancer profiles. Nature Medicine , 22, 685–691. doi: 10.1038/nm.4085
- Chin, H. G., Sun, Z., Vishnu, U. S., Hao, P., Cejas, P., Spracklin, G., … Pradhan, S. (2020). Universal NicE-seq for high-resolution accessible chromatin profiling for formaldehyde-fixed and FFPE tissues. Clinical Epigenetics , 12, 143. doi: 10.1186/s13148-020-00921-6
- Corces, M. R., Granja, J. M., Shams, S., Louie, B. H., Seoane, J. A., Zhou, W., … Chang, H. Y. (2018). The chromatin accessibility landscape of primary human cancers. Science , 362, eaav1898. doi: 10.1126/science.aav1898
- Corces, M. R., Trevino, A. E., Hamilton, E. G., Greenside, P. G., Sinnott-Armstrong, N. A., Vesuna, S., … Chang, H. Y. (2017). An improved ATAC-seq protocol reduces background and enables interrogation of frozen tissues. Nature Methods , 14, 959–962. doi: 10.1038/nmeth.4396
- Fanelli, M., Amatori, S., Barozzi, I., Soncini, M., Dal Zuffo, R., Bucci, G., … Minucci, S. (2010). Pathology tissue-chromatin immunoprecipitation, coupled with high-throughput sequencing, allows the epigenetic profiling of patient samples. Proceedings of the National Academy of Sciences USA , 107, 21535–21540. doi: 10.1073/pnas.1007647107
- Gaffney, E. F., Riegman, P. H., Grizzle, W. E., & Watson, P. H. (2018). Factors that drive the increasing use of FFPE tissue in basic and translational cancer research. Biotechnic & Histochemistry, 93, 373–386. doi: 10.1080/10520295.2018.1446101
- Giresi, P. G., Kim, J., McDaniell, R. M., Iyer, V. R., & Lieb, J. D. (2007). FAIRE (Formaldehyde-Assisted Isolation of Regulatory Elements) isolates active regulatory elements from human chromatin. Genome Research , 17, 877–885. doi: 10.1101/gr.5533506
- Handa, T., Harada, A., Maehara, K., Sato, S., Nakao, M., Goto, N., … Kimura, H. (2020). Chromatin integration labeling for mapping DNA-binding proteins and modifications with low input. Nature Protocol , 15, 3334–3360. doi: 10.1038/s41596-020-0375-8
- Harada, A., Maehara, K., Handa, T., Arimura, Y., Nogami, J., Hayashi-Takanaka, Y., … Ohkawa, Y. (2019). A chromatin integration labelling method enables epigenomic profiling with lower input. Nature Cell Biology , 21, 287–296. doi: 10.1038/s41556-018-0248-3
- Jin, W., Tang, Q., Wan, M., Cui, K., Zhang, Y., Ren, G., … Zhao, K. (2015). Genome-wide detection of DNase I hypersensitive sites in single cells and FFPE tissue samples. Nature , 528, 142–146. doi: 10.1038/nature15740
- Johnson, D. S., Mortazavi, A., Myers, R. M., & Wold, B. (2007). Genome-wide mapping of in vivo protein-DNA interactions. Science , 316, 1497–1502. doi: 10.1126/science.1141319
- Kaya-Okur, H. S., Wu, S. J., Codomo, C. A., Pledger, E. S., Bryson, T. D., Henikoff, J. G., … Henikoff, S. (2019). CUT&Tag for efficient epigenomic profiling of small samples and single cells. Nature Communication , 10, 1930. doi: 10.1038/s41467-019-09982-5
- Kennedy-Darling, J., & Smith, L. M. (2014). Measuring the formaldehyde Protein-DNA cross-link reversal rate. Analytical Chemistry , 86, 5678–5681. doi: 10.1021/ac501354y
- Klemm, S. L., Shipony, Z., & Greenleaf, W. J. (2019). Chromatin accessibility and the regulatory epigenome. Nature Reviews Genetics , 20, 207–220. doi: 10.1038/s41576-018-0089-8
- Landt, S. G., Marinov, G. K., Kundaje, A., Kheradpour, P., Pauli, F., Batzoglou, S., … Snyder, M. (2012). ChIP-seq guidelines and practices of the ENCODE and modENCODE consortia. Genome Research , 22, 1813–1831. doi: 10.1101/gr.136184.111
- Lu, X., Maturi, N. P., Jarvius, M., Yildirim, I., Dang, Y., Zhao, L., … Chen, X. (2022). Cell-lineage controlled epigenetic regulation in glioblastoma stem cells determines functionally distinct subgroups and predicts patient survival. Nature Communication , 13, 2236. doi: 10.1038/s41467-022-29912-2
- Qu, K., Zaba, L. C., Satpathy, A. T., Giresi, P. G., Li, R., Jin, Y., … Chang, H. Y. (2017). Chromatin accessibility landscape of cutaneous T cell lymphoma and dynamic response to HDAC inhibitors. Cancer Cell , 32, 27–41.e24. doi: 10.1016/j.ccell.2017.05.008
- Skene, P. J., Henikoff, J. G., & Henikoff, S. (2018). Targeted in situ genome-wide profiling with high efficiency for low cell numbers. Nature Protocol , 13, 1006–1019. doi: 10.1038/nprot.2018.015
- Skene, P. J., & Henikoff, S. (2017). An efficient targeted nuclease strategy for high-resolution mapping of DNA binding sites. Elife , 6, e21856. doi: 10.7554/eLife.21856
- Wang, Q. H., Xiong, H. Q., Ai, S. S., Yu, X. H., Liu, Y. X., Zhang, J. J., & He, A. B. (2019). CoBATCH for high-throughput single-cell epigenomic profiling. Molecular Cell , 76, 206–+. doi: 10.1016/j.molcel.2019.07.015
- Zaret, K. (2005). Micrococcal nuclease analysis of chromatin structure. Current Protocols in Molecular Biology , 69, 21.1.1–21.1.17. doi: 10.1002/0471142727.mb2101s69
- Zhang, H., Polavarapu, V. K., Xing, P., Zhao, M., Mathot, L., Zhao, L., … Chen, X. (2022). Profiling chromatin accessibility in formalin-fixed paraffin-embedded samples. Genome Research , 32, 150–161. doi: 10.1101/gr.275269.121