Assessing Lupus-Like Disease in Murine Model Systems
Hui Yin Lee, Hui Yin Lee, Teja Celhar, Teja Celhar, Anna-Marie Fairhurst, Anna-Marie Fairhurst
Abstract
Systemic Lupus Erythematosus (SLE) is a complex and heterogenous autoimmune disease, where genetics, immunology, and environmental factors all play a role. Murine models have contributed critical information on mechanisms of disease and prospective therapeutics. The key features that have been used to study the disease include the development of anti-nuclear autoantibodies (ANAs), splenomegaly, and kidney disease. The loss of tolerance and subsequent autoimmune features, and the progression to severe disease, are all dependent on immune dysregulation. In this article, we will describe the methods used to evaluate the underlying immunological features of the disease, as a more sensitive strategy to understand the disease itself and the mechanisms of potential novel therapeutics. © 2021 The Authors. Current Protocols published by Wiley Periodicals LLC.
Basic Protocol 1 : End study protocols for tissue harvesting
Basic Protocol 2 : End study protocols for tissue processing
Basic Protocol 3 : Immunophenotyping using flow cytometry protocols
Support Protocol : Tissue processing for cold storage
Basic Protocol 4 : Additional tissue processing for later analyses
Basic Protocol 5 : Analysis of serum auto-antibodies by ELISAs (ANAs, snRNP, and dsDNA)
INTRODUCTION
Systemic Lupus Erythematosus (SLE) is an autoimmune disease with a complex etiology and accompanying heterogeneous clinical presentation (Aringer et al., 2019; Fairhurst, Wandstrat, & Wakeland, 2006). It is polygenic, with environmental and immunological factors additionally playing a role. The most recent classification for SLE supported by the American College of Rheumatology (ACR) and European League Against Rheumatism (EULAR) includes a single occurrence of anti-nuclear antibodies (ANAs), together with additional weighted clinical and immunologic criteria (Aringer et al., 2019). Immune dysregulation and the loss of self-tolerance are critical events in the development and progression of autoimmune disease. ANA production by B cells is assisted by autoreactive T cells, together with dendritic cells (DCs). These ANAs form immune complexes (ICs) with self-ligands, such as RNA and DNA, that can then activate the immune system. The continuing activation of the immune system and B cells results in a positive feedback loop for antibody production and an ongoing chronic inflammatory response (Zharkova et al., 2017). The consequent tissue destruction of organs including the lungs, heart, and kidney contribute to primary comorbidities of SLE that include pneumonia, cardiac disease, and glomerulonephritis (GN) (Kuo et al., 2018; Maroz & Segal, 2013). Treatment options are limited, with mainstream therapy focused on suppressing the immune system in a broad manner, such as through use of steroids. This results in severe and challenging side effects and an increased susceptibility to infections and mortality (Durcan & Petri, 2016). The ramifications of heterogeneity and medications present major challenges in ascertaining both the mechanisms involved in disease initiation and progression and the potential for novel therapeutic interventions. Murine models continue to provide crucial information in these areas (Celhar & Fairhurst, 2017; Theofilopoulos & Dixon, 1985). Therefore, it is essential that researchers have a competent understanding of the model systems available, which include any known mechanisms, the time frame for immunological and pathological changes, and the final phenotypes associated with the lupus-like disease.
In these protocols, we describe methods to assess immune activation and disease in three murine strains, B6, Sle1, and Sle123 (overview in Fig. 1). In Basic Protocol 1, the procedures for the collection of (i) urine, (ii) peripheral blood, (iii) spleen, and (iv) kidneys are described. Basic Protocol 2 details the methods for tissue processing, and Basic Protocol 3 details immunophenotyping strategies. In addition, the harvesting of tissues for cold storage and later analyses, including serum ANAs, are also described in Basic Protocols 4 and 5.
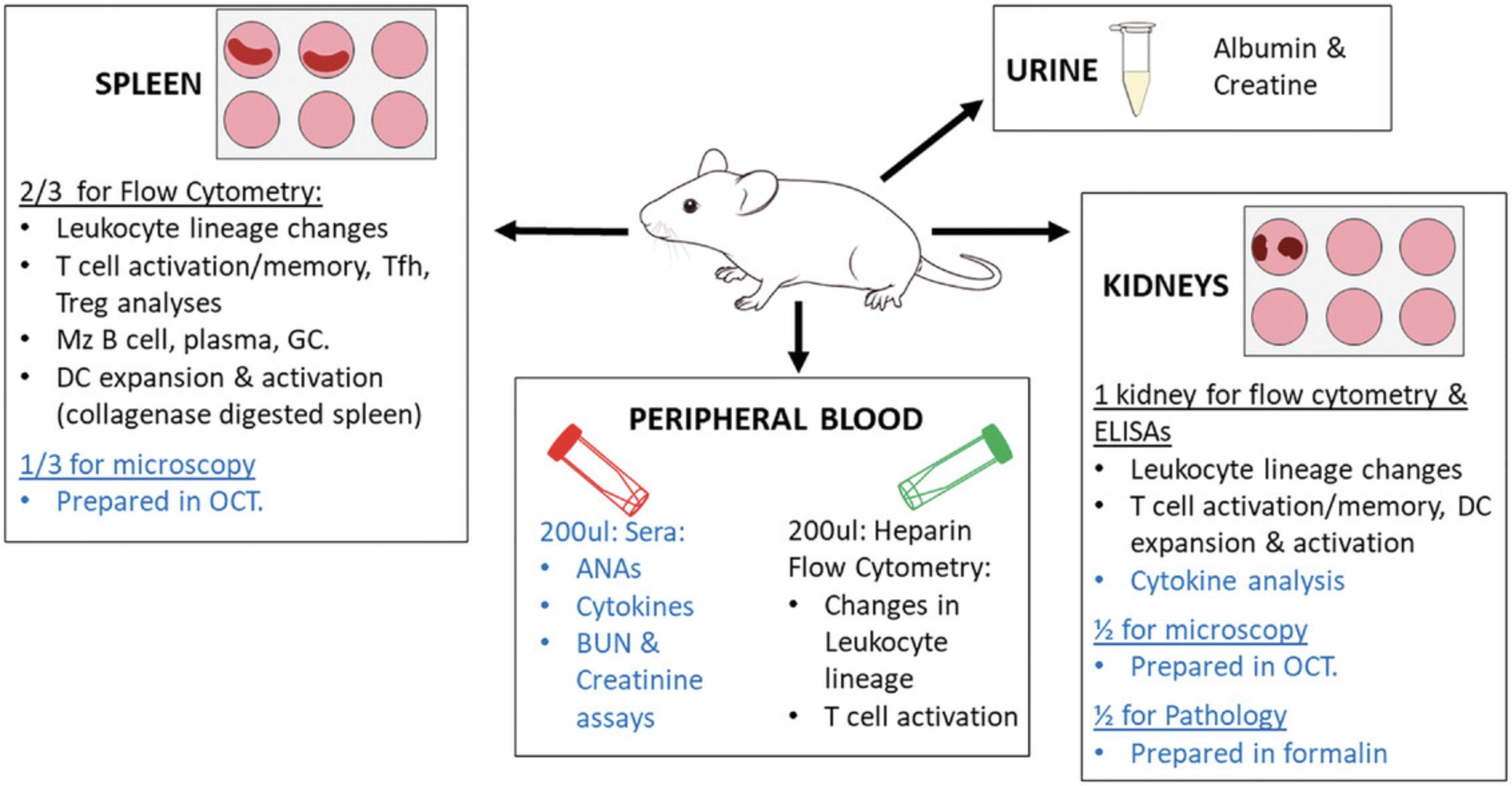
STRATEGIC PLANNING
Prior to commencing any experiments detailed here, researchers should complete the necessary theoretical and practical training for experimental use of animals. In addition, the methods described here rely on understanding and competence in flow cytometry techniques. The user should undertake training to complete the immunological assessments, or collaborate with an individual with expertise.
Spontaneous disease in lupus mice develops over weeks. Therefore, it is important to understand whether the aim of the study is to prevent the onset of disease or suppress an ongoing manifestation of inflammation and halt or reverse pathology, before embarking on variable time point analyses with these models. When planning end-study protocols, at least two cohorts should be set up, separated by weeks to mitigate influences of microbiome clustering from breeders and cage microenvironment changes over time (McCafferty et al., 2013; Robertson et al., 2019; Ubeda et al., 2012). Furthermore, the variability of the disease and incomplete penetrance due to stochastic events require at least two cages per group, and between 6 and 15 replicates per group, depending on the phenotype being assessed, to provide robust reproducible data. Wherever possible, multiple strains should be used to evaluate novel therapeutic targets, since no one mouse model recapitulates the full spectrum of clinical disease.
All experimental procedures must be approved by an Institutional Animal Care and Use Committee and follow the NIH guidelines. Mice detailed in these protocols were kept on the normal 12:12 hr light-dark cycle of the facility, on corn cob bedding, with Teklad Global 18% protein rodent diet #2918 irradiated feed, with reverse osmosis and chlorinated water available ad libitum.
Purchase and Maintenance of Mice
Acclimate purchased mice for at least 1 week in the animal facility prior to commencing experiments. We recommend procuring mice at 6-10 weeks old, depending on the timepoint for end-study evaluation. Harem breeding for these strains is also advised, to increase the overall well-being and survival of the female parent in the first few weeks post-birth.
Care needs to be taken with monitoring the health status throughout the study. Inspect the mice once a week until 20 weeks old, then twice a week. Euthanize if signs of illness occur, such as ataxia, dehydration, hunched or stretched posture, soiled and ruffled hair coat, and body weight loss.
Basic Protocol 1: END STUDY PROTOCOLS FOR TISSUE HARVESTING
At the cessation of the study, cohorts of mice enter into the end protocols depicted in Figure 1.Preparation should occur at least 1 week in advance, including all tube labeling.
Materials
-
70% ethanol
-
8- to 9-month-old female B6 (C57BL/6J) mice (Jackson Laboratory, Stock No. 000664)
-
8- to 9-month-old female B6.Sle1 (Sle1) mice (Jackson Laboratory, Stock No. 021569, B6.Cg-Sle1NZM2410/AegYaa/DcrJ)
-
8- to 9-month-old female B6.Sle1Sle2Sle3 mice (Sle123) (Jackson Laboratory, Stock No. 002676, B6;NZM-Sle1NZM2410/AegSle2NZM2410/AegSle3NZM2410/Aeg/LmoJ)
-
Selection of these strains provides data from a model developing severe disease with glomerulonephritis (GN) (Sle123) or mild autoimmunity (Sle1); B6 is a non-autoimmune strain (B6). Sle1 lupus mice deficient in TLR9, or that have increased TLR7 expression, develop similar phenotypes as Sle123.
-
Albustix Protein Urinalysis Test Strips (Siemens Medical Solutions, cat. no. 1471625)
-
Isoflurane (Piramal, cat. no. NDC 66794-013-10) or 1.25% Avertin (see recipe)
-
RPMI 1640 medium (Gibco, cat. no. 11875)
-
Whatman® Benchkote® surface protectors (Whatman, cat. no. WHA2300916)
-
Microcentrifuge tubes, (Axygen, cat. no. MCT-175-C)
-
Animal balance
-
Ultra-Fine™ insulin syringes, 6 mm/31G (BD, cat. no. 324903)
-
Capillary tubes, 1.1-1.2 mm Ø int., 75 µl (Paul Marienfeld, cat. no. 29 010 00)
-
Microvette® CB 300 Z, Clotting Activator (Sarstedt, cat. no. 16.440.100)
-
Microvette® CB 300 LH, Lithium Heparin (Sarstedt, cat. no. 16.443.100)
-
Surgical forceps and scissors
-
12-well plate (Nunc, cat. no. 3513)
-
Additional reagents and equipment for anesthesia (see Current Protocols article: Donovan & Brown, 2001), injection (see Current Protocols article: Donovan & Brown, 2006b), and euthanasia (see Current Protocols article: Donovan & Brown, 2006a) of rodents
Urine collection and proteinuria assessment
This simple urine collection procedure described below can be completed during aging to monitor kidney disease every 1-2 weeks, recommended after 20 weeks age, and at 2-5 days before the day for euthanizing the cohort, to allow second attempts at collection from diseased mice. The collection procedure described here is adapted from Kurien, Everds, & Scofield (2004).
1.Prepare a clean surface and spray down with 70% ethanol, or use a tabletop bench protector with the polyethylene side up. Ensure the surface is dry before commencing.
2.To collect urine, apply gentle trans-abdominal pressure over the mouse's bladder to overcome normal urethral pressure.
3.Collect the urine on the tabletop bench protector using a pipette and transfer to a clean 1.7-ml microcentrifuge tube. Alternatively, the urine can be collected directly into the microcentrifuge tube. Place the urine on ice until analysis to avoid sample degradation.
4.To complete a rapid estimation of proteinuria, pipette 20 µl of urine onto an Albustix Protein Urinalysis Test Strip. Read and tabulate the result.
5.Store the remaining urine at −80°C for further assays.
Sedation of mice for procedures
If perfusion is being performed, it is recommended that sedation be completed using a reagent such as isoflurane or Avertin, depending on authorization.
6a. Sedation with isoflurane:
- Anesthetize mice with isoflurane by inhalation in a closed system in a fume hood (Donovan & Brown, 2001). 2.Monitor the mouse for respiratory rate and depth. Once non-responsive to toe pinching, proceed to retro-orbital bleeding.
6b. Sedation with Avertin:
- Weigh mice.
- Inject 0.1 ml/10 g body weight of prepared Avertin into the peritoneal cavity (see Donovan & Brown, 2006, for injection technique) using a 1-ml insulin syringe. 3.Wait for 5-10 min until the mouse is sedated, i.e., when it is non-responsive to toe pinching, before taking blood samples
Retro-orbital bleeding
The volumes indicated in this protocol are for pre-euthanasia. Recommended times pre-disease are at 6-8 weeks old, and then at 4 months to assess the onset of disease, and 5-6 months to reveal major pathological changes. It is recommended that no more than 100 µl should be taken every 4 weeks, since excessive bleeding can lower the overall B cell counts, in particular, which will affect the development of lupus traits and the overall wellbeing of the mice.
7.Check the mouse for anesthetic depth by pinching the toe.
8.Once the mouse is anesthetized, restrain the mouse gently at the back of the neck until the eye is slightly bulging.
9.Collect blood as follows:
-
Place the tip of a capillary tube at approximately a 90° angle to the nose at the medial canthus of the eye, under the nictitating membrane.
-
Apply slight pressure to break the conjunctival membranes and the ocular plexus/sinus. The blood will flow into the hematocrit tube by capillary action. Move the capillary tube so the angle to the nose is reduced to about 45° to enable faster blood flow.
-
Collect the blood in a Microvette®Z (for sera) or Microvette®LH (for plasma).
Once blood begins to flow, maintain gentle pressure to keep the eye protruded. Tilting the head slightly downward improves blood flow. At the end of study, prior to immediate euthanasia, 200 µl can be collected in each tube. The blood flow should stop when the capillary tube is withdrawn, the grip is loosened, and pressure is gently applied to the eye for a few seconds.
10.Place the blood collection tube on ice immediately after collection to prevent high background in future assays.
11.End study protocol : Euthanize the mouse (Donovan & Brown, 2006a) either with carbon dioxide inhalation or cervical dislocation, according to the approved IACUC protocol.
Collection of tissues
Glomerulonephritis (GN) develops at 5-7 months of age in male BXSB mice, and female NZB/W, Sle123 , Sle1Yaa , Sle1 Tg7, and 4-5 months in Sle1 TLR9-/- mice. It is a key feature assessed in these systems due to its prevalence in clinical disease. Overt kidney disease can be observed as a reduction in total organ size (Fig. 2A), or increased pallor. In most cases, however, in the absence of stimulation (e.g., IFNα), gross changes are not evident. It is recommended that investigators outsource all GN examinations to a clinical pathologist to obtain independent results, and to ensure relevance to clinical disease (Seavey, Lu, & Stump, 2011).
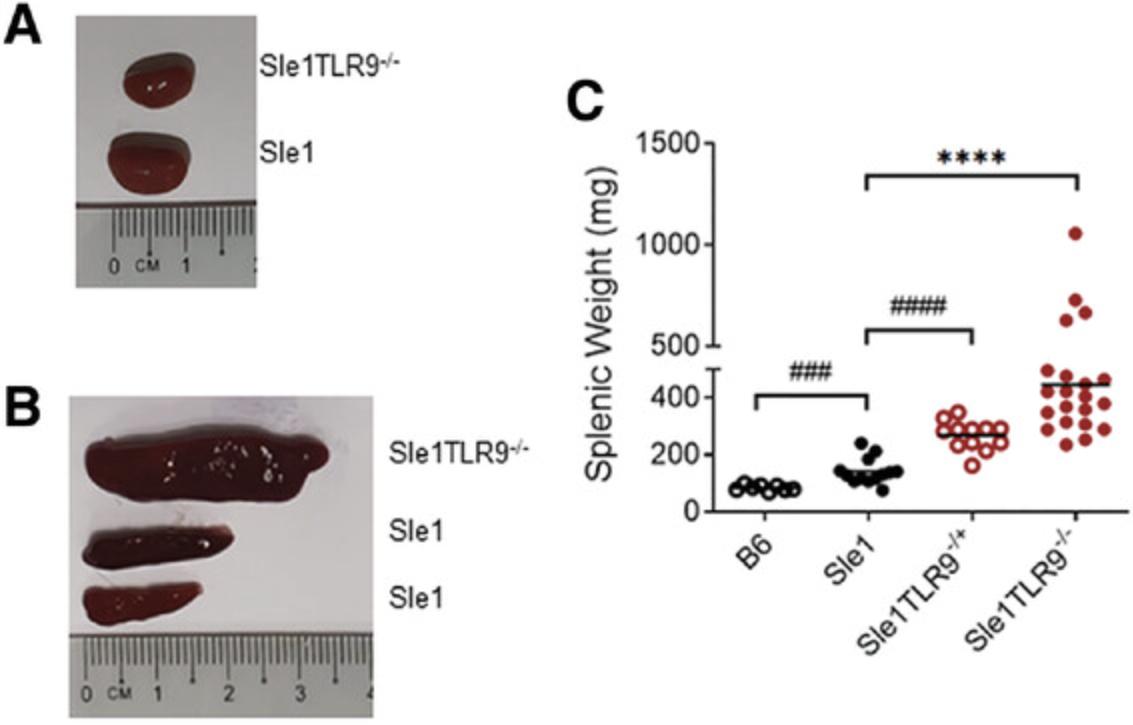
The development of splenomegaly is a characteristic trait of most murine lupus models and not observed in the majority of SLE patients. It provides a rapid assessment of lupus-associated traits. Overt signs can be detected between 4 and 6 months in the NZB/W, BXSB, Sle123 and Sle1 -associated strains (Fig. 2B and 2C).
12.After euthanizing, spray the mouse torso with 70% ethanol.
