Antibody-Based CAR T Cells Produced by Lentiviral Transduction
Sabrina Prommersberger, Sabrina Prommersberger, Michael Hudecek, Michael Hudecek, Thomas Nerreter, Thomas Nerreter
Abstract
One promising approach to treat hematologic malignancies is the usage of patient-derived CAR T cells. There are continuous efforts to improve the function of these cells, to optimize their receptor, and to use them for the treatment of additional types of cancer and especially solid tumors. In this protocol, an easy and reliable approach for CAR T cell generation is described. T cells are first isolated from peripheral blood (here: leukoreduction system chambers) and afterwards activated for one day with anti-CD3/CD28 Dynabeads. The gene transfer is performed by lentiviral transduction and gene transfer rate can be verified by flowcytometric analysis. Six days after transduction, the stimulatory Dynabeads are removed. T cells are cultured in interleukin-2 conditioned medium for several days for expansion. There is an option to expand CAR T cells further by co-incubation with irradiated, antigen-expressing feeder cell lines. The CAR T cells are ready to use after 10 (without feeder cell expansion) to 24 days (with feeder cell expansion). © 2020 The Authors.
Basic Protocol : Generation of CAR T cells by lentiviral transduction
INTRODUCTION
During the last three decades, novel treatment options for the therapy of cancer patients have been introduced to the fields of hematology and oncology. Especially novel immunotherapies, including the use of cancer-antigen-specific monoclonal antibodies, bi-specific antibodies and checkpoint inhibitors that improve antitumor responses with less pronounced off-target effects as compared to chemotherapy, diversified the therapeutic options (June & Sadelain, 2018). One approach for the treatment of cancer patients is the redirection of autologous, patient-derived T cells toward a novel specificity by the introduction of a chimeric antigen receptor (CAR) transgene. Utilizing the binding moiety of a monoclonal antibody in a scFv format, the CAR is able to detect surface antigens without the need for a presentation via molecules of the major histocompatibility complex. A breakthrough for the field of CAR therapy was achieved in 2017, when the FDA approved two CD19-specific CAR T cell products, Tisagenleleucel and Axicabtagene Ciloeucel, for the treatment of B cell acute lymphocytic leukemia and non-Hodgkin lymphoma, or Large B cell lymphoma, respectively (Maude et al., 2018; Neelapu et al., 2017). Due to the success of CD19 CAR T cell therapy, a wide panel of cancer-associated cell surface antigens has been investigated as targets for CAR T cells in preclinical and clinical studies, including but not limited to BCMA, Mesothelin, GD2, EGFR, Her2, CD22, CD123, CD30, ROR1, ROR2, SLAMF7, or αvβ3 (Charrot & Hallam, 2019; Gogishvili et al., 2017; Hudecek et al., 2010; Jetani et al., 2018; Peng et al., 2017; Prommersberger et al., 2018).
Techniques for isolation, activation, and culture of human T cells as well as the gene transfer for expression of synthetic designer molecules are broadly available to the field, nowadays also in kit-based formats leading to reduced variation and operator-related bias. Nevertheless, we have made the experience that manufacturing of a high-quality CAR T cell product often fails due to operational factors.
Therefore, this protocol is intended to enable the user to reliably generate T cells expressing a given chimeric antigen receptor targeting a specific antigen. The workflow includes the isolation of donor-derived T cells, followed by T cell activation and lentiviral transfer of the CAR transgene.
STRATEGIC PLANNING (optional)
A mandatory prerequisite for conducting this protocol is the availability of lentiviral particles encoding for the CAR transgene. The underlying plasmid, which can be either self-assembled or purchased (e.g., Creative Biolabs) has to be packaged in viral particles (Lana & Strauss, 2020; Moco, de Abreu Neto, Fantacini, & Picanco-Castro, 2020) or the virus particles for certain CAR constructs can be purchased directly (e.g., G&P Biosciences, Creative Biolabs).
