Characterization and Functional Analysis of Tumor-Derived Microparticles
Jingwei Ma, Jingwei Ma, Ke Tang, Ke Tang, Huafeng Zhang, Huafeng Zhang, Keke Wei, Keke Wei, Bo Huang, Bo Huang
Abstract
Microparticles (MPs) are heterogeneous populations of cell-derived vesicles that play an important role in intercellular communications. The release of MPs by tumor cells is a very common event in tumor microenvironments (TMEs). Tumor cell−derived MPs (T-MPs) contain a variety of bioactive molecules, thus modulating various biological processes, including the regulation of immune cell phenotype and function, as well as immune responses. Moreover, T-MPs can be used as natural carriers to deliver therapeutic drugs into tumor cells and immune cells, thus remodeling TMEs and modifying anti-tumor immune responses. These features allow T-MPs to function as potential biomaterials to be applied in tumor immunotherapies and vaccines. This article describes protocols for the isolation of T-MPs from supernatants of cultured tumor cells by multi-step centrifugations. Tools and protocols are also provided in order to characterize and validate the isolated MPs and to analyze the interaction between T-MPs and different target cells. © 2021 The Authors. Current Protocols published by Wiley Periodicals LLC.
Basic Protocol 1 : Isolation of tumor cell-derived microparticles by multi-step centrifugations
Basic Protocol 2 : Characterization and validation of tumor cell−derived microparticles
Basic Protocol 3 : Functional analysis of the uptake of tumor cell−derived microparticles by different cell types
INTRODUCTION
During activation or cell apoptosis, cells undergo cytoskeletal changes and membrane rearrangement, leading to the formation and release of microparticles (MPs; Cocucci, Racchetti, & Meldolesi, 2009). They are heterogeneous populations with small plasma membrane structures ranging in size from 100 to 1000 nm (Mause & Weber, 2010). In 1967, MPs were first described as “dust” when Wolf (1967) reported platelet membrane fragments in human plasma. In fact, almost all cell types can release MPs. MPs contain a variety of bioactive molecules, including proteins, biolipids, RNAs, and even DNA fragments, which can be transferred between cells, thus facilitating intercellular communications.
In tumor microenvironments, tumor cells can also release MPs into extracellular space or bloodstream (Ma, Zhang, Tang, & Huang, 2020). Various factors including damage-associated molecular patterns (DAMPs), nutrient deficiency and hypoxia, chemotherapy, and radiotherapy can act on tumor cells and induce them to release T-MPs (Chen et al., 2020; Li et al., 2012). T-MPs have been successfully detected in body fluids of cancer patients, and represent a unique biomarker for tumor diagnosis (Gong, Jaiswal, Dalla, Luk, & Bebawy, 2015). Due to their enrichment in cytoplasmic contents of parental cells, T-MPs are well demonstrated as important modulators of the immune system (Thery, Ostrowski, & Segura, 2009; Zhou et al., 2020). T-MPs act as a double-edged sword in tumor immunology (Ma et al., 2020). They can be taken up by macrophages, and induce the development of macrophages toward an M2-like phenotype, thus inhibiting antitumor immune responses in TME (Geddings & Mackman, 2013; Zhang et al., 2018). On the other hand, they can be taken up by dendritic cells and play an important role in antitumor immunity by activating the cGAS-STING signaling pathway, conferring on T-MPs a potential role in tumor vaccines (Zhang et al., 2015). Furthermore, considering the similarities in size, structure, and vector function between artificial nanoparticles and T-MPs, T-MPs can also be used as endogenous natural vehicles that deliver therapeutic drugs to tumor cells or even immune cells, thus remodeling TME and resetting antitumor immune response (Ma et al., 2016; Xu et al., 2020).
Studies on multiple features of T-MPs have led to the development of therapeutic and diagnostic strategies. Therefore, to improve the diagnostic and therapeutic utility of T-MPs, an isolation method enabling users to obtain a high yield and good purity of T-MPs is required. Due to the inhomogeneity of T-MPs, further identification and characterization are required after T-MPsisolation, which are also helpful to improve the isolation methods and to prepare T-MPs with high quality. Different types of parent cells and different induction modes (ultraviolet, drugs, etc.) may generate different size distributions and distinct markers of T-MPs. Mainly, T-MP size can range from 200-1000 nm, and T-MPs may be annexin V positive if tumor cell apoptosis is induced. In general, membrane molecules or markers of parental tumor cells may also be displayed in T-MPs. For example, CD44 is highly expressed on tumor cells, and is also present in T-MPs. In addition to proteins, RNA and other molecules can be used to identify specific T-MPs.
Here, we describe protocols for the isolation of T-MPs and methods for the characterization and validation of T-MPs, together with immunological functional analysis. In Basic Protocol 1, we describe how to isolate T-MPs or drug-MPs from cell culture supernatants using multi-step differential centrifugations. In Basic Protocol 2, we describe how to apply multiple techniques for MP characterization, including electron microscopy, nanoparticle tracking analysis, and protein analysis. Finally, in Basic Protocol 3, we describe some experiments to assess the uptake of T-MPs by immune cells and tumor cells, and for functional analysis.
Basic Protocol 1: ISOLATION OF TUMOR CELL−DERIVED MICROPARTICLES BY MULTI-STEP CENTRIFUGATIONS
This protocol describes a series of procedures required to isolate T-MPs and drug-loaded T-MPs from tumor cell culture supernatant based on differential centrifugation. The protocol begins with the method for tumor cell culture. Tumor cells are exposed to ultraviolet irradiation for 1 hr in order to release T-MPs. 16 hr later, T-MP-containing supernatants are harvested and then undergo a series of differential centrifugations aimed at the removal of cells, cell debris, and apoptotic bodies, ending with a high-speed ultracentrifugation step to pellet T-MPs. The general principles of a basic differential ultracentrifugation workflow for T-MP isolation are outlined in Figure 1.
