Characterization of Klebsiella pneumoniae Extracellular Polysaccharides
Drew A. Pariseau, Drew A. Pariseau, Brooke E. Ring, Brooke E. Ring, Saroj Khadka, Saroj Khadka, Laura A. Mike, Laura A. Mike
Abstract
Klebsiella pneumoniae is a clinically significant, Gram-negative pathogen in which the production of extracellular polysaccharides is a key virulence factor. Extracellular polysaccharides such as the capsule and its mucoviscosity play a significant role in K. pneumoniae infection. In this article, we explain several standard protocols used to characterize the extracellular polysaccharides of K. pneumoniae. Several of these protocols are adapted specifically for K. pneumoniae and describe methods to purify and quantify the extracellular polysaccharide of K. pneumoniae. We also present a standardized protocol to quantify K. pneumoniae mucoviscosity, a unique feature of K. pneumoniae extracellular polysaccharide. These protocols will help create uniformity in standard protocols used in K. pneumoniae extracellular polysaccharide studies. © 2023 The Authors. Current Protocols published by Wiley Periodicals LLC.
Basic Protocol 1 : Extracellular polysaccharide isolation and purification
Basic Protocol 2 : Large-scale isolation and purification of extracellular polysaccharide
Basic Protocol 3 : Uronic acid quantification of extracellular polysaccharide
Basic Protocol 4 : Extracellular polysaccharide visualization by SDS-PAGE
Basic Protocol 5 : Klebsiella pneumoniae mucoviscosity measurement by sedimentation resistance assay
Alternate Protocol 5 : 96-well plate-based Klebsiella pneumoniae sedimentation resistance assay
Support Protocol 5 : Determination of plate to cuvette conversion factor
INTRODUCTION
Klebsiella pneumoniae , a clinically important human pathogen, is globally responsible for a considerable amount of mortality and morbidity (Ikuta et al., 2022). The acquisition of virulence-associated genes and antimicrobial resistance from closely related species has complicated the clinical management of K. pneumoniae infections. K. pneumoniae virulence depends on various factors such as capsular polysaccharide (K-antigen), mucoviscosity, lipopolysaccharide (O-antigen), siderophores, and fimbriae. Capsular polysaccharide (CPS) is a prominent extracellular polysaccharide (EPS) synthesized by K. pneumoniae. So far, more than 80 capsular types have been identified in the K. pneumoniae complex (Pan et al., 2015). Additionally, hypermucoviscosity is a unique virulence feature of K. pneumoniae , characterized by sticky bacterial colonies and associated with changes in capsule polymer chain length (Khadka et al., 2023; Ovchinnikova et al., 2023). The capsule protects K. pneumoniae from opsonophagocytosis and complement-mediated killing, while hypermucoviscosity is often associated with increased dissemination in the host (Domenico et al., 1994; Mike et al., 2021). Given the pivotal role of EPS and related features in K. pneumoniae virulence and infection, there is a critical need to establish tools and techniques for studying K. pneumoniae EPS. Although some tools and techniques are available, they either lack standardization or are primarily described in the context of other bacterial species, requiring adaptation in K. pneumoniae.
This article presents an extensive list of protocols that facilitate the characterization of extracellular polysaccharides in K. pneumoniae. These protocols employ routinely available reagents and instruments and have been consistently followed by our research group with excellent reproducibility. We believe the standardized assays explained here will help form a uniform approach to studying K. pneumoniae EPS.
Basic Protocol 1 describes K. pneumoniae extracellular polysaccharide extraction and purification. Basic Protocol 2 details the large-scale purification of extracellular polysaccharides. Basic Protocol 3 explains the use of uronic acid measurement to quantify K. pneumoniae extracellular polysaccharide abundance. Further, Basic Protocol 4 describes a combined Alcian blue-silver stain technique to visualize purified extracellular polysaccharide. Finally, Basic Protocol 5 and Alternate Protocol 5 describe a sedimentation resistance assay to quantify K. pneumoniae mucoviscosity. Support Protocol 5 facilitates adaptation of the sedimentation assay between 96-well plate and cuvette-based platforms.
CAUTION: K. pneumoniae is a Biosafety Level 2 pathogen. Appropriate biosafety guidelines and regulations for handling pathogenic microorganisms must be followed.
CAUTION : Some of the protocols in this article require handling corrosive and flammable chemical reagents. Appropriate guidelines and regulations for handling hazardous chemicals should be followed. Wear protective personal equipment and handle such reagents in a fume hood.
Basic Protocol 1: EXTRACELLULAR POLYSACCHARIDE ISOLATION AND PURIFICATION
This protocol describes a method to isolate and purify surface-associated and cell-free (non-attached) extracellular polysaccharides of K. pneumoniae. The protocol is based on a previously reported method and has been well-established for K. pneumoniae (Anderson et al., 2017; Favre-Bonte et al., 1999; Mike et al., 2021). A K. pneumoniae culture is subjected to detergent treatment to release capsular polysaccharides from the bacterial cell surface into the supernatant. Cold ethanol is used to precipitate the extracellular polysaccharides, which are rehydrated to dissolve. This protocol provides a high-throughput method to obtain purified extracellular polysaccharides from a large number of samples at once for quick quantification and/or staining.
Materials
-
Isolated colonies of any K. pneumoniae strain on a nutrient agar plate [e.g., K. pneumoniae ATCC 43816 (KPPR1) on low-salt Luria-Bertani]
-
Low-salt Luria Bertani (LB) broth (see recipe)
-
1× Phosphate-buffered saline (PBS; sterile or non-sterile)
-
1% Zwittergent 3-14 in 100 mM citric acid (see recipe)
-
Anhydrous ethanol (Fisher Scientific, cat. no. A405P-4)
-
Ultrapure water
-
Pipettor and sterile pipette tips (200 µl and 1000 µl)
-
Pipette controller (e.g., MidSci, cat. no. ASMPIP1)
-
5- or 10-ml sterile serological pipettes (e.g., Genesee Scientific, cat. no. 12-104)
-
Sterile applicator sticks (e.g., Fisher Scientific, cat. no. 22-029-491)
-
17 × 100-mm sterile aeration culture tubes (or other pertinent culture tube) (e.g., Genesee Scientific, cat. no. 21-131)
-
96-well flat-bottom plate (sterile or non-sterile) (e.g., Genesee Scientific, cat. no. 25-104)
-
37°C Shaking incubator (e.g., New Brunswick INNOVA 44/44R)
-
Vortex mixer (e.g., Benchmark Scientific, cat. no. BV1003)
-
Centrifuge 24 × 1.5/2 ml, ≥17,000 × g (e.g., Eppendorf Centrifuge 5425)
-
Refrigerated centrifuge 24 × 1.5/2 ml, ≥17,000 × g (e.g., Eppendorf Centrifuge 5425R)
-
Heat block set at 50°C (e.g., Eppendorf ThermoMixer C)
-
1.7-ml sterile microcentrifuge tubes (e.g., Genesee Scientific, cat. no. 24-282C)
-
Visible-spectrum microplate reader (e.g., Biotek EPOCH2)
Setting up overnight cultures of KPPR1
1.Label three 17 × 100-mm culture tubes for three biological replicates. Include one additional tube for a negative growth control.
2.Add 3 ml low-salt LB broth to each tube.
3.Use a sterile applicator stick or inoculating loop to introduce one bacterial colony to each tube, except the negative growth control tube.
4.Incubate tubes at 37°C and 200 rpm for 16-18 hr in a 37°C shaking incubator.
[Optional] Measuring overnight culture density
5.Take a 96-well flat-bottom plate and transfer 270 µl of 1× PBS into three wells. Transfer 300 µl of 1× PBS to three additional wells for blank correction.
6.Briefly vortex the tubes incubated overnight and pipette 30 µl of the culture into a corresponding well containing 270 µl of 1× PBS. Mix well by pipetting up and down 5-6 times.
7.Measure the absorbance of the blank controls and bacterial suspensions at 600 nm (OD600) in a 96-well plate reader.
8.Calculate the overnight OD600 of the culture as described below.
- OD600 = (Sample A600 – Blank A600) × dilution factor × plate-to-cuvette conversion factor
- = (Sample A600 – Blank A600) × 10 × 1.181599
Sample A600: OD600 of the overnight sample at 600 nm
Blank A600: OD600 of 300 µl 1× PBS at 600 nm
Dilution factor: Ratio of total volume (culture + PBS) to culture volume used (e.g., 300 µl total sample volume/30 µl bacterial culture = 10 dilution factor)
Plate to cuvette conversion factor: Converts absorbance reading from the path length in a 96-well plate to a standard 1-cm path length in a cuvette (1.181599). This should be experimentally determined for each microplate spectrophotometer. Refer to Support Protocol 5 for conversion factor determination.
Alternatively, a serial dilution of the sample can be plated to determine the colony-forming units (CFU) per ml for normalization.
Extracellular polysaccharide purification
9.Use one clean 1.7-ml tube per sample. Transfer 50 µl of 1% Zwittergent 3-14 in 100 mM citric acid, pH 2, to each tube.
10.Add 250 µl samples of well-vortexed overnight culture to the Zwittergent-containing tubes and mix well by inverting the tubes multiple times.
11.Incubate the Zwittergent-sample mixture at 50°C for 20 min, inverting periodically.
12.Centrifuge the Zwittergent/water-sample mixture for 5 min at 17,000 × g and room temperature.
13.Carefully transfer 100 µl of the supernatant to the 1.7-ml tube containing 400 µl absolute ethanol (80% final ethanol concentration) and mix by inverting the tube.
14.Incubate the supernatant-ethanol-containing tube on ice for at least 20 min.
15.Centrifuge the tubes for 5 min at 17,000 × g and 4°C.
16.Decant and discard the supernatant carefully and let the tubes dry at room temperature for 5-10 min.
17.Add 200 µl ultrapure water to the tubes and vortex for 5-10 s.
18.Incubate the tubes at 37°C for 30 min to solubilize the polysaccharide and vortex again.
19.Store the purified, hydrated polysaccharide samples at -20°C or proceed directly to Basic Protocol 3.
Basic Protocol 2: LARGE-SCALE ISOLATION AND PURIFICATION OF EXTRACELLULAR POLYSACCHARIDE
This protocol is a modification of Basic Protocol 1 and illustrates the large-scale extraction and purification of K. pneumoniae extracellular polysaccharide. The process involves extracting polysaccharides from the bacterial cell surface using a detergent, followed by ethanol precipitation. The precipitated polysaccharides are dialyzed over several days while treating with nuclease and proteinase to remove the contaminating nucleic acids and proteins (Alvarez & Cobb, 2019). The polysaccharide sample can be used for further chemical analysis, such as composition analyses by mass spectrometry or HPLC.
Materials
-
Isolated colonies of any K. pneumoniae strain on a nutrient agar plate [e.g., K. pneumoniae ATCC 43816 (KPPR1) on low-salt LB]
-
Low-salt LB broth (see recipe)
-
1% Zwittergent 3-14 in 100 mM citric acid (see recipe)
-
Ice-cold anhydrous ethanol (Fisher Scientific, cat. no. A405P-4)
-
Ultrapure water
-
5% (w/v) sodium azide (see recipe)
-
Ribonuclease A (RNase, Worthington Biochemical Corp., cat. no. LS005650)
-
Deoxyribonuclease I (DNase, Worthington Biochemical Corp., cat. no. LS002007)
-
PRONASE (Millipore, cat. no. 537088-10KU)
-
1 M Tris, pH 7.5 (see recipe)
-
1 M CaCl2 (see recipe)
-
1 M MgCl2 (see recipe)
-
Pipettor and sterile pipette tips (200 µl and 1000 µl)
-
Pipette controller (e.g., MidSci, cat. no. ASMPIP1)
-
5- or 10-ml sterile serological pipettes (e.g., Genesee Scientific, cat. no. 12-104)
-
Sterile applicator sticks (e.g., Fisher Scientific, cat. no. 22-029-491)
-
17 × 100-mm sterile aeration culture tubes (or other pertinent culture tube) (e.g., Genesee Scientific, cat. no. 21-131)
-
37°C Shaking incubator (e.g., New Brunswick INNOVA 44/44R)
-
Plastic bucket, minimum 1 L volume (e.g., Eisco Labs 5000 ml plastic beaker)
-
Vortex mixer (e.g., Benchmark Scientific, cat. no. BV1003)
-
2-ml microcentrifuge tubes (e.g., Genesee Scientific, cat. no. 24-283)
-
15-ml sterile conical tube (e.g., Griener Bio-One CELLSTAR, cat. no. 181-271)
-
50-ml sterile conical tubes (e.g., USA Scientific, cat. no. 5622-7261)
-
Centrifuge 24 × 1.5/2 ml, minimum speed 17,000 × g (e.g., Eppendorf Centrifuge 5425)
-
Refrigerated centrifuge, fixed-angle rotors able to fit 50 ml conical tubes, minimum speed 15,000 × g (e.g., Eppendorf Centrifuge 5910Ri)
-
Heat block set at 50°C (e.g., Eppendorf Thermomixer C)
-
4°C refrigerator or 4°C cold room
-
Magnetic stirrer (e.g., Benchmark Scientific, cat. no. H4000-HS/H4000-S)
-
Dialysis membrane, ≤18 mm wide, 25 kD molecular weight cut-off (Spectra/Por 6 Regenerated Cellulose Dialysis Membrane, Repligen, cat. no. 128218)
-
Dialysis tubing closures, ≤35 mm (Spectra/Por 6 Closures, Repligen, cat. no. 132736)
-
Sterile Syringes, 10 ml (e.g., Fisher Scientific, cat. no. 14-955-459)
-
Syringe Filters, 0.2 µm pore size (Fisher Scientific, cat. no. 13-100-106)
-
Rotating shaker (e.g., Labline Instruments, cat. no. 1314)
-
Clean borosilicate glass vials with screw cap (Vial volume depends on sample volume)
-
Lyophilizer (e.g., FreeZone 1 L Benchtop Freeze Dry System, Labconco cat. no. 7740020)
Day 1: Inoculation and incubation of KPPR1
1.Inoculate the strains of interest into 3 ml LB broth in a 17 × 100-mm culture tube and incubate at 37°C and 200 rpm for 16-18 hr.