13.Harvest the spleen from the left side of the mouse carefully and place in a labeled 12-well plate in cold RPMI.
14.Harvest the kidneys carefully using a round edged pair of forceps and scissors, and place in a labelled 12-well plate containing cold RPMI.
15.Remove additional fat and connective tissue from the outside of the spleen and weigh, recording the findings.
Basic Protocol 2: END STUDY PROTOCOLS FOR TISSUE PROCESSING
Following the euthanasia and collection of tissues for immunological and pathology examination, the tissues are processed into single-cell suspensions for flow cytometry or prepared in fixatives or tissue preservatives. Given the length of time of the protocol, the kidney for single-cell analysis is processed initially; the portion of the spleen for DC analysis second; and the section of the spleen for plasmacytoid (p)DC, B, T, and myeloid cell analysis after that. All tissues should be kept on ice as much as possible unless the protocol dictates otherwise. Tissues for pathology and those set aside for microscopy analyses can be prepared for storage during the incubation and centrifugation times if personnel is limited. Templates for tissue processing (Fig. 3A) and cell count calculations for subsequent cell staining (Fig. 3B) can also be set up prior to the experiment.
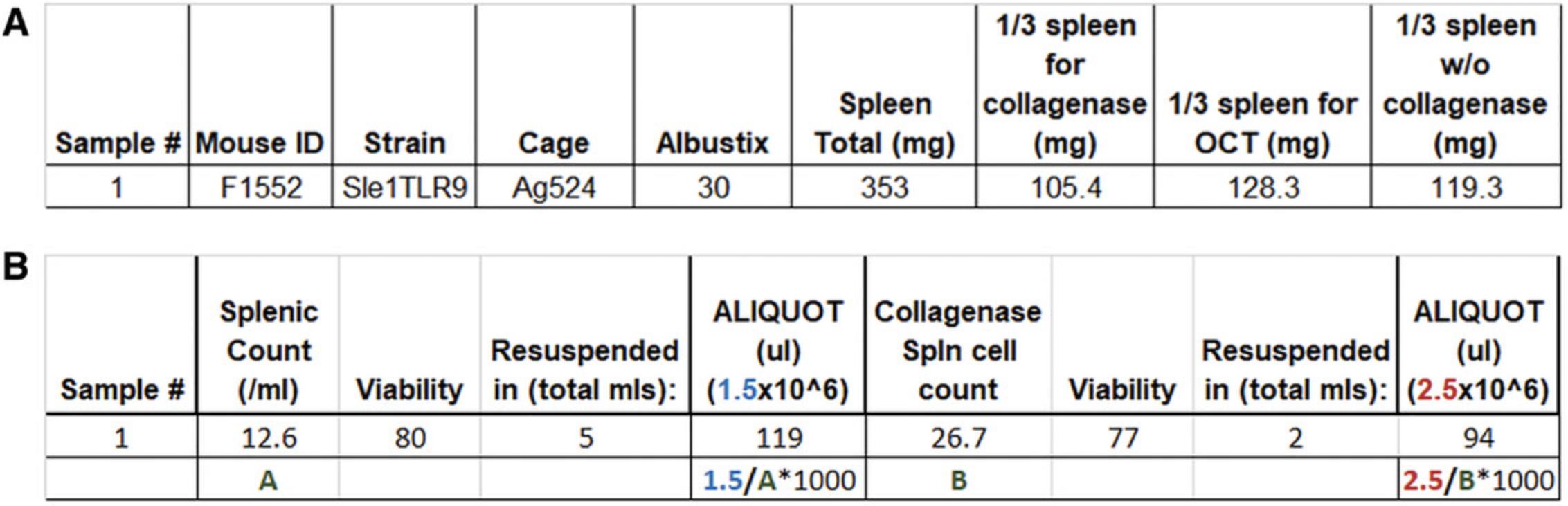
Materials
-
Spleens and kidneys collected from Basic Protocol 1
-
Phosphate-buffered saline (PBS), pH 7.4 (Gibco, cat. no. 10010)
-
Digestion buffer (see recipe)
-
RPMI 1640 medium (Gibco, cat. no.11875)
-
40% Percoll (see recipe)
-
FACS staining buffer (see recipe)
-
RPMI complete medium (RPMI-CM) (see recipe)
-
0.4% trypan blue solution (Sigma-Aldrich, cat. no. T8154)
-
Forceps
-
Single-edge razor blades (American Line, cat. no. 66-0089)
-
60 × 16 mm cell culture dishes (Nunc, cat. no. 150462)
-
1.7-ml microcentrifuge tubes (Axygen, cat. no. MCT-175-C)
-
Eppendorf 5810R refrigerated centrifuge
-
15-ml (Falcon, cat. no. 352070) and 50-ml (Falcon, cat. no. 352070) conical tubes
-
Orbital shaker
-
Syringes and needles:
- 20-ml Luer Lok syringe (BD, cat. no. 300141)
- 3-ml Luer Lok Syringe (BD, cat. no. 302113)
- 10-ml Luer Lok Syringe (BD, cat. no. 302149)
- 23-G PrecisionGlide™ needles (BD, cat. no. 302008)
- 20-G PrecisionGlide™ needles (BD, cat. no. 302026)
- 20-G PrecisionGlide™ needles (BD, cat. no. 302026)
- 23-G PrecisionGlide™ Needles (BD, cat. no. 302008)
-
70-µm nylon mesh (Sefar, cat. no. 03-70/33) or sterile 70-µm cell strainers (Falcon, cat. no. 352350)
-
Disposable Hemocytometer C-Chip Neubauer (Incyto, cat. no. DHC-N01)
-
96-well round-bottom tissue culture plates (Nunc, cat. no. 163320)
-
Additional reagents and equipment for counting viable cells by trypan blue exclusion (see Current Protocols article: Phelan & May, 2015)
Kidney processing for leukocyte infiltration and pathology
1.Remove the outer fibrous capsule layer of one kidney with forceps.
2.Chop the whole kidney in a petri dish using a square razor blade until finely minced.
3.Transfer to a new 1.5-ml microcentrifuge tube carefully using the razor blade. Add 500 µl PBS to the petri dish to wash out remaining tissue, and transfer to the microcentrifuge tube.
4.Centrifuge 5 min at 500 × g , 7°C.
5.Aspirate the kidney supernatant and aliquot 250 µl into one tube and the remainder in a new tube. Mark one of the tubes for future identification.
6.Add 1 ml room temperature digestion buffer to the pellet, breaking it up by pipetting up and down gently, and resuspending fully before transferring to a 50-ml conical tube.
7.Add 9 ml digestion buffer for a total of 10 ml.
8.Incubate at 37°C for 30 min on an orbital shaker at 150 rpm. Transfer to ice.
9.Using a 20-ml syringe with 20-G needle, aspirate the complete volume up and down until the suspension easily passes through the needle. Repeat with a syringe with 23-G needle.
10.Centrifuge 5 min at 453 × g , 4°C, then aspirate and discard the supernatant.
11.Resuspend the pellet in 5 ml RPMI 1640 and repeat steps 9 and 10 above.
12.Uncap a new set of 50-ml conical tubes and cut nylon mesh to cover the opening of each tube.
13.Transfer the 5-ml kidney suspension through the filter into the new 50-ml conical tube.
14.Wash the old tube with an additional 5 ml RPMI 1640 and transfer to the new tube through the filter (total 10 ml). Remove and discard the filter.
15.To the 10 ml cell suspension, add 10 ml of 40% Percoll, and mix by inverting.
16.Centrifuge 20 min at 1864 × g , room temperature, with no brake.
17.Aspirate the supernatant with debris and smaller tissue cells carefully. Since the pellet is soft, leave a small volume of liquid if necessary.
18.Resuspend the pellet in 1 ml and add 4 ml of FACS staining buffer (total 5 ml).
19.Centrifuge 5 min at 453 × g , 4°C, with the brake on.
20.Aspirate the supernatant and resuspend in 1 ml FACS staining buffer. Maintain on ice until staining.
Splenic processing into a single-cell suspension
21.Remove fat and surrounding the spleen.
22.Weigh the spleen and record the value.
23.Cut 1/3 for collagenase digestion for staining of DCs for flow cytometry
24.Cut 1/3 for processing and staining of B and T cells, myeloid populations, and pDCs.
25.Weigh cut spleen portions and record values.
26.Crush spleen portions with a piston from a 5-ml syringe in a petri dish.
For DC analysis (<1/3)
27a. Add 5 ml digestion buffer.
28a. Transfer to a 50-ml conical tube and incubate at 37ºC for 30 min on an orbital shaker at 150 rpm.
29a. Using a 10-ml syringe with 20-G needle, aspirate the complete volume up and down until the suspension easily passes through.
30a. Centrifuge 5 min at 453 × g , 4ºC.
31a. Aspirate the supernatant and resuspend in 5 ml FACS staining buffer.
32a. Using a 10-ml syringe with 20-G needle, aspirate the complete volume up and down until the suspension easily passes through. Repeat with a syringe with 23-G needle.
33a. Transfer to a 15-ml conical tube through nylon mesh (see step 12).
34a. Wash the old 50-ml tube with 5 ml FACS staining buffer and transfer to the new 15-ml tube through the mesh.
35a. Centrifuge 5 min at 453 × g , 4ºC.
36a. Aspirate the supernatant and resuspend in 1 ml FACS staining buffer.
37a. Using a hemocytometer and trypan blue exclusion (Phelan & May, 2015), count viable cells and resuspend to a concentration of 20 × 106/ml immediately prior to aliquotting using FACS staining buffer.
38a. Aliquot 100 µl/well in a round-bottom 96-well plate.
For B/T/pDC/myeloid analysis (>1/3)
27b. Add 5 ml FACS staining buffer.
28b. Transfer to 50-ml tube through either nylon mesh or a sterile 70-µm strainer.
29b. Centrifuge 5 min at 453 × g , 4ºC.
30b. Aspirate the supernatant and resuspend in 5 ml FACS staining buffer.
31b. Using a hemocytometer and trypan blue exclusion, count viable cells (Phelan & May, 2015) and resuspend to a concentration of 10 × 106/ml immediately prior to aliquotting using FACS staining buffer.
32b. Aliquot 100 µl/well in a round-bottom 96-well plate.
39.At this stage, there should be 2 million cells from the collagenase-processed spleen and 1 million cells from the non-collagenase-processed spleen. Maintain the cells on ice until ready to add the flow cytometry staining reagents. This time should not exceed 1 hr.
Basic Protocol 3: IMMUNOPHENOTYPING USING FLOW CYTOMETRY PROTOCOLS
SLE is an immunological disease; however, the majority of described protocols do not assess the immunological cellular changes that occur with disease onset. An expansion and hyperactivation of leukocytes, including B cells, T cells, dendritic cells (DCs), and myeloid cells, contributes to the development of end-organ pathology (Pathak & Mohan, 2011). Therefore, the flow cytometry assays described here are used to identify key changes in these leukocytes exhibited in lupus-prone models. It is recommended that the user be experienced at flow cytometry. Excellent guidelines for flow cytometry have been described (Cossarizza et al., 2017). Use standard flow cytometer machine setup protocols. Ensure that the negative unstained control is on scale and that photomultiplier tube (PMT) settings are adjusted so that the fluorochrome is brightest in its designated channel compared to every other channel. Before running any sample, check the setup with one fully stained B6 sample to make sure that the flow cytometer has been set up appropriately. All antibodies used in the panels should be titrated for optimal use, and the multi-color panel should be tested prior to the end of study. This is usually done with a naïve, B6 strain and a severe lupus-prone strain, given the changes indicated in this protocol.
Materials
- Splenocytes (Basic Protocol 2, step 38a or 32b) or kidney cells (Basic Protocol 2, step 20)
- Blocking Ig: Rat anti–mouse FcγRII/III (BD, cat. no. 553142) or supernatant of the 24G2 cell line
- This should be titrated to optimize for blocking by using a directly conjugated anti-FcγRII/III antibody in a similar staining strategy to that described below.
- Antibody master mix (examples of panels created for the analysis of B, T and myeloid are shown below in Tables 1, 2 and 3, respectively; an example of the preparation of a B cell panel for 10 samples is shown in Table 4)
- FACS staining buffer (see recipe)
- FACS Lysing Solution (BD, cat. no. 349202; prepared as per manufacturer's recommendations)
- 1% paraformaldehyde solution (see recipe)
- Peripheral blood (see Basic Protocol 1)
- Phosphate-buffered saline (PBS), pH 7.4 (Gibco, cat. no. 10010)
Antibody reactivity | Conjugate | Clone | Company | Cat. no. |
---|---|---|---|---|
GL7 | FITC | GL7 | BD | 553666 |
CD138 | PE | 281-2 | BD | 553714 |
CD19 | PE-Texas Red | 6D5 | Thermo Fisher | RM7717 |
CD23 | PE-Cyanine7 | B3B4 | Thermo Fisher | 25-0232-82 |
CD5 | APC | 53-7.3 | BD | 550035 |
B220 | APC/Cyanine7 | RA3-6B2 | Biolegend | 103224 |
CD11b | Alexa Fluor 700 | M1/70 | Thermo Fisher | 56-0112-82 |
CD21/CD35 | eFluor 450 | eBio4E3 (4E3) | Thermo Fisher | 48-0212-82 |
CD45 | V500 | 30-F11 | BD | 561487 |
IgM | BV650 | R6-60.2 | BD | 564027 |
Fas | Biotin | 15A7 | Thermo Fisher | 13-0951-85 |
Streptavidin | BV711 | BD | 563262 |
- a
The table shows a list of the antibodies to the 11 markers which are directly conjugated to the respective fluorochromes shown. This panel was used in the gating strategy and example of expected results shown in Figures 4, 5. The biotinylated antibody was detected using secondary streptavidin staining, as described above. This data was acquired on a BD LSR II 4 laser flow cytometer with the indicated lasers for fluorochrome detection: blue-488 nM (blue shading), yellow/green-561 nM (green shading), red-633 nM (red shading), and violet-405 nm (violet shading).
Antibody reactivity | Conjugate | Clone | Company | Cat. no. |
---|---|---|---|---|
PD1 (CD279) | FITC | J43 | Thermo Fisher | 11-9985-82 |
ICOS (CD278) | PE | 7E.17G9 | Thermo Fisher | 12-9942-82 |
CD62L (L-selectin) | PE-Texas Red | MEL-14 | Thermo Fisher | RM4317 |
CD45 | PE-Cyanine7 | 30-F11 | Thermo Fisher | 25-0451-82 |
CD44 | APC | IM7 | BD | 559250 |
CD4 | APC/Cyanine7 | GK1.5 | BD | 561830 |
CD3 | eFluor 450 | 17A2 | Thermo Fisher | 48-0032-82 |
CXCR5 | Biotin | 2G8 | BD | 551960 |
Streptavidin | BV655 | BD | 563855 |
- a
The table shows a list of the antibodies to the 8 markers which are directly conjugated to the respective fluorochromes or biotin shown. The biotinylated antibody was detected using secondary streptavidin staining, as described above. This panel is designed for a 4-laser system. For the expected results in Figures 6, 7, data was acquired on a BD LSR II 4 laser flow cytometer with the indicated lasers for fluorochrome detection: blue-488 nM (blue shading), yellow/green-561 nM (green shading), red-633 nM (red shading), and violet-405 (violet shading).
Antibody reactivity | Conjugate | Clone | Company | Cat. no. |
---|---|---|---|---|
mPDCA1 (CD317) | FITC | eBio927 | Thermo Fisher | 11-3172-82 |
SiglecH | PerCP/Cyanine5.5 | 551 | Biolegend | 129614 |
CD103 | PE | 2E7. | Biolegend | 121406 |
CD11c | PE-Texas Red | N418 | Thermo Fisher | MCD11c17 |
CD45 | PE-Cyanine7 | 30-F11 | Thermo Fisher | 25-0451-82 |
CD115 | APC | AFS98 | Thermo Fisher | 17-1152-82 |
Gr1 (Ly6G/Ly6C) | APC/Cyanine7 | RB6-8C5 | BD | 557661 |
NK1.1 | Alexa Fluor 700 | PK136 | Biolegend | 108730 |
CD11b | eFluor 450 | M1/70 | Thermo Fisher | 48-0112-82 |
Live/Dead (L/D) | Zombie Aqua | Fixable Viability Kit | Biolegend | 423102 |
CD4 | BV605 | RM4-5 | Biolegend | 100548 |
MHC II (I-A/I-E) | BV650 | M5/114.15.2 | Biolegend | 107641 |
CD138 (Syndecan-1) | BV711 | 281-2 | Biolegend | 142519 |
F4/80 | BV785 | BM8 | Biolegend | 123141 |
Ly6G | BUV395 | 1A8 | BD | 563978 |
B220 | BUV661 | RA3-6B2 | BD | 612972 |
CD3 | BUV737 | 145-2C11 | BD | 564618 |
CD8 | BUV805 | 53-6.7 | BD | 564920 |
- a
The table shows a list of the antibodies to the 18 markers which are directly conjugated to the respective fluorochromes shown. This panel is designed for a 5-laser system. For the results in Figures 9-12, data was acquired on a BD Symphony A5 flow cytometer with the indicated lasers for fluorochrome detection: blue-488 nM (blue shading), yellow/green-561 nM (green shading), red-633 nM (red shading), and violet-405 (violet shading).
Staining step | Antibody reactivity | Conjugate | Dilution (2×) | Volume at 2× (μl) | Final concentration (μg/ml) |
---|---|---|---|---|---|
Fc Block (2×) | CD16/CD32 | None | 1:100 | 2.5 | 2.5 |
FACS staining buffer | 247.50 | ||||
Total | 250 | ||||
Aliquot 25 µl to each well | |||||
Primary antibody stain | GL7 | FITC | 1:50 | 5 | 5.00 |
CD138 | PE | 1:100 | 2.5 | 1.00 | |
CD19 | PE-Texas Red | 1:100 | 2.5 | 0.25 | |
CD23 | PE-Cyanine7 | 1:300 | 0.83 | 0.33 | |
CD5 | APC | 1:400 | 0.63 | 0.25 | |
B220 | APC/Cyanine7 | 1:100 | 2.5 | 1.00 | |
CD11b | Alexa Fluor 700 | 1:100 | 2.5 | 1.00 | |
CD21/CD35 | eFluor 450 | 1:75 | 3.33 | 1.33 | |
CD45 | V500 | 1:100 | 2.5 | 1.00 | |
IgM | BV650 | 1:200 | 1.25 | 0.50 | |
Fas | Biotin | 1:50 | 5 | 5.00 | |
FACS Buffer | 221.46 | ||||
Total | 250 | ||||
Aliquot 25 µl to each well | |||||
Wash steps | |||||
Dilution (1×) | Volume at 1× (μl) | Final concentration (μg/ml) | |||
Secondary antibody stain | Streptavidin | BV711 | 1:400 | 1.25 | 0.25 |
FACS Buffer | 497.75 | ||||
Total | 500 | ||||
Aliquot 50 µl to each well |
- a
The dilutions of fluorescent antibodies described in Table 2 are shown. The table shows calculated volumes for preparation of the master mix for 10 samples, with void volume included (recommended 1-2 extra sample volumes).