If the CAR vector is assembled on a custom basis, the user has to have the sequence of a specific antibody in scFv format and needs to consider which promotor should drive CAR transgene expression in the T cells. In addition, all non-antigen-binding parts of the CAR receptor strongly influence its function. For example, we have shown that the length and amino acid sequence of the extracellular spacer domain of the CAR play important roles in optimal antigen recognition and anti-tumor function (Hudecek et al., 2013, 2015). Moreover, choice and composition of the intracellular signaling module (e.g., CD28 or 4-1BB costimulation domains) is crucial for a pronounced antitumor function (Guedan et al., 2018). Furthermore, the user has to decide which kind of T cells or T cell subsets (e.g., CD4+ and/or CD8+, naïve vs memory T cells) should be selected for modification with the CAR transgene (Sommermeyer et al., 2016; Turtle, Hanafi, Berger, Gooley, et al., 2016; Turtle, Hanafi, Berger, Hudecek, et al., 2016).
Another important point to think of is the way if and how the CAR can be detected on the T cell surface after gene transfer. One possibility, also implemented in the CAR vector design in our lab is the use of a transduction marker like truncated EGF receptor separated from the CAR itself by a T2A ribosomal skipping sequence (Wang et al., 2011). Other options are the incorporation of a short c-myc or FLAG tag directly in the CAR molecule itself (Birkholz et al., 2009; Bridgeman et al., 2010). A third possibility is the use of specific target antigen-based detection reagents like the one that is available to detect CD19-specific CAR T cells (e.g., CD19 CAR Detection Reagent, Miltenyi Biotec).
Basic Protocol: GENERATION OF CAR T CELLS BY LENTIVIRAL TRANSDUCTION
By processing this protocol, the user will be able to generate CAR T cells functional evaluation within 7-14 days (excluding time needed for optional purification and further expansion steps).
Materials
-
Biocoll separating solution (RT, density 1.077 g/ml, isotone; Merck, cat. no. L6115)
-
Peripheral blood from leukoreduction system chamber (or other source of PBMC)
-
DPBS (Gibco, cat. no. 14190-094)
-
DPBS/EDTA (4°-8°C): DPBS (Gibco, cat. no. 14190-094) + 0.4% v/v 0.5 M EDTA (final concentration 2 mM; Gibco: cat. no. 15575-020)
-
MACS buffer (degassed, 4°-8°C): PBS (Gibco, cat. no. 14190-094) + 0.5% v/v FCS (Gibco, cat. no. 10500064) + 0.4% (v/v) 0.5 M EDTA (final concentration 2 mM; Gibco, cat. no. 15575-020)
-
Dynabeads Human T-Activator CD3/CD28 (Gibco, cat. no. 11161D)
-
Immunomagnetic cell sorting Kit (MACS Miltenyi Biotec, e.g. CD8 Microbeads, cat. no. 130 045 201 for CD8-expressing cells)
-
7-AAD Staining solution (Miltenyi Biotec cat. no. 130-111-568)
-
T cell medium (37°C): 1640 RPMI with HEPES and Glutamax (Gibco, cat. no. 72400054) + 10% (v/v) human serum (e.g., from Red Cross Blood Service) + 1% (v/v) Glutamax + 1% (v/v) Penicillin–Streptomycin (Gibco, cat. no. 15140122) + 0.1% (v/v) 2-Mercaptoethanol (final concentration 50 µM; Gibco, cat. no. 31350010)
-
Recombinant human IL-2, premium grade (Miltenyi, cat. no. 130-097-746)
-
Lentiviral vector encoding the CAR [produced on a custom basis as described e.g., in Moco et al., 2020 or commercially available, e.g., from Creative BioLabs (Lenti-CD19 CAR (scFv-BBτ, 104882)-VP, cat no. VP-CAR-LC633]
-
Polybrene (Millipore, cat. no. TR-1003-G)
-
Antibodies for QC of separation, e.g., CD3-PE (Miltenyi Biotec, REA613, cat no. 130-113-139), CD4-PE-Vio770 (Miltenyi Biotec, REA623, 130-113-227), CD8-VioBlue (Miltenyi Biotec, REA734, 130-110-683)
-