Day 2: Extraction of extracellular polysaccharide (EPS)
2.Sub-culture the overnight culture (1:100) in 3 ml LB and incubate at 37°C with shaking (200 rpm).
3.Incubate the culture until it reaches the mid-log growth phase.
4.For each sample, transfer 500 µl of 1% Zwittergent 3-14 in 100 mM citric acid to a clean 2-ml tube.
5.Add 1.5 ml mid-log phase culture to the corresponding Zwittergent tube. Mix well by inverting.
6.Incubate the tubes at 50°C for 20 min.
7.Following incubation, centrifuge the tubes for 5 min at 21,000 × g and room temperature.
8.Carefully transfer 1.5 ml supernatant to the conical tube containing ethanol. Mix by inverting and incubate on ice for 30 min.
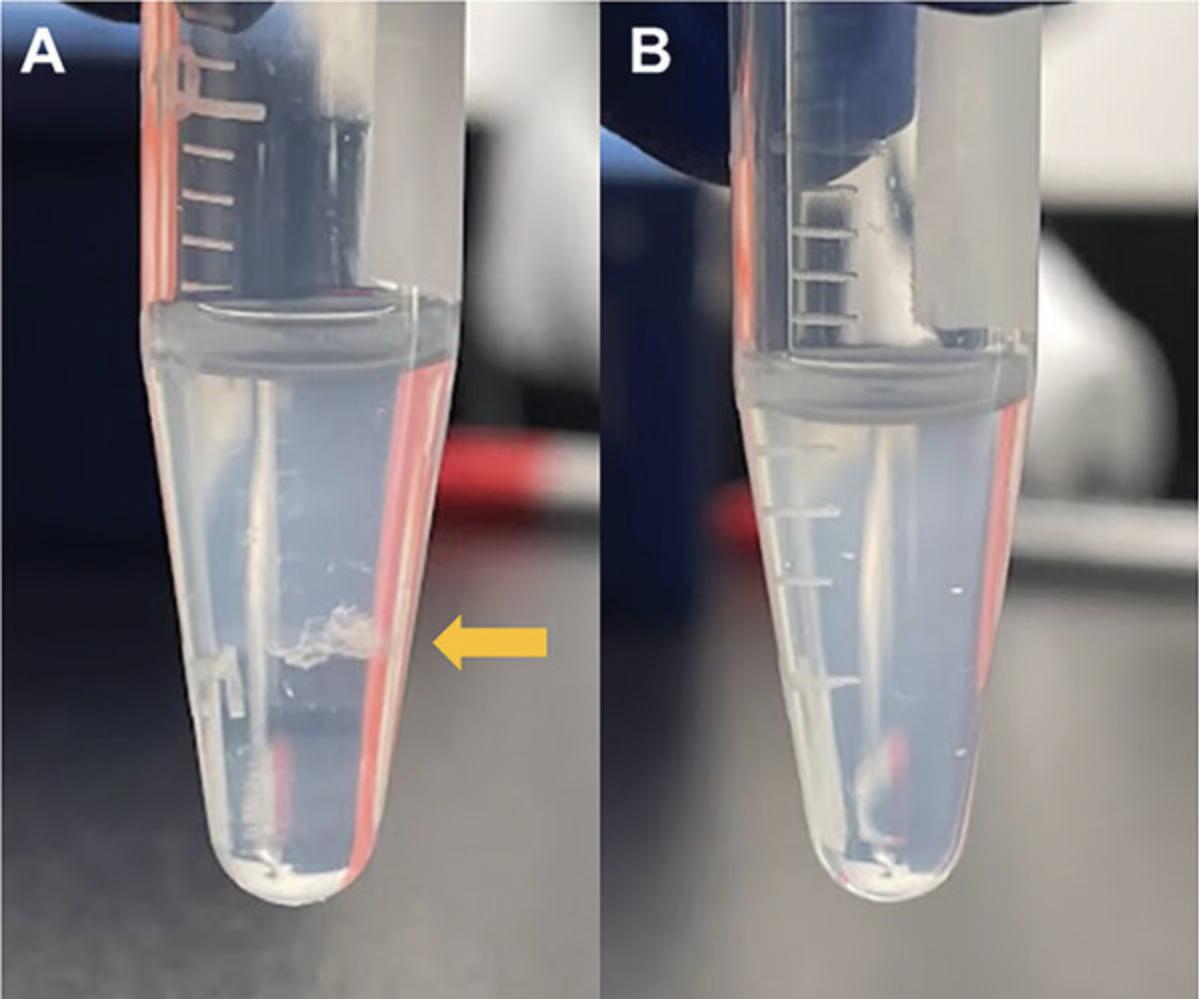
9.Centrifuge the conical tube for 5 min at 15,000 × g and 4°C.
10.Remove the supernatant carefully and discard. Add 2 ml of ultrapure water. Incubate the tube at room temperature for 30 min on a rotator to rehydrate the polysaccharide pellet.
11.Transfer the 2 ml of rehydrated sample to a 25 kD cut-off dialysis membrane and secure with tube closures. Dialyze against 1 L ultrapure water for 1 hr at room temperature with constant stirring.
12.After 1 hr, transfer the dialysis tubing to 1 L of fresh ultrapure water containing 0.05% sodium azide (add 10 ml of 5% (w/v) sodium azide per 1 L ultrapure water). Dialyze with constant stirring overnight at 4°C.
Day 3: Nuclease treatment of EPS
13.Transfer the dialysis tubing to 1 L fresh ultrapure water containing 0.05% sodium azide. Dialyze with constant stirring at room temperature for 8-10 hr.
14.Prepare 2 ml nuclease solution by adding 6 mg RNase A and 1.4 mg DNase to 1 ml of 1 M Tris, pH 7.5, 20 µl 1 M CaCl2, 20 µl MgCl2, and 1 ml ultrapure water. Sterilize by filtering through a 0.2-µm syringe filter.
15.Remove the sample from the dialysis membrane and transfer to a clean 15-ml conical tube. Add 20 µl of 5% sodium azide (final concentration 0.05%) and bring the final volume to 2 ml with sterile ultrapure water.
16.Add 220 µl of the nuclease solution to the 2 ml sample to achieve a final concentration of 0.3 mg/ml RNase and 0.07 mg/ml DNase in 50 mM Tris, 1 mM CaCl2, and 1 mM MgCl2.
17.Incubate the nuclease-added sample overnight at 37°C with shaking (200 rpm).
Day 4: Proteinase treatment of EPS
18.Test the pH of the nuclease-treated sample by placing a few drops of the solution on a pH strip. If the pH is not ∼7.5, adjust by slowly adding either weak acid or base.
19.Prepare 2 ml of proteinase solution by adding 6.6 mg PRONASE to a solution of 100 µl of 1M Tris, pH 7.5, 2 µl of 1 M CaCl2, and 1880 µl sterile ultrapure water to obtain 3.3 mg/ml proteinase in 50 mM Tris, pH 7.5 with 1 mM CaCl2. Sterilize by 0.2-µm syringe filtration and store at 4°C.
20.Add proteinase solution to the nuclease-treated sample at a 1:10 ratio (proteinase:sample). For example, add 240 µl proteinase solution to 2.2-ml nuclease-treated sample. This will achieve a final concentration of 50 mM Tris, 1 mM CaCl2, and 0.33 mg/ml proteinase.
21.Add 2.4 µl of 5% sodium azide to get a final concentration of 0.05% sodium azide.
22.Incubate the proteinase-containing sample overnight at 37°C with shaking (200 rpm).
Day 5: Ethanol precipitation of EPS
23.Add another 260 µl proteinase solution and 2.6 µl of 5% sodium azide (0.05% final concentration), then incubate at 37°C for 2 hr with shaking (200 rpm).
24.Inactivate the proteinase by adding 1 M EDTA, pH 8, to a final concentration of 50 mM EDTA. For example, add 135 µl of 1 M EDTA, pH 8 to ∼2.6 ml nuclease- and proteinase-treated sample.
25.Mix the EDTA-sample solution on a rotating shaker for 30 min at 4°C.
26.Add 2 M NaCl to a final concentration of 200 mM. For example, add 273 µl of 2 M NaCl to ∼2.73 ml sample.
27.Add pre-chilled absolute ethanol at a 1:4 ratio (sample:ethanol) to a final concentration of 80% ethanol. For example, add 12 ml ethanol to ∼3 ml sample.
28.Leave the sample to precipitate at -20°C overnight.
Day 6: Rehydrating EPS precipitate
29.Centrifuge the ethanol-precipitated sample for 1 hr at 10,000 × g and 4°C.
30.Carefully remove the supernatant and dry the sample by inverting the tube at a downward angle over clean absorbent paper in a biosafety cabinet for 2 hr.
31.Resuspend the pellet in 1.5 ml sterile ultrapure water and incubate at 37°C with shaking (200 rpm) for 1.5 hr to rehydrate the polysaccharides.
32.[Optional] Transfer the sample to a 25 kD cut-off dialysis membrane and dialyze against 1 L ultrapure water with 0.05% sodium azide for 1-2 hr at room temperature with constant stirring. Use 1 L ultrapure water per 2-3 ml sample.
33.If dialysis was done in the previous step, change the water with 0.05% sodium azide and dialyze overnight at 4°C with constant stirring. Otherwise, transfer the sample from step 31 to 25 kD cut-off dialysis membrane and dialyze against 1 L ultrapure water with 0.05% sodium azide overnight at 4°C with constant stirring. Use 1 L ultrapure water per 2-3 ml sample.
Day 7: Freezing of purified EPS
34.Dialyze the sample again against 1 L ultrapure water with 0.05% sodium azide at room temperature for 8 hr.
35.Transfer the sample to a clean glass vial and freeze at -20°C overnight.
Day 8: Lyophilization
36.Remove the tube lid and wrap the opening with parafilm. Then, poke small holes through the parafilm.
37.Freeze-dry the sample in a lyophilizer for 14-18 hr at ∼0.1 Torr pressure and collector temperature of -40°C.
Day 9: EPS storage
38.Following freeze-drying, take the dry weight of the lyophilized purified polysaccharide and store at -20°C.
Basic Protocol 3: URONIC ACID QUANTIFICATION OF EXTRACELLULAR POLYSACCHARIDE
This protocol illustrates a method to quantify extracellular polysaccharides produced by K. pneumoniae. Extracellular polysaccharide purified using Basic Protocol 1 is acid-hydrolyzed by boiling with sodium tetraborate in sulfuric acid. The quantity of uronic acid is detected by conjugating the free hexuronic acids to 3-phenylphenol chromagen. The change in absorbance before and after adding 3-phenylphenol is measured to deduce the concentration of glucuronic acid in the sample (Blumenkrantz & Asboe-Hansen, 1973). Measuring the glucuronic acid concentration is an indirect way of quantifying total K. pneumoniae extracellular polysaccharide as it does not quantify neutral sugar content (Anderson et al., 2017; Khadka & Ring et al., 2023; Mike et al., 2021). This protocol provides a quantitative method to measure the amount of extracellular polysaccharide produced by K. pneumoniae.