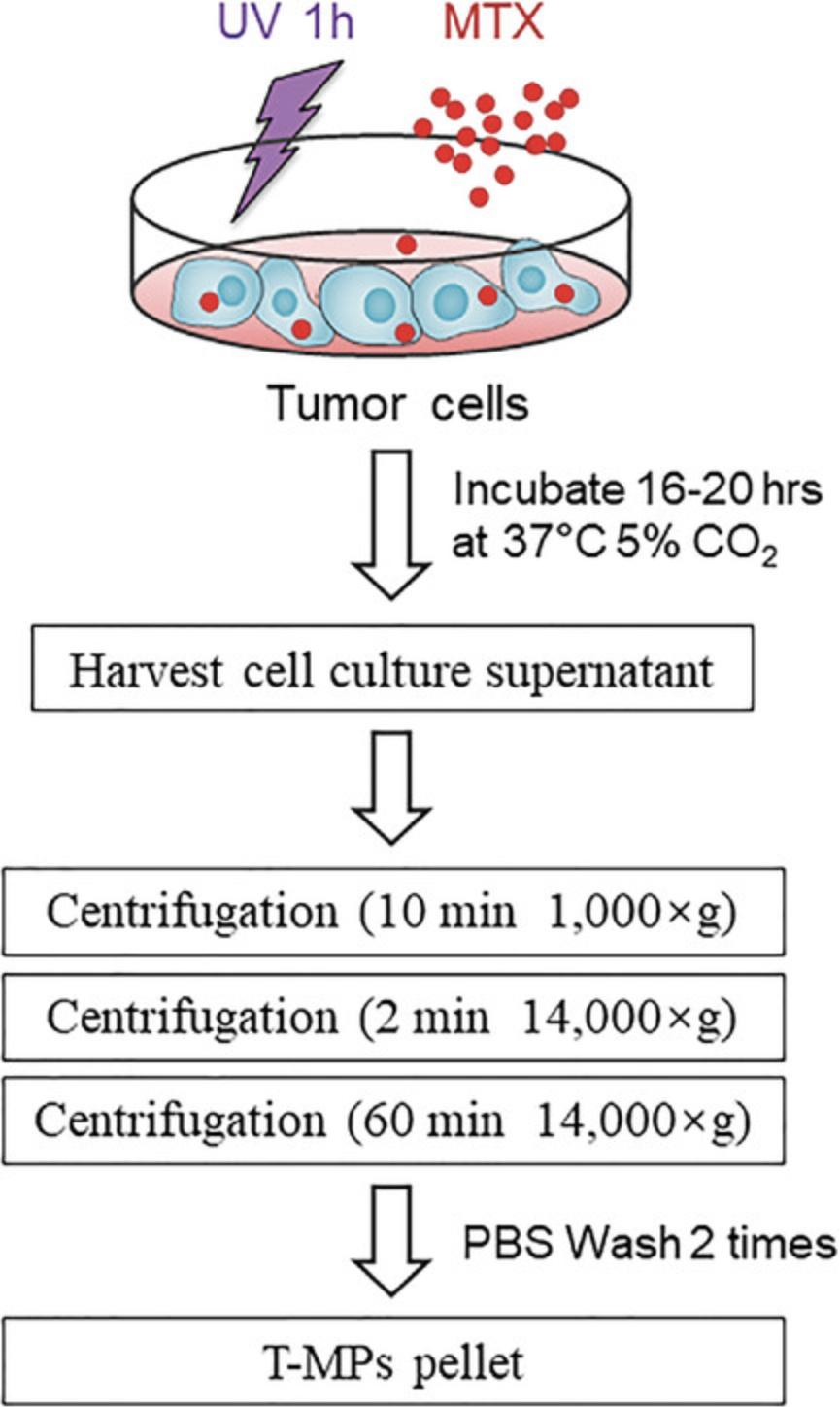
NOTE : Murine or human tumor cell lines used for T-MP studies should be validated to confirm their cellular origin. All tumor cells must be Mycoplasma-negative.
Materials
-
We used the following murine or human tumor cell lines:
- H22 mouse hepatocarcinoma cells (China Center for Type Culture Collection)
- B16 mouse melanoma cell (e.g., ATCC® CRL-6323™)
- A549 human lung cancer cell line (e.g., ATCC® CCL-185™)
- HL-60 human leukemia cell (e.g., ATCC® CCL-240™)
-
Culture medium: RPMI-1640 (e.g., Gibco, cat. no. 61870127) or DMEM (e.g., Gibco, cat. no. 11965118) supplemented with 1% (v/v) penicillin/streptomycin (Beyotime, cat. no. C0222) and 10% (v/v) heat-inactivated FBS (sterile filter and store up to 2 months at 4°C)
-
Methotrexate (MTX, Heng Rui Pharmaceutical)
-
PBS, no calcium or magnesium (e.g., Gibco, cat. no. 14190144; also see recipe)
-
FBS-free culture medium: e.g., RPMI-1640 (Gibco, cat. no. 61870127) or DMEM (Gibco, cat. no. 11965118)
-
100-mm TC-treated culture dishes (e.g., Corning®, cat. no. 430167)
-
37°C, 5% CO2 cell culture incubator
-
Ultraviolet radiation source
-
10-ml serological pipets (e.g., Corning®, cat. no. 4488)
-
50-ml sterile polypropylene centrifuge tubes (e.g., Corning®, cat. no. 430828)
-
Centrifuge (e.g., Eppendorf 5810R) and microcentrifuge
-
1.5-ml microcentrifuge tube (e.g., Thermo Scientific™, cat. no. AM12400)
Preparation of T-MP-containing conditioned medium
1.Culture 2 × 106 A549 cells in a 100-mm cell culture dish using 10 ml DMEM supplemented with 1% (v/v) penicillin/streptomycin and 10% (v/v) heat-inactivated FBS at 37°C with 5% CO2.
2.When the tumor cells reach about 80% confluence, expose to ultraviolet irradiation (UVB, 300 J/m2) for 1 hr in a biosafety cabinet. For isolation of drug-loaded MPs, add drugs (e.g., MTX, 2 mg/ml) to medium after ultraviolet irradiation.
3.Incubate 16-20 hr at 37°C with 5% CO2.
Harvest cell culture supernatant and perform centrifugations
4.Harvest cell culture supernatant into a 50-ml polypropylene tube using a sterile pipet at 16 hr after ultraviolet irradiation. Wash out the dish with PBS twice to make sure that the T-MPs or drug-MPs sticking to the dish are collected.
5.Centrifuge cell culture supernatant 10 min at 1000 × g , 4°C.
6.Transfer the supernatant to a new 50-ml polypropylene tube and centrifuge 2 min at 14,000 × g , 4°C.
7.Transfer the supernatant to a new 50-ml polypropylene tube and centrifuge 60 min at 14,000 × g , 4°C, to pellet T-MPs.
8.Gently remove tubes from centrifuge and discard the supernatant from each tube.
9.Add 1 ml pre-chilled PBS and transfer the resuspended pellet to a new 1.5-ml microcentrifuge tube.
10.Wash the pellet twice, each time microcentrifuging 60 min at 14,000 × g , 4°C.
11.Finally, resuspend the T-MPs or drug-MPs pellet in FBS-free culture medium or PBS for the subsequent experiments.
Basic Protocol 2: CHARACTERIZATION AND VALIDATION OF TUMOR CELL-DERIVED MICROPARTICLES
Due to the small size (100-1000 nm) and detection approach limitation, verification of extracellular vesicles should rely on a combination of multiple methods, including electron microscopy (EM, including scanning electron microscopy, SEM, or transmission electron microscopy, TEM), nanoparticle tracking analysis (NTA), flow cytometry (FCM), specific protein detection, and so on. EM is used to observe the morphology and structure, allowing T-MP detection with 100% confidence. In T-MP counting, FCM is used to determine T-MP number, but presently NTA offers more of an advantage in counting and tracking. Nevertheless, FCM is widely used for fluorescence staining on T-MPs, which is used for T-MP tracing in vitro and in vivo, and also used to detect protein and other molecular probes. Meanwhile, proteins carried by T-MPs can be detected by immunogold staining EM or western blotting. Because their heterogeneity and small size make T-MPs complex and poorly reproducible, at least two of the methods mentioned above should be used to characterize tumor cell-derived microparticles. (Thery et al., 2018).