Optional : Antibodies/detection reagents to detect the CAR; please refer to the Strategic Planning section, also in biotinylated form if enrichment of CAR-positive T cell product is wanted
-
Optional for enrichment of CAR-positive T cells : Anti-Biotin MicroBeads (Miltenyi Biotec, cat no. 130-090-485
-
(Optional for expansion: target-antigen-positive feeder cell line, e.g., for CD19-specific CAR T cells: Jeko-1)
-
Trypan Blue solution 0.4% (Gibco cat. no. 15250061)
-
Leucosep tubes (Greiner, cat. no. 227290) Centrifuge
-
15- and 50-ml conical tubes
-
Neubauer improved counting chamber (Brand, cat. no. 717805)
-
Incubator (CO2, humidified)
-
Laboratory microscope (e.g., Zeiss PrimoVert, cat. no. 491206-0006-000)
-
QuadroMACS Separator (Miltenyi, cat. no. 130-090-976)
-
LS columns (Miltenyi, cat. no. 130-042-401)
-
Flow cytometer (needed for QC)
-
48-well, 24-well, 12-well, 6-well cell culture plates (tissue-culture treated)
-
Vortex mixer
-
MACS MultiStand (Miltenyi, cat. no. 130-042-303)
-
DynaMag - 15 Magnet (ThermoFisher Scientific, cat. no. 12301D)
-
25-cm2 cell culture flasks (tissue-culture treated)
-
Gamma irradiator, optional
NOTE : Size of columns (e.g., LS or MS) depends on the number of labeled cells, please refer to the specific manual of the Immunomagnetic sorting kit
Day 1, 1/3—Isolation of PBMC from peripheral blood by density gradient centrifugation
1.Prepare two Leucosep tubes by adding 15 ml Biocoll separating solution and centrifuge for 1 min at 700 × g , 20°C.
2.Distribute the content of one leukoreduction system chamber into two 50-ml conical tubes.
3.Fill each tube with room temperature DPBS to a total volume of 35 ml.
4.Carefully transfer the diluted blood cells into the Leucosep tubes.
5.Centrifuge for 15 min at 700 × g , 20°C, deceleration without break.
6.Harvest PBMC layer and transfer into two new 50-ml conical tubes.
7.Wash PBMC twice, each time with 40 ml cold PBS/EDTA (0.4 %) by centrifugation for 10 min at 370 × g , 8°C.
8.Aspirate the supernatant completely.
9.Count the cells using 0.4 % Trypan Blue solution and counting chamber and continue processing.
Day 1, 2/3—Immunomagnetic purification of T cells or T cell subsets from PBMC using Miltenyi MicroBeads, MACS columns and separators
The isolation of the desired cell population is performed according manufacturer's instruction. Keep cells and buffers at 4°C during the procedure whenever possible.
Following is an example for the positive selection of human CD8+ T cells (use CD8 MicroBeads cat. no. 130-045-201) with LS Columns (cat. no. 130-042-401):
10.Centrifuge the cell suspension for 10 min at 300 × g , 4°C.
11.Aspirate the supernatant completely.
12.Resuspend the cell pellet in 80 μl of MACS buffer per 107 total cells.
13.Add 20 μl of CD8 MicroBeads per 107 total cells.
14.Mix well by inverting the tube 5 times and incubate for 15 min at 4°−8°C.
15.Wash the cells by adding 1−2 ml of MACS buffer per 107 cells.
16.Centrifuge for 10 min at 300 × g , 4°C.
17.Aspirate the supernatant completely.
18.Resuspend up to 108 cells in 500 μl MACS buffer.
19.Place the column in the magnetic field of a suitable MACS Separator.
20.Prepare the column by rinsing with 3 ml MACS buffer.
21.Apply the cell suspension onto the column.
22.Collect the unlabeled cells that pass through and wash the column 3 times, each time with 3 ml MACS buffer.
23.Remove the column from the separator and place it on a 15-ml conical tube.
24.Pipette appropriate amount of MACS buffer onto the column. Immediately flush out magnetically labeled cells by firmly pushing the plunger (provided with the LS/MS columns) into the column.