Materials
-
Purified extracellular polysaccharides (see Basic Protocol 1)
-
3-Phenylphenol (Sigma-Aldrich, cat. no. 262250)
-
0.125 M Sodium hydroxide (see recipe)
-
0.0125 M Sodium tetraborate in concentrated sulfuric acid (>95%) (see recipe)
-
Glucuronic acid standards: 100, 50, 25, 12.5, 6.25, 3.125 µg/ml (see recipe)
-
Ice-filled bucket
-
Vortex mixer (e.g., Benchmark Scientific, cat. no. BV1003)
-
Pipettor and sterile pipette tips (10-20 µl)
-
Multichannel pipettor and filter-tips (1000 µl)
-
Pipette controller (e.g., MIDSCI, cat. no. ASMPIP1)
-
10-ml Serological pipettes (e.g., Genesee Scientific, cat. no. 12-104)
-
Timer
-
1.7-ml microcentrifuge tubes (e.g., Genesee cat. no. 24-282C)
-
1.7-ml tube lid locks
-
Heat block set to 100°C (e.g., MIDSCI, cat. no. ASDRYBATH5)
-
1.5-ml disposable cuvettes (e.g., Fisher Scientific, cat. no. 14-955-127)
-
Visible-spectrum cuvette spectrophotometer (e.g., Thermo Scientific GENESYS 30)
Extracellular polysaccharide purification and preparation
1.Refer to Basic Protocol 1 for extracellular polysaccharide purification. Record the culture OD600 or determine the colony forming units per ml (CFU/ml) for each sample for downstream normalization. Refer to steps 5 through 8 in Basic Protocol 1 to measure culture OD600.
2.Thaw frozen glucuronic acid standards and stored experimental samples prepared using Basic Protocol 1 at room temperature. In the meantime, prepare 0.3% (3 mg/ml) w/v 3-phenylphenol in 0.125 M NaOH (e.g., 0.003 g 3-phenylphenol in 1 ml of 0.125 M NaOH).
3.Once completely thawed, transfer 200 µl of each glucuronic acid standard to a new, labeled 1.7-ml microcentrifuge tube. Include 200 µl of ultrapure water in a separate tube as a blank.
4.Vortex the extracellular polysaccharide samples briefly. Then, in a chemical hood, add 1.2 ml of 0.0125 M sodium tetraborate in concentrated sulfuric acid to the 200 µl polysaccharide sample, each glucuronic acid standard, and the blank.
5.Mix by inverting the tubes, then incubate the samples with lid locks at 100°C for 5 min in a heat block.
6.Cool the samples to room temperature by incubating the tubes in ice for 5 min.
7.Once cooled, transfer the standards and each sample to a labeled cuvette.
8.Measure the absorbance of the standards and samples at 520 nm after measuring the baseline with the ‘blank’.
9.Add 10 µl of 0.3% (3 mg/ml) w/v 3-phenylphenol in 0.5% (0.125 M) NaOH to each cuvette and mix by gently pipetting up and down.
10.Measure a second round of absorbance at 520 nm for each sample including the ‘blank’.
11.Calculate the uronic acid concentration as described below:
- a. Subtract the first A520 from the second A520 reading to get ΔA520 for every sample, including the blank and standards.
- b. Plot the ΔA520 of each standard and blank on the y-axis and respective uronic acid concentration (in µg/ml) on the x-axis. Calculate the slope and y-intercept from the linear standard curve using y = mx + b, where m is the slope and b is the y-intercept.
- c. Calculate the uronic acid concentration in each sample as:
Uronic acid concentration (µg/ml) = (ΔA520 – y-intercept)/slope
- d. Normalize the uronic acid concentration of each sample to its respective overnight OD600 or CFU/ml.
12.Calculate total cell-attached and non-attached extracellular polysaccharides (EPS) as described below.
13.Wipe all surfaces that came in contact with sodium tetraborate in sulfuric acid using a damp paper towel and dispose of the hazardous waste following institutional guidelines.
Basic Protocol 4: EXTRACELLULAR POLYSACCHARIDE VISUALIZATION BY SDS-PAGE
This protocol illustrates an optimized method to visualize purified K. pneumoniae extracellular polysaccharide by SDS-PAGE using a combination of Alcian blue and silver stain. The combination staining protocol was adapted to K. pneumoniae extracellular polysaccharide from previously described methods (Khadka et al., 2023; Ovchinnikova et al., 2023; Tipton & Rather, 2019). Extracellular polysaccharides purified from K. pneumoniae using Basic Protocol 1 are resolved using a gradient SDS-PAGE gel. Polysaccharides are stained with Alcian blue and then enhanced by silver stain. The protocol takes a minimum of 2 days to complete. This protocol is useful for examining extracellular polysaccharide chain length diversity.
Materials
-
Isolated colonies of any K. pneumoniae strain on a nutrient agar plate [e.g., K. pneumoniae ATCC 43816 (KPPR1) on low-salt LB] (see recipe)
-
Low-salt LB broth (see recipe)
-
1× phosphate-buffered saline (PBS; sterile or non-sterile)
-
1% Zwittergent 3-14 in 100 mM citric acid (see recipe)
-
Anhydrous ethanol (e.g., Fisher Scientific, cat. no. A405P-4)
-
Ultrapure water
-
4× SDS-PAGE loading buffer (see recipe)
-
4%-15% Mini-PROTEAN TGX Stain-Free Protein Gel (BioRad, cat. no. 4568083)
-
Tris-glycine-SDS gel running buffer (see recipe)
-
Precision Plus Protein All Blue Prestained Protein Standards (Biorad, cat. no. 1610373)
-
0.1% Alcian blue solution (see recipe)
-
Alcian blue stain base solution (see recipe)
-
Pierce Silver Stain Kit (ThermoFisher Scientific, cat. no. 24612)
-
10% ethanol (see recipe)
-
Silver stain gel fixing solution (see recipe)
-
Stop solution (5% Acetic acid solution; see recipe)
-
Pipettor and pipette tips (200 µl and 1000 µl)
-
17 × 100-mm sterile aeration culture tubes (or other pertinent culture tube) (e.g., Genesee Scientific, cat. no. 21-131)
-
96-well flat-bottom plate (sterile or non-sterile) (e.g., Genesee Scientific, cat. no. 25-104)
-
37°C shaking incubator (e.g., New Brunswick INNOVA 44/44R)
-
Vortex mixer (e.g., Benchmark Scientific, cat. no. BV1003)
-
Centrifuge 24 × 1.5/2 ml, ≥17,000 × g (e.g., Eppendorf Centrifuge 5425)
-
Refrigerated centrifuge 24 × 1.5/2 ml, ≥17,000 × g (e.g., Eppendorf Centrifuge 5425R)
-
Heat block set at 50°C (e.g., Eppendorf ThermoMixer C)
-
Visible-spectrum microplate reader (e.g., BioTek EPOCH2)
-
1.7- and 2-ml sterile microcentrifuge tubes (e.g., Genesee Scientific, cat. no. 24-282C and cat. no. 24-283)
-
Electrophoresis cell (e.g., Biorad Mini-PROTEAN Tetra Vertical Electrophoresis Cell; cat. no. 1658004)
-
Ice-pack able to fit in the electrophoresis cell
-
Power supply (e.g., Biorad PowerPac Basic Power Supply; cat. no. 1645050)
-
Clean staining tray able to fit 8.6 × 6.7 cm gel (glass or plastic)
-
Benchtop rotator (e.g., Lab-Line, cat. no. 21580)
-
Gel imaging system (Syngene G:box Chemi XX6/XX9)
Extracellular polysaccharide purification and preparation
1.Refer to Basic Protocol 1 , steps 1 through 8, to set up overnight cultures and measure culture density.
2.Take 1.5 OD600 equivalent of culture in a 1.7- or 2-ml tube.
3.Bring the final volume of each sample to that of the largest sample volume used.
4.Centrifuge the normalized samples for 15 min at 21,000 × g and room temperature.
5.Remove all but 50 µl of the supernatant and resuspend the pellet in 950 µl 1× PBS.
6.Centrifuge for 15 min at 21,000 × g and room temperature.
7.Remove 750 µl of the upper supernatant, leaving behind about 250 µl of the pellet and supernatant solution.
8.Add 50 µl of 1% Zwittergent 3-14 in 100 mM citric acid, pH 2, to a final ratio of 1:5 (Zwittergent:sample) and mix well by inverting the tubes.
9.Incubate the Zwittergent-sample mixture at 50°C for 20 min in a heat block.
10.Centrifuge the mixture for 5 min at 17,000 × g and room temperature.
11.Carefully transfer 100 µl of the supernatant to the 1.7-ml tube containing 400 µl absolute ethanol (80% final ethanol concentration) and mix by inverting the tube.
12.Incubate the supernatant-ethanol containing tube on ice for 20 min.
13.Centrifuge the tubes for 5 min at 17,000 × g and 4°C.
14.Decant the supernatant carefully and let the tubes dry at room temperature for 5-10 min.
15.Add 200 µl ultrapure water to the tubes and vortex for 5-10 s.
16.Incubate the tubes at 37°C for 30 min to solubilize the polysaccharides and vortex again before proceeding to the next step.
17.In a new set of tubes containing 25 µl of 4× SDS-PAGE loading buffer, add 75 µl purified polysaccharide solution and vortex briefly.
SDS-PAGE of purified extracellular polysaccharide
18.Take the tape off the bottom of a pre-cast 4%-15% SDS-PAGE gel and fit the gel in a running module. Insert the running module into the electrophoresis cell.
19.Add the ice pack to the electrophoresis cell and fill the tank to the top or ‘4 gels’ mark with Tris-Glycine-SDS gel running buffer.
20.Clean the wells by flushing them with running buffer using a P1000 pipette. Gently pipette the running buffer up and down to clean the wells.
21.Load 20 µl sample into the respective well and include one well with 12-15 µl Precision Plus Protein All Blue Prestained standards as a molecular weight marker.
22.Transfer the gel-fitted electrophoresis cell to a bucket filled with ice. Cover the four sides of the electrophoresis cell with ice to keep it cold.
23.Transfer the ice-filled bucket containing the prepared apparatus with electrophoresis cell to a 4°C refrigerator or cold room.
24.Connect the electrophoresis cell to a power supply and run the gel at 300 V for 4-5 hr.
Staining of extracellular polysaccharide with Alcian blue
25.Following SDS-PAGE, carefully remove the gel from the cassette and transfer it to a box containing 200 ml ultrapure water. Wash the gel in ultrapure water for 10 min, a total of five times.
26.Stain the gel with 25-30 ml of 0.1% Alcian Blue Stain (or volume enough to cover the gel) at room temperature for 1 hr on a shaker.
27.Quickly wash the gel with Stain Base Solution to remove excess stain.
28.Once excess stain is removed, de-stain with 100-200 ml Stain Base Solution overnight at room temperature on a shaker.
Staining with Pierce Silver Stain Kit
29.Prepare Gel Fixing Solution, 10% ethanol, and Stop Solution in advance.
30.Slowly drain the Stain Base Solution used for de-staining the gel and wash the gel twice in ultrapure water for 5 min.
31.Remove ultrapure water and add Gel Fixing Solution (30% ethanol:10% acetic acid) for 15 min. Repeat the process a total of two times.
32.Wash the gel twice in 10% ethanol.
33.Wash the gel twice in ultrapure water for 5 min.
34.Incubate the gel in Sensitizer Working Solution for 1 min, then wash twice with ultrapure water for 1 min.
35.Prepare 25 ml Stain Working Solution per gel by mixing 0.5 ml (1 part) Silver Stain Enhancer with 25 ml (50 parts) Silver Stain.
36.Incubate the gel in Stain Working Solution for 30 min.
37.Quickly wash the stained gel twice with ultrapure water for 20 s.
38.Add Developer Working Solution and incubate with gentle shaking until dark brown bands start to appear.
39.When the desired contrast between polysaccharide bands is reached, discard the Developer Working Solution and immediately wash the gel with Stop Solution.
40.Add 25 ml Stop Solution and incubate the gel for 10 min before imaging.
Gel imaging
41.Lay the gel on the white reflective plate of a Syngene G:box.
42.Select the ‘Silver Stain’ imaging program within the ‘Visible Protein Gel’ settings of the Syngene G:box.
43.Capture the image.
Basic Protocol 5: Klebsiella pneumoniae MUCOVISCOSITY QUANTIFICATION BY SEDIMENTATION RESISTANCE ASSAY
This protocol presents a robust and precise method for quantifying mucoviscosity in K. pneumoniae through a sedimentation resistance assay. Increased mucoidy is speculated to increase the drag forces on the bacteria, increasing sedimentation resistance. However, this hypothesis necessitates experimental validation. The procedure encompasses culturing the bacterium, conducting low-speed centrifugation, and quantifying absorbance at OD600. By adhering to these systematic instructions, researchers can confidently achieve consistent mucoviscosity measurements and discern hypermucoid K. pneumoniae strains. This assay serves as a valuable tool for characterizing the virulence potential and pathogenicity of different bacterial isolates, aiding in studying K. pneumoniae infections.