Additional Materials (also see Basic Protocol 1)
-
T-MP suspension (Basic Protocol 1)
-
4% paraformaldehyde (PFA)
-
1% osmium tetroxide (OsO4)
-
Graded ethanol series
-
1% uranyl acetate
-
Ultrapure water (Heal ForceR Water Purification System; (Heal ForceR, cat. no. NW10VF)
-
APC-anti human CD44 antibody (Biolegend, cat. no. 338806)
-
APC mouse IgG1, κ (isotype control antibody; Biolegend, cat. no. 400119)
-
Protein lysis buffer for western blotting, containing 1% SDS
-
3% Triton X-100
-
10 mg/ml proteinase K stock solution
-
10 μg/ml daunorubicin (e.g., Heng Rui Pharmaceutical)
-
1 mM phenylmethylsulfonyl fluoride (PMSF)
-
400 mg/ml ZnSO4
-
Methanol
-
Filter (0.1 μm and 1 μm; Millipore, Merck)
-
Copper grids coated with formvar/carbon (see Current Protocols article: Burghardt & Droleskey, 2006)
-
Transmission electron microscope (TEM; JEOL, cat no. JEM1010)
-
Nanoparticle Tracking Analysis (NTA) instrument (Malvern Panalytical Nano Sight NS300)
-
Flow cytometer (Accuri C6, BD Bioscience)
-
Analysis software for flow cytometry data (e.g., FlowJo software)
-
Column with parameters including particle size 5 μm and 4.6 × 150 mm2 specification (Waters, cat. no. 186002737)
-
HPLC system with 2475 Multi-Wavelength Fluorescence Detector, 1525 Binary HPLC Pump, and 717 Plus Autosampler (Waters Corporation, Milford, CT)
-
Empower 2 software (Waters Corporation)
-
Additional reagents and equipment for electron microscopy (see Current Protocols article: Burghardt & Droleskey, 2006) and western blotting (immunoblotting; see Current Protocols article: Ni, Xu, & Gallagher, 2016)
Imaging of T-MPs using electron microscopy
Electron microscopy (EM) is the gold standard method to identify MP structure and morphology. Detection of proteins in MPs by immunogold staining in conjunction with EM is also considered a reliable method. For more detail on the electron microscopic procedures described below, see Current Protocols article Burghardt & Droleskey (2006).
NOTE : Sample preparation for EM requires specialized technicians, especially for fixation of T-MPs and ultrathin sectioning. High-purity T-MPs are needed for EM observation, and a suitable fixing solution concentration and well-prepared sections are key to obtaining these.
1a. Pass T-MPs through 1-μm filter to remove debris.
2a. Keep the concentration of T-MPs at ≥ 5 × 108/100 μl (as previously determined by NTA; see steps 1b-6b, below).
3a. Add 10-15 μl T-MPs suspended in PBS on copper grid coated with formvar/carbon in a dish with Parafilm on the bottom.
4a. Keep dish covered with lid to avoid contamination on the grid. Incubate T-MPs on grid for 20 min at room temperature.
5a. Add 100 µl of 4% PFA onto the grid on the Parafilm and incubate at room temperature for 1 hr.
6a. Add 1 ml PBS onto the grid and shake it slowly for 5 min.
7a. Add 100 μl of 1% OsO4 and incubate at room temperature for 30 min.
8a. After washing with ultrapure water, treat the pellets with a graded ethanol which contains 1% uranyl acetate in 50% ethanol for 30 min, and then embed them in OsO4.
9a. Polymerize samples at 60°C overnight.
10a. Prepare ultrathin sections and observe under EM (see Current Protocols article: Burghardt & Droleskey, 2006).
Measurement and counting of T-MPs by nanoparticle tracking analysis (NTA)
The technique of NTA offers real-time, multiple-functional analysis of nanoparticles in liquid suspensions, including concentration, size distribution, and direct imaging. Particles in a sample can be visualized in real time by a charge-coupled device (CCD) camera through tracing the light scattering. By means of this process, each particle's Brownian motion is recorded in real time, then size and distribution are tracked by image recording. Finally, data are processed and analyzed by software. If particles are labeled with fluorescent dye, the positively stained particles can also be detected by fluorescence. For the optimal instrument performance, the procedure should be carried out as follows.
1b. Fill the fluidics of the NTA instrument with particle-free ultrapure water (which has been passed through a 0.1-μm filter), making sure no bubbles are in the sample chamber and tubing.
2b. Adjust NTA parameters under flow conditions with undiluted T-MP stock to determine the concentration of T-MPs.
3b. Dilute the T-MP stock in PBS to obtain an appropriate concentration, which should range from 20-60 particles per field.
4b. The image contrast can be adjusted by the Threshold in Advanced Camera settings.
5b. Make sure that at least 200 completed tracks are analyzed in each video recording. Each sample should be repeatedly analyzed three times to balance out sample inhomogeneity.
Surface marker staining of T-MPs by flow cytometry
T-MPs are shed from tumor cells and inherit membrane protein; thus, the T-MPs may be characterized through a tumor cell−specific marker.
1c. After isolation, resuspend T-MPs with PBS that has been prefiltered through a 0.1-μm filter.
2c. Pass T-MPs in PBS through a 1-μm filter to further exclude background noise or nonspecific events.
3c. Centrifuge 60 min at 14,000 × g , and resuspended pelleted T-MPs with 100 μl filtered PBS.
4c. Add 5 μl APC-anti human CD44 antibody (1:100) and co-incubate on ice in dark area for 60 min. Use 5 µl APC Mouse IgG1 isotype control antibody (1:100) for isotype control.
5c. Wash T-MPs with PBS twice and resuspend T-MPs with 100-µl prefiltered PBS.
6c. Detect CD11b-positive T-MPs with flow cytometry, using isotype antibody group for baseline setup.
Detection of T-MPS protein by western blotting
T-MPs encapsulate a large amount of information from the parent cell, including that from proteins, RNAs, and lipids. Specific protein detection is generally used to verify the source of MPs, more so than RNA or other macromolecules. In addition to flow cytometry and immunogold staining (TEM), western blotting (WB) is also a classic method for protein detection. The detailed protocol (see Ni et al., 2016) on MP protein detection by WB is similar to the procedure for WB on the parent cell. Specific issues are mentioned as follow.
1d. Pass T-MPs through a 1-μm filter to remove debris.
2d. Protein lysis buffer should be added.
3d. The housekeeping protein is not invariable; β-actin is missed in some T-MP samples.