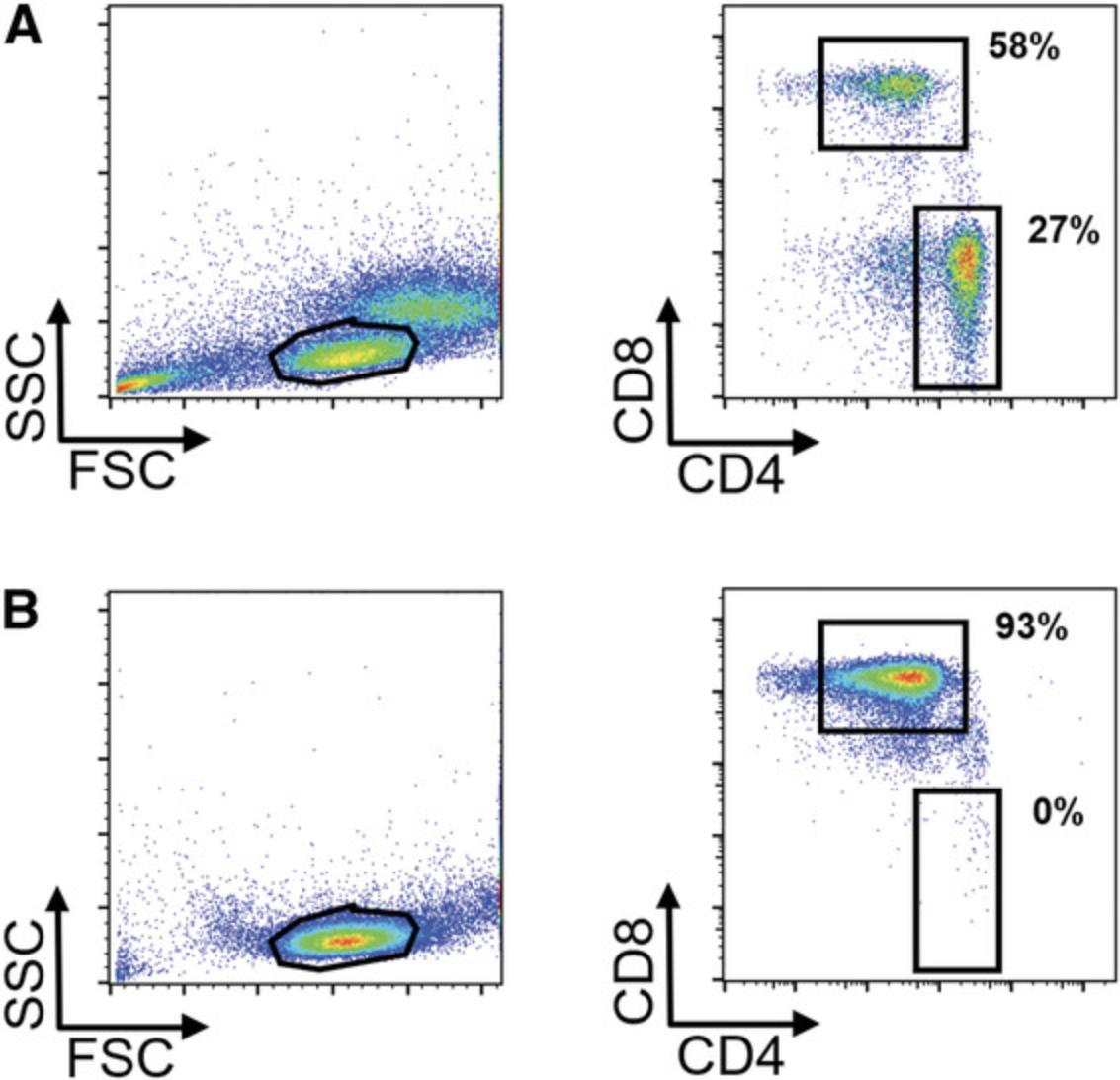
Day 1, 3/3—Activation of T cells with anti-CD3/CD28 Dynabeads
25.Count isolated T cells and prepare an appropriate amount of T cell medium.
26.Take off the amount of cells required for transduction. We use 5 × 105 cells per transduction condition and save some cells to use as an untransduced control.
27.Centrifuge for 10 min at 300 × g , 4°C.
28.Aspirate the supernatant completely.
29.Resuspend the cells in T cell medium at a concentration of 1 × 106 cells per ml.
30.Distribute 0.5 ml per well of a 48-well plate.
31.Vortex the Dynabeads and take the appropriate amount—basically use 5 × 105 beads per transduction condition (1:1 cell-to-bead ratio)
32.Wash the Dynabeads once as described in manufacturer's instruction.
33.Resuspend Dynabeads in T cell medium at a concentration of 1 × 106 beads per transduction condition.
34.Distribute Dynabeads to the T cells by adding 0.5 ml of the resuspended Dynabeads per well.
35.Add 50 IU/ml IL-2 to the T cells
36.Incubate in a