Materials
-
Isolated colonies of any K. pneumoniae strain on a nutrient agar plate [e.g., K. pneumoniae ATCC 43816 (KPPR1) on low-salt Luria-Bertani] (see recipe)
-
Low-salt LB broth (see recipe)
-
1× phosphate-buffered saline (PBS; sterile or nonsterile)
-
Pipettor and sterile pipette tips (200 µl and 1000 µl)
-
17 × 100-mm sterile aeration culture tubes (or other pertinent culture tube) (e.g., Genesee Scientific, cat. no. 21-131)
-
37°C shaking incubator (e.g., New Brunswick INNOVA 44/44R)
-
2-ml microcentrifuge tubes (e.g., Genesee Scientific, cat. no. 24-283)
-
1.5-ml disposable, 1-cm cuvettes (e.g., Fisher Scientific, cat. no. 14-955-127)
-
Visible-spectrum cuvette spectrophotometer (e.g., Thermo Scientific GENESYS 30)
-
Centrifuge 24 × 1.5/2 ml, ≥17,000 × g (e.g., Eppendorf Centrifuge 5425)
Setting up overnight cultures of KPPR1
1.Inoculate a single colony of KPPR1 into three 17 × 100-mm culture tubes containing 3 ml low-salt LB broth. Incubate overnight at 37°C with aeration and continuous shaking (200 rpm) at a 60° angle.
Measuring overnight culture density
2.Briefly vortex the overnight cultures and transfer a 100 µl sample to a 1.5 ml disposable cuvette filled with 900 µl of PBS. Mix thoroughly by pipetting.
3.Measure the culture density (OD600) of the sample. Subtract the blank value and multiply by 10 to account for the 1:10 dilution. The resulting value is the OD600 of the overnight culture.
Sedimentation resistance measurement
4.Gently vortex the overnight cultures and transfer 1 OD600 unit to a 2-ml tube.
5.Add 1× PBS to the 2-ml tube for a final volume of 1 ml. Mix the contents thoroughly by vortexing.
6.Centrifuge the sample for 5 min at 1000 × g and room temperature to pellet the bacterial cells.
7.Transfer 900 μl of the upper supernatant into a cuvette.
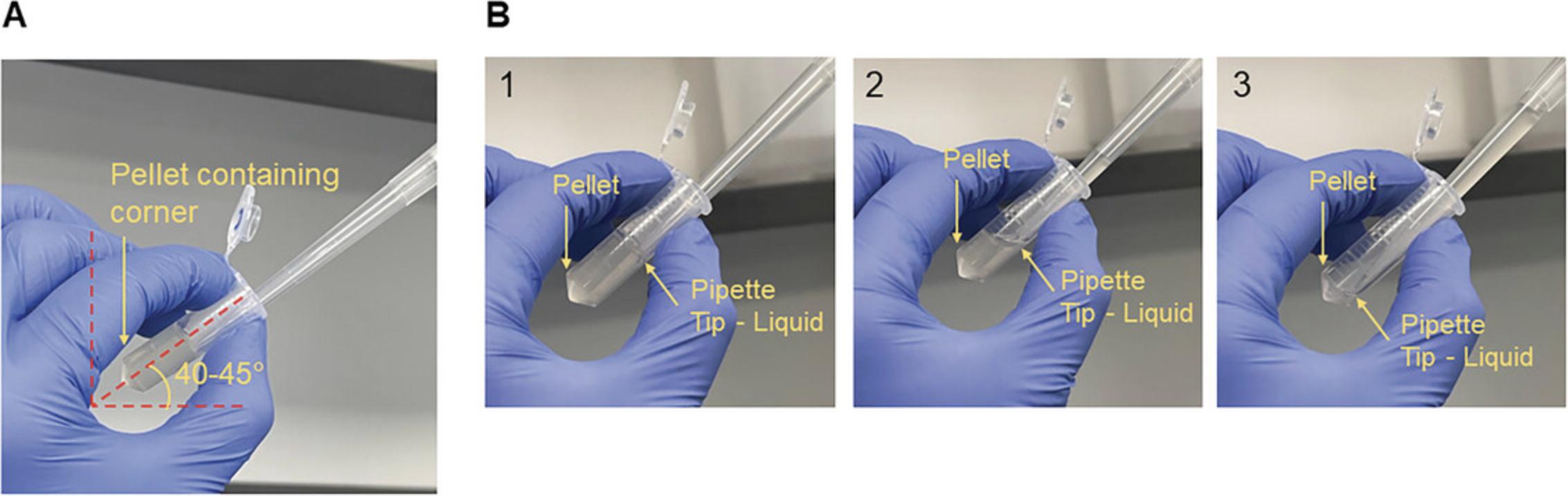
8.Measure the OD600 of the supernatant.
9.Calculate the sedimentation resistance by taking the supernatant OD600 and dividing by the total OD600.
Alternate Protocol 1: 96-WELL PLATE-BASED K. pneumoniae SEDIMENTATION RESISTANCE ASSAY
This alternate protocol describes the adaptation of the cuvette-based sedimentation resistance assay (Basic Protocol 5) to a 96-well plate-based format. A K. pneumoniae strain of interest is cultured overnight in liquid growth medium. Following incubation, the culture density represented by absorbance at 600 nm is measured in a 96-well plate reader. An equal density of culture is centrifuged at slow speed, and the density of cells (OD600) remaining in the supernatant is measured using a 96-well plate reader. This protocol requires following appropriate biosafety measures for working with a biosafety level-2 agent, such as K. pneumoniae. Generally, an increase in mucoviscosity is associated with increased sedimentation resistance. This adaption of Basic Protocol 5 provides an efficient way to measure mucoviscosity for a large number of strains.
Additional Materials
- 96-well flat-bottom plate (sterile or non-sterile) (e.g., Genesee Scientific, cat. no. 25-104)
- Visible-spectrum microplate reader (e.g., Biotek EPOCH2)
- Multichannel pipettor and pipette tips (200 or 300 µl) (e.g., USA Scientific, cat. no. 7112-3300)
- 12-well V-bottom reservoir (e.g., USA Scientific, cat. no. 3823-3412)
Setting up overnight cultures of KPPR1
1.Take three (for triplicate data measurement) 17 × 100-mm culture tubes and label appropriately. Include one additional tube as a negative growth control.
2.Add 3 ml low-salt LB broth to each tube.
3.Suspend one colony of KPPR1 in each tube except the negative growth control tube. Place the cap in the venting position to allow for aeration.
4.Incubate the tubes at 37°C and 200 rpm for 16-18 hr in a 37°C shaking incubator.
Measuring overnight culture density
5.Take a 96-well flat-bottom plate and transfer 270 µl of 1× PBS into three wells. Transfer 300 µl of 1× PBS to three additional wells for blank correction.
6.Briefly vortex the tubes and pipette 30 µl of the culture into the respective wells containing 270 µl of 1× PBS. Mix well by pipetting up and down 5-6 times.
7.Take the OD600 of the diluted samples in a 96-well plate reader.
8.Calculate the overnight OD600 of the culture:
- OD600 = (Sample OD600 – Blank OD600) × dilution factor × plate to cuvette conversion factor
- = (Sample OD600 – Blank OD600) × 10 × 1.181599
Sample OD600: Absorbance of overnight sample at 600 nm
Blank OD600: Absorbance of 300 µl 1× PBS at 600 nm
Dilution factor: Ratio of total volume (culture + PBS) to culture volume used (i.e., 10, if 30 µl sample is mixed with 270 µl 1× PBS)
Plate to cuvette conversion factor: Converts absorbance reading from 96-well plate to 1-cm cuvette absorbance (1.181599). This value should be validated for each plate reader. Refer to Support Protocol 5 for conversion factor determination.
Measuring sedimentation resistance
9.Transfer 1 OD600 unit of gently vortexed overnight culture into a 2-ml tube and bring the final volume to 1000 µl with LB or the culture medium used to grow the bacteria.
10.Centrifuge the tubes for 5 min at 1000 × g and room temperature.
11.Transfer 900 µl of the upper supernatant from the tube to a divided multichannel pipette reservoir. After transferring to the reservoir, pipette the supernatant up and down 5-6 times to mix well.
12.Take a 96-well plate and transfer 300 µl of the well-mixed supernatant from the reservoir to a clean microplate well. Include 300 µl LB (or any other diluent used in step 9) in three wells as blanks.
13.Take the OD600 of the samples in a 96-well plate reader.
14.Calculate the supernatant OD600 of the sample as described below.
- Supernatant OD600 = (Sample OD600 – Blank OD600) × plate to cuvette conversion factor
- = (Sample OD600 – Blank OD600) × 1.181599
Sample OD600: Absorbance of supernatant at 600 nm
Blank OD600: Absorbance of 300 µl LB (or any other diluent used in step 9) at 600 nm
Plate to cuvette conversion factor: Converts absorbance reading from 96-well plate to 1-cm cuvette absorbance (1.181599). This value should be validated for each plate reader. Refer to Support Protocol 5 for conversion factor determination.
15.Calculate the average and standard deviation of the triplicate values and plot the mucoviscosity value as ‘supernatant OD600 per 1 OD600 culture’ or ‘supernatant OD600 per 0.5 OD600 culture’ depending on the density of the overnight culture used in Step 9.
Support Protocol 1: DETERMINATION OF PLATE TO CUVETTE CONVERSION FACTOR
This support protocol outlines the method to establish a ‘conversion factor’ that effectively correlates OD600 readings obtained from a 96-well plate and to a 1-cm cuvette. The process involves making a serial dilution of the bacterial culture and measuring the OD600 using both the microplate reader and cuvette spectrophotometer. By comparing the readings from the two instruments across different dilutions, a conversion factor is determined. The protocol involves the use of a biosafety level 2 pathogen, so appropriate biosafety measures must be followed while handling the organism. The protocol provides a valuable means of converting OD600 readings between systems, thereby enabling the adaptation of cuvette-based lab techniques to a 96-well plate format. This adaptation allows assessment of large numbers of samples at a time.
Additional Materials
- 96-well flat-bottom plate (sterile or non-sterile) (e.g., Genesee Scientific, cat. no. 25-104)
- Visible-spectrum microplate reader (e.g., BioTek EPOCH2)
- Multichannel pipettor and pipette tips (200 or 300 µl) (e.g., USA Scientific, cat. no. 7112-3300)
- 12-well V-bottom reservoir (e.g., USA Scientific, cat. no. 3823-3412)
- 1.5-ml disposable, 1-cm cuvettes (Fisher Scientific, cat. no. 14-955-127)
- 15-ml sterile conical tube (e.g., Griener Bio-One CELLSTAR, cat. no. 181-271)
- Visible-spectrum cuvette spectrophotometer (e.g., Thermo Scientific GENESYS 30)
Conversion of plate OD600 to cuvette OD600
1.Inoculate a single isolated colony of KPPR1 in 4 ml LB and incubate at 37°C with shaking (200 rpm), overnight.
2.Transfer 1 ml of well-vortexed overnight culture to a clean cuvette and measure the OD600 in a spectrophotometer; this is what is referred to as ‘100%’.
3.Serially dilute the sample 1:1 with 1× PBS 10 times to achieve a dilution range from 100% to 0.1953%. Repeat this step to include three sets of dilutions (set A, B, C; triplicate measurement).
4.Transfer 1 ml of each dilution with three replicates to clean 1-cm cuvettes and measure the OD600. Use 1× PBS as a blank.
5.From each dilution tube, transfer 300 µl to a clean 96-well plate, then measure the OD600 in a microplate reader. Include 300 µl of 1× PBS in three wells as a blank.
6.Take the average of the three sets for each dilution.
7.Plot the % dilution on the x-axis and the cuvette OD600 on the y-axis. Identify the linear range.