Detection of drug concentration in T-MPs by high-performance liquid chromatography (HPLC)
To detect the drug concentration in tumor-cell derived microparticles, drugs should be first extracted and then detected by HPLC. Doxorubicin-encapsulated H22 derived MPs are as an example described in the following steps.
1e. Wash drug-encapsulated H22-MPs with PBS twice using the centrifugation conditions described in Basic Protocol 1.
2e. Resuspend T-MPs in 5 μl of 3% Triton X-100 and 190 μl of PBS.
3e. Add 5 µl of 10 mg/ml proteinase K stock solution to sample.
4e. Incubate samples in a water bath at 65°C for 45 min.
5e. Add 56.4 μl of 10 μg/ml daunorubicin. Make up to 400 μl final volume with PBS.
6e. Add 5 µl of 1 mM phenylmethylsulfonyl fluoride and incubate for 10 min, then add 10 μl of 0.4 M MgCl2 and 20 μl of 1 µg/ml DNase I to sample.
7e. Centrifuge 10 min at 12,000 × g , then incubate the sample in a 37°C water bath for 30 min. Add 45 μl of 400 mg/ml ZnSO4 mixed with 450 μl methanol to 450 μl of each sample.
8e. Mix the sample, then centrifuge 5 min at 12,000 × g , and collect 100 μl of the supernatant for HPLC analysis.
9e. Perform HPLC using a column with parameters including particle size 5 μm and 4.6 × 150 mm2 specification and HPLC system with 2475 Multi-Wavelength Fluorescence Detector, 1525 Binary HPLC Pump, and 717 Plus Autosampler.
10e. Monitor peaks at a 480 nm excitation wavelength and a 560 nm emission wavelength at 40°C. Analyze tyhe chromatographic peaks with Empower 2 software.
Basic Protocol 3: FUNCTIONAL ANALYSIS OF THE UPTAKE OF TUMOR CELL-DERIVED MICROPARTICLES BY DIFFERENT CELL TYPES
T-MPs can be used for functional analysis after collection by co-incubation with tumor cells or immune cells. Here, we describe different downstream applications of T-MPs. For functional studies, the first step is to confirm whether the T-MPs can be taken up by target cells. Flow cytometry is a convenient method to quantify the uptake of labeled T-MPs by target cells. High-resolution fluorescence microscopy is an intuitive method to observe the precise localization of T-MPs in target cells. In this protocol, T-MPs are stained with PKH67 (green) for fluorescent labeling, and then co-incubated with dendritic cells, macrophages, or tumor cells. Finally, the uptake rate is analyzed by flow cytometry.
Additional Materials (also see Basic Protocols 1 and 2)
-
T-MP suspension (Basic Protocol 1)
-
PKH67 Green Fluorescent Cell Linker Kit (e.g., Sigma-Aldrich, cat. no. MINI67-1KT)
-
Mice to be used for collection of dendritic cells and macrophages
-
Mouse recombinant GM-CSF (e.g., Peprotech, cat. no. 315-03)
-
IL-4 (e.g., Peprotech, cat. no. 214-14)
-
Mouse recombinant M-CSF (e.g., Peprotech, cat. no. 315-02)
-
LPS (Sigma, cat. no. L2630)
-
IFN-γ (e.g., Peprotech, cat. no. 315-05)
-
APC-conjugated anti-CD80 (Biolegend, cat. no. 104713)
-
FITC-conjugated anti-CD86 (Biolegend, cat. no. 105005)
-
FITC-conjugated anti-CD206 (Biolegend, cat. no. 141703)
-
PE-conjugated anti-CD301 (Biolegend, cat. no. 145703)
-
FITC-conjugated anti-IL-10 (Biolegend, cat. no. 505005)
-
PE-conjugated anti-TNF-α (Biolegend, cat. no. 506305)
-
APC-conjugated anti-IL-12 (Biolegend, cat. no. 505205)
-
Fixation buffer (Invitrogen, cat. no. 2290902)
-
Permeabilization buffer (Invitrogen, cat. no. 2178649)
-
FITC Annexin V Apoptosis Detection Kit (Biolegend, cat. no. 640922)
-
Tumor-bearing BALB/c mice
-
6-well culture plates (e.g., Corning®, cat. no. 2628640)
-
Cell strainer
-
24-well culture plates
-
Additional reagents and equipment for isolation of dendritic cells (see Current Protocols article: Inaba et al., 2009) and macrophages (see Current Protocols article: Zhang, Goncalves, & Mosser, 2008)
NOTE : All protocol steps are performed under sterile conditions.
Preparation of bone marrow-derived dendritic cells (BMDCs) and macrophages (BMDMs)
1.Also see Current Protocols articles Zhang et al. (2008) and Inaba et al. (2009).
2.Flush mouse tibiae and femurs with ice-cold PBS plus 1% FBS through a cell strainer. Collect bone marrow cells.
3.Centrifuge 6 min at 300 × g , and carefully aspirate the supernatant. Wash cells two more times with PBS plus 1% FBS using the same centrifugation procedure.
4.Resuspend bone marrow cells and culture the cells in 6-well plates with RPMI 1640 supplemented with 10% FBS, 100 U/ml penicillin, 100 mg/ml streptomycin, 20 ng/ml GM-CSF, and 20 ng/ml IL-4.
5.Incubate the cells at 37°C with 5% CO2, and change half of medium described in step 13 on days 4 and 6.
6.On day 7, the percentage of CD11c+ cells should be higher than 90% as measured by flow cytometry. Immature BMDCs are then ready for further experiments.
7.For BMDMs, culture bone marrow cells (step 2) for 5 days in the complete RPMI-1640 medium containing 10% FBS, 100 U/ml penicillin, 100 mg/ml streptomycin, and 20 ng/ml recombinant mouse M-CSF.
8.Incubate the cells at 37°C with 5% CO2, change medium described in step 16 on days 3 and 5.
9.On day 5, add 100 ng/ml LPS plus 20 ng/ml IFN-γ to generate inflammatory (M1) or 10 ng/ml IL-4 for 24 hr to generate anti-inflammatory macrophages (M2).
PKH67 labeling of T-MPs
10.Prepare T-MPs by multi-step centrifugations as described in Basic Protocol 1.Carefully aspirate and discard the supernatant, being careful not to remove any T-MPs.
11.Use Diluent C from the PKH67 kit to increase the volume of each sample to 500 μl, and resuspend gently to ensure complete dispersion.
12.Add 2 μl of the PKH67 dye to 500 μl Diluent C to prepare a 2× dye solution. Mix gently to disperse.
13.Rapidly add 500 μl T-MP suspension (step 2) to 500 μl of 2× dye solution (step 3), and mix gently for 30 s. Avoid formation of bubbles.
14.Incubate the MP/dye suspension at room temperature for less 3-5 min with periodic mixing.
15.Quench by adding an equal volume of FBS and incubate for 1 min.
16.Centrifuge the T-MPs 1 hr at 14,000 × g , 4°C, and remove the supernatant carefully to ensure that T-MPs are not removed.