- 96-well round-bottom tissue culture plates (Nunc, cat. no. 163320)
- Nunc Sealing Tapes (ThermoFisher, cat. no. 236370)
- Refrigerated centrifuge
- 70-µm nylon mesh filtering (Sefar, cat. no. 03-70/33)
- Falcon® round-bottom polystyrene tubes (Corning, cat. no. 352001)
- Flow cytometer
Methods I: splenocytes/kidney
1a. Pipette 100 µl splenocyte suspension (Basic Protocol 1, step 38a or 32b) or 200 µl kidney cells (Basic Protocol, step 20) into a 96-well round bottom tissue culture plate at a concentration of 500,000-2 × 106/well, depending on cell subsets to be analyzed. Prepare the peripheral blood (see “b” steps below) in a separate plate.
2a. Pellet the cells by centrifuging the plates 5 min at 453 × g , 4°C.
3a. Check that the cells have pelleted at the bottoms of the wells of the 96-well plate. Flick off the supernatant from the spleen and kidney plates.
4a. To the cell pellet, add 25 µl (for splenocytes), or 35 µl (for collagenase splenocytes/kidney cells) of blocking IgG in FACS staining buffer at twice the concentration (2×) of the final desired concentration (5 µg/ml anti-FcγRII/III or 2× of the titrated 24G2 supernatant). Do not wash.
5a. Add an equal volume of antibody at 2× concentration in FACS staining buffer. For single-fluorochrome color compensation controls, 25 µl of the specific primary antibody at optimal concentration in FACS staining buffer should be added. This can be anything from 1:25 to 1:1600, so the final concentration is 1:50 to 1:800.
6a. Incubate the cells on ice for 30 min in the dark.
7a. For T cell panels, include 2× anti-CXCR5 (see Table 2) in the initial anti-FcγRII/III stage (step 4a). Incubate with 25 µl of the anti-CXCR5/block solution for 15 min at 37°C (depending on the clone of antibody). Add all other antibodies in a master mix (2×) as for other panels, and incubate on ice for 30 min, before continuing as for all other panels.
8a. Wash the cells three times with FACS staining buffer, each time by centrifuging 5 min at 453 × g , 4°C.
9a. For panels with additional secondary antibody staining, such as the B cell and T cell panels, add the fluorescently labeled streptavidin anti-biotin conjugate (Table 1) for a period of 30 min, on ice.
10a. Wash cells once with FACS staining buffer as in step 8a, above, then add 100 µl BD FACS Lysing Solution, making sure to break the cell pellets up when adding. After 30 s, add 100 µl FACS staining buffer and centrifuge immediately for 5 min at 1500 rpm/5 min/4°C
11a. Wash the cells two times with FACS staining buffer , each time by centrifuging 5 min at 453 × g , 4°C.
12a. Flick off supernatant as above and add 1% paraformaldehyde. Maintain at 4°C in the dark until analysis on the flow cytometer. Acquire on a flow cytometer within 36 hr.
13a. If storing flow cytometry samples overnight before acquiring data, it is recommended that time be set aside for centrifuging and resuspending the samples in FACS staining buffer before filtering with 70-µm nylon mesh into Falcon® round-bottom polystyrene tubes before running FACS. This will reduce variability between samples and prevent clogging of the machine.
Methods II: Peripheral blood
In some cases, detection of peripheral blood T cell activation can provide useful information about ongoing disease. The following protocol can be used to assess immunological phenotypes in the peripheral blood of lupus mice. Due to the leukopenia and anemia observed in some models with severe disease, care should be taken at the lysis step to prevent over-lysing and leukocyte cell death.
1b. Pipette 100 µl of peripheral blood (from same mouse as kidney/spleen) into a 96-well round bottom tissue culture plate separate from the kidney/splenocytes.
2b. Add 100 µl of PBS to the peripheral blood and attach a plate sealer to eliminate well-to-well contamination.
3b. Centrifuge the plates 5 min at 453 × g , 4°C.
4b. Aspirate 130 µl of the clear plasma/PBS from the peripheral blood carefully.
5b. Add 70 µl of master antibody mix for B, T, or myeloid cells (1, 2, or 3) and incubate in the refrigerator for 30 min.
6b. Following the incubation, add 80 µl of FACS staining buffer, and centrifuge as in step 3b, above.
7b. Aspirate the supernatant carefully and add 150 µl BD FACS Lysing Solution. Mix well by pipetting up and down and incubate for 5 full minutes. Centrifuge as in step 3b, above.
8b. Aspirate the supernatant carefully and add 100 µl of BD FACS Lysing Solution. Mix well by pipetting up and down and incubate for 1-2 min. Observe the cell suspension for translucency. Add 100 µl FACS staining buffer to the wells when the cell suspension has turned translucent.
9b. Wash the cells two times in staining buffer, each time for 5 min at 453 × g , 4°C. Resuspend in 1% paraformaldehyde and keep in the dark and on ice before running on a flow cytometer.
10b. If storing flow cytometry samples overnight before acquiring, it is recommended that time be set aside for centrifuging and resuspending the samples in staining buffer before filtering with 70-µm nylon mesh into Falcon® round-bottom polystyrene tubes before FACS. This will reduce variability between samples and prevent clogging of the machine.
Flow cytometry analysis of key leukocytes in SLE
The fluorochromes and capacity of the flow cytometry panel depend on the capability and equipment that the user has access to. Therefore, in this protocol, suggestions are given of panels to identify key changes in spleens and kidney of lupus-prone mice, with some examples. The investigator should optimize their own panels to suit their flow cytometry skills and equipment.
B cell subsets
B cells play a critical role in the development of lupus. They produce the autoantibodies that are a hallmark of SLE. The major splenic B cell changes that occur across multiple lupus models include the expansion of plasma cells and germinal center (GC) B cells, and the reduction of the marginal zone (Mz) B cells. These can be analyzed separately across several panels in a 4-color format (Table 5), or in a larger (single) panel (Table 1, Fig. 4), depending on the available equipment. Exclusion of dead cells using viability dyes and the use of open (unused) channels for the exclusion of auto-fluorescent particles will also provide increased resolution. Additional antibodies can also be used to assess activation and maturation. CD69 is up-regulated in a transient manner following activation. Age-associated B cells (ABCs) are expanded in lupus, and can be detected using CD11c+CD11b+. Transitional and B1 subsets can also be identified using additional markers.
Plasma/plasmablast | Marginal zone (MZ) | Germinal center (GC) |
---|---|---|
CD45 | CD45 | CD45 |
B220 | B220 | B220 |
CD138 | CD21 | GL7 |
CD23 | Fasb |
- a
B cell subsets can be identified using the combinations shown. B cells are CD45+ and B220+, plasma cells are B220-CD138+ and plasmablasts are B220+CD138+. Additional resolution can be achieved using live/dead stains, CD19 to confirm mature B cells, and an open/blank channel to exclude autofluorescence from other cells. Germinal center B cells are GL7 and Fas positive.
- b
Note that the detection of the Fas marker for germinal center cells is challenging. Peanut Agglutinin (PNA) can be used instead for resolution if this is an issue.
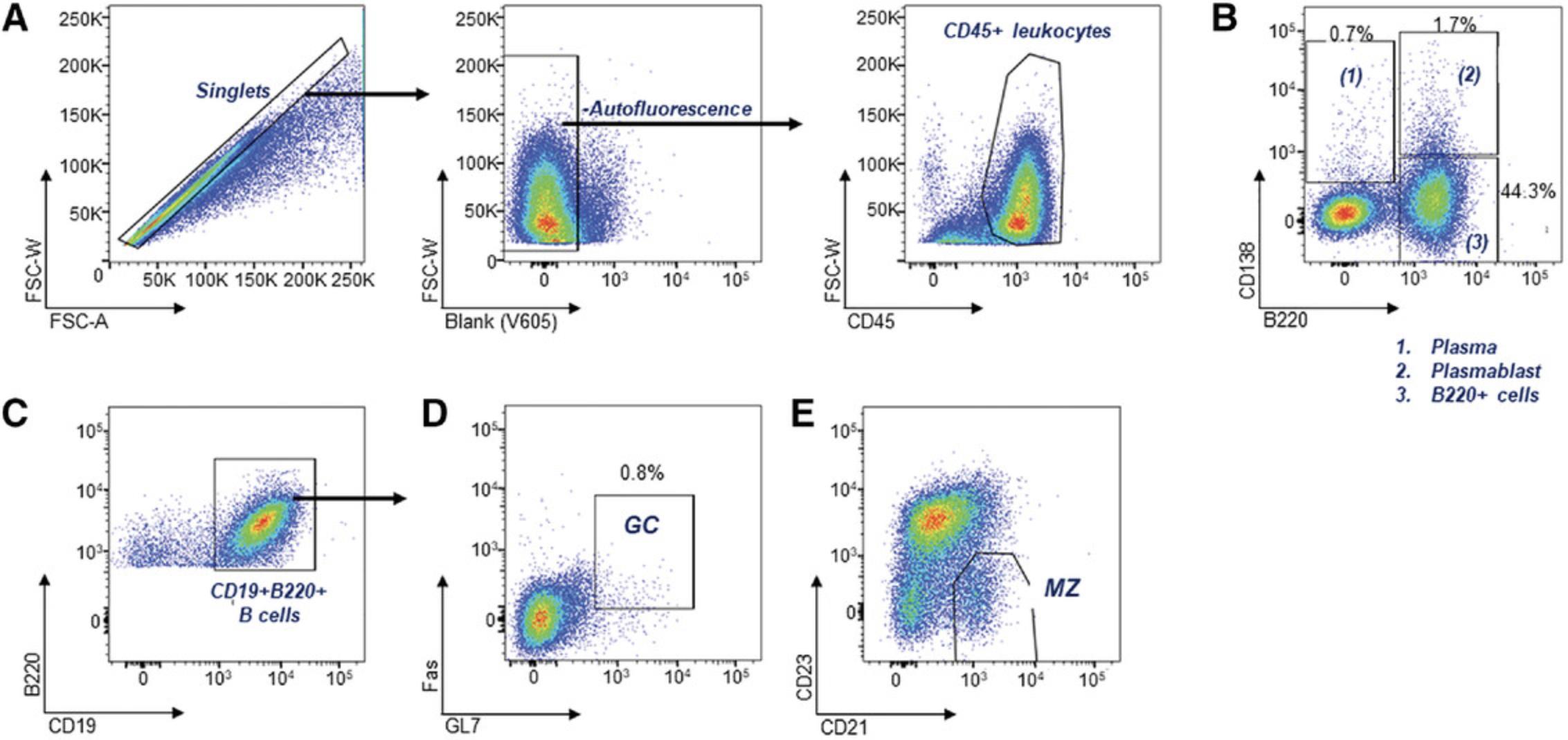
An example of an 11-color B cell panel is shown in Table 1. An example of B cell subset data in lupus-prone mice is shown in Figure 5.
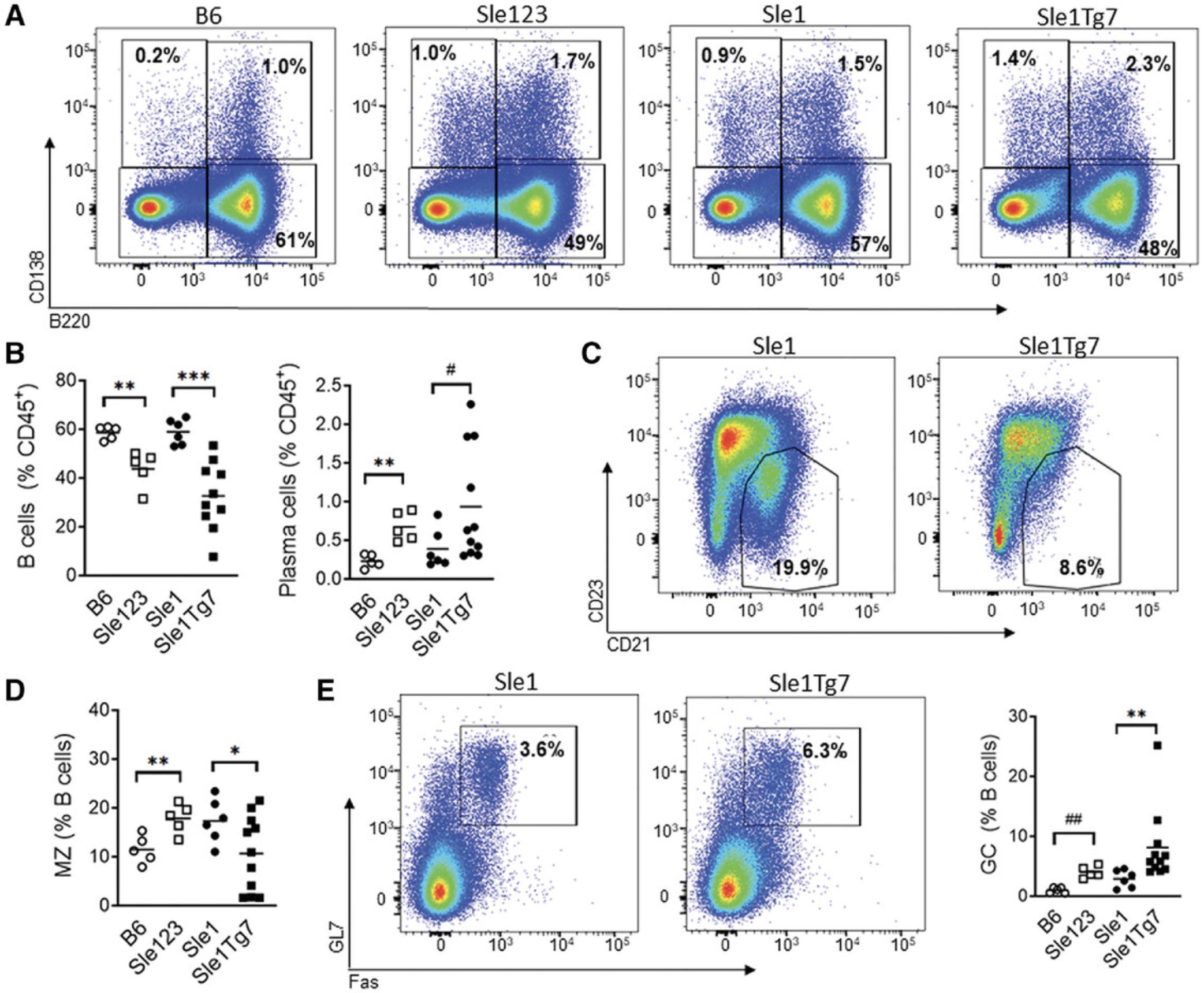
An example of the dilutions and actual volumes used in this panel is shown in Table 4. Note that actual dilutions of antibody will differ according to fluorescent conjugate, manufacturing company, and lot number for the fluorescently conjugated antibody, and the flow cytometer used. This table is for example only.
T cell subsets
CD4+ T cell subsets play critical roles in disease development and progression. They provide help to other leukocytes through ligand-receptor interactions with dendritic cells (DCs) and B cells, and through the secretion of cytokines. Activated and memory T cells are expanded in SLE, whereas regulatory T (TREG) compartments are reduced, with lower efficacies (Ohl & Tenbrock, 2015). In addition, there is an expansion of the T follicular helper population (Tfh) with a concomitant increase in the expression of PD-1 and ICOS. The key T cell subsets used in lupus-prone murine systems are shown in Table 6; these can be analyzed separately across several panels in a 4-5 color format, or alternatively combined in a single panel (Table 2), depending on the available flow cytometry equipment. Additionally, regulatory T cells (Tregs) can be assessed using antibodies to CD25 and the intracellular transcription factor, foxp3.
T cell lineage | Naïve/memory | T follicular helper (Tfh) and activation |
---|---|---|
CD45 | CD45 | CD45 |
CD3 | CD3 | CD3 |
CD4 | CD4 | CD4 |
CD8 | CD8 | ICOS |
CD62L | CXCR5 | |
CD44 | PD1 |
- a
Following single-cell resolution, reduction of auto-fluorescent cells and CD45+ leukocyte identification, T cell subsets can be identified using the combinations shown. T cells are identified as CD45+CD3+ with 2 major subsets as CD4+ and CD8+. Naïve T cells are identified as CD62Lhigh and CD44low, and memory T cells as CD62Llow and CD44high; T follicular helper cells are identified as CD3+CD4+ and CXCR5+PD1+ and ICOS+. Fluorescent antibodies to these markers can be selected according to the flow cytometer that is available to the investigator.
An example of a gating strategy used to identify key T cell subsets and activation in the spleen is shown in Figures 6 and 7, within examples of renal T cell gating and changes following lupus disease in Figure 8, which are described further in the Understanding Results section.