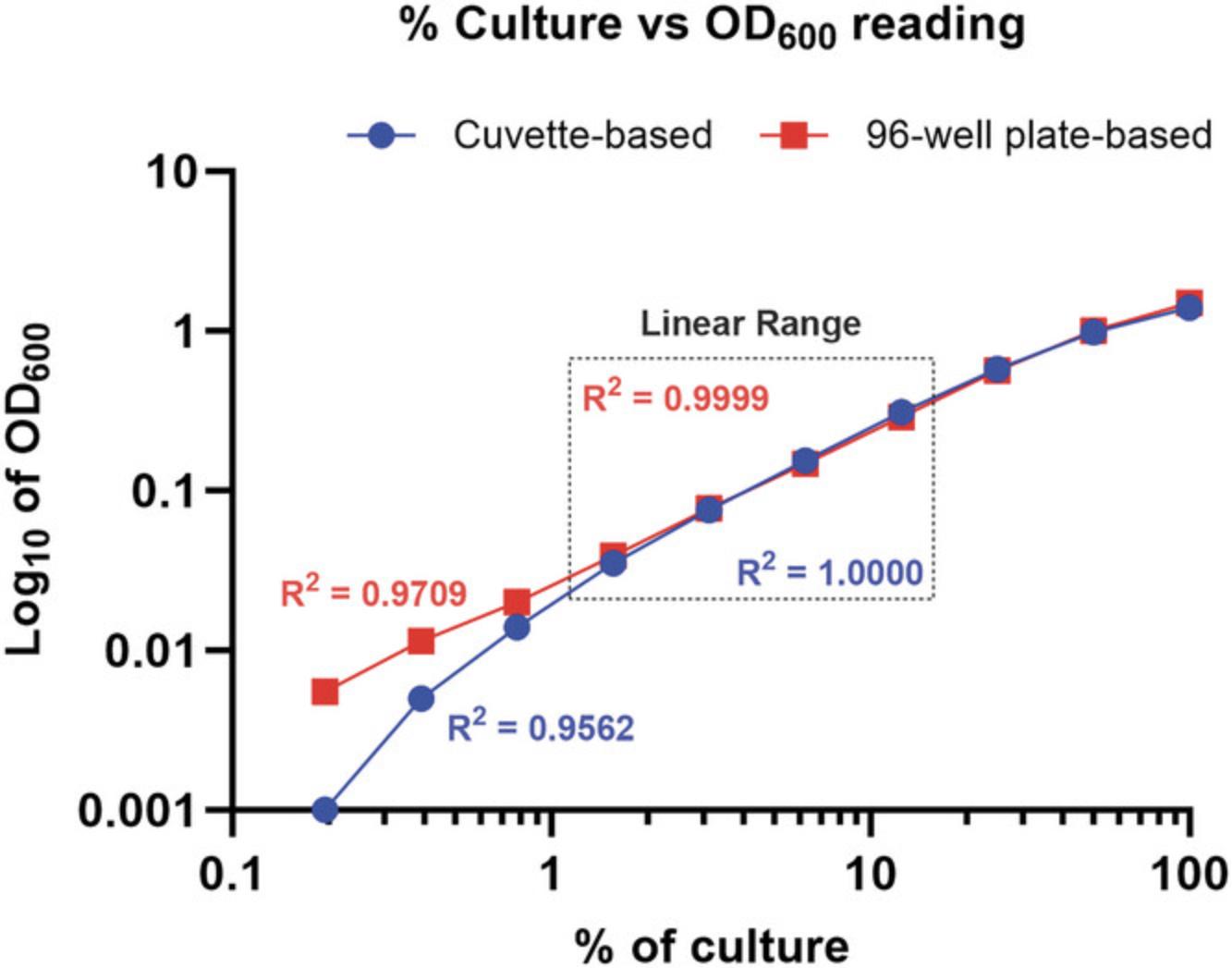
8.Plot the % dilution on the x-axis and the plate OD600 on the y-axis. Identify the linear range.
9.For each % dilution that falls in the linear range for both cuvette and microplate measurements, calculate the cuvette OD600/plate OD600 ratio.
10.Calculate the conversion factor using the following equation.
REAGENTS AND SOLUTIONS
Alcian blue, 0.1%, 100 ml
- 100 ml Alcian blue stain base solution
- 0.1 g Alcian Blue 8GX (ThermoFisher Scientific, cat. no. J60122.14)
Add 0.1 g Alcian blue powder to 100 ml stain base solution. Mix thoroughly using a magnetic stirrer. If precipitate appears even after stirring, warm the solution in a 60°C water bath with periodic stirring for 10 min. Store in the dark at room temperature for up to 1 month.
Alcian blue stain base solution, 1 L
- 600 ml sodium acetate, 20 mM, pH 4.75
- 400 ml Anhydrous ethanol
- Ultrapure water
Prepare 20 mM sodium acetate by adding 1.6407 g sodium acetate to 500 ml ultrapure water. Adjust the pH to 4.75 by adding 6N HCl and raise the final volume to 600 ml. To 600 ml of 20 mM sodium acetate, add 400 ml anhydrous ethanol to prepare 1 L Alcian blue stain base solution. The solution may appear opaque even after mixing. It will not affect the performance. Store up to 1 year at room temperature.
CaCl2, 1 M, sterile, 50 ml
- 5.549 g CaCl2 (M.W. 110 g/mol)
- 50 ml Ultrapure water
Dissolve 5.549 g CaCl2 in 40 ml ultrapure water. Mix well by stirring or vortexing and raise the final volume of the solution to 50 ml using ultrapure water. Sterilize using a 0.2-µm filter. Store up to 1 year at room temperature.
EDTA, 1 M, pH 8.0, sterile, 100 ml
- 58.4 g Anhydrous EDTA (M.W. 584.48 g/mol)
- 100 ml Ultrapure water
Dissolve 58.4 g anhydrous EDTA in 80 ml ultrapure water. Adjust the pH to 8.0 with NaOH pellets with constant stirring. The pH of the solution needs to be 8.0 for EDTA to dissolve. Once completely dissolved, raise the final volume of the solution to 100 ml by adding ultrapure water. Sterilize the solution using a 0.2-µm filter and store up to 1 year at room temperature.
Ethanol, 10%, 500 ml
- 50 ml Anhydrous ethanol
- 450 ml Ultrapure water
Add 50 ml anhydrous ethanol to 450 ml ultrapure water and mix well. Store the solution in a well-sealed container for up to 1 month at room temperature.
Glucuronic acid standards (10 ml each concentration)
- 25 g Glucuronic acid
- Ultrapure water, sterile
Add 0.002 g glucuronic acid to a sterile tube containing 20 ml sterile ultrapure water to make 100 µg/ml glucuronic acid standard. Mix well by vortexing briefly and label the solution ‘100 µg/ml glucuronic acid’. From 100 µg/ml tube, transfer 10 ml of the standard to a new tube containing 10 ml sterile ultrapure water to make 50 µg/ml standard. Mix well by vortexing briefly and label the solution ‘50 µg/ml glucuronic acid’. Repeat the serial dilution by halving the concentration to generate 100, 50, 25, 12.5, 6.25, and 3.125 µg/ml standards. From each tube (100, 50, 25, 12.5, 6.25, 3.125 µg/ml), aliquot each 10 ml stock into five 2-ml tubes and store indefinitely at -20°C.
Low-salt LB agar, 1 L
- 5 g Yeast extract
- 10 g Tryptone
- 0.5 g NaCl
- 20 g Bacto-agar
- Ultrapure water
Weigh all the ingredients and add everything except Bacto-agar to 800 ml ultrapure water in an autoclavable bottle, leaving an equal volume of head space (i.e., 2 L flask or bottle). Mix the solution by using a magnetic stirrer. Once ingredients are fully dissolved, add agar and bring the volume to 1 L with ultrapure water. Heat the solution to boiling until the agar is completely dissolved. Once dissolved, sterilize by autoclaving at 121°C and 15 psi for 20 min in an autoclave bin filled with 1’’ tap water; loosen the cap to ensure that steam can vent. Allow the medium to cool to 60°C by stirring on a stir plate or in a hot water bath. Once cooled to 60°C, aseptically pour about 20 ml medium to a sterile 100-mm Petri dish. Let the agar solidify at room temperature. Store the agar plates for up to 2 weeks at 4°C.
Low-salt LB broth, 1 L
- 5 g Yeast extract
- 10 g Tryptone
- 0.5 g NaCl
- Ultrapure water
Weigh all the ingredients and add to 800 ml of ultrapure water in an autoclavable 1 L bottle. Mix the solution by using a magnetic stirrer. Once ingredients are fully dissolved, bring the volume to 1 L with ultrapure water. Sterilize by autoclaving at 121°C and 15 psi for 20 min. Allow the medium to cool before use. Store up to 1 month at room temperature.
MgCl2, 1 M, sterile, 50 ml
- 4.76055 g MgCl2 (M.W. 95.211 g/mol)
- 50 ml Ultrapure water
Dissolve 4.76055 g MgCl2 in 40 ml ultrapure water. Mix well by stirring or vortexing and bring the volume to 50 ml with ultrapure water. Sterilize the solution using a 0.2-µm filter and store up to 6 months at room temperature.
SDS-PAGE loading buffer, 4×, 50 ml
- 2.22 ml of 0.9 M Tris·HCl, pH 6.8
- 0.8 g SDS
- 1 ml of 100% glycerol
- 1 ml of 0.5 M EDTA
- ≤8 mg Bromophenol blue
- 0.4 ml of 14.7 M BME (2-mercaptoethanol)
- Ultrapure water
Add all the ingredients to 40 ml ultrapure water and mix well by stirring. SDS does not readily go into solution. Slightly warming the mixture in a 60°C water bath can facilitate dissolving the ingredients. Once completely dissolved, bring the volume to 50 ml with ultrapure water. Aliquot 1-2 ml of the loading buffer in microcentrifuge tubes and store up to 1 year at -20°C.
Silver stain gel fixing solution (30% ethanol:10% acetic acid), 500 ml
- 150 ml Anhydrous ethanol
- 50 ml Glacial acetic acid
- 300 ml Ultrapure water
Mix in a bottle by stirring and store up to 6 months at room temperature.
Silver stain stop solution (5% acetic acid), 500 ml
- 25 ml Glacial acetic acid
- 475 ml Ultrapure water
Add 25 ml glacial acetic acid to 475 ml ultrapure water and mix well by stirring. Store up to 6 months at room temperature.
Sodium azide, 5% (w/v), 500 ml
- 25 g Sodium azide
- 500 ml Ultrapure water
Dissolve 25 g sodium azide in 400 ml ultrapure water. Mix well by stirring and bring the volume to 500 ml with ultrapure water. Store up to 6 months at room temperature.
Sodium chloride, 2 M, sterile, 100 ml
- 11.68 g NaCl (M.W. 58.4 g/mol)
- 100 ml Ultrapure water
Dissolve 11.68 g NaCl in 80 ml ultrapure water. Mix well by stirring or vortexing. Bring the volume to 100 ml with ultrapure water. Sterilize the solution using a 0.2-µm filter and store up to 6 months at room temperature.
Sodium hydroxide, 0.125 M, 50 ml
- 0.2499 g Sodium hydroxide
- 50 ml Ultrapure water
Place a magnetic bar in 45 ml ultrapure water in a beaker with a magnetic stir bar. Add 0.2499 g sodium hydroxide to 45 ml ultrapure water and dissolve using the magnetic stirrer. Bring the volume to 50 ml using ultrapure water. Store indefinitely at room temperature.
Sodium tetraborate, 0.0125 M in sulfuric acid (≥95%), 500 ml
- 2.385 g Sodium tetraborate
- 500 ml Concentrated sulfuric acid (>95%)
Follow appropriate safety measures related to acid and corrosive hazards. In a fume hood, transfer 500 ml concentrated sulfuric acid to an acid-resistant glass bottle. Add 2.385 g sodium tetraborate and mix by gently inverting the bottle. If sodium tetraborate crystals are still visible, let the solution sit at room temperature in the fume hood overnight and mix well by inverting before the first use. Store indefinitely at room temperature.
Tris, 1 M, pH 7.5, sterile, 100 ml
- 12.11 g Tris-base (M.W. 121.1 g/mol)
- 15.76 g Tris·HCl (M.W. 157.6 g/mol)
- 100 ml Ultrapure water
Separately prepare 1 M Tris-base (12.11 g Tris-base in 100 ml ultrapure water) and 1 M Tris·HCl (15.76 g Tris·HCl in 100 ml ultrapure water). Mix well by stirring. Titrate 100 ml Tris-base with Tris·HCl with constant stirring until the pH reaches 7.5. Sterilize using a 0.2-µm filter and store up to 3 years at room temperature.
Tris-glycine-SDS gel running buffer, 4 L
- 6.05 g Tris-base
- 28.8 g Glycine
- 2 g SDS
- Ultrapure water
Add all ingredients to 3.5 L ultrapure water and mix well by stirring. Once completely dissolved, bring the volume to 4 L with ultrapure water. Store up to 2 weeks at room temperature.
1% Zwittergent 3-14 in 100 mM citric acid, 50 ml
- 0.5 g Zwittergent 3-14
- 50 ml of 100 mM Citric acid
- Ultrapure water
Prepare 50 ml of 100 mM citric acid by weighing 0.9606 g citric acid and adding ultrapure water to 50 ml. Mix well by using a magnetic stirrer. To 50 ml of 100 mM citric acid, add 0.5 g Zwittergent 3-14 to prepare 1% Zwittergent 3-14 in 100 mM citric acid. Store up to 1 month at room temperature.