17.Wash T-MPs two more times, each time with 1 ml PBS.
18.Resuspend T-MPs in 300 μl PBS to quantitatively detect fluorescence intensity.
Analysis of T-MP uptake by different target cells in vitro
19.Add PKH67 labeled-MPs to the culture medium of BMDCs, BMDMs, or tumor cells (MP/cell ratio=1:1, 2:1, 5:1, 10:1, etc.) for different times (e.g., 4 hr, 8 hr, 12 hr, and 24 hr).
20.Collect cells from the wells and transfer cell suspension to fresh 1.5-ml microcentrifuge tubes.
21.Centrifuge 10 min at 300 × g , 4°C, carefully aspirate the supernatant, then wash cells two more times with 1 ml PBS using the same centrifugation conditions.
22.Resuspend cell pellet in 300 μl PBS and analyze the time-dependent and dose-dependent uptake of T-MPs by flow cytometry.
23.Analyze flow cytometry data using FlowJo software.
T-MP-loaded BMDCs act as vaccines
24.Incubate T-MPs with immature BMDCs for different times (e.g., 4 hr, 8 hr, 12 hr, and 24 hr).
25.Collect cells from the wells and transfer to fresh tubes.
26.Centrifuge 10 min at 300 × g , 4°C, carefully aspirate the supernatant, then wash cells two more times with 1 ml PBS using the same centrifugation conditions.
27.Resuspend cell pellet in 100 μl PBS and stain with antibodies (anti-CD80 and anti-CD86). Prepare unstained and single-stained controls for flow cytometry. Incubate at 4°C for 30 min.
28.Wash cells twice, each time with 1 ml PBS by centrifuging 10 min at 300 × g , 4°C.
29.Resuspend cell pellet in 300 μl PBS and analyze by flow cytometry.
29a. For tumor model : Pre-challenge BALB/c mice with tumor cells (3 × 105 cells/mouse) in the lateral right flank. Five days after tumor inoculation, subcutaneously inject mice with T-MPs or T-MP-loaded BMDCs three times at 5-day intervals. Measure palpable tumors by calipers, and calculate tumor volume.
29b. For the metastasis model : Intravenously inject C57BL/6 mice with 1 × 105 B16 melanoma cells. Five days later, intravenously inject C57BL/6 mice with T-MPs or T-MP-loaded BMDCs three times at 5-day intervals. 21 days after tumor incubation, sacrifice mice and measure lung metastasis nodules.
T-MPs reset macrophages toward M2-like phenotype
30.Incubate T-MPs with immature BMDCs for different times (e.g., 4 hr, 8 hr, 12 hr, and 24 hr).
31.Collect cells from the wells and transfer cell suspensions to fresh tubes.
32.Centrifuge 10 min at 300 × g , 4°C, carefully aspirate the supernatant, then wash cells two more times with 1 ml PBS using the same centrifugation conditions.
33.Resuspend cell pellet in 100 μl PBS and stain with antibodies.
34a. For cell surface staining : Stain BMDMs with FITC-conjugated anti-CD206 or PE-conjugated anti-CD301 antibody at 4°C.
34b. For intracellular staining : Fix live cells with fixation buffer and treat cells with permeabilization buffer. Stain with FITC-conjugated anti-IL-10, PE-conjugated anti-TNF-α, or APC-conjugated anti-IL-12 antibody.
35.Acquire data on Accuri C6 flow cytometer and analyze by FlowJo software.
Annexin V-based apoptosis assays with tumor cells
36.Seed 5 × 104 tumor cells per well of a 24-well plate in RPMI 1640 medium (plus 10% FBS and 1% (v/v) penicillin/streptomycin).
37.Isolate drug-MPs from cell culture supernatants according to Basic Protocol 1.
38.Add different concentrations of drug-MPs to the cell culture plate and create the appropriate controls.
39.Incubate at 37°C with 5% CO2 for 24 to 48 hr.
40.Collect cells from the wells of the plate and transfer cell suspensions to new tubes.
41.Centrifuge 10 min at 300 × g , 4°C, carefully aspirate the supernatant, then wash cells two more times with 1 ml PBS using the same centrifugation conditions.
42.Resuspend cell pellet in 100 μl PBS and stain with annexin V.
43.Measure apoptosis at different points by flow cytometry using Accuri C6.Analyze data using FlowJo software.
Detecting the distribution and fate of MPs in tumor-bearing mice
44.Intravenously inject PKH67-stained MPs into tumor-bearing mice.
45.Evaluate the distribution of MPs in spleen, bone marrow, lymph node, lung, kidney, liver and tumor by flow cytometry at different time points (0 hr, 4 hr, 12 hr, 24 hr, 48 hr, and 72 hr). Use CD45 pan-immune cell marker to distinguish immune cells and tumor cells.
46.Collect urine and feces and detect PKH67-positive MPs by flow cytometry.
47.To detect the final drug concentration in organs, intravenously inject 600 μg MTX or 2 × 106 MTX-MPs into tumor-bearing mice, homogenize the tissues for HPLC analysis of MTX concentration at different time points (0.5 hr, 1 hr, 2 hr, 4 hr, 12 hr, 24 hr, and 48 hr) by HPLC (see Basic Protocol 2).
REAGENTS AND SOLUTIONS
Phosphate-buffered saline (PBS), pH 7.2-7.5
- Prepare in 1 L of Heal ForceR-purified or distilled, deionized H2O:
- 3.58 g Na2HPO4 ⋅ 2H2O
- 0.24 g KH2PO4
- 0.2 g KCl
- 8.0 g NaCl
- Adjust pH to 7.2-7.5
- Sterilize by autoclaving
- Store up to several months at 4°C or room temperature
COMMENTARY
Background Information
In tumor microenvironments, tumor cells can release MPs into extracellular space or the bloodstream. Elevated levels of MPs have been detected in the spectrum of body fluids, which makes them useful prognostic and predictive biomarkers and possible therapeutic means (Kooijmans, Schiffelers, Zarovni, & Vago, 2016; Sedgwick & D'Souza-Schorey, 2018). T-MPs can transfer small molecules among cells and are important modulators of the immune system. Hypoxic tumor cell−derived MPs reprogram macrophages into M2 phenotype and contribute to tumor metastasis by initiating premetastatic niche formation in distant organs (Zhang et al., 2018). However, irradiated cell−derived MPs can activate dendric cells as tumor vaccines, as they carry both tumor antigens and innate signaling molecules (Zhang et al., 2015). Furthermore, T-MPs can function as natural delivery vehicles to deliver drugs or oncolytic viruses to tumor sites for better killing of tumor cells, with potential clinical applications (Agrahari, Agrahari, Burnouf, Chew, & Burnouf, 2019; Ran et al., 2016; Tang et al., 2012). Indeed, drug-loaded MPs profoundly improve the clinical outcomes in malignant pleural effusion (MPE) and obstructive cholangiocarcinoma (CCA), accompanied by immunoregulation functions such as polarizing tumor-associated macrophages into M1 phenotype and promoting release of NETs by tumor-infiltrating neutrophils (Gao et al., 2020; Guo et al., 2019; Xu et al., 2020). In addition, soft MPs extravasate more easily from blood vessels and penetrate into the tumor parenchyma, where they efficiently deliver chemotherapeutic drugs (Liang et al., 2019).