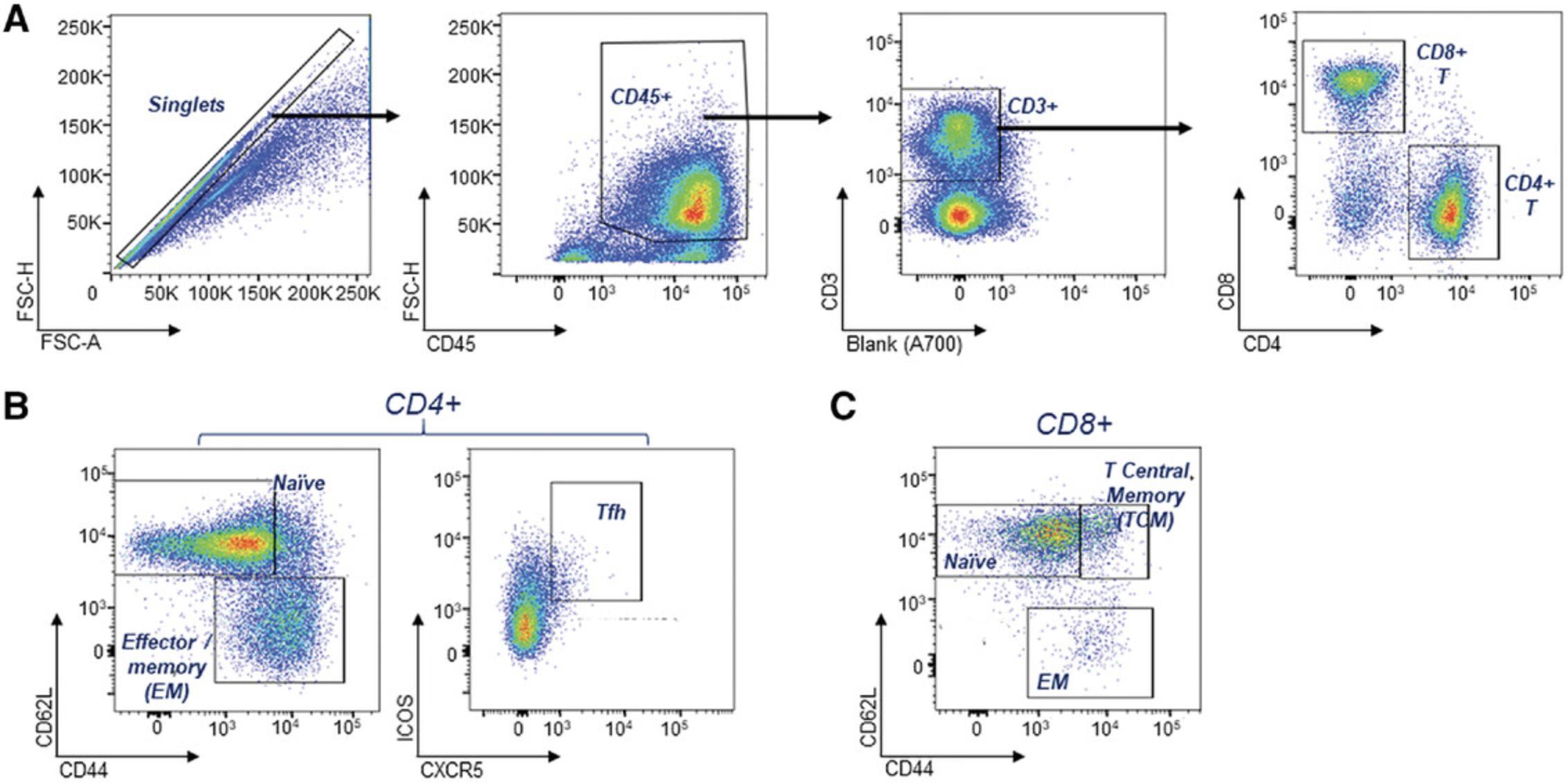
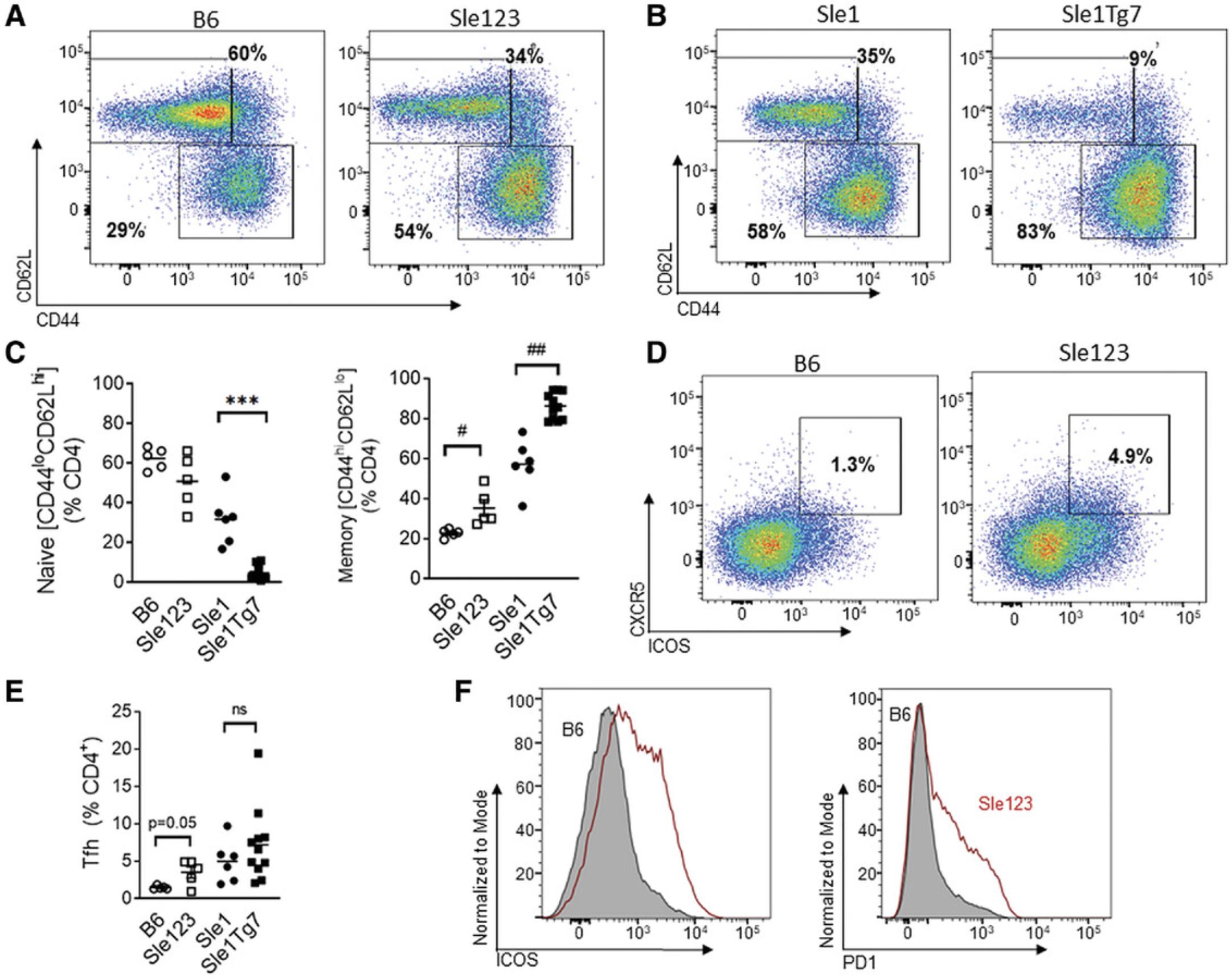
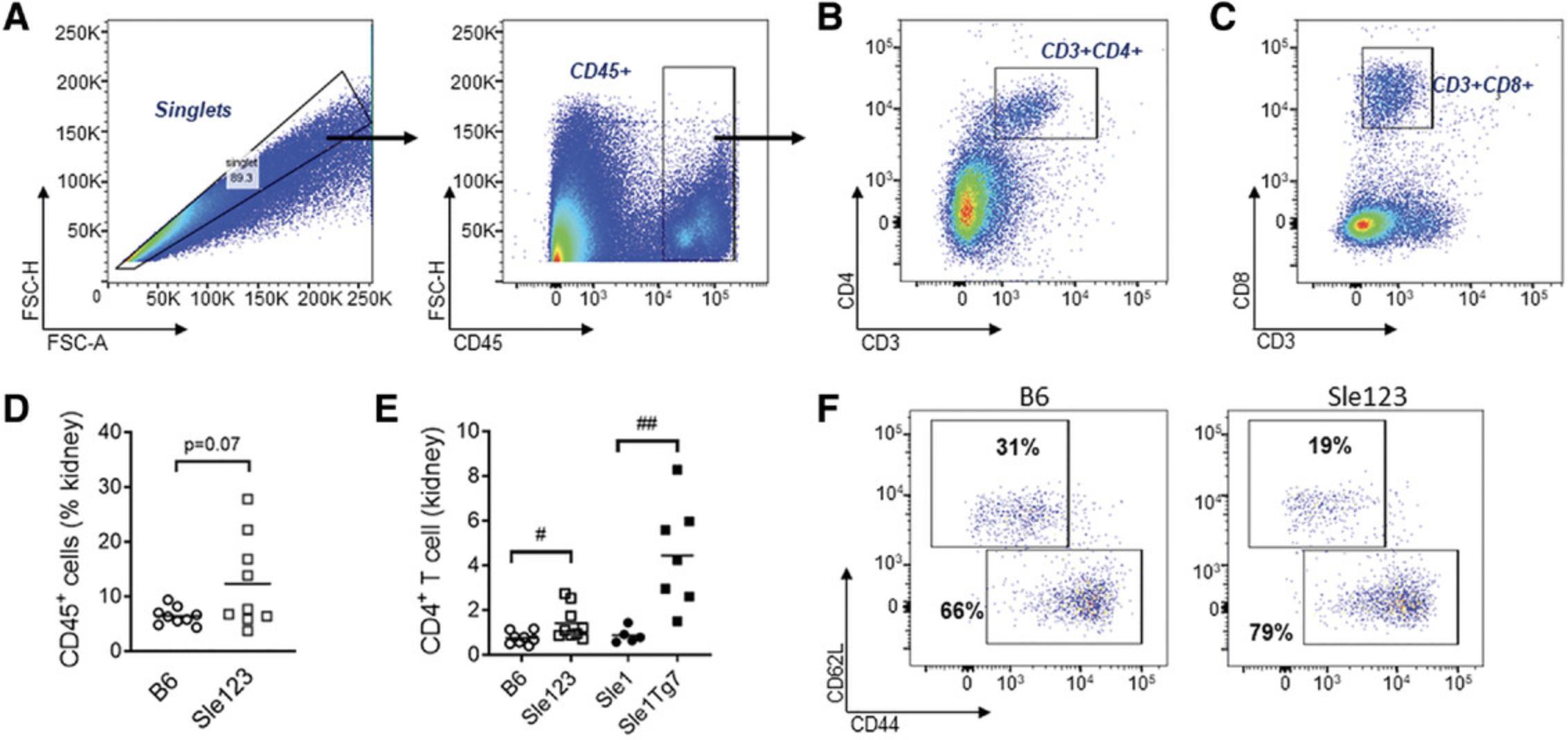
Identification of major leukocyte subsets
NZB/W, NZM2410, Sle123 and Sle1 -related severe disease strains are associated with myelopoesis. The introduction of a simple or complex staining panel can be used to identify the major change in the leukocyte subsets, which will show this myeloid expansion, or changes in less frequent populations. Table 7 describes key markers used to identify key leukocyte alterations associated with lupus-like traits. The use of additional markers (CD19, CD3, NK1.1) enables the exclusion of B, T, and NK cells, which is necessary given their capacity to also express myeloid-associated surface expression markers. In addition, the autofluorescence of granulocytes, which may result in their inclusion of other myeloid and dendritic cell (DC) populations, may be removed using anti-Gr1. DC panels identified in Table 7 Table 8 can be further divided into smaller panels to identify plasmacytoid DCs (pDCs; CD45+mPDCA1+B220+SiglecH+), which secrete high levels of type I interferon (IFN), and conventional (c) CD45+CD11c+MHC+ DC subsets, which include CD8+ and CD103+ cDCs (DC1) and CD11b+ cDCs (cDC2) (Desch et al., 2011; Jakubzick, Helft, Kaplan, & Randolph, 2008; Sung et al., 2006; Vermaelen & Pauwels, 2004).
Lineage, myelopoeisis, and DC expansion | ||
---|---|---|
Major leukocyte lineages | Myeloid subsets | DC subsets |
CD45 | CD45 | CD45 |
CD4 | CD3/B220/NK1.1b | Gr1/CD3/CD19/NK1.1b |
CD8 | CD11b | B220 |
CD19 | Gr1 | mPDCA1 |
CD11b | CD11c | CD8 |
CD3 | Ly6G | CD11c |
F4/80 | MHCII | |
CD115c and/or Ly6G | CD11b | |
F4/80 | ||
CD103 |
- a
Following single-cell resolution, reduction of auto-fluorescent cells, and CD45+ leukocyte identification, assessment of the most frequent populations, together with more refined analyses of the typical myeloid expansion, can be completed using the combinations shown.
- b
Other leukocytes maybe gated out using a “sink” gate, with all antibodies to non-desired markers bound to the same bright fluorochrome.
- c
CD115 does not work well for kidney samples due to the collagenase in the preparation. In this case, exclusion of NK cells, T cells, and DCs can be used to confirm monocytes. Neutrophils can also be separated out by their specificity to Ly6G. Full clarification of leukocyte subsets is shown in Figure 9.
Observation | Possible problem | Rationale and solution | |
---|---|---|---|
1. | Poor cell staining/too few cells on sample acquisition at flow cytometer | Time taken to process tissue | These protocols described here attempt to assess a wide range of tissues and cellular activation. We recommend teamwork at the cohort processing stage to keep processing time at a minimum (see Time Considerations). Extended time taken can result in apoptosis and the loss of surface markers on leukocytes. |
2. | High variability in ANA values; high ANAs titers in B6 control mice. | Maintenance of blood at the incorrect temperature | Blood is stored immediately on wet ice for this protocol to reduce background in ANA ELISAs |
3. | Incomplete resolution of leukocytes in peripheral blood. | Incomplete or too much lysis of tissue | The presence of too many red blood cells in the peripheral blood staining particularly can result in challenges in the analysis of the leukocyte population. Using a time without optimization can result in leukocyte cell death, particularly neutrophils. |
4. | No neutrophils in the lineage panel | Poor storage or excessive red blood cell lysis | Blood and tissues are maintained on wet ice, and changes in temperature should be avoided to prevent clumping and loss of neutrophils. Excessive lysis, or use of ACK lysis buffer, will eliminate neutrophils. Lysis should be completed at the end of the flow cytometry protocol, in a fix/lyse step to maintain the more delicate leukocyte subsets, particularly the myeloid population. |
5. | Increased background in flow plots; no clear resolution of leukocyte subsets | Dim fluorochrome chosen for CD45; or fluorescently labeled antibodies not titrated effectively; or increased dead cells in sample preparation | We have found that pecy7 and APC are optimal for CD45 for tissue analysis of infiltrating leukocytes. The use of a viability dye to exclude dead cells will enhance resolution of cell populations in flow cytometry analysis: Zombie-apccy7 (Zombie NIR™ Fixable Viability Kit, Biolegend cat. no. 423106) or 7-AAD. |
6. | Unable to collect urine from sick mice for protein analysis | Sick mice urinate less | As others have recommended before us (Seavey, Lu et al. 2011), we recommend allocating time for sampling 2-3 weeks, prior to the endpoint sacrifice date to ensure urine collection. |
7. | Difficulty in syringing kidney tissue during single cell preparation | This indicates that the DNase is not of sufficient quality or degraded | Optimization of DNase concentration is then recommended, or purchase fresh DNase |
8. | Poor resolution of CD45, despite choosing optimal fluorochrome and optimizing dilution | This indicates apoptosis or overloaded cell number for the volume of master mix. It can also indicate decoupling of antibody tandem dyes. | Do not overload cell number in plate; if larger cell numbers are needed for acquisition, titrate cell number and antibody solution volume. Store all antibodies in the dark, and check before use if they exceed the storage life indicated by the manufacturer. |
Table 3 shows a panel that provides a capacity to analyze all populations shown in Table 7. Similar panel designs have been described previously (Celhar et al., 2018; Celhar et al., 2019). Additional antibodies can be included to assess activation, such as CD80 and CD86, which increase on DCs, and CD62L, but are lost from the cell surface of neutrophils and monocytes. The advantages of reducing time during a cohort-euthanizing day by using one expansive panel can be offset by the user's ability to run high-dimensional panels. Therefore, this must be considered with care.
An example of the gating strategy to stratify subsets using the panel indicated in Table 3 is shown in Figure 9. A full description with the changes in the splenic leukocytes exhibited in lupus-prone mice is shown in Figure 10. Furthermore, an example of gating leukocytes in the kidney is shown in Figure 11, with examples of the differences due to lupus severity shown in Figure 12. This is detailed in the expected results section.