COMMENTARY
Background Information
The Klebsiella pneumoniae species complex is comprised of several Klebsiella subspecies that encode a variety of sequence, capsule, and LPS types. Different K. pneumoniae sequence types (STs) are prevalent in different geographical regions. More than 80 capsule types have been identified for K. pneumoniae but only a few are associated with hypervirulent isolates. For example, K. pneumoniae ST258 is the prevalent sequence type in the United States (Kitchel et al., 2009). Similarly, K1 and K2 capsule are more frequently associated with hypervirulent K. pneumoniae (Yu et al., 2008). All of these highlight the importance of characterizing K. pneumoniae.
Basic Protocols 1 and 2 describe the extraction and purification of extracellular polysaccharide (EPS) and are adapted from Favre-Bonte et al. (1999), Domenico et al. (1989), and Blumenkrantz and Asboe-Hansen (1973). Zwittergent 3-14 is a zwitterionic detergent that solubilizes membrane-bound proteins. This causes release of membrane-associated EPS to the culture supernatant which is then precipitated with 80% ethanol. Basic Protocol 2 uses additional steps of treatment with nuclease and proteinase followed by dialysis to remove contaminating nucleic acid and proteins from the EPS. The advantages of Basic Protocol 2 compared to Basic Protocol 1 are its use in the large-scale purification of EPS and the increased purity of the final sample. Samples prepared by Basic Protocol 2 can be used directly for analysis by mass spectrometry or other analytical techniques.
Capsular polysaccharide (CPS) is a major EPS produced by K. pneumoniae. These polysaccharides are generally composed of repeating sugar units connected by glycosidic bonds. The difference in repeating sugar units gives rise to various CPS K-types. KPPR1 synthesizes K2-type CPS containing glucose (Glc), mannose (Man), and glucuronic acid (GlcA) in a repeating sugar unit that includes an →3)β-D-Glcp(1 → 4)β-D-Manp(1 → 4)α-D-Glcp(1 → backbone, an α-D-GlcpA(1 → 3)β-D-Manp (1 → 4) side chain, and approximately 70% of the Man residues are O- acetylated at position 6 (Geyer et al., 1983; Ovchinnikova et al., 2023). Many biological mucopolysaccharides contain uronic acids, which are monosaccharides oxidized to form a carboxylic acid. Therefore, uronic acid is used as a measure of EPS abundance. To quantify uronic acid content, polysaccharides are hydrolyzed in concentrated sulfuric acid/sodium tetraborate at 100°C and then reacted with 3-phenylphenol to form a chromogenic compound (Blumenkrantz & Asboe-Hansen, 1973). The concentration of uronic acid in unknown samples can be determined using the absorbance of this chromogenic compound at 520 nm when compared with a glucuronic acid standard curve.
Hypermucoviscosity is caused by changes in capsular polysaccharide chain length (Khadka et al., 2023; Ovchinnikova et al., 2023). Mucoviscosity was conventionally measured by the ‘string test’. However, this qualitative test yields results that vary between individuals and test conditions. Sedimentation resistance is a feature of hypermucoid colonies. The mechanism behind this phenomenon is not fully understood, but this feature of hypermucoid strains forms the basis of quantifying mucoviscosity in K. pneumoniae. At low-speed centrifugation, hypermucoid colonies sediment poorly compared to non-mucoid colonies. The data, presented as the percentage of bacterial culture suspended in the supernatant after centrifugation, provide efficient mucoviscosity quantification. The sedimentation resistance measured by Basic Protocol 5 and Alternate Protocol 5 are similar. Basic Protocol 5 does not require an additional calculation of ‘conversion factor’ and follows a simpler method than Alternate Protocol 5. However, a large number of samples can be assayed more quickly using Alternate Protocol 5.
Polysaccharide staining can be used to assess the diversity of EPS polymer chains. Long-chain polymers have a higher molecular weight than short-chain polymers. Hence, long-chain polymers run more slowly through an SDS-PAGE gel and appear at a different band size than short polymers, if sufficient time for band separation is allowed. However, EPS polymers are usually high-molecular-weight compounds, making it difficult to separate them by SDS-PAGE. Therefore, the protocol recommends running the electrophoresis for 4-5 hr at 4°C for KPPR1 EPS. Basic Protocol 4 is adapted from Tipton and Rather (2019) and Bonnell et al. (1999). Alcian blue is a cationic dye that binds to anionic polysaccharide. However, the signal of Alcian blue bound to a low concentration of polysaccharide is not easily detected. Therefore, an additional step of silver staining intensifies the signal and facilitates the detection of polysaccharide bands. Silver stain works via binding of silver ions to functional groups, including Alcian blue (Corzo et al., 1991).
Critical Parameters and Troubleshooting
Extracellular polysaccharide isolation and purification
Several steps in the protocol require special attention to ensure that the polysaccharide is properly isolated and purified. The bacterial growth conditions can affect the amount of polysaccharide purified. Conditions such as an agent to induce gene expression, antibiotics to maintain the desired plasmid, enough headspace within tubes for aeration, and incubation with variable shaking can all affect the amount of polysaccharide produced by the bacteria. The conditions ideal to the strain being tested should be maintained for consistent results. Inadvertently pipetting up pellets while removing the upper 100 µl supernatant can overestimate the purified polysaccharide abundance. Drawing the supernatant slowly is critical. Adding ice-cold ethanol and and a longer incubation on ice improves polysaccharide precipitation. At the final step, vortexing the sample with ultrapure water before and after incubation at 37°C ensures the sample is properly mixed and improves rehydration. Unevenly mixed hydrated polysaccharide can affect downstream applications such as uronic acid quantification.
Large-scale isolation and purification of extracellular polysaccharide
The critical parameters described for Basic Protocol 1 are also applicable for this protocol. Furthermore, preventing contamination of the polysaccharide throughout the process is critical. The buffer used for overnight dialysis can be supplemented with 0.05% sodium azide to prevent contamination. Using filter-sterilized solutions whenever possible reduces the chances of contamination. Dialysis membrane cutoff size can be optimized for each case. Ensuring that the final concentration of ethanol during polysaccharide precipitation is 80% is important. Additionally, ‘pre-chilled/cold’ ethanol is recommended for better precipitation of the polysaccharide. The starting culture volume can be adjusted as required for the targeted yield of extracellular polysaccharide. It is very important for the sample to be at frozen solid right before placing it in a lyophilizer. Dipping the glass vial containing sample in liquid nitrogen or methanol-dry ice mix before lyophilization can ensure the small sample volume stays frozen.
Uronic acid quantification assay for quantification of extracellular polysaccharide
It is recommended that culture conditions permitting optimal extracellular polysaccharide synthesis be used. The extracellular polysaccharide purification process, as explained in Basic Protocol 1, can impact the quantification described in this protocol. Refer to Critical Parameters of ‘Extracellular polysaccharide isolation and purification’ to ensure good sample quality. It is critical to ensure the quality and concentration of glucuronic acid standards used in the assay are reliable, as they are used to generate the standard curve for determining the concentration of unknown samples. Repeated freeze-thawing and long-term storage of standards at -20°C can impact the integrity of the standards. Longer incubation on ice for polysaccharide precipitation, longer incubation at 37°C to hydrate the polysaccharide, and avoiding significant delays in A592 measurement between samples can improve assay output; however, cooling the boiled samples on ice should be limited to 5 min. Hypermucoviscous and hypercapsule-producing strains can affect OD600 measurement. In such cases, presenting data as ‘µg/ml uronic acid per CFU/ml’ instead of ‘µg/ml per 1 OD’ may be appropriate. Additional troubleshooting information is listed in Table 1.
Problem | Possible cause | Solution |
---|---|---|
Sample turned black after adding sodium tetraborate in sulfuric acid | Some sugars (e.g., 2-deoxy D-glucose and 2-deoxy D-galactose) dehydrated by concentrated sulfuric acid into a black-colored substance | The change in color after adding 3-phenylphenol in NaOH may still be quantifiable even if the solution has already turned black. If the problematic sugar is not critical for growth, consider preparing media without the problematic sugar. |
No change in color after adding 3-phenylphenol in NaOH | No quantifiable amount of glucuronic acid in the sample | The strain may not have uronic acid as a component of the capsule repeating unit or the abundance of uronic acids may be too low. Try increasing the input number of cells. |
Degraded 3-phenylphenol | Consider repeating the assay with a new batch of 3-phenylphenol. | |
Polysaccharide precipitate lost while removing supernatant ethanol in Basic Protocol 1 | Gently pour off the supernatant ethanol without disturbing the polysaccharide pellet. | |
Uneven change in color across replicates after adding 3-phenylphenol in NaOH | Improper mixing of samples after adding sodium tetraborate in sulfuric acid | It is critical to mix the samples by inverting the tubes multiple times after adding sodium tetraborate to the sample. |
Improper mixing of samples after adding 3-phenylphenol | Ensure that the samples are mixed by pipetting up and down multiple times (at least 6 times). | |
Glucuronic acid standards have an unusual color change | Glucuronic acid degradation after long-term storage or too many freeze-thaw cycles | Prepare a fresh batch of glucuronic acid standards. |
Extracellular polysaccharide visualization by SDS-PAGE and silver stain
In addition to appropriate culture conditions, there are several other steps requiring special attention. Fresh SDS-PAGE running buffer must be used for gel electrophoresis. Reused buffers can lead to non-specific bands or smears in the final image. Running buffer must be kept cold during electrophoresis to avoid the gel overheating. We recommend adding icepacks in the electrophoresis cell to prevent overheating. Avoid touching the gels with bare hands or dirty gloves. If possible, use the corner of the gel where the sample is not expected to be present as an anchor point to touch the gel while moving it. Impure water and unclean staining trays can add background noise to the final image, so ultrapure water and clean staining trays are recommended. The final step of gel development is highly time sensitive. Add the Stop Solution a few seconds before the desired band intensity is reached as the bands continue to develop for a few more seconds after adding the stop solution. Depending on the extracellular polysaccharide molecular weight, the electrophoresis run time may have to be optimized. Different strains produce different amounts of polysaccharide, so if required, the starting sample volume can be increased to >1.5 OD600, if the desired intensity of polysaccharide bands is not obtained. If the Alcian blue-stained gel gets destained quickly upon adding stain base solution, repeat staining with freshly prepared Alcian blue. Do not use the Alcian blue solution after it has separated during storage.
Klebsiella pneumoniae mucoviscosity measurement by sedimentation resistance assay
Several steps within this protocol, if not done properly, can have a significant impact on the assay results. Similar to extracellular polysaccharide purification, culture conditions can affect the mucoviscosity phenotype. Some of the critical agents that require special attention are aeration, salt concentrations, and antibiotics in the growth medium. We have observed that higher salt concentrations, lower pH, and certain antibiotics (e.g., chloramphenicol) can significantly reduce mucoviscosity. Thus, we recommend using low-salt LB broth with a neutral pH for the culture and appropriate controls to assess whether culture additives such as antibiotics impact mucoviscosity. Pipetting the supernatant for OD600 measurement is the most critical step in the assay. Special attention must be given to not accidentally siphon the cell pellet while removing supernatant. Keeping the tube at a 40-45° angle with the pellet facing upwards provides better separation between pellet and the supernatant (Fig. 2). If electric automated pipettors are used, set the pipettor to a minimum drawing speed.
96-well plate-based Klebsiella pneumoniae sedimentation resistance assay
All the critical parameters described for Basic Protocol 5 are also applicable to this protocol. In addition, proper mixing of the overnight sample with 1× PBS (or another diluent) is critical. Improperly mixed samples can lead to erroneous overnight OD600 values, which ultimately affects the volume of sample used for 1 OD600 equivalent culture and hence incorrect supernatant OD600.
Determination of plate to cuvette conversion factor
The culture density is critical for the whole protocol. It is important to use only absorbance values that fall in the linear range of the instrument to calculate the conversion factor. Correspondingly, it is critical that future experimental samples must fall within the linear range of the instrument. In addition, ensuring that samples are well mixed before use is important for obtaining consistent results. Thus, vortexing or vigorous pipetting to mix is encouraged.
Table 1. Troubleshooting Guide for Uronic Acid Quantification Assay for Quantification of Extracellular Polysaccharide
Understanding Results
Extracellular polysaccharide isolation, purification and quantification by uronic acid assay (Basic Protocols 1 and 3)
Following Basic Protocol 1, one should expect to observe a white precipitate or cloudiness after adding ice-cold ethanol. However, this can vary between strains as they differ in extracellular polysaccharide abundance. When Basic Protocol 1 and Basic Protocol 3 are paired, we generally obtain 6-10 µg total uronic acid/OD600 from KPPR1 (cell-attached: ∼5 µg/OD600 and non-attached: ∼3 µg/OD600; Fig. 3).