The emerging roles of T-MPs as potential cancer biomarkers and therapeutic delivery platforms have led to a rapid development of suitable methods for their isolation, identification, and characterization. T-MPs are usually generated when tumor cells undergo apoptosis, induced by hypoxia, nutrient deficiency, chemotherapy, or radiotherapy. Thus, T-MPs are always present in a mixture with other EVs such as apoptotic bodies and exosomes, which share similar features with MPs (Valenti et al., 2007). These EVs produced in different ways are characterized by different sizes, densities, contents, and functions (Ratajczak & Ratajczak, 2020). Exosomes are the most popular subset of EVs (30 to 150 nm in diameter) formed from multivesicular bodies (MVBs) of parent cells (see Current Protocols article: Ludwig et al., 2019). MPs (100 to 1000 nm in diameter) are formed in early apoptosis and bleb from the cellular membrane of parent cells; apoptotic bodies (1-5 μm) are formed in the final phase of apoptotic death and contain the post-apoptotic remnants, including condensed chromatin and cytoplasm, of parent cells (Caruso & Poon, 2018). The International Society for Extracellular Vesicles (ISEV) performed a worldwide survey and revealed the appropriate methods for isolation of EVs from cells or biological fluids. Differential centrifugation methods are the oldest method and have been widely used for T-MP isolation (Thery et al., 2018). Flow cytometry−based approaches can be used for the separation of annexin V−positive MPs derived from apoptotic cells (van der Heyde, Gramaglia, Combes, George, & Grau, 2011). Due to the absence of strict MP-specific markers, multiple tools and methods for characterization and quantification of T-MPs have been developed. Electron microscopy (EM), western blotting (WB), and nanoparticle tracer analysis (NTA) are routine methods that are used to characterize MPs, including morphology, particle size, purity, and biomarker proteins. The isolation protocol—combined with analysis methods—provides an efficient, easily operated method for high yield and maximal purity of T-MPs.
Critical Parameters and Troubleshooting
T-MPs are an ideal carrier to deliver chemotherapeutic drugs to tumor cells, leading to effective killing of tumor cells (Tang et al., 2012). Thus, in order to maintain the stability and reproducibility of drug encapsulation, the time course and intensity of apoptosis induction is crucial for these processes (Kim et al., 2019; van Niel, D'angelo, & Raposo, 2018). Ultraviolet radiation (UV) is a convenient method for cell apoptosis which mediates formation of covalently linked dimers between the adjacent pyrimidines in the same DNA strand (Schuch, Moreno, Schuch, Menck, & Garcia, 2017). UV lamps in biosafety cabinets are normally UVC type with emission wavelength of 253.7 nm; one-half hour of irradiation ensures thorough death of microorganisms (Kim & Kang, 2018). For induction of tumor cell−derived MPs, one-half to 1 hr of UV irradiation is needed, which can overcome the anti-apoptosis capacity of tumor cells (Chen et al., 2020; Tang et al., 2012). Another issue for UV irradiation is the strength. Due to decay, the UV source should be tested at least once a month. Considering the low-power penetration of UV, a small volume of tumor cell culture medium is suitable for apoptosis induction. Normally, a 0.5-cm depth of medium should not be exceeded.
Tumor cell−derived microparticles have been applied for drug delivery; however, the concentration of encapsulated chemo-drugs is a critical parameter. In the process of drug packaging with T-MPs, a large amount of drugs is added to the tumor cell culture medium, which is much higher than the final drug loading. Thus, in the course of T-MP purification, residual drugs in culture supernatants should be removed. After T-MPs are pelleted, PBS or normal saline (at least 10 ml volume) is needed for washing; two washes are reasonable, which leave little drug remaining and avoids significant T-MP loss.
Finally, cell culture state is also a critical parameter for the stability of drug-packaging T-MPs. After 20 passages of tumor cell proliferation, fresh cells are needed. Until tumor cell proliferation rate reaches a stable state (2-5 passages), they cannot be used for T-MP preparation.
Understanding Results
The data presented in Figure 2 represent the expected results of the performed experiments, including: (1) the production of vesicles is closely related to the number of parent cells and the irradiation conditions; (2) in the first step of centrifugation, the volume of the solution should be expanded as much as possible to prevent the cells from adhering and pulling down the vesicles; (3) a large volume of supernatant enables a high MP yield; (4) the MP pellets are usually visible; and (5) MPs can be detected by NTA or FCM.
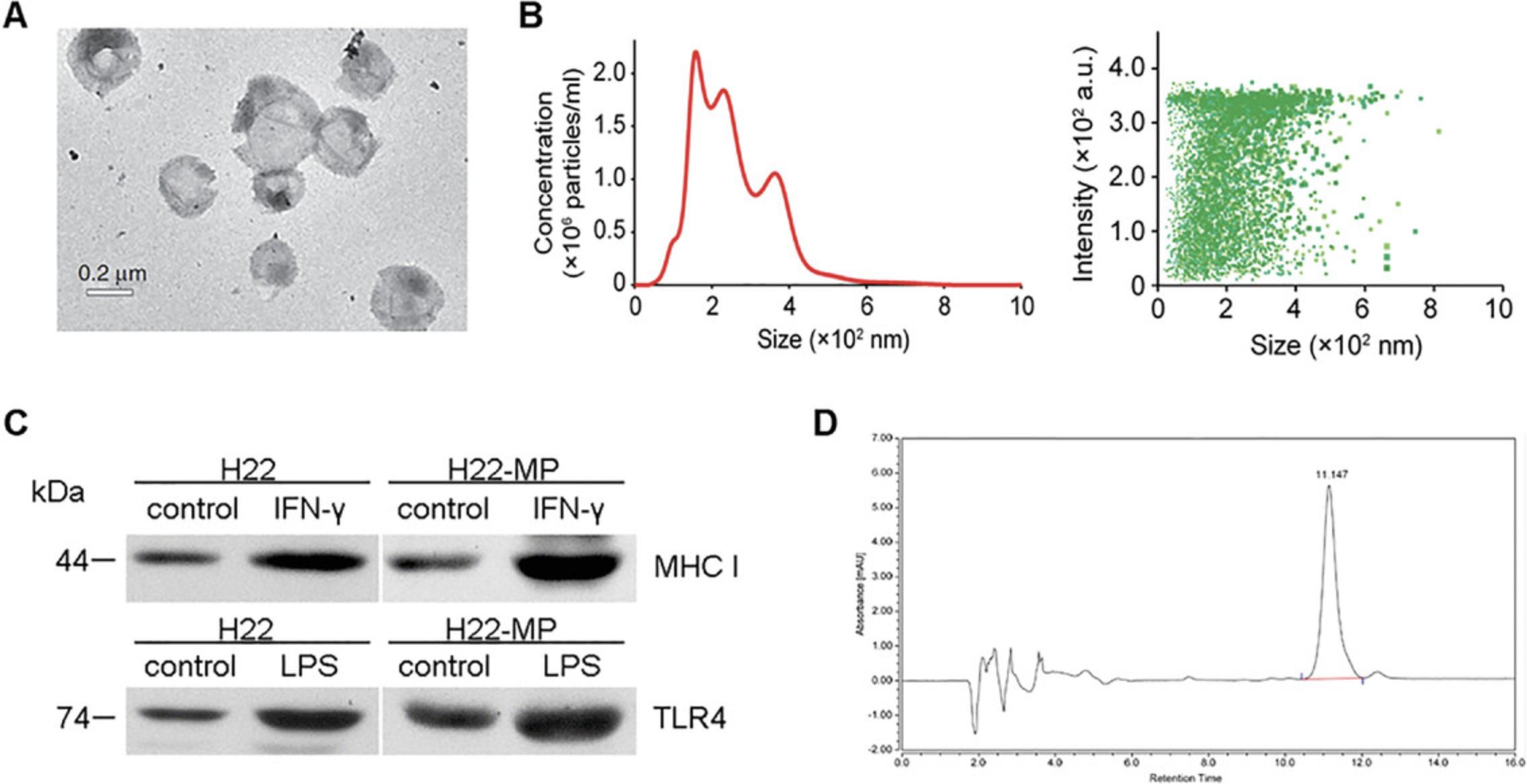
Time Considerations
Basic Protocol Basic Protocol 1: The entire protocol for MP isolation takes about 2-3 days. The tumor cells are seeded on day 1. On day 2, the cells are treated with 1 to 1.5 hr UV irradiation, followed by 16-20 hr culture (overnight). The MP-containing supernatants are harvested, and the multi-step centrifugations takes around 3 to 4 hr.