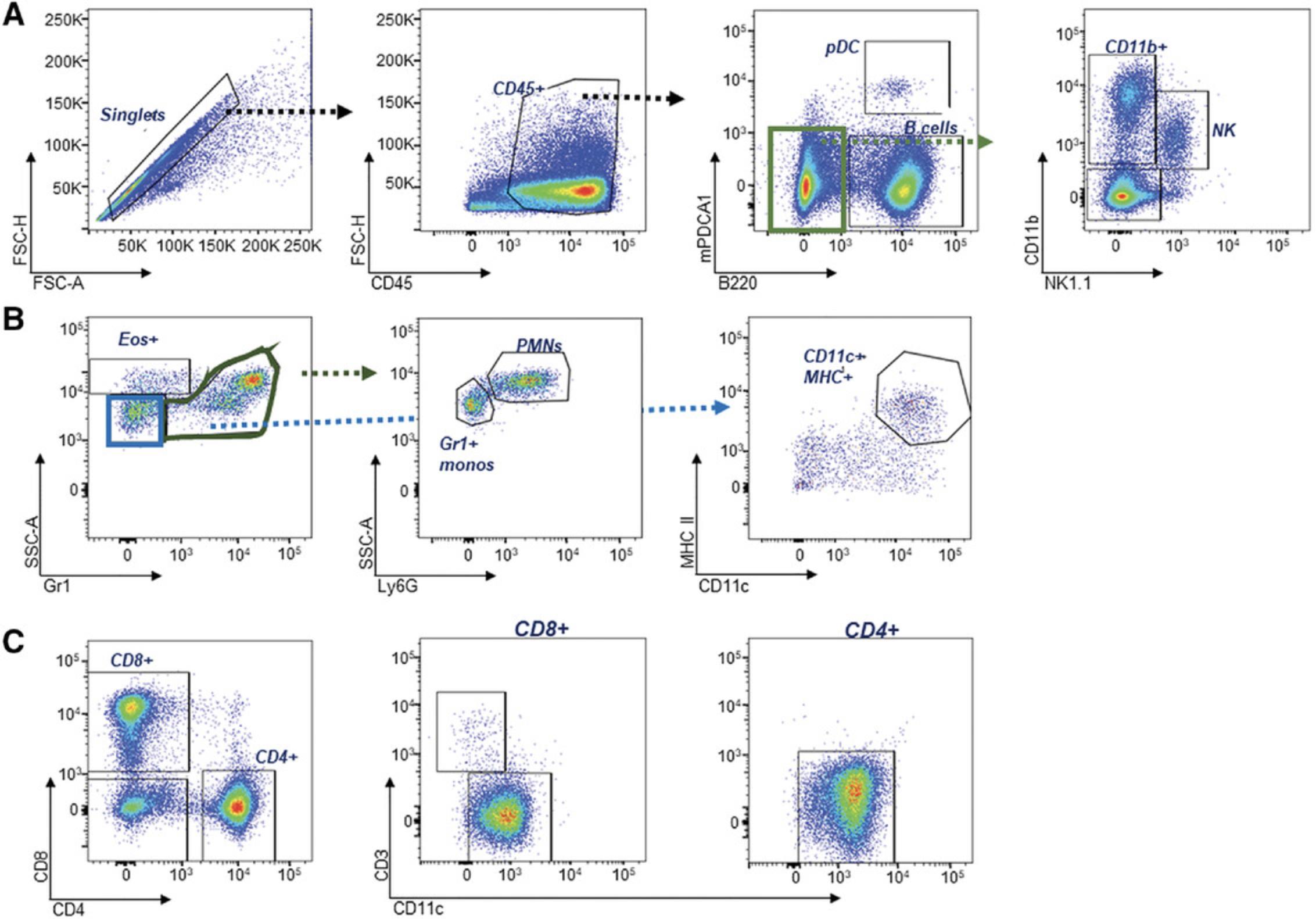
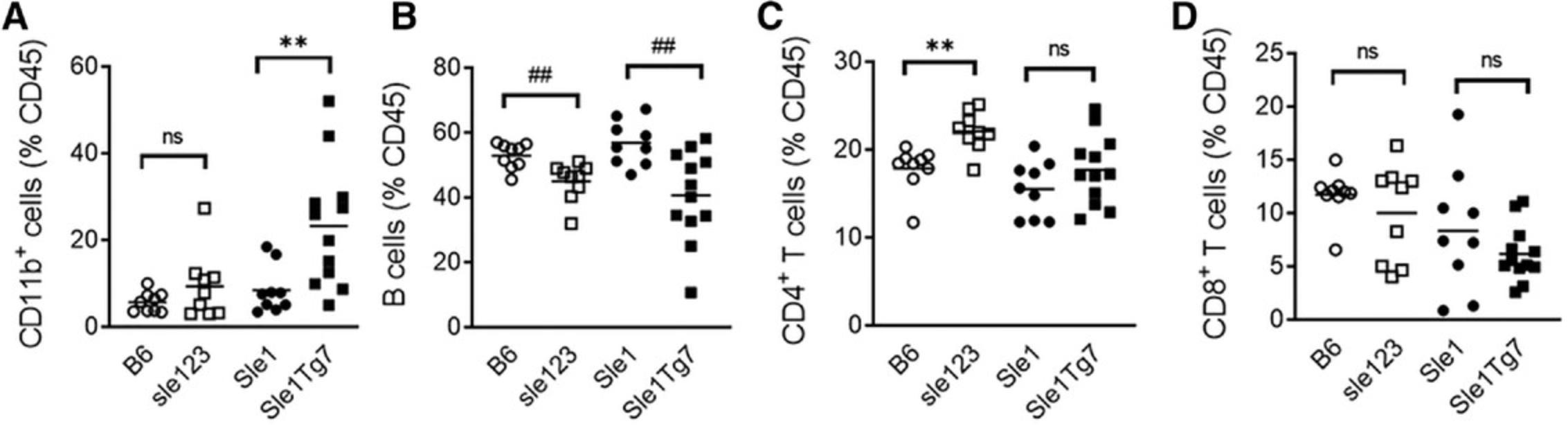
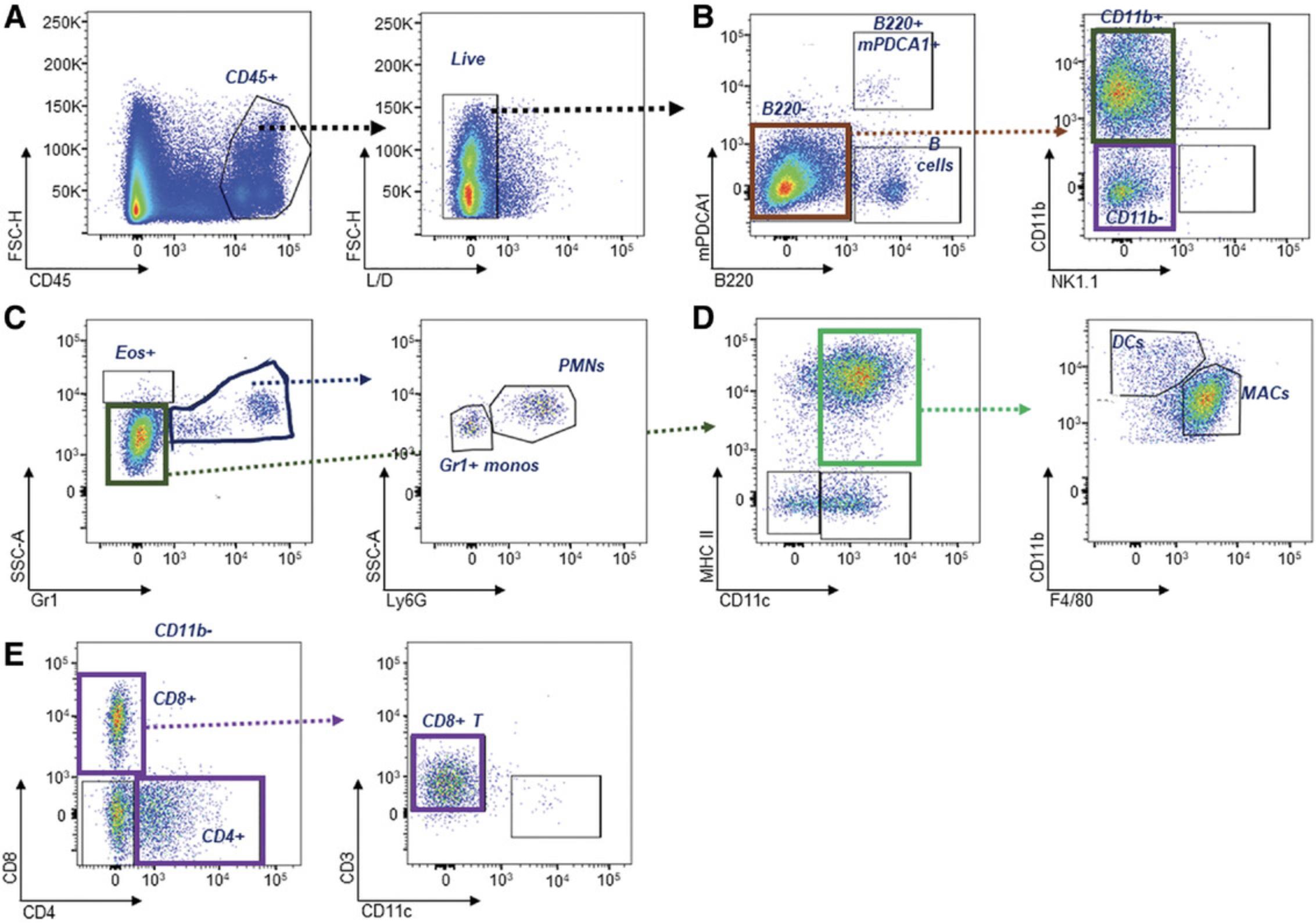
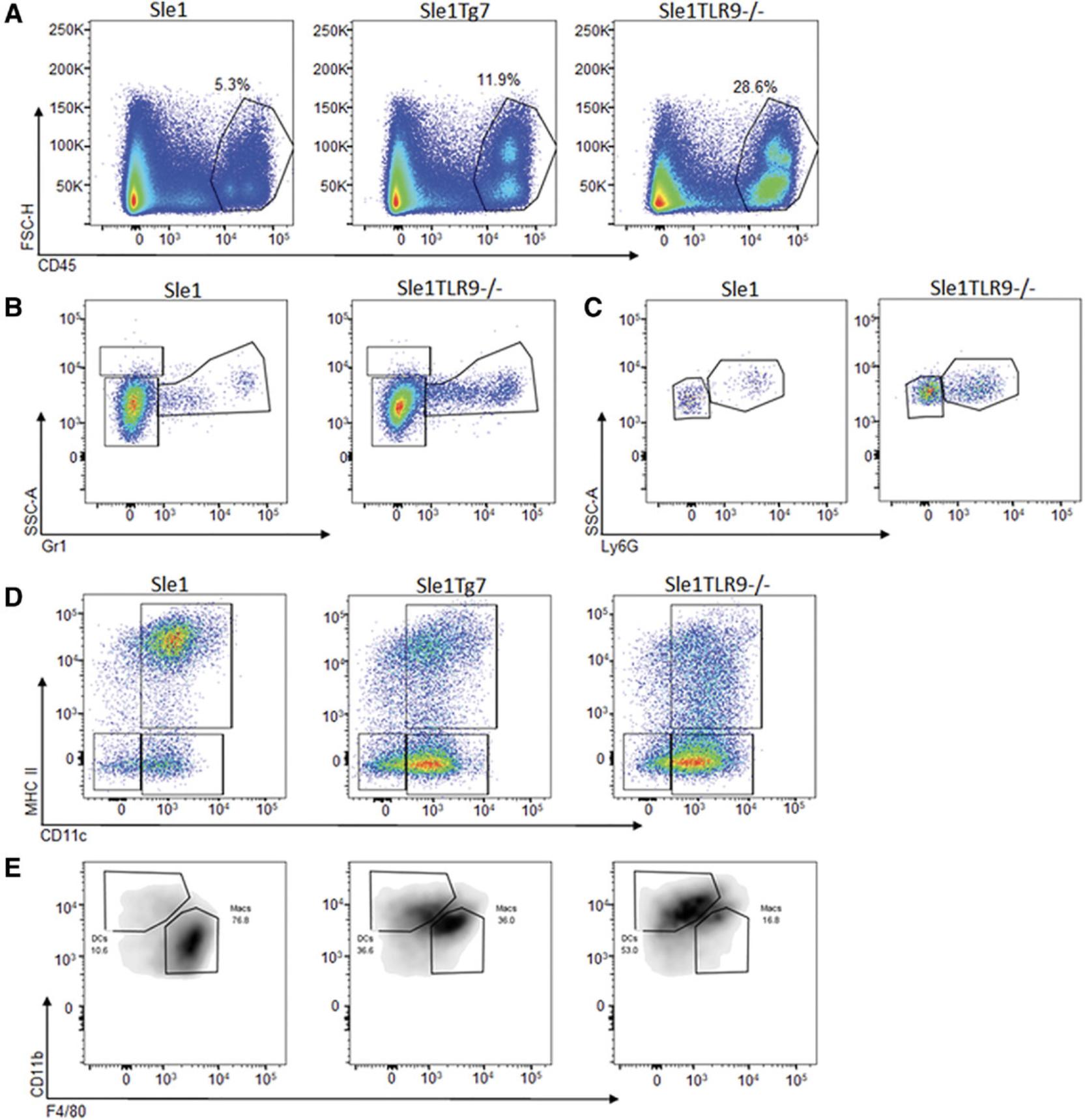
Support Protocol: TISSUE PROCESSING FOR COLD STORAGE
The protocol described below details the methods required for freezing and long-term storage of serum and tissue.
Materials
-
Mouse peripheral blood acquired in Basic Protocol 1, steps 7-10
-
Tissue: One kidney obtained in Basic Protocol 1, steps 12-15
-
Formalin solution, neutral-buffered, 10% (Sigma-Aldrich, cat. no. HT5012)
-
Tissue-Tek® O.C.T. Compound, Sakura Finetek (EMS, cat. no. 62550-12)
-
1.7-ml microcentrifuge tubes
-
Refrigerated microcentrifuge
-
0.6-ml microcentrifuge tubes, two per mouse (Axygen, cat. no. MCT-060-C-S)
-
200-µl pipettor with tips (Rainin, cat. no. 30389299)
-
Sharp blade and curved surgical tweezers
-
15-ml conical tubes (Falcon, cat. no. 352070)
-
Tissue-Tek® Cryomold®, Sakura Finetek Intermediate 15 × 15 × 5 mm (EMS, cat. no. 62534-15)
Serum/plasma processing for long-term storage
1.After a minimum of 2 hr post-collection on ice, transfer the blood from the Microvette tube obtained in Basic Protocol 1, step 7-10, to a 1.7-ml microcentrifuge tube.
2.Microcentrifuge the tube 5 min at 10,319 × g , 4°C.
3.Aliquot serum into two separate 0.6-ml microcentrifuge tubes and store at −80°C until use.
Tissue processing for histopathology and immunohistochemistry
4.Extract a fresh kidney from a mouse according to Basic Protocol 1 step 12-15.
5.Remove the renal capsule (outer membrane) from the remaining kidney not used for single-cell analysis.
6.Cut in half in a sagittal section using a sharp blade and curved surgical tweezers.
7.Using the tweezers, place in a 15-ml conical tube containing formalin. Leave at room temperature until it is transferred to a pathology lab.
8.Place the remaining half of the kidney in an intermediate cryomold half full with O.C.T compound. Cover to the top of the cryomold with O.C.T., and place on dry ice. Wrap with aluminum foil and place in a storage box before maintaining samples at −80°C.
9.Samples can be used to verify the findings of flow cytometry or assess spatial orientation of leukocytes with regard to tissue structure. In addition, immune complex deposition can also be assessed.
Basic Protocol 4: ANALYSIS OF SERUM AUTO-ANTIBODIES BY ELISAs (ANAs, snRNP, and dsDNA)
ANAs in mice that develop severe disease (Sle123 , S le1 Tg7, Sle1 TLR9-/-) are usually identified in 10%-30% mice at around 12 weeks, with 90% penetrance at around 6 months of age (Celhar et al., 2018; Fairhurst et al., 2008; Hwang et al., 2012). In general, male mice develop these phenotypes later, due to the female bias of the Sle1 susceptibility region, with the obvious exception of Sle1Yaa males (Hwang et al., 2012). Due to the variability of serum ANAs, it is recommended that at least 8 mice per group across 2 cohorts be assessed for these assays. It is recommended that the user includes negative controls (sera from non-autoimmune mice, such as B6) and positive controls (serum from aged lupus mice which develop severe disease).
Materials
-
Methylated albumin from bovine serum (mBSA; see recipe)
-
Phosphate-buffered saline (PBS), pH 7.4 (Gibco, cat. no. 10010)
-
Calf thymus double-stranded DNA (dsDNA) (see recipe)
-
Histones (see recipe)
-
0.5, 0.06, and 0.025 M sodium bicarbonate (see recipe)
-
U1snRNP-68, U1snRNP-C, and U1snRNP-A stock solutions (see recipe)
-
Serum diluent (see recipe)
-
Blocking buffer (see recipe)
-
Secondary antibody diluent (see recipe)
-
Secondary antibody: alkaline phosphatase AffiniPure F(ab')₂ fragment goat anti-mouse IgG, F(ab')₂ fragment−specific (Jackson ImmunoResearch, cat. no. 115-056-072)
-
Tween® 20, molecular biology grade (Promega, cat. no. H5152)
-
1 M magnesium chloride (Sigma-Aldrich, cat. no. M8266);aliquot 100 µl/tube and store at 20°C
-
4-Nitrophenyl phosphate disodium salt hexahydrate (pNPP tablets; Sigma-Aldrich, cat. no. N2765)
-
96-well clear flat-bottom polystyrene high-bind microplate (Corning, cat. no. 9018)
-
Plate sealers: Nunc Sealing Tapes (ThermoFisher, cat. no. 236370)
-
1.7-ml microcentrifuge tubes (Axygen, cat. no. MCT-175-C)
-
500-ml Vacuum Filter/Storage Bottle System, 0.22 µm (Corning, cat. no. 431097)
-
Nunc™ 96-Well, Nunclon Delta-treated, U-bottom microplate (ThermoFisher, cat. no. 163320)
-
Microplate shaker
-
200-µl pipettor with tips (Rainin, cat. no. 30389299)
-
20-µl pipettor with tips (Rainin, cat. no. 30389225)
-
200-µl multichannel pipettor with tips (Rainin, cat. no. 30389299)
Day 1 (plate coating)
1.Add 60 µl of stock mBSA to 6 ml PBS. Mix well and add 50 µl/well to a 96-well flat-bottom polystyrene high-bind microplate.
2.Seal with a sealing film to prevent evaporation. Incubate for 30 min at 37°C.
3.Wash the plate twice with at least 200 µl of PBS. Flick the plate dry. For the second wash, rest the plate for 5 min before flicking off the supernatant into a sink. Tap the plate dry onto stacked paper towels.
For ANAs ELISA
4a. Add 250 µl of stock dsDNA to 4.75 ml PBS. Mix well and add 50 µl/well to the plate.
5a. Seal with plate sealer to prevent evaporation. Incubate for 30 min at 37°C.
6a. Repeat step 3.
7a. Add 50 µl stock histones to 5 ml of 0.06 M sodium bicarbonate. Mix well and add 50 µl/well into the plate.
8a. Seal with plate sealer to prevent evaporation. Incubate for 30 min at 37°C.
9a. Repeat step 3.
For dsDNA ELISA
4b. Add 250 µl of stock dsDNA at 1 mg/ml to 4.75 ml PBS. Mix well and add 50 µl/well into the plate.
5b. Seal with a plate sealer to prevent evaporation. Incubate for 30 min at 37°C.
6b. Repeat step 3.
For snRNP ELISA
4c. Add 10 µl each of stock U1snRNP-68, stock U1snRNP-C, and stock U1snRNP-A to 5 ml PBS. Mix well and add 50 µl/well into the plate.
5c. Seal with a plate sealer to prevent evaporation. Incubate for 2 hr at 37°C.
6c. Repeat step 3.
For all ELISAs
10.Add 50 µl of blocking buffer to each well.
11.Seal with plate sealer. Block overnight at 4°C.
Day 2 (Sample loading and secondary antibody incubation)
12.Dilute serum samples at 1:50 or 1:200 dilution with the serum diluent in 96-well U-bottom microplate. Ensure that the samples are well-mixed with minimum bubbles generated.
13.Wash the plate twice with at least 200 µl of PBS. Flick the plate dry. For the second wash, rest the plate for 5 min before flicking. Tap the plate onto stacked paper towels to ensure that most of the liquid is expelled from the plate.
14.Add 50 µl/well of diluted serum to the plate in triplicate.
15.Seal with a plate sealer to prevent evaporation. Incubate for 2 hr, at room temperature on a plate shaker.
16.Wash the plate four times with at least 200 µl/well of PBS. Flick the plate dry. For the last wash, rest the plate for 5 min before flicking and pat dry onto stacked paper towels.
17.Prepare secondary antibody by adding 1 µl of secondary antibody, goat anti-mouse gamma IgG-alkaline phosphatase, to 5 ml secondary antibody diluent. Mix well and add 50 µl/well to the plate.
18.Seal with plate sealer. Incubate overnight at 4°C.
Day 3 (substrate addition and absorbance reading)
19.Wash the plate twice with at least 200 µl/well of PBS with 0.05% Tween 20.Flick the plate dry. For the second wash, rest the plate for 5 min before flicking and pat dry onto stacked paper towels.
20.Wash the plate twice with at least 200 µl of PBS. Flick the plate dry. For the second wash, rest the plate for 5 min before flicking and pat dry onto stacked paper towels. Complete this step only when the substrate is ready to load.
21.Combine 5 ml of 0.025 M sodium bicarbonate (pH 9.6), 20 µl of stock 1 M magnesium chloride, and one pNPP tablet. Ensure that it is mixed well without any particulates.
22.Add 50 µl/well rapidly, using a multichannel pipettor.
23.Incubate on a shaker in the dark for at least 30 min.
24.Read the absorbance at 405 nM at 30, 60, 90 and 120 min after the addition of substrate.
ELISA analysis and expected results
When using a standard from a severe disease strain, log curves should be generated in the appropriate software to find best-fit association (Fig. 13A). Samples with values obtained in the extrapolation region above the fitted curve or line can be diluted further and re-run in additional assays. It is recommended that the lowest dilution used in the assays be 1:50, to avoid background signals, and therefore values below the fitted line or equation can be viewed as estimates. GraphPad Prism software provides a user-friendly approach to calculating ELISA values.