Large-scale isolation and purification of extracellular polysaccharide
Our lab generally obtains ∼0.2 mg lyophilized product (total extracellular polysaccharide) per 1.5 ml KPPR1 culture by following Basic Protocol 2. The amount obtained may vary depending on the dialysis membrane pore size. Further, we usually get a small amount of Lipid A and O-antigen contamination when using a 25-kDa dialysis membrane. Lipopolysaccharide (LPS) contamination can be visualized by electrophoresis of purified polysaccharide for a shorter time (1 hr) and staining with a Pierce Glycoprotein Staining Kit (ThermoFisher; cat. no. 24562) or Pro-Q Emerald 300 LPS Gel Stain Kit (ThermoFisher; cat. no. P20495).
Extracellular polysaccharide visualization by SDS-PAGE and silver stain
Three distinct smears are expected from KPPR1 upon completing Basic Protocol 4 (Fig. 4). The ‘type A’ band appears as a long smear at the middle of the gel, the ‘type B’ polysaccharides are uniform chain length bands that appear as a single distinct band, and ‘type C’ polysaccharides migrate in an ultra-high molecular weight band at the top of the gel (Khadka et al., 2023; Ovchinnikova et al., 2023). Optimal separation of the KPPR1 capsular polysaccharide smear is obtained after electrophoresis for 4-5 hr. However, due to differences in the power supply, slight variations in time may be required. Distinct bands and/or smears should also be anticipated after de-staining with Silver Stain Base Solution. We recommend using KPPR1 wild-type as a positive control to visualize the three capsule varieties (A, B, and C).
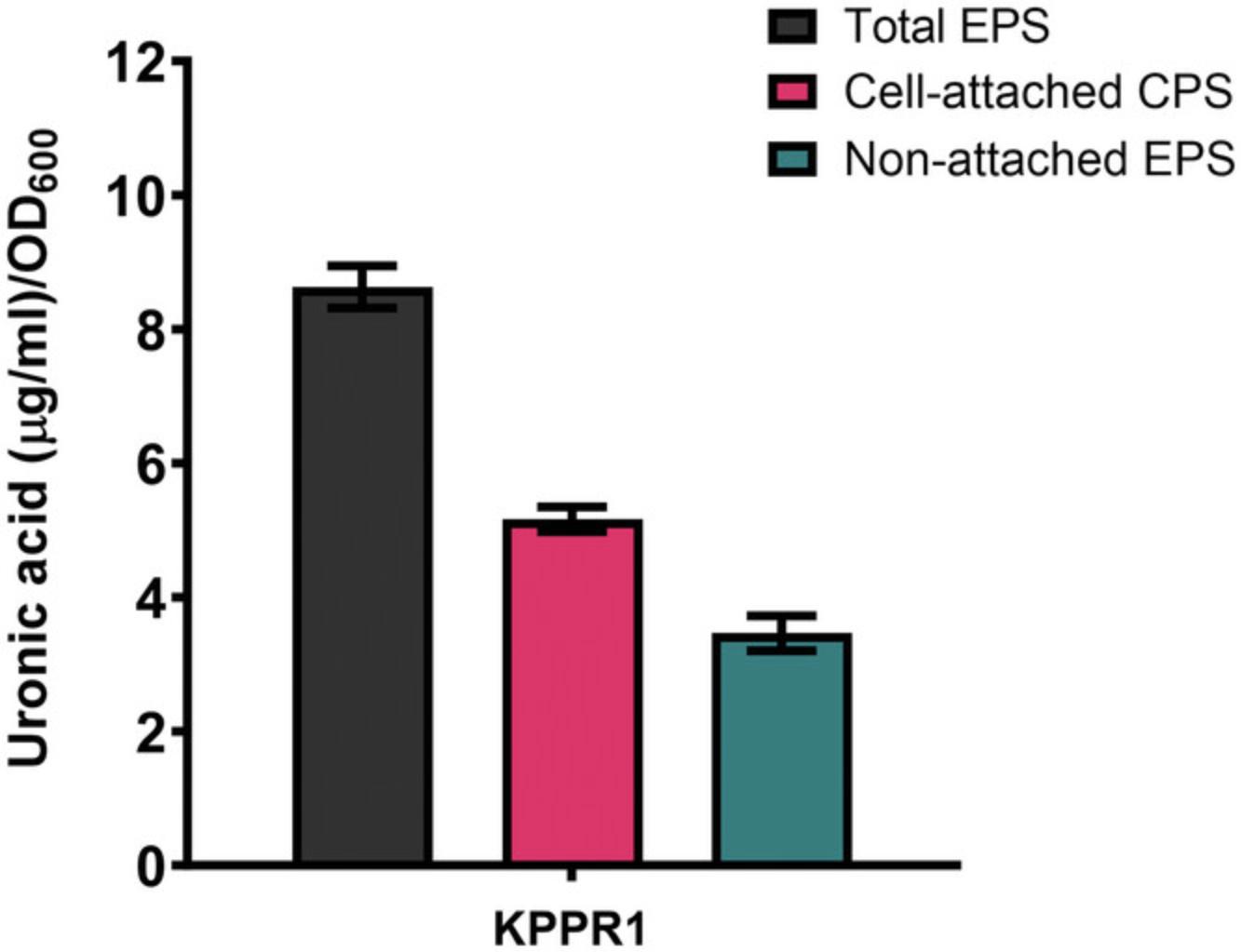
Sedimentation resistance assay (Basic Protocol 5, Alternate Protocol 5 and Support Protocol 5)
After completing either Basic Protocol 5 or Alternate Protocol 5, 0.4-0.6 supernatant OD600 per 1 OD600 unit is anticipated for KPPR1 (Figs. 5 and 6). Slight variations are expected because of interindividual differences in pipette handling. Hypermucoid strains are expected to result in a cloudy supernatant after low-speed centrifugation compared to non-mucoid strains, which tend to result in a clear supernatant. A conversion factor of ∼1.18 is anticipated when 300 µl KPPR1 is assessed using BioTek EPOCH2 plate reader and Thermo Scientific GENESYS 30 Visible Spectrophotometer.
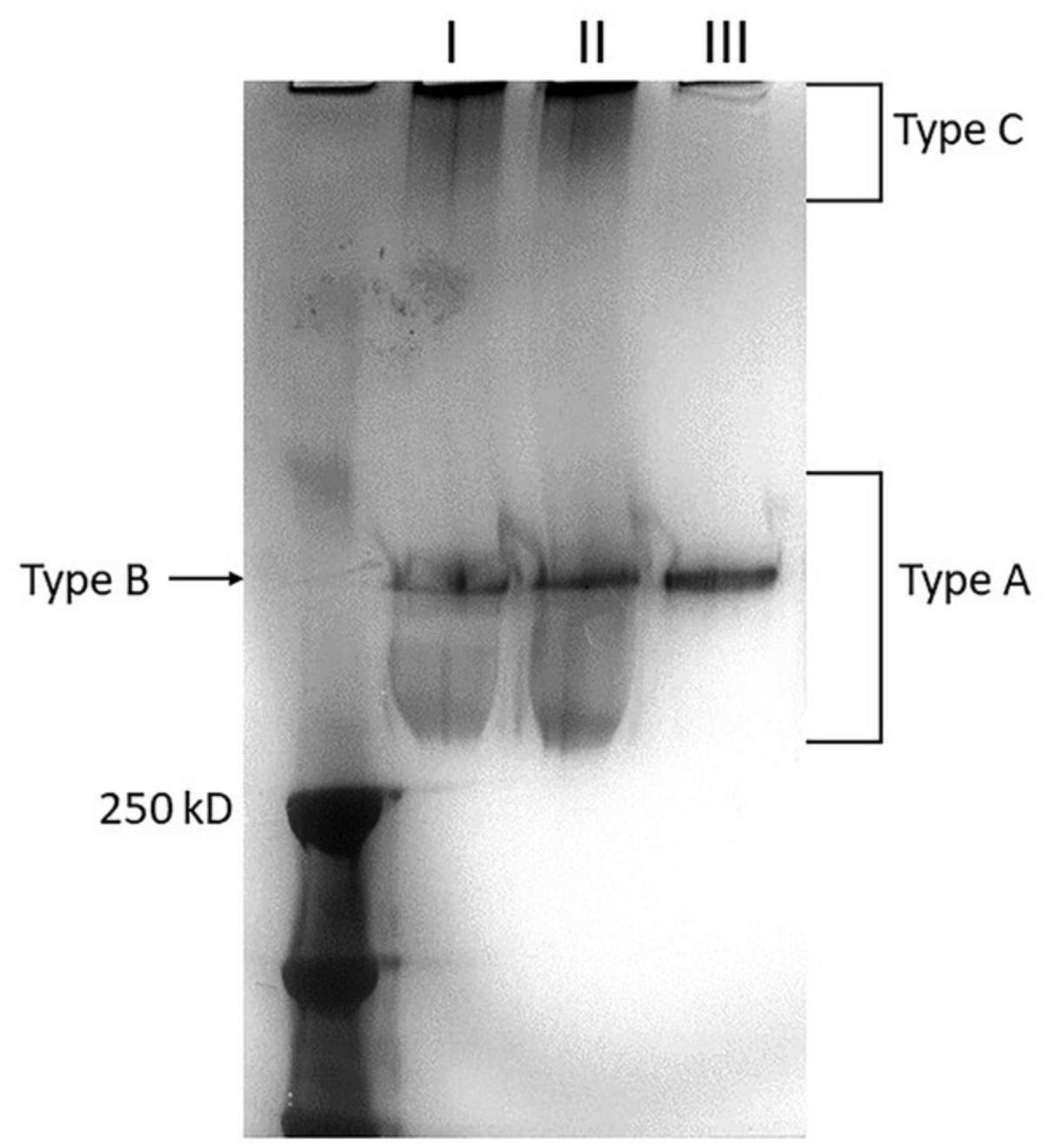
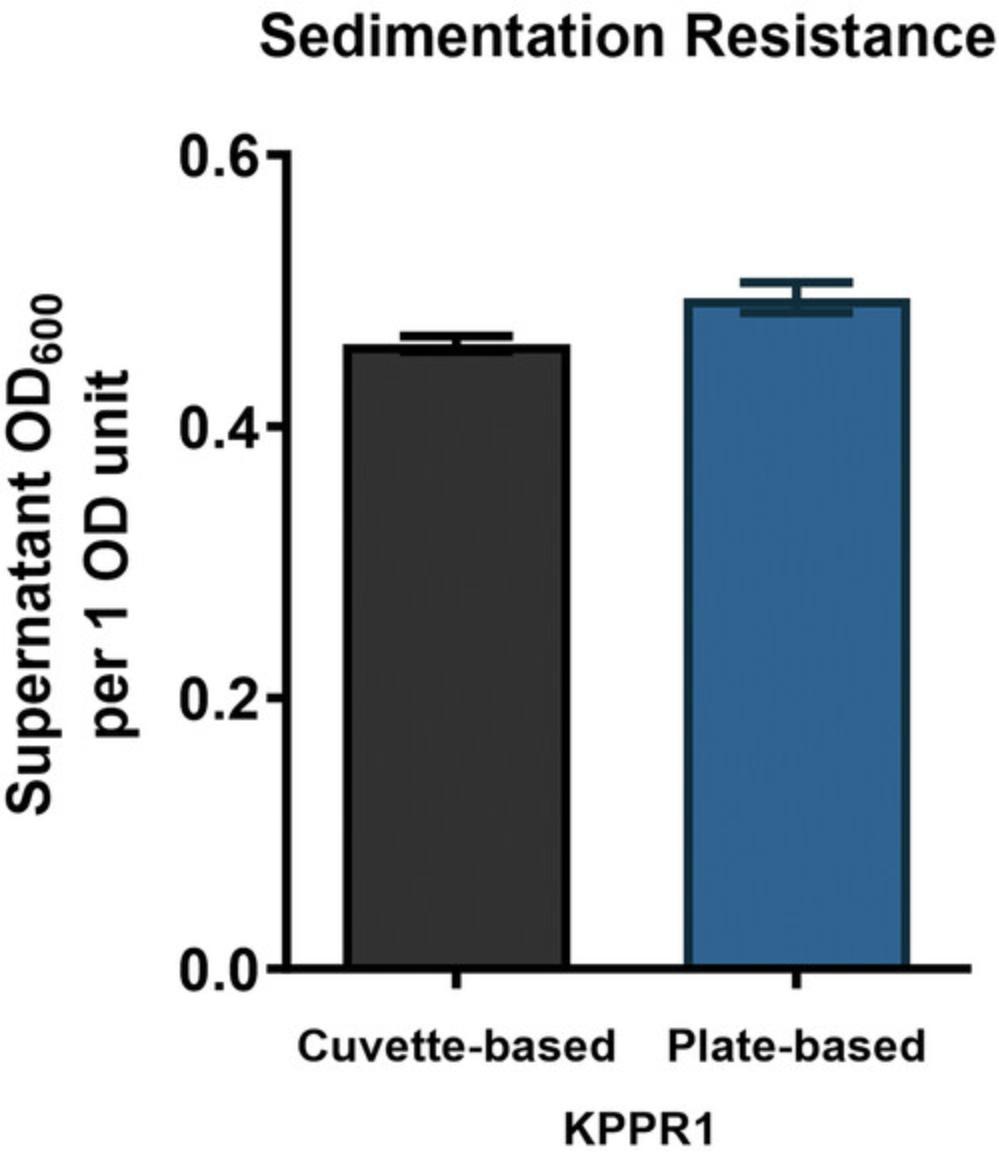
Time Considerations
Basic Protocol 1: Extracellular polysaccharide isolation and purification
Reviving KPPR1 on solid agar media is necessary, which is an overnight process requiring 12 to 15 hr. Preparing overnight cultures of KPPR1 should require only a few minutes. The samples must incubate overnight for 16 to 18 hr in a 37°C shaking incubator to achieve optimal culture density. If measuring the overnight culture density, the time from preparing the 96-well flat bottom plate to measuring the absorbance of the overnight cultures should only require 5 to 10 min.