Basic Protocol Basic Protocol 2: Preparation of samples for EM requires around 2 days. A standard time required for western blotting is around 1.5 days. NTA analysis requires around 30 min for one sample. For flow cytometry, the entire protocol for capture and staining of T-MPs takes 1 day.
Basic Protocol Basic Protocol 3: PKH67 labeling of T-MPs requires around 1 day. Additional time is required to isolate and culture bone marrow (around 5-8 days), as well as around 3 hr for collection of cell cultures, antibody staining, and analysis by flow cytometry. The distribution and fate of T-MPs in the tumor-bearing mice takes 3-4 days.
Acknowledgments
This work was supported by the National Natural Science Foundation of China (grant numbers 32090053, 81788101, 81701544), the CAMS Initiative for Innovative Medicine (2016-I2M-1-007), and China Postdoctoral Science Foundation funded project (2018T110773).
Author Contributions
Jingwei Ma : investigation, methodology, writing original draft, writing review and editing; Ke Tang : writing original draft; Huafeng Zhang : writing original draft; Keke Wei : methodology; Bo Huang : writing original draft, writing review and editing.
Conflict of Interest
The authors declare no conflict of interest.
Open Research
Data Availability Statement
Data sharing not applicable—no new data generated.
Literature Cited
- Agrahari, V., Agrahari, V., Burnouf, P. A., Chew, C. H., & Burnouf, T. (2019). Extracellular microvesicles as new industrial therapeutic frontiers. Trends Biotechnology , 37(7), 707–729.
- Burghardt, R. C., & Droleskey, R. (2006). Transmission electron microscopy. Current Protocols in Microbiology , 3, 2B.1−.1−2B.1.39. doi: 10.1002/9780471729259.mc02b01s03
- Caruso, S., & Poon, I. (2018). Apoptotic cell-derived extracellular vesicles: More than just debris. Frontiers in Immunology , 9, 91486.
- Chen, J., Sun, W., Zhang, H., Ma, J., Xu, P., … Huang, B. (2020). Macrophages reprogrammed by lung cancer microparticles promote tumor development via release of IL-1beta. Cellular and Molecular Immunology , 17(12), 1233–1244.
- Cocucci, E., Racchetti, G., & Meldolesi, J. (2009). Shedding microvesicles: Artefacts no more. Trends Cell Biology , 19(2), 43–51.
- Gao, Y., Zhang, H., Zhou, N., Xu, P., Wang, J., … Huang, B. (2020). Methotrexate-loaded tumour-cell-derived microvesicles can relieve biliary obstruction in patients with extrahepatic cholangiocarcinoma. Nature Biomedical Engineering , 4(7), 743–753.
- Geddings, J. E., & Mackman, N. (2013). Tumor-derived tissue factor-positive microparticles and venous thrombosis in cancer patients. Blood , 122(11), 1873–1880.
- Gong, J., Jaiswal, R., Dalla, P., Luk, F., & Bebawy, M. (2015). Microparticles in cancer: A review of recent developments and the potential for clinical application. Seminars in Cell and Developmental Biology , 40, 35–40.
- Guo, M., Wu, F., Hu, G., Chen, L., Xu, J., … Jin, Y. (2019). Autologous tumor cell-derived microparticle-based targeted chemotherapy in lung cancer patients with malignant pleural effusion. Science Translational Medicine , 11(474), eaat5690.
- Inaba, K., Swiggard, W. J., Steinman, R. M., Romani, N., Schuler, G., & Brinster, C. (2009). Isolation of dendritic cells. Current Protocols in Immunology , 86, 3.7.1–3.7.19. doi: 10.1002/0471142735.im0307s86
- Kim, D. K., & Kang, D. H. (2018). UVC LED Irradiation effectively inactivates aerosolized viruses, bacteria, and fungi in a chamber-type air disinfection system. Applied and Environmental Microbiology , 84 (17), e00944-18.
- Kim, Y. B., Ahn, Y. H., Jung, J. H., Lee, Y. J., Lee, J. H., & Kang, J. L. (2019). Programming of macrophages by UV-irradiated apoptotic cancer cells inhibits cancer progression and lung metastasis. Cellular and Molecular Immunology , 16(11), 851–867.
- Kooijmans, S., Schiffelers, R. M., Zarovni, N., & Vago, R. (2016). Modulation of tissue tropism and biological activity of exosomes and other extracellular vesicles: New nanotools for cancer treatment. Pharmacological Research , 111, 487–500.
- Li, D., Jia, H., Zhang, H., Lv, M., Liu, J., … Huang, B. (2012). TLR4 signaling induces the release of microparticles by tumor cells that regulate inflammatory cytokine IL-6 of macrophages via microRNA let-7b. Oncoimmunology , 1(5), 687–693.
- Liang, Q., Bie, N., Yong, T., Tang, K., Shi, X., … Yang, X. (2019). The softness of tumour-cell-derived microparticles regulates their drug-delivery efficiency. Nature Biomedical Engineering , 3(9), 729–740.