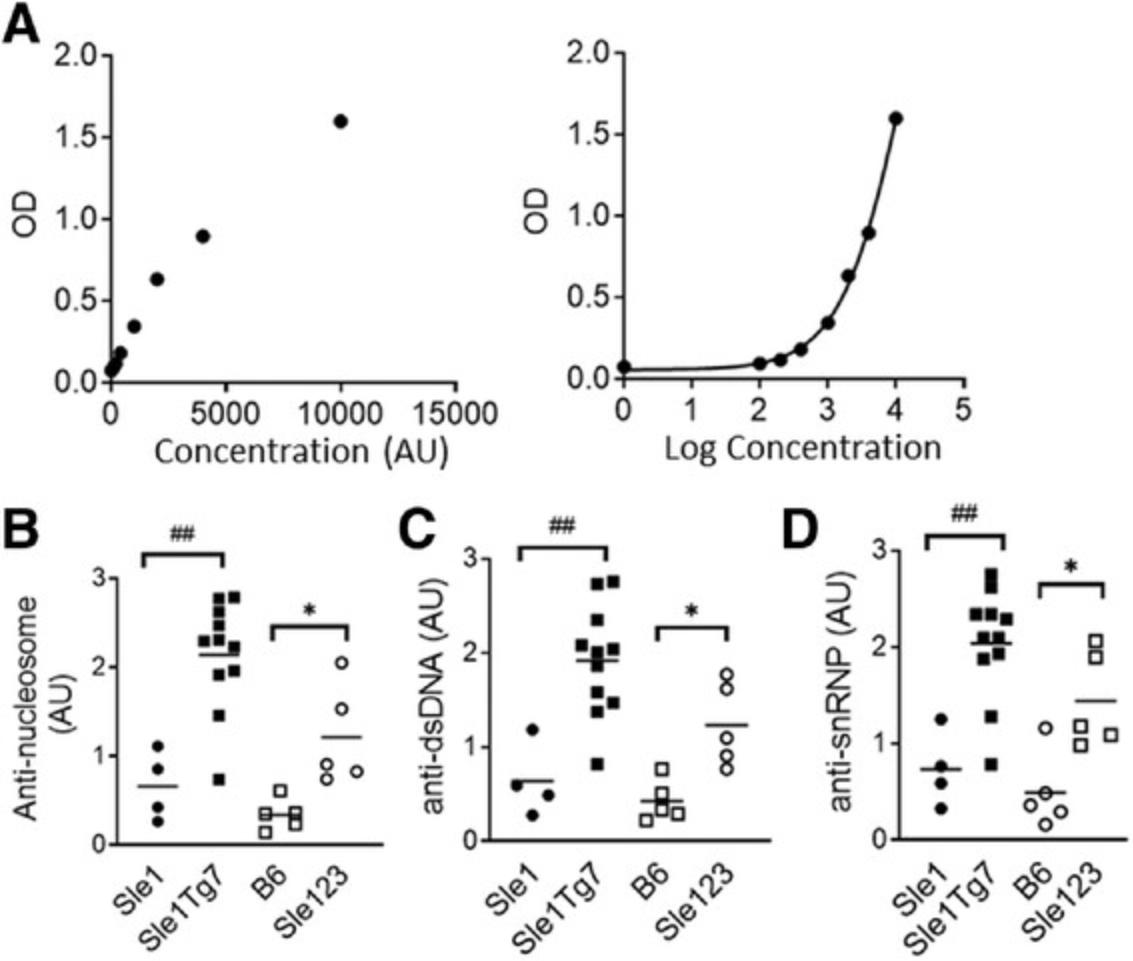
To assess positivity and penetrance of ANAs, the average (mean) of the B6 strain should be taken and added to 2 standard deviation values of the B6 strain (mean ± 2StDev). All samples above this value can then be viewed as positive. Examples of the expected results of the serum ANA are shown in Figure 13C-E.
REAGENTS AND SOLUTIONS
Albumin, methylated, from bovine serum (mBSA)
Dissolve mBSA (Sigma, cat. no. A1009) in warm 1× PBS (Gibco, cat. no. 10010) for a stock solution of 10 mg/ml. Aliquot 70 µl/tube; store at −20°C until use.
Avertin, 1.25%
Mix 0.5 ml stock Avertin (Sigma, cat. no. T48402) with 20 ml sodium chloride (NaCl) to give a 2.5% working volume. Keep at 4°C until use within 2 weeks.
Blocking buffer
Mix 3 g of BSA (Sigma-Aldrich, cat. no. A7906-100G) and 600 µl of 0.5 M EDTA (ThermoFisher, cat. no. 15575020) in 100 ml of warm 1× PBS (Gibco, cat. no. 10010). Store at 4°C until use. Store at 4°C for 1 month.
Calf thymus double-stranded DNA (dsDNA)
Dissolve calf thymus DNA (ThermoFisher, cat. no. 15633019) in warm 1× PBS (Gibco, cat. no. 10010) for a stock solution of 1 mg/ml. Aliquot into 260 µl/tube; store at −20°C until use.
Digestion buffer
- Materials :
- Collagenase Type IV (Sigma-Aldrich, cat. no. C5138)
- DNase I, >2000 U/mg, 100 mg (Roche, cat. no. 11284932001)
- 1.7-ml microcentrifuge tubes (Axygen, cat. no. MCT-175-C)
- RPMI-CM (see recipe)
- Preparation :
- Prepare DNase I stock in advance by adding 10 ml tissue-culture-grade water to 100 mg lyophilized stock. Aliquot 500 µl into microcentrifuge tubes and store at −80°C.
- Weigh out Collagenase IV for a final 1 mg/ml solution.
- Add to the appropriate volume of RPMI CM.
- Add DNase to make a final 0.05 mg/ml solution.
- Mix well and keep at 4°C until use.
- For 9 mice, prepare 150 ml: weigh out 150 mg collagenase, add 750 µl diluted DNase, and add to 149.25 ml RPMI complete medium (RPMI-CM).
FACS staining buffer
- Materials :
- Fetal bovine serum (Biowest, cat. no. S181B)
- 0.22-µm filter system, 500 ml (Corning, cat. no. 431097)
- 1 M HEPES (Gibco, cat. no. 15630080)
- Phosphate-buffered saline (PBS; Gibco, cat. no. 10010)
- Preparation :
- Add 10 ml of the heat-inactivated FBS (see recipe for RPMI CM) to a 0.22-µm, 500-ml filter system
- Add 7.5 ml of 1 M HEPES.
- Add 482.5 ml PBS, pH 7.4, and complete filtration.
Sodium azide is often added to staining buffers to prevent bacterial and fungal growth for long-term storage. However, it has been reported that it reduces sensitivity of CXCR5 staining. In addition, it is also toxic to leukocytes, and therefore its use should be avoided. We recommend preparing staining buffer the day before, or aliquotting sterile volumes for later use.
Histones
Dissolve histone (Roche, cat. no. 10223565001) in water for a stock solution of 10 mg/ml. Aliquot at 55 µl/tube, store at −20°C.
Paraformaldehyde, 1%
- Materials :
- Paraformaldehyde aqueous solution, 16% (EMS, cat. no. 15710)
- FACS staining buffer (see recipe)
- Preparation :
- Add 1 ml of 16% PFA to a 15 ml of FACS staining buffer in a 50-ml tube.
- Store at 4°C until use.
Percoll, 40%
- Materials :
- Percoll pH 8.5-9.5 (Sigma-Aldrich, cat. no. P1644)
- 10× Sterile PBS (1st Base, cat. no. BUF-2047-10 × 1 L)
- 1× Sterile PBS (Gibco, cat. no. 10010)
- 1× clean glass 150-ml bottle, or sterile bottle (150 ml; Corning, cat. no. 431175)
- All solutions are to be prepared at room temperature.
- Preparation :
- Dilute the Percoll® stock with 10× PBS in a ratio of 9 parts of Percoll® and one part of 10× PBS to create a working stock solution of Percoll.
- For 9 mice prepare 1 ml 10× PBS + 9 ml Percoll to obtain the working stock solution.
- Dilute the working Percoll stock solution to 40% with 1× PBS.
- For 9 mice prepare 100 ml of 40% Percoll, by adding 40 ml of Percoll working stock to 60 ml of 1× PBS.
- Prepare on day of use.
RPMI complete medium (RPMI-CM)
- Materials :
- RPMI 1640 Medium (Gibco, cat. no. 11875)
- Fetal bovine serum (Biowest, cat. no. S181B)
- HEPES, 1 M (ThermoFisher, cat. no. 15630080)
- Sodium pyruvate (ThermoFisher, cat. no. 11360070)
- Non-essential amino acids (NEAA) (ThermoFisher, cat. no. 11140050)
- Penicillin-Streptomycin (10,000 U/ml) (ThermoFisher, cat. no. 15140122)
- 2-Mercaptoethanol (Sigma-Aldrich, cat. no. M6250)
- 0.22-µm filter system, 500 ml (Corning, cat. no. 431097)
- 50-ml conical tubes (Falcon, cat. no. 352070)
- Preparation :
Heat inactivate the FBS at 56°C for 30 min. Allow to cool to room temperature. Store in aliquots of 50 ml at −80°C. Add 50 ml heat-inactivated FBS, 7.5 ml 1 M HEPES, 5 ml each of sodium pyruvate and NEAA, 1.2 µl 2-mercaptoethanol, and 200 ml of RPMI, and begin filtering. Add in the remaining RPMI to make 500 ml RPMI-CM.
Alternatively, take 67.5 ml of plain RPMI and add in 50 ml heat-inactivated FBS, 7.5 ml HEPES, 5 ml each of sodium pyruvate and NEAA, plus 1.2 µl 2-mercaptoethanol.
Mix well and filter through a 0.22-µm filter unit.
Secondary antibody diluent
Mix 1 g of BSA (Sigma-Aldrich, cat. no. A7906-100G) and 50 µl Tween 20 (Promega, cat. no. H5152) to 100 ml of warm 1× PBS (Gibco, cat. no. 10010). Store at 4°C for 1 month.
Serum diluent
- Add 0.25 g of gelatin (Sigma-Aldrich, cat. no. 48723) to 250 ml 1× PBS and microwave on medium power for 5 min. Cool until the solution reaches room temperature.
- Add 5 g BSA (Sigma-Aldrich, cat. no. A7906-100G) and 300 µl 0.5 M EDTA (ThermoFisher, cat. no. 15575020) and filter through a 0.22-µm, 500-ml filter system.
- Add 125 µl of Tween 20. Mix well. And store at 4°C for 1 month.
Composition: 2% BSA, 2 mM EDTA, 0.1% gelatin, 0.05% Tween 20 in 250 ml 1× PBS.
Sodium bicarbonate
- Prepare a stock solution of 0.5 M of sodium bicarbonate (Sigma-Aldrich, cat. no. S5761) by dissolving 2.1003 g of sodium bicarbonate in 50 ml MilliQ water.
- To prepare 0.06 M sodium bicarbonate, add 12 ml of 0.5 M stock sodium bicarbonate to 88 ml MilliQ water to have a final volume of 100 ml.
- To prepare 0.025 M sodium bicarbonate (pH 9.6), add 5 ml of 0.5 M stock sodium bicarbonate to 95 ml MilliQ water to have a final volume of 100 ml. Adjust to pH 9.6.
- Keep the sodium bicarbonate in room temperature.
U1snRNP-68, U1snRNP-C, U1snRNP-A stock solutions
Dissolve U1snRNP-68(DIARECT GmbH, cat. no. 13001), U1snRNP-C (DIARECT GmbH, cat. no. 13201), U1snRNP-A (DIARECT GmbH, cat. no. 13101) to give a stock solution of 1 mg/ml, respectively. Aliquot into 12 µl/tube, store at −20°C.
COMMENTARY
Background Information
Historically, the key features that were commonly evaluated for lupus disease in mice included ANAs, splenomegaly with leukocyte expansion, and glomerulonephritis (Seavey et al., 2011). However, over the last two decades, there has been a continued progression of knowledge in the mechanisms that underpin immune dysregulation. This has ensured more sophisticated assays to study immune dysregulation.
The main spontaneous murine models that are commercially available are the following:
1.the Murphy Roths Large (MRL), with a homozygous mutation for lymphoproliferation (lpr), known as the MRL-lpr. This mutation results in a loss-of-function of the Fas gene (LPR), a cell-surface protein that mediates apoptosis (Watanabe-Fukunaga, Brannan, Copeland, Jenkins, & Nagata, 1992); 2.the BXSB strain, in which males develop severe disease due to the Y-autoimmune accelerator (Yaa) locus. This region contains a cluster of 16 X-linked genes translocated onto the Y chromosome, including a dysregulated copy of toll like receptor (TLR)-7 (Pisitkun et al., 2006; Subramanian et al., 2006; 3.F1 progeny from the New Zealand Black and New Zealand White strain, known as NZB/W. Crossing and selective inbreeding of these strains also generated homozygous New Zealand mixed (NZM) strains, including NZM2410 and NZM2328 (Anderson, Cairns, Rudofsky, & Sinclair, 1995; Rudofsky & Lawrence, 1999; Waters et al., 2001).
These SLE models all develop ANAs, splenomegaly with leukocyte activation, and tissue inflammation with kidney disease; however, they differ in sex and the age of onset and severity of disease. Comparisons of these strains have been made at length (Celhar & Fairhurst, 2017; Fairhurst et al., 2006; Richard & Gilkeson, 2018). In addition, a detailed approach to evaluating novel therapeutic targets using the MRL-lpr model has been described by Seavey and colleagues (Seavey et al., 2011).
Sle1 , Sle2 , and Sle3 are the three main lupus susceptibility regions on chromosome 1, 4, and 7, respectively, that contribute to lupus pathogenesis of the NZM2410 strain (Morel et al., 1997; Morel et al., 2000). Sle1 confers a loss of tolerance to chromatin, together with B and T cell activation and mild splenomegaly (Mohan, Alas, Morel, Yang, & Wakeland, 1998). Sle2 is associated with B cell dysregulation, including B1a expansion (Mohan, Morel, Yang, & Wakeland, 1997), and Sle3 confers increased TLR hyperactivity and subsequent T cell activation (Mohan, Yu, Morel, Yang, & Wakeland, 1999). Together, these regions recapitulate the main features of disease that develop in the parental NZM2410, but with delayed onset at 6 months, and improved breeding (Fairhurst et al., 2008; Morel et al., 2000). The latter is typically challenging in homozygous lupus-prone strains in standard mouse colonies.
The Sle1 region on chromosome 1 is a common susceptibility region across several lupus-prone strains (referred to as Nba1, Sbw1, Lbw1, Cgnz1, Bxs1, and Bxs2, (Fairhurst et al., 2006). It is comparatively easier to assess specific disease mechanisms using this strain, since it can be crossed to other disease susceptibility loci and transgenic systems without a complex backcrossing strategy. This is necessary since integration of the non-autoimmune B6 genome reduces the severity of lupus disease. The combination of Sle1 with a susceptibility gene or region, including lpr, sle3, and yaa, results in a severe lupus-like disease. The yaa locus confers a 2-fold increase in TLR7 expression and function, which is necessary and sufficient for severe disease progression (Deane et al., 2007; Fairhurst et al., 2008; Santiago-Raber et al., 2008). We developed a TLR7 bacterial artificial chromosome (BAC) transgenic system (Tg7) which can be used to study this specific biology. The Sle1 Tg7 strain develops disease in an almost identical manner to Sle1 Yaa mice (Hwang et al., 2012). Importantly, in lupus model systems, TLR9, the receptor to dsDNA, is protective for severe lupus traits (Bossaller et al., 2016; Celhar et al.; 2018; Christensen et al., 2006; Jackson et al., 2014; Nickerson et al., 2010; Nickerson, Wang, Bastacky, & Shlomchik, 2017; Santiago-Raber et al., 2010; Wu & Peng, 2006; Yu et al., 2006). TLR9-deficient Sle1 mice (Sle1 TLR9-/-), develop similar phenotypes to Sle1Yaa and Sle1 Tg7 mice, including the characteristic upregulation of TLR7 expression within renal dendritic cells (Celhar et al., 2018). Sle1 is always used as a mild autoimmune phenotype control in these studies, as is B6, for non-autoimmune baseline values.
We have spent the majority of our work dissecting the mechanisms involved in the initiation and progression of disease in Sle123 mice and related B6.Sle1 strains, particularly with respect to the Toll-like receptor (TLR) family. Therefore, the protocols described have been developed with relevance to these strains. Small volumes of peripheral blood can also be taken at 10-12 weeks, and at 4 months old, to characterize differences in serum autoantibodies, myelopoiesis and T cell activation. This workflow can also be applied to other NZB/W and BXSB related strains. MRL-lpr mice develop additional key traits including severe lymphadenopathy and an expansion of a double negative CD4-CD8-CD3+B220+ T cell population, which should be considered when evaluating this strain (Zhou, Bluethmann, Eldridge, Berry, & Mountz, 1993).
Additional assays
Other relevant assays that are not described in this protocol include commercial kits and assays.
1.ANA confirmation
Additional sera analyses include Hep2 and dsDNA Crithidia luciliae substrate slides (such as NOVA Lite from Inova Diagnostics) and C3 ELISA kits which are commercially available with full instructions. The Hep2 assay should be completed at the same dilution as the ELISAs indicated above (1:200). A goat-anti-mouse IgG-secondary antibody should be used in place of the anti-human IgG secondary antibody that is provided in the kit (goat-anti-mouse IgG DyLight®488, Abcam. cat. no. Ab96879). Examples of staining patterns are provided in the kit.
2.Serum Immunoglobulin
Total immunoglobulin levels can be assessed with ELISAs or Luminex. We have quantified IgA, IgM, IgG1, IgG2a/c, IgG2b, and IgG3 antibody subsets and subtypes using Luminex [Mouse Immunoglobulin Isotyping Magnetic Bead Panel, Merck Millipore (Celhar et al., 2018)].
3.Serum measures of kidney disease
Blood Urea Nitrogen (BUN) should be assessed using colorimetric tests such as the QuantiChrom Urea Assay Kit (BioAssay Systems, DIUR-500). Normalization to serum albumin can be calculated using a Mouse Albumin ELISA Kit (Abcam, ab108792).
Kidney pathology
It is recommended to outsource the analysis of pathology of the kidney to a clinical lab to ensure expertise and relevance to clinical disease. In addition, samples should remain blinded, to provide independent assessment of disease. Others have described these protocols previously (Seavey et al., 2011; Stevens & Oltean, 2018; Xie, Zhou, Liu, & Mohan, 2003).
Critical Parameters and Troubleshooting
The most common problems we have observed when assessing the immunological traits in the tissues are listed in Table 7 along with possible solutions.
Understanding Results
There is a female gender bias in the susceptibility and severity of lupus-prone mice that lack the BXSB-derived Yaa SLE susceptibility locus. Splenomegaly, although not unique to lupus-prone models, is a key indicator of disease. An example showing the differences at 6 months between the mildly lupus-prone Sle1 strain and the Sle1 TLR9-/- strain, which develops aggressive autoimmunity, is shown in Figure 2. Typically, mice are euthanized when there is approximately 50% mortality, which is at 6-7 months in Sle1Yaa and Sle1 Tg7 and 8-9 months in Sle123 mice (Hwang et al., 2012; Morel et al., 2000). The Sle1 TLR9-/- model develops disease more aggressively when compared with other strains (Fig. 14A), displaying 50% mortality at 5 months (Fig. 14B).