When purifying the extracellular polysaccharide, there are multiple incubation steps that should take a total of 75 to 80 min. The addition of 10 min of centrifugation result in the protocol taking a total of 1.5 to 2 hr.
Basic Protocol 2: Large-scale isolation and purification of extracellular polysaccharide
The whole protocol takes a minimum of 9 days to complete. Setting up overnight cultures on day 1 takes about 20 min if you already have isolated colonies of strains of interest on an agar plate. On day 2, sub-culturing can take several hours based on the strain type and growth conditions. For example, a 1:100 sub-culture of KPPR1 in LB without any antibiotics takes about 3 to 4 hr to reach the desired OD600. Altogether, it will take a minimum of 6 hr on day 2 to extract KPPR1 EPS. On day 3, dialysis and nuclease treatment take a minimum of 10 hr combined. However, the actual hands-on time can be expected to be around 45 min. Proteinase treatment of EPS on day 4 takes about 40 min of hands-on time. On day 5, proteinase inactivation takes about 2 hr and ethanol precipitation takes about 30 min of hands-on time. On day 6, rehydration takes a minimum of 3.5 hr during the day with actual hands-on time of 30 min. Day 7 takes about 30 min for dialysis setup. Days 8 and 9 take about 30 min of hands-on time each.
Basic Protocol 3: Uronic acid quantification of extracellular polysaccharide
Refer to Basic Protocol 1 for time considerations regarding extracellular polysaccharide purification. To ensure safety when handling sodium tetraborate in sulfuric acid, it is recommended that the reagent is added slowly to each sample. Nonetheless, this step should require only a few mins. There is 10 min of incubation to consider. To ensure sufficient mixing, adding the 3-phenylphenol in NaOH may take several minutes, depending on the use of a single-or multi-channel pipette. Each round of absorbance readings at 520 nm should take only a couple of minutes. The entire protocol requires a total of 30 to 45 min to complete, depending on the number of samples.
Basic Protocol 4: Extracellular polysaccharide visualization by SDS-PAGE and silver stain
Refer to Basic Protocol 1 for time considerations for preparing overnight cultures and measuring overnight culture density. When purifying the extracellular polysaccharide, there are multiple incubation steps that should take a total of 75 to 80 min. The addition of 40 min of centrifugation results in this part of the protocol taking a total of 2 to 2.5 hr. Preparing the cassette for SDS-PAGE and loading the samples in each well will take several minutes. The gel needs to run for approximately 4 to 5 hr for sufficient separation of the polysaccharides. During Alcian blue staining, the gel must shake at room temperature for 1 hr and de-stain overnight. When staining with the Pierce Silver Stain kit, there is an approximate total of 25 min of washing. The gel must be incubated in Pierce Silver Stain solutions for an approximate total of 70 min. The entire protocol from preparing overnight cultures to imaging the gel will take approximately 24 to 26 hr to complete, which includes an overnight de-staining period.
Basic Protocol 5: Klebsiella pneumoniae mucoviscosity measurement by sedimentation resistance assay
Refer to Basic Protocol 1 for time considerations when preparing overnight cultures. There is a 5 min centrifugation step when preparing the samples for mucoviscosity measurement, but vortexing and transferring samples should take only a couple of minutes. The entire protocol requires a total of 20 to 30 min to complete, depending on the number of samples.
Alternate Protocol 5: 96-well plate-based Klebsiella pneumoniae sedimentation resistance assay
Refer to Basic Protocol 1 for time considerations when preparing overnight cultures and measuring overnight culture density. There is a 5 min centrifugation step prior to measuring sedimentation resistance. Loading the 96-well plate with samples and blanks should take only a couple of minutes if using a multichannel pipette. The entire protocol requires a total of 20 to 30 min to complete, depending on the number of samples.
Support Protocol 5: Determination of plate to cuvette conversion factor
Refer to Basic Protocol 1 for time considerations when preparing overnight cultures. Performing three sets of serial dilutions in PBS may take 10 to 15 min. Transferring each dilution to cuvettes and a 96-well microplate may take 5 to 10 min. The absorbance readings will take longer for measuring the cuvettes than with the 96-well microplate, but the total time for both sets of readings should not exceed 10 min, especially if done simultaneously. The entire protocol requires a total of 20 to 30 min to complete, depending on the number of samples.
Acknowledgments
Support for the authors and development of these methods was provided by the University of Toledo College of Medicine and Life Sciences, American Heart Association 23CDA1056712 (L.A.M.), K22 AI145849 (L.A.M.) and R35 GM150588 (L.A.M.) from the National Institutes of Health. The content is solely the responsibility of the authors and does not necessarily represent the official views of the American Heart Association or the National Institutes of Health.
Author Contributions
Saroj Khadka : Conceptualization; data curation; formal analysis; investigation; methodology; validation; original draft writing, review, and editing. Brooke E. Ring : Original draft writing, review, and editing. Drew A. Pariseau : Original draft writing, review, and editing. Laura A. Mike : Conceptualization; funding acquisition; investigation; project administration; resources; supervision; validation; original draft writing, review, and editing.
Conflict of Interest
The authors declare no conflict of interest.
Open Research
Data Availability Statement
Data sharing is not applicable to this article as no new data were created or analyzed in this study.
Literature Cited
- Alvarez, C. A., & Cobb, B. A. (2019). Purification of capsular polysaccharide complex from Gram-negative bacteria. In I. Brockhausen (Ed.), Bacterial Polysaccharides (Vol. 1954, pp. 25–35). Springer New York. https://doi.org/10.1007/978-1-4939-9154-9_3
- Anderson, M. T., Mitchell, L. A., Zhao, L., & Mobley, H. L. T. (2017). Capsule production and glucose metabolism dictate fitness during Serratia marcescens bacteremia. MBio , 8(3), e00740–17. https://doi.org/10.1128/mBio.00740-17
- Blumenkrantz, N., & Asboe-Hansen, G. (1973). New method for quantitative determination of uronic acids. Analytical Biochemistry , 54(2), 484–489. https://doi.org/10.1016/0003-2697(73)90377-1
- Bonnell, B. S., Smith, S. G., & Hedrick, J. L. (1999). Dichromatic staining of electrophoretically departed extracellular matrix macromolecules. Analytical Biochemistry , 271(1), 91–93. https://doi.org/10.1006/abio.1999.4121
- Corzo, J., Pérez-Galdona, R., León-Barrios, M., & Gutiérrez-Navarro, A. M. (1991). Alcian blue fixation allows silver staining of the isolated polysaccharide component of bacterial lipopolysaccharides in polyacrylamide gels. Electrophoresis , 12(6), 439–441. https://doi.org/10.1002/elps.1150120611
- Domenico, P., Salo, R. J., Cross, A. S., & Cunha, B. A. (1994). Polysaccharide capsule-mediated resistance to opsonophagocytosis in Klebsiella pneumoniae. Infection and Immunity , 62(10), 4495–4499. https://doi.org/10.1128/iai.62.10.4495-4499.1994
- Domenico, P., Schwartz, S., & Cunha, B. A. (1989). Reduction of capsular polysaccharide production in Klebsiella pneumoniae by sodium salicylate. Infection and Immunity , 57(12), 3778–3782. https://doi.org/10.1128/iai.57.12.3778-3782.1989
- Favre-Bonte, S., Joly, B., & Forestier, C. (1999). Consequences of reduction of Klebsiella pneumoniae capsule expression on interactions of this bacterium with epithelial cells. Infection and Immunity , 67(2), 554–561. https://doi.org/10.1128/IAI.67.2.554-561.1999
- Geyer, H., Himmelspach, K., Kwiatkowski, B., Schlecht, S., & Stirm, S. (1983). Degradation of bacterial surface carbohydrates by virus-associated enzymes. Pure and Applied Chemistry , 55(4), 637–653. https://doi.org/10.1351/pac198855040637
- Ikuta, K. S., Swetschinski, L. R., Robles Aguilar, G., Sharara, F., Mestrovic, T., Gray, A. P., Davis Weaver, N., Wool, E. E., Han, C., Gershberg Hayoon, A., Aali, A., Abate, S. M., Abbasi-Kangevari, M., Abbasi-Kangevari, Z., Abd-Elsalam, S., Abebe, G., Abedi, A., Abhari, A. P., Abidi, H., … Naghavi, M. (2022). Global mortality associated with 33 bacterial pathogens in 2019: A systematic analysis for the Global Burden of Disease Study 2019. The Lancet , 400(10369), 2221–2248. https://doi.org/10.1016/S0140-6736(22)02185-7
- Khadka, S., Ring, B. E., Walker, R. S., Krzeminski, L. R., Pariseau, D. A., Hathaway, M., Mobley, H. L. T., & Mike, L. A. (2023). Urine-mediated suppression of Klebsiella pneumoniae mucoidy is counteracted by spontaneous Wzc variants altering capsule chain length. https://doi.org/10.1128/msphere.00288-23
- Kitchel, B., Rasheed, J. K., Patel, J. B., Srinivasan, A., Navon-Venezia, S., Carmeli, Y., Brolund, A., & Giske, C. G. (2009). Molecular epidemiology of KPC-producing Klebsiella pneumoniae isolates in the United States: Clonal expansion of multilocus sequence type 258. Antimicrobial Agents and Chemotherapy , 53(8), 3365–3370. https://doi.org/10.1128/AAC.00126-09
- Mike, L. A., Stark, A. J., Forsyth, V. S., Vornhagen, J., Smith, S. N., Bachman, M. A., & Mobley, H. L. T. (2021). A systematic analysis of hypermucoviscosity and capsule reveals distinct and overlapping genes that impact Klebsiella pneumoniae fitness. PLOS Pathogens , 17(3), e1009376. https://doi.org/10.1371/journal.ppat.1009376
- Ovchinnikova, O. G., Treat, L. P., Teelucksingh, T., Clarke, B. R., Miner, T. A., Whitfield, C., Walker, K. A., & Miller, V. L. (2023). Hypermucoviscosity regulator RmpD interacts with Wzc and controls capsular polysaccharide chain length. MBio , 14(3), e00800–23. https://doi.org/10.1128/mbio.00800-23
- Pan, Y. J., Lin, T. L., Chen, C. T., Chen, Y. Y., Hsieh, P. F., Hsu, C. R., Wu, M. C., & Wang, J. T. (2015). Genetic analysis of capsular polysaccharide synthesis gene clusters in 79 capsular types of Klebsiella spp. Scientific Reports , 5(1), 15573. https://doi.org/10.1038/srep15573
- Tipton, K. A., & Rather, P. N. (2019). Extraction and visualization of capsular polysaccharide from Acinetobacter baumannii. In I. Biswas & P. N. Rather (Eds.), Acinetobacter baumannii (Vol. 1946, pp. 227–231). Springer New York. https://doi.org/10.1007/978-1-4939-9118-1_21
- Yu, W. L., Ko, W. C., Cheng, K. C., Lee, C. C., Lai, C. C., & Chuang, Y. C. (2008). Comparison of prevalence of virulence factors for Klebsiella pneumoniae liver abscesses between isolates with capsular K1/K2 and non-K1/K2 serotypes. Diagnostic Microbiology and Infectious Disease , 62(1), 1–6. https://doi.org/10.1016/j.diagmicrobio.2008.04.007
Citing Literature
Number of times cited according to CrossRef: 1
- Drew A. Pariseau, Brooke E. Ring, Saroj Khadka, Laura A. Mike, Cultivation and Genomic DNA Extraction of Klebsiella pneumoniae, Current Protocols, 10.1002/cpz1.932, 4 , 1, (2024).