- Ludwig, N., Hong, C. S., Ludwig, S., Azambuja, J. H., Sharma, P., Theodoraki, M. N., & Whiteside, T. L. (2019). Isolation and analysis of tumor-derived exosomes, Current Protocols in Immunology , 127(1), e91.
- Ma, J., Zhang, H., Tang, K., & Huang, B. (2020). Tumor-derived microparticles in tumor immunology and immunotherapy. European Journal of Immunology , 50(11), 1653–1662.
- Ma, J., Zhang, Y., Tang, K., Zhang, H., Yin, X., … Huang, B. (2016). Reversing drug resistance of soft tumor−repopulating cells by tumor cell-derived chemotherapeutic microparticles. Cell Research , 26(6), 713–727.
- Mause, S. F., & Weber, C. (2010). Microparticles: Protagonists of a novel communication network for intercellular information exchange. Circulation Research , 107(9), 1047–1057.
- Ni, D., Xu, P., and Gallagher, S. (2016). Immunoblotting and immunodetection. Current Protocols in Immunology , 114, 8.10.1–8.10.36. doi: 10.1002/cpim.10
- Ran, L., Tan, X., Li, Y., Zhang, H., Ma, R., … Huang, B. (2016). Delivery of oncolytic adenovirus into the nucleus of tumorigenic cells by tumor microparticles for virotherapy. Biomaterials , 89, 56–66.
- Ratajczak, M. Z., & Ratajczak, J. (2020). Extracellular microvesicles/exosomes: Discovery, disbelief, acceptance, and the future? Leukemia , 34(12), 3126–3135.
- Schuch, A. P., Moreno, N. C., Schuch, N. J., Menck, C., & Garcia, C. (2017). Sunlight damage to cellular DNA: Focus on oxidatively generated lesions. Free Radical Biology and Medicine , 107, 110–124.
- Sedgwick, A. E., & D'souza-schorey, C. (2018). The biology of extracellular microvesicles. Traffic , 19(5), 319–327.
- Tang, K., Zhang, Y., Zhang, H., Xu, P., Liu, J., … Huang, B. (2012). Delivery of chemotherapeutic drugs in tumour cell-derived microparticles. Nature Communication , 3, 1282.
- Thery, C., Ostrowski, M., & Segura, E. (2009). Membrane vesicles as conveyors of immune responses. Nature Review Immunology , 9(8), 581–593.
- Thery, C., Witwer, K. W., Aikawa, E., Alcaraz, M. J., Andriantsitohaina, R., Antoniou, A, Arab, T, … Zuba-Surma, E. K. (2018). Minimal information for studies of extracellular vesicles 2018 (MISEV2018): A position statement of the International Society for Extracellular Vesicles and update of the MISEV2014 guidelines. Journal of Extracellular Vesicles , 7(1), 1535750.
- Valenti, R., Huber, V., Iero, M., Filipazzi, P., Parmiani, G., & Rivoltini, L. (2007). Tumor-released microvesicles as vehicles of immunosuppression. Cancer Research , 67(7), 2912–2915.
- van der Heyde, H. C., Gramaglia, I., Combes, V., George, T. C., & Grau, G. E. (2011). Flow cytometric analysis of microparticles. Methods in Molecular Biology , 699, 337–54.
- van Niel, G., D'angelo, G., & Raposo, G. (2018). Shedding light on the cell biology of extracellular vesicles. Nature Reviews Molecular Cell Biology , 19(4), 213–228.
- Wolf, P. (1967). The nature and significance of platelet products in human plasma. British Journal of Haematology , 13(3), 269–288.
- Xu, P., Tang, K., Ma, J., Zhang, H., Wang, D., … Huang, B. (2020). Chemotherapeutic tumor microparticles elicit a neutrophil response targeting malignant pleural effusions. Cancer Immunology Research , 8(9), 1193–1205.
- Zhang, H., Tang, K., Zhang, Y., Ma, R., Ma, J., … Huang, B. (2015). Cell-free tumor microparticle vaccines stimulate dendritic cells via cGAS/STING signaling. Cancer Immunology Research , 3(2), 196–205.
- Zhang, H., Yu, Y., Zhou, L., Ma, J., Tang, K., … Huang, B. (2018). Circulating tumor microparticles promote lung metastasis by reprogramming inflammatory and mechanical niches via a macrophage-dependent pathway. Cancer Immunology Research , 6(9), 1046–1056.
- Zhang, X., Goncalves, R., & Mosser, D. M. (2008). The isolation and characterization of murine macrophages. Current Protocols in Immunology , 83, 14.1.1–14.1.14. doi: 10.1002/0471142735.im1401s83
- Zhou, X., Xie, F., Wang, L., Zhang, L., Zhang, S., Fang, M., & Zhou, F. (2020). The function and clinical application of extracellular vesicles in innate immune regulation. Cellular and Molecular Immunology , 17(4), 323–334.
Citing Literature
Number of times cited according to CrossRef: 4
- Shuya Lu, Qingfa Cui, Huan Zheng, Yuan Ma, Yanchun Kang, Ke Tang, Challenges and Opportunities for Extracellular Vesicles in Clinical Oncology Therapy, Bioengineering, 10.3390/bioengineering10030325, 10 , 3, (325), (2023).
- Weiwei Sun, Lili Dai, Yuqing Cao, Pengtao Pan, Lijuan Zhi, Xinke Wang, Xinzhong Yuan, Zi Gao, Sheng Guo, Guoyan Liu, Junlei Yin, Liangliang Xie, Liping Wang, Yanling Wang, Wensheng Li, Hong Li, Yunjie Jia, Monocytes reprogrammed by tumor microparticle vaccine inhibit tumorigenesis and tumor development, Cancer Nanotechnology, 10.1186/s12645-023-00190-x, 14 , 1, (2023).
- Keke Wei, Huafeng Zhang, Shuaishuai Yang, Yuxiao Cui, Bingxia Zhang, Jincheng Liu, Liang Tang, Yaoyao Tan, Simin Liu, Shiqi Chen, Wu Yuan, Xiao Luo, Chen Chen, Fei Li, Junwei Liu, Jie Chen, Pingwei Xu, Jiadi Lv, Ke Tang, Yi Zhang, Jingwei Ma, Bo Huang, Chemo-drugs in cell microparticles reset antitumor activity of macrophages by activating lysosomal P450 and nuclear hnRNPA2B1, Signal Transduction and Targeted Therapy, 10.1038/s41392-022-01212-7, 8 , 1, (2023).
- Shuxin Lv, Jinghua Sun, Chunyan Guo, Yufei Qin, Ruiping Zhang, PAI/MRI Visualization of Tumor Derived Cellular Microvesicles with Endogenous Biopolymer Nanoparticles Modification, International Journal of Nanomedicine, 10.2147/IJN.S367721, Volume 17 , (2883-2890), (2022).