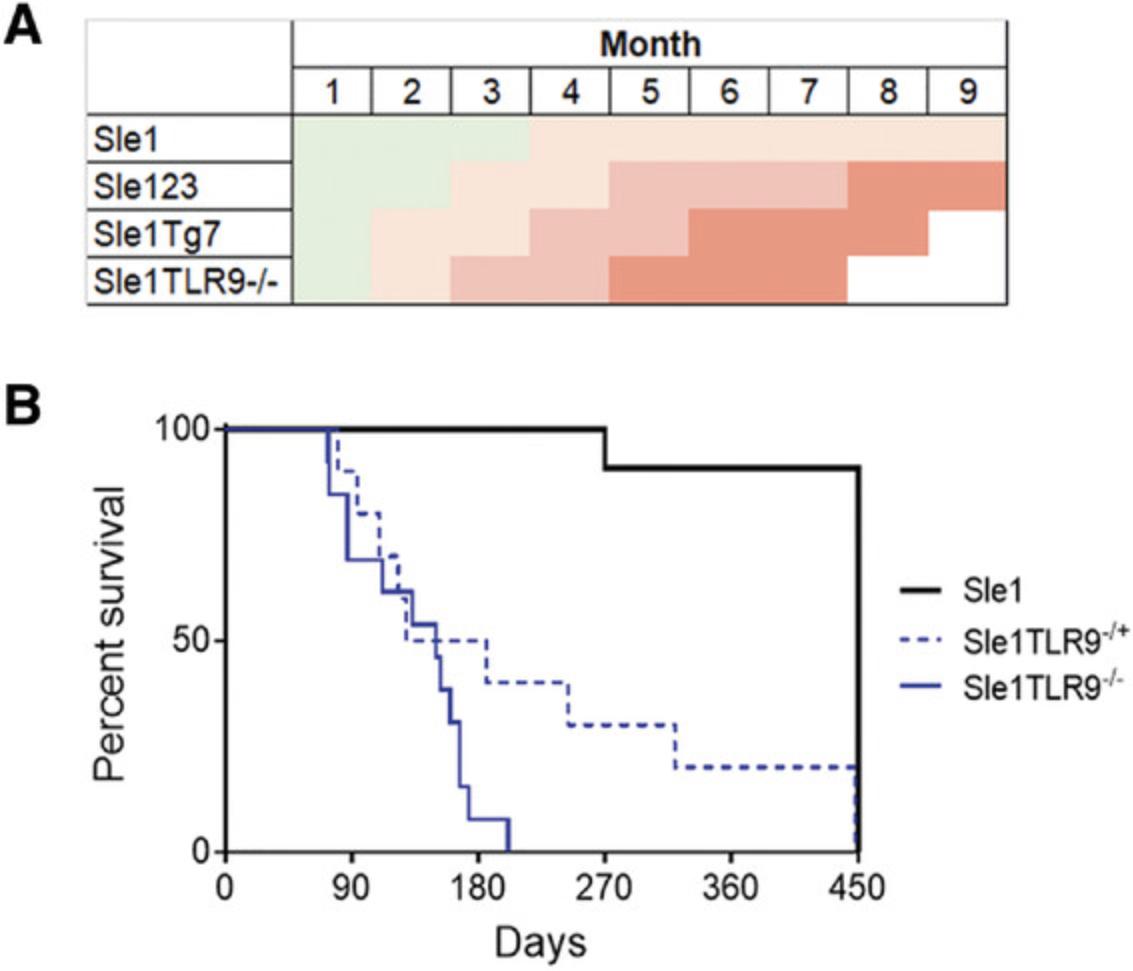
The earliest leukocyte phenotype that our lab has detected in Sle1 -associated strains with severe disease is increased T cell activation. This can be identified as early as 6-10 weeks in the peripheral blood of Sle1Yaa and female Sle1 Tg7 and Sle1T LR9-/- mice and at 12 weeks in female Sle123 mice, and remains evident at 6-9 months. There is an increase in the frequency of the effector memory CD4+CD62L-CD44high T cell population in lupus-prone mice, described below. This population also increases with age; therefore, mice should be age-matched to a maximum difference of 2 weeks in age. T cell activation can be determined using the flow cytometry assay described in Basic Protocol 3, with the T cell subset panel in Table 2. An example of the gating strategy for analysis using this panel is shown in Figure 6. Splenocytes are gated for single cells using forward scatter (FSC) parameters, followed by leukocyte identification using CD45. T cells are then identified using CD3 and the main subset markers, CD4 and CD8 (Fig. 6A).
Maturation and activation in CD4+ or CD8+ T cells are assessed using CD62L and CD44, as shown in Figure 6B, left panel, and CD4+ T follicular helper cells (Tfh) are identified using ICOS and CXCR5 (Fig. 6B, right panel). Similar changes in CD8+ T cell maturation are also measured using CD62L and CD44 (Fig. 6C). An increase in positivity and expression of CD69 and PD1 can additionally be used to measure activation. An example of the expected results of increased T cell maturation due to lupus-like disease is shown in Figure 7A. Lupus mice developing severe disease, Sle123 or Sle1 Tg7, have reduced CD4+ naïve CD62L+CD44-low T cells with a concomitant increase in the effector memory CD62L-CD44high population compared to age-matched controls (Fig. 7A and 7B).
CD4+ T follicular helper cells (Tfh) are expanded in models of SLE. A representative flow plot showing the expansion of Tfh in Sle123 mice, compared to B6 controls, is shown in Figure 7C. An example of cumulative data of splenic Tfh expansion in a single cohort of Sle123 mice compared to B6, and a combined cohort of 6-month-old Sle1 and Sle1 Tg7 mice, is shown in Figure 7D. CD4+ cells in severe disease strains exhibit an increase in ICOS and PD-1, and an example of a flow plot showing this in Sle123 mice compared to B6 nonautoimmune controls is shown in Figure 7E.
This flow cytometry panel can be used to assess T cell infiltration and activation in the kidney. An example of the gating strategy is shown in Figure 8A. CD45+ leukocytes are gated from single cells and then CD3+ cells are gated for CD4+ or CD8+ subsets (Fig. 8B and C, respectively). We have optimized our kidney protocol described in Basic Protocol 2 to characterize infiltrating leukocytes in the kidney, which precedes and correlates with the development of glomerulonephritis (Celhar et al., 2019). A bright fluorochrome for CD45 must be chosen to separate the leukocytes clearly from the high auto-fluorescent background of the kidney. An example of cumulative data showing the increase in infiltrating leukocytes in kidneys of Sle123 mice is shown in Figure 8D, and CD4+ T cell infiltration is shown in Figure 8E. The frequency of effector memory populations of T cells in the tissues are higher compared with lymphoid organs, yet an expansion is still evident in Sle123 diseased mice (Fig. 8F).
B cells are essential for the initiation and progression of disease; however, statistical changes in subsets are less evident in young mice. Clear differences between severe and mild disease are usually observed at around 6 months. An example of a panel of antibodies used to characterize key changes in B cell subsets within the spleen is shown in Table 1, with an example of the gating strategy used to characterize them in Figure 4. Splenocytes are gated for single cells, and autofluorescent cells are removed with an empty channel before selecting for CD45+ leukocytes (Fig. 4A). B220 and CD138 are then used to select plasma cells (B220-CD138+), which are expanded in most lupus strains, and plasmablasts (B220+CD138+) (Fig. 4B). B220+ cells are further gated for CD19 expression to confirm B cell identity (Fig. 4C), and GL7+Fas+ germinal center (GC) cells which are expanded in lupus mice can then be gated (Fig. 4D). Gating for the CD21+CD23- marginal zone (Mz) B cell subset is also shown in Figure 4E.
An example of expected results is shown in Figure 5. Plasma cells and plasmablasts (B220+CD138+) increase in the severe lupus-prone strains Sle123 and Sle1 Tg7, compared to age-matched respective controls, the non-autoimmune B6 mice, and the mild autoimmune Sle1 (Fig. 5A). For the strains described, the progression of disease is accompanied by a drop in the total B cell frequencies in the spleen. An example of expected cumulative data for the decreased B cells and increased plasma cells is shown in Figure 5B. The reduction in the B cell frequency within the spleen is due to the expansion of other leukocyte populations, and therefore it is recommended that the cell numbers of each leukocyte population also be calculated. Marginal B zone cell depletion is a characteristic feature of strains which develop severe lupus due to increased TLR7 activation, such as Sle1Yaa , Sle1 Tg7, or Sle1 TLR9-/-. An example of the reduction in Sle1 Tg7 mice is shown in the representative flow cytometry plot in Figure 5C, and cumulative data shown in Figure 5D. The Sle123 mice develop severe lupus due to polymorphisms in several genes, and typically do not develop the B cell marginal zone deficiency, as shown in Figure 5D.
A key feature of B cell dysregulation is the production of autoantibodies. In Basic Protocol 4 we have described methods to assess ANAs (chromatin and dsDNA), as well as anti-dsDNA and anti-snRNP antibodies. Examples of a typical analysis are displayed in Figure 13A. In these investigations, the relative differences in ANAs between samples are measured at the same dilution. A positive serum control (pooled sera from aged lupus mice with severe disease) is used as a reference to construct the standard curve–then the ANA values will represent “dilution equivalents” to the positive sera. Alternatively, the user could report the optical density (OD) for samples being compared on the same plate. If there are more samples across multiple plates, an internal control should be loaded on each plate for normalization purposes.
Detectable ANAs appear at approximately 3 months of age in the strains described here, with 90%-100% penetrance in severe lupus-prone strains at 6 months of age. Expected results from 6-month-old Sle1 Tg7 mice and Sle1 controls, and 5-month Sle123 mice, with B6 controls, are shown in Figure 13B-D. For comparisons in female mice with mild versus severe disease, a 6-month time point is preferable. Mildly autoimmune-prone mice have reduced penetrance (around 50%) and lower titers. For full immunological changes in mild disease in females, or male mice (not Yaa related), mice should be aged around 9-10 months old.
Many lupus-prone strains are characterized by an expansion in CD11b+ myeloid cells. This can be detected using a simple lineage panel that detects all major leukocyte lineages, or a more complex panel that assesses myeloid subsets, indicated in Table 7 and Table 3. An example of a recommended gating strategy based on the panel shown in Table 3 is shown in Figure 9. After selection for single cells, B220 and mPDCA1 allow discrimination of B cells (B220+mPDCA1-) and pDCs (B220+mPDCA-1+) in CD45+ leukocytes (Fig. 9A). The B220-mPDCA1- population can then be gated for CD11b+. Myeloid subpopulations of neutrophils, monocytes, macrophages, and DCs can be identified as shown in Figure 9B. Eosinophils have a high side-scatter (SSC) profile and have no/little Gr1 (Ly6C/G) expression. This can be confirmed by the expression of SiglecE (not shown). Gr1+ with lower SSC profiles are then gated for neutrophils using the specific marker, Ly6G, or for monocytes, which are Ly6G- and can be confirmed with CD115 (not shown). CD11b+Gr1- cells can then be gated for CD11c+MHC+ to identify DCs (Fig. 9B). Additionally, given the breadth of this panel, CD11b-CD3+ T cells and the CD4+ or CD8+ subsets can be selected to give an accurate calculation of the frequency of cell lineages in the spleen (Fig. 9C, Fig. 10A-D).
An example of the expected expansion in CD11b+ myeloid cells observed in spleens of Sle123 and Sle1 Tg7 mice is shown in Figure 10A, together with the relative changes in B cells (Fig. 10B), and CD4+ and CD8+ T cells (Fig. 10C and D, respectively). This data also demonstrates that strains with severe disease display increased variability in the data across mice (observable with the CD11b+ and B cell frequencies).
A similar gating strategy can be used to identify leukocyte subsets in the kidneys of lupus-prone mice (Fig. 11). However, the myeloid lineage and DC populations occupying the tissue are not the same as those in the lymphoid tissue, and therefore require further resolution (Celhar et al., 2019). Dead cells are removed from CD45+ leukocytes using a fluorescent viability dye (Fig. 11A), and a similar strategy to that described for splenocytes above is used to select for pDCs, B cells (Fig. 11B), and myeloid cells (Fig. 11C).
We have identified an expanding CD11b+ CD11c+MHCII+ population in the kidneys of lupus mice with severe disease (Celhar et al., 2015; Celhar et al., 2018; Celhar et al., 2019). Figure 11D shows the gating strategy used to identify this population. T cells are gated for CD4+ and CD8+ subsets, with the CD8+ population discerning T cells from CD11c+ CD8+ DCs (Fig. 11E).
In Figure 12A, an example of the flow cytometry plots shows the expanding leukocyte infiltration in the kidney in 6-month-old Sle1 Tg7 and Sle1 TLR9-/- mice compared to Sle1 controls. In Figure 12B, the expansion of the Gr1+ population results in the loss of resolution of clear populations of neutrophils and monocytes, but this can be resolved with Ly6G, as shown in Figure 12C. In Figure 12D, the expansion of the CD11c+MHCII+ population in Sle1 Tg7 and Sle1 TLR9-/- mice is evident, compared to age-matched Sle1 controls, and the majority of these are F4/80- in mice with severe disease (Fig. 12E).
In summary, the flow cytometry analyses described here will demonstrate the extent of myeloid expansion, T cell maturation, and B cell germinal center and plasma cell increases due to lupus-like disease.
Using models for the evaluation of therapeutic targets
Evaluation of therapeutic targets is often completed in MRL-lpr mice given the availability of the mice, ease of breeding, speed of disease progression, and historical data for reference. However, this is not recommended given that this model is driven by a single point mutation (lpr), which is not representative of the polygenic nature of the disease. Here, the authors recommend the addition of target evaluation in polygenic strains to examine impact on the systemic immune response. These include NZB/W, NZM2410, and Sle123 , all available commercially. The obvious caveat is the length of time these mice take to develop disease. The investigator should consider whether the aim of the novel target is to prevent progression of autoimmunity and organ damage, or reduce the severity of existing disease. In Sle123 mice, for example, a target that aims to prevent disease would be administered around 12 weeks of age, for up to 3 months. For reduction of inflammation in existing renal disease, this would start around 6 months.
Given the mainstay of steroid therapy in clinical disease, steroids are often used in mouse models as a reference point for disease reduction for the evaluation of novel targets. However, most publications do not examine immunological changes due to their historical nature. The administration of methylprednisalone results in preservation of the kidney integrity, resulting in decreased immune complex deposition in the kidney, reduced proteinuria, and increased survival in NZB/W and MRL-lpr mice ( Cavallo, Graves, & Granholm, 1983; Cavallo, Graves, & Granholm, 1984). Long-term treatment of MRL-lpr mice with prednisone (2.5 or 5 mg/kg/day for 13 weeks) decreased proteinuria, prolonged survival, and decreased serum auto-antibody levels (Yuan et al., 2012; Zhao et al., 2021). FACS analysis of splenic lymphocytes showed a decreased frequency of CD138+ plasma cells and increased levels of naïve CD62L+CD4+ T cells (Yan, Deng, Wang, Sun, & Wei, 2015).
Belimumab was the first biologic approved by the FDA for SLE in over 50 years. This is an antibody that neutralizes the B cell−activating factor (BAFF) expressed on B cells at various stages of maturation. More recently, it has been approved in combination with standard therapy for active lupus nephritis (https://www.gsk.com/en-gb/media/press-releases/fda-approves-gsk-s-benlysta-as-the-first-medicine-for-adult-patients-with-active-lupus-nephritis-in-the-us/). BAFF blockade in NZB/W and BXSB mice results in reduced glomerulonephritis, lower proteinuria, and prolonged survival (Ding et al., 2010; Ramanujam et al., 2006). Upon treatment, the spleens of NZB/W mice were smaller and contained fewer immune cells, especially B cells. Treatment caused a marked decrease of memory CD44+CD62- CD4+ T cells and prevented the accumulation of CD11c+/CD11b+ dendritic cells in the spleen (Ramanujam et al., 2006).
Similarly, BAFF-TRAP, a BAFF antagonist, reduced proteinuria, relieved glomerulonephritis, reduced autoantibody levels, and increased survival of MRL-lpr mice. The treatment decreased B cell numbers in the spleen and frequencies of dendritic cells in both spleen and kidneys (Yu et al., 2021).
In summary, these accepted therapies result in a prevention of disease and the accompanying leukocyte expansion and activation. The use of more complex flow cytometry panels for measuring leukocyte lineages and activation, such as those outlined in this article, will clarify the immune mechanisms involved and result in improved therapeutic development.
Time Considerations
Initial planning of disease assessment starts 4 months ahead of time, when the mice are 6-8 weeks old. To reduce the number of breeder cages, cohorts can be set up 3 weeks apart. It is recommended that at least 2-3 cohorts be set up to reduce cage-dependent findings. During the aging period, peripheral T cell analyses or ANAs may be assessed, as indicated above. In addition, antibody panels for immunophenotyping should be checked in optimization experiments. 1-2 weeks prior to the end of the study, the final urine assessment should be completed, the spreadsheets should be prepared for note taking, and tubes should be labeled. Buffers can be prepared up to a week before, and stored in the refrigerator. Generally, 6 mice can be processed by two individuals, from start to the end of staining, approximately 8 hr, with storage overnight, and samples run on the day after. 9-12 mice should be processed by three people for a similar time frame. We do not recommend using this protocol for more than 18 mice, with 4-5 people. The ELISAs are run at the completion of all cohorts, and take 3 days.
Acknowledgments
All data depicted in this manuscript have been obtained in experiments by the authors. The authors would like to acknowledge individuals who assisted in the optimization of protocols and experiments used to describe these protocols. Wei Yee Ong, Zi Xin Wong, Daniel Anand Silva, Hao Lu, Olga Zharkova of ASTAR, Singapore, and Sun Hee Hwang, Andrew Wang, Nana Tian, Katalin Tus and Hillery Gray of UT Southwestern Medical Center in Dallas, Texas. In addition, the support of Profs. Chandra Mohan and Ward Wakeland are gratefully acknowledged. The work described here was supported by the Institute for Molecular Biology (IMCB), and the Singapore Immunology Network (SIgN) at the Agency for Science Technology and Research (ASTAR), in Singapore.
Author Contributions
Hui Yin Lee : formal analysis, methodology, writing review and editing; Teja Celhar : formal analysis, investigation, methodology, writing review and editing; Anna-Marie Fairhurst : conceptualization, formal analysis, funding acquisition, methodology, project administration
Conflict of Interest
The authors declare no conflict of interest.
Open Research
Data Availability Statement
Data sharing not applicable: no new data were generated.
Literature Cited
- Anderson, C. C., Cairns, E., Rudofsky, U. H., & Sinclair, N. R. (1995). Defective antigen-receptor-mediated regulation of immunoglobulin production in B cells from autoimmune strains of mice. Cellular Immunology , 164(1), 141–149. doi: 10.1006/cimm.1995.1153.
- Aringer, M., Costenbader, K., Daikh, D., Brinks, R., Mosca, M., Ramsey-Goldman, R., … Johnson, S. R. (2019). 2019 European League Against Rheumatism/American College of Rheumatology Classification criteria for systemic lupus erythematosus. Arthritis & Rheumatology, 71(9), 1400–1412.
- Bossaller, L., Christ, A., Pelka, K., Nundel, K., Chiang, P. I., Pang, C., … Marshak-Rothstein, A. (2016). TLR9 deficiency leads to accelerated renal disease and myeloid lineage abnormalities in pristane-induced murine lupus. Journal of Immunology , 197(4), 1044–1053. doi: 10.4049/jimmunol.1501943.
- Cavallo, T., Graves, K., & Granholm, N. A. (1983). Murine lupus nephritis. Effects of glucocorticoid on circulating and tissue-bound immunoreactants. Laboratory Investigation; A Journal of Technical Methods and Pathology , 49(4), 476–481.
- Cavallo, T., Graves, K., & Granholm, N. A. (1984). Murine lupus nephritis. Effects of glucocorticoid on glomerular permeability. Laboratory Investigation , 50(4), 378–384.
- Celhar, T., & Fairhurst, A. M. (2017). Modelling clinical systemic lupus erythematosus: Similarities, differences and success stories. Rheumatology , 56(suppl_1), i88–i99.
- Celhar, T., Hopkins, R., Thornhill, S. I., De Magalhaes, R., Hwang, S. H., Lee, H. Y., … Fairhurst, A. M. (2015). RNA sensing by conventional dendritic cells is central to the development of lupus nephritis. Proceedings of the National Academy of Sciences of the United States of America , 112(45), E6195–6204. doi: 10.1073/pnas.1507052112.
- Celhar, T., Lu, H. K., Benso, L., Rakhilina, L., Lee, H. Y., Tripathi, S., … Fairhurst, A. M. (2019). TLR7 protein expression in mild and severe lupus-prone models is regulated in a leukocyte, genetic, and IRAK4 dependent manner. Frontiers in Immunology , 10, 1546. doi: 10.3389/fimmu.2019.01546.
- Celhar, T., Yasuga, H., Lee, H. Y., Zharkova, O., Tripathi, S., Thornhill, S. I., … Fairhurst, A. M. (2018). TLR9 deficiency breaks tolerance to RNA-associated antigens and upregulates TLR7 protein in Sle1 mice. Arthritis & Rheumatology, 70(10), 1597–1609.
- Christensen, S. R., Shupe, J., Nickerson, K., Kashgarian, M., Flavell, R. A., & Shlomchik, M. J. (2006). Toll-like receptor 7 and TLR9 dictate autoantibody specificity and have opposing inflammatory and regulatory roles in a murine model of lupus. Immunity , 25(3), 417–428. doi: 10.1016/j.immuni.2006.07.013.
- Cossarizza, A., Chang, H. D., Radbruch, A., Akdis, M., Andra, I., Annunziato, F., … Zimmermann, J. (2017). Guidelines for the use of flow cytometry and cell sorting in immunological studies. European Journal of Immunology , 47(10), 1584–1797. doi: 10.1002/eji.201646632.
- Deane, J. A., Pisitkun, P., Barrett, R. S., Feigenbaum, L., Town, T., Ward, J. M., … Bolland, S. (2007). Control of toll-like receptor 7 expression is essential to restrict autoimmunity and dendritic cell proliferation. Immunity , 27(5), 801–810. doi: 10.1016/j.immuni.2007.09.009.
- Desch, A. N., Randolph, G. J., Murphy, K., Gautier, E. L., Kedl, R. M., Lahoud, M. H., … Jakubzick, C. V. (2011). CD103+ pulmonary dendritic cells preferentially acquire and present apoptotic cell-associated antigen. Journal of Experimental Medicine , 208(9), 1789–1797. doi: 10.1084/jem.20110538.
- Ding, H., Wang, L., Wu, X., Yan, J., He, Y., Ni, B., … Zhong, X. (2010). Blockade of B-cell-activating factor suppresses lupus-like syndrome in autoimmune BXSB mice. Journal of Cellular and Molecular Medicine , 14(6B), 1717–1725. doi: 10.1111/j.1582-4934.2009.00817.x.
- Donovan, J., & Brown, P. (2001). Anesthesia. Current Protocols in Immunology , 27, 1.4.1–1.6.5. doi: 10.1002/0471142735.im0106s73
- Donovan, J., & Brown, P. (2006a). Euthanasia. Current Protocols in Immunology , 73, 1.8.1–1.8.4. doi: 10.1002/0471142735.im0106s73
- Donovan, J., & Brown, P. (2006b). Parenteral injections. Current Protocols in Immunology , 73, 1.6.1–1.6.10. doi: 10.1002/0471142735.im0106s73
- Durcan, L., & Petri, M. (2016). Immunomodulators in SLE: Clinical evidence and immunologic actions. Journal of Autoimmunity , 74, 73–84. doi: 10.1016/j.jaut.2016.06.010.
- Fairhurst, A. M., Hwang, S. H., Wang, A., Tian, X. H., Boudreaux, C., Zhou, X. J., … Wakeland, E. K. (2008). Yaa autoimmune phenotypes are conferred by overexpression of TLR7. European Journal of Immunology , 38(7), 1971–1978. doi: 10.1002/eji.200838138.
- Fairhurst, A. M., Mathian, A., Connolly, J. E., Wang, A., Gray, H. F., George, T. A., … Wakeland, E. K. (2008). Systemic IFN-alpha drives kidney nephritis in B6.Sle123 mice. European Journal of Immunology , 38(7), 1948–1960. doi: 10.1002/eji.200837925.
- Fairhurst, A. M., Wandstrat, A. E., & Wakeland, E. K. (2006). Systemic lupus erythematosus: Multiple immunological phenotypes in a complex genetic disease. Advances in Immunology , 92, 1–69. doi: 10.1016/S0065-2776(06)92001-X.
- Hwang, S. H., Lee, H., Yamamoto, M., Jones, L. A., Dayalan, J., Hopkins, R., … Fairhurst, A. M. (2012). B cell TLR7 expression drives anti-RNA autoantibody production and exacerbates disease in systemic lupus erythematosus-prone mice. Journal of Immunology , 189(12), 5786–5796. doi: 10.4049/jimmunol.1202195.
- Jackson, S. W., Scharping, N. E., Kolhatkar, N. S., Khim, S., Schwartz, M. A., Li, Q. Z., … Rawlings, D. J. (2014). Opposing impact of B cell-intrinsic TLR7 and TLR9 signals on autoantibody repertoire and systemic inflammation. Journal of Immunology , 192(10), 4525–4532. doi: 10.4049/jimmunol.1400098.
- Jakubzick, C., Helft, J., Kaplan, T. J., & Randolph, G. J. (2008). Optimization of methods to study pulmonary dendritic cell migration reveals distinct capacities of DC subsets to acquire soluble versus particulate antigen. Journal of Immunological Methods , 337(2), 121–131. doi: 10.1016/j.jim.2008.07.005.
- Kuo, C. F., Chou, I. J., Rees, F., Grainge, M. J., Lanyon, P., Davenport, G., … Doherty, M. (2018). Temporal relationships between systemic lupus erythematosus and comorbidities. Rheumatology , 58(5), 840–848.
- Kurien, B. T., Everds, N. E., & Scofield, R. H. (2004). Experimental animal urine collection: A review. Laboratory Animals , 38(4), 333–361. doi: 10.1258/0023677041958945.
- Maroz, N., & Segal, M. S. (2013). Lupus nephritis and end-stage kidney disease. American Journal of the Medical Sciences , 346(4), 319–323. doi: 10.1097/MAJ.0b013e31827f4ee3.
- McCafferty, J., Muhlbauer, M., Gharaibeh, R. Z., Arthur, J. C., Perez-Chanona, E., Sha, W., … Fodor, A. A. (2013). Stochastic changes over time and not founder effects drive cage effects in microbial community assembly in a mouse model. The ISME Journal , 7(11), 2116–2125. doi: 10.1038/ismej.2013.106.
- Mohan, C., Alas, E., Morel, L., Yang, P., & Wakeland, E. K. (1998). Genetic dissection of SLE pathogenesis. Sle1 on murine chromosome 1 leads to a selective loss of tolerance to H2A/H2B/DNA subnucleosomes. Journal of Clinical Investigation , 101(6), 1362–1372.
- Mohan, C., Morel, L., Yang, P., & Wakeland, E. K. (1997). Genetic dissection of systemic lupus erythematosus pathogenesis: Sle2 on murine chromosome 4 leads to B cell hyperactivity. Journal of Immunology , 159(1), 454–465.
- Mohan, C., Yu, Y., Morel, L., Yang, P., & Wakeland, E. K. (1999). Genetic dissection of Sle pathogenesis: Sle3 on murine chromosome 7 impacts T cell activation, differentiation, and cell death. Journal of Immunology , 162(11), 6492–6502.
- Morel, L., Croker, B. P., Blenman, K. R., Mohan, C., Huang, G., Gilkeson, G., & Wakeland, E. K. (2000). Genetic reconstitution of systemic lupus erythematosus immunopathology with polycongenic murine strains. Proceedings of the National Academy of Sciences of the United States of America , 97(12), 6670–6675. doi: 10.1073/pnas.97.12.6670.
- Morel, L., Mohan, C., Yu, Y., Croker, B. P., Tian, N., Deng, A., & Wakeland, E. K. (1997). Functional dissection of systemic lupus erythematosus using congenic mouse strains. Journal of Immunology , 158(12), 6019–6028.
- Nickerson, K. M., Christensen, S. R., Shupe, J., Kashgarian, M., Kim, D., Elkon, K., & Shlomchik, M. J. (2010). TLR9 regulates TLR7- and MyD88-dependent autoantibody production and disease in a murine model of lupus. Journal of Immunology , 184(4), 1840–1848. doi: 10.4049/jimmunol.0902592.
- Nickerson, K. M., Wang, Y., Bastacky, S., & Shlomchik, M. J. (2017). Toll-like receptor 9 suppresses lupus disease in Fas-sufficient MRL Mice. PloS One , 12(3), e0173471. doi: 10.1371/journal.pone.0173471.
- Ohl, K., & Tenbrock, K. (2015). Regulatory T cells in systemic lupus erythematosus. European Journal of Immunology , 45(2), 344–355. doi: 10.1002/eji.201344280.
- Pathak, S., & Mohan, C. (2011). Cellular and molecular pathogenesis of systemic lupus erythematosus: Lessons from animal models. Arthritis Research & Therapy, 13(5), 241.
- Phelan, K., & May, K. M. (2015). Basic techniques in mammalian cell tissue culture. Current Protocols in Cell Biology , 66, 1.1.1–1.1.22. doi: 10.1002/0471143030.cb0101s66.
- Pisitkun, P., Deane, J. A., Difilippantonio, M. J., Tarasenko, T., Satterthwaite, A. B., & Bolland, S. (2006). Autoreactive B cell responses to RNA-related antigens due to TLR7 gene duplication. Science , 312(5780), 1669–1672. doi: 10.1126/science.1124978.
- Ramanujam, M., Wang, X., Huang, W., Liu, Z., Schiffer, L., Tao, H., … Davidson, A. (2006). Similarities and differences between selective and nonselective BAFF blockade in murine SLE. Journal of Clinical Investigation , 116(3), 724–734. doi: 10.1172/JCI26385.
- Richard, M. L., & Gilkeson, G. (2018). Mouse models of lupus: What they tell us and what they don't. Lupus Science & Medicine, 5(1), e000199.
- Robertson, S. J., Lemire, P., Maughan, H., Goethel, A., Turpin, W., Bedrani, L., … Philpott, D. J. (2019). Comparison of co-housing and littermate methods for microbiota standardization in mouse models. Cell Reports , 27(6), 1910–1919.e1912. doi: 10.1016/j.celrep.2019.04.023.
- Rudofsky, U. H., & Lawrence, D. A. (1999). New Zealand mixed mice: A genetic systemic lupus erythematosus model for assessing environmental effects. Environmental Health Perspectives , 107(Suppl 5), 713–721. doi: 10.1289/ehp.99107s5713.
- Santiago-Raber, M. L., Dunand-Sauthier, I., Wu, T., Li, Q. Z., Uematsu, S., Akira, S., … Izui, S. (2010). Critical role of TLR7 in the acceleration of systemic lupus erythematosus in TLR9-deficient mice. Journal of Autoimmunity , 34(4), 339–348. doi: 10.1016/j.jaut.2009.11.001.
- Santiago-Raber, M. L., Kikuchi, S., Borel, P., Uematsu, S., Akira, S., Kotzin, B. L., & Izui, S. (2008). Evidence for genes in addition to Tlr7 in the Yaa translocation linked with acceleration of systemic lupus erythematosus. Journal of Immunology , 181(2), 1556–1562. doi: 10.4049/jimmunol.181.2.1556.
- Seavey, M. M., Lu, L. D., & Stump, K. L. (2011). Animal models of systemic lupus erythematosus (SLE) and ex vivo assay design for drug discovery. Current Protocols in Pharmacology , 53, 5.60.1–5.60.40. doi: 10.1002/0471141755.ph0560s53.
- Stevens, M., & Oltean, S. (2018). Assessment of kidney function in mouse models of glomerular disease. Journal of Visualized Experiments , (136), 57764. doi: 10.3791/57764.
- Subramanian, S., Tus, K., Li, Q. Z., Wang, A., Tian, X. H., Zhou, J., … Wakeland, E. K. (2006). A Tlr7 translocation accelerates systemic autoimmunity in murine lupus. Proceedings of the National Academy of Sciences of the United States of America , 103(26), 9970–9975. doi: 10.1073/pnas.0603912103.
- Sung, S. S., Fu, S. M., Rose, C. E. Jr., Gaskin, F., Ju, S. T., & Beaty, S. R. (2006). A major lung CD103 (alphaE)-beta7 integrin-positive epithelial dendritic cell population expressing Langerin and tight junction proteins. Journal of Immunology , 176(4), 2161–2172. doi: 10.4049/jimmunol.176.4.2161.
- Theofilopoulos, A. N., & Dixon, F. J. (1985). Murine models of systemic lupus erythematosus. Advances in Immunology , 37, 269–390. doi: 10.1016/S0065-2776(08)60342-9.
- Ubeda, C., Lipuma, L., Gobourne, A., Viale, A., Leiner, I., Equinda, M., … Pamer, E. G. (2012). Familial transmission rather than defective innate immunity shapes the distinct intestinal microbiota of TLR-deficient mice. Journal of Experimental Medicine , 209(8), 1445–1456. doi: 10.1084/jem.20120504.
- Vermaelen, K., & Pauwels, R. (2004). Accurate and simple discrimination of mouse pulmonary dendritic cell and macrophage populations by flow cytometry: Methodology and new insights. Cytometry. Part A , 61(2), 170–177. doi: 10.1002/cyto.a.20064.
- Watanabe-Fukunaga, R., Brannan, C. I., Copeland, N. G., Jenkins, N. A., & Nagata, S. (1992). Lymphoproliferation disorder in mice explained by defects in Fas antigen that mediates apoptosis. Nature , 356(6367), 314–317. doi: 10.1038/356314a0.
- Waters, S. T., Fu, S. M., Gaskin, F., Deshmukh, U. S., Sung, S. S., Kannapell, C. C., … McDuffie, M. (2001). NZM2328: A new mouse model of systemic lupus erythematosus with unique genetic susceptibility loci. Clinical Immunology , 100(3), 372–383. doi: 10.1006/clim.2001.5079.
- Weening, J. J., D'Agati, V. D., Schwartz, M. M., Seshan, S. V., Alpers, C. E., Appel, G. B., … Nagata, M. (2004). The classification of glomerulonephritis in systemic lupus erythematosus revisited. Journal of the American Society of Nephrology , 15, 241–250. doi: 10.1097/01.asn.0000108969.21691.5d.
- Wu, X., & Peng, S. L. (2006). Toll-like receptor 9 signaling protects against murine lupus. Arthritis and Rheumatism , 54(1), 336–342. doi: 10.1002/art.21553.
- Xie, C., Zhou, X. J., Liu, X., & Mohan, C. (2003). Enhanced susceptibility to end-organ disease in the lupus-facilitating NZW mouse strain. Arthritis and Rheumatism , 48(4), 1080–1092. doi: 10.1002/art.10887.
- Yan, S. X., Deng, X. M., Wang, Q. T., Sun, X. J., & Wei, W. (2015). Prednisone treatment inhibits the differentiation of B lymphocytes into plasma cells in MRL/MpSlac-lpr mice. Acta Pharmacologica Sinica , 36(11), 1367–1376. doi: 10.1038/aps.2015.76.
- Yu, C., Chen, S., Zhou, B., Zhang, H., Su, X., Luo, Y., & Yang, L. (2021). A novel BAFF antagonist, BAFF-Trap, effectively alleviates the disease progression of systemic lupus erythematosus in MRL/lpr mice. Molecular Immunology , 129, 1–11. doi: 10.1016/j.molimm.2020.11.010.
- Yu, P., Wellmann, U., Kunder, S., Quintanilla-Martinez, L., Jennen, L., Dear, N., … Wagner, H. (2006). Toll-like receptor 9-independent aggravation of glomerulonephritis in a novel model of SLE. International Immunology , 18(8), 1211–1219. doi: 10.1093/intimm/dxl067.
- Yuan, F., Nelson, R. K., Tabor, D. E., Zhang, Y., Akhter, M. P., Gould, K. A., & Wang, D. (2012). Dexamethasone prodrug treatment prevents nephritis in lupus-prone (NZB × NZW)F1 mice without causing systemic side effects. Arthritis and Rheumatism , 64(12), 4029–4039. doi: 10.1002/art.34667.
- Zhao, Z., Jia, Z., Foster, K. W., Wei, X., Qiao, F., Jiang, H., … Wang, D. (2021). Dexamethasone prodrug nanomedicine (ZSJ-0228) treatment significantly reduces lupus nephritis in mice without measurable side effects - A 5-month study. Nanomedicine , 31, 102302. doi: 10.1016/j.nano.2020.102302.
- Zharkova, O., Celhar, T., Cravens, P. D., Satterthwaite, A. B., Fairhurst, A. M., & Davis, L. S. (2017). Pathways leading to an immunological disease: Systemic lupus erythematosus. Rheumatology , 56(suppl_1), i55–i66. doi: 10.1093/rheumatology/kew427.
- Zhou, T., Bluethmann, H., Eldridge, J., Berry, K., & Mountz, J. D. (1993). Origin of CD4-CD8-B220+ T cells in MRL-lpr/lpr mice. Clues from a T cell receptor beta transgenic mouse. Journal of Immunology , 150(8 Pt 1), 3651–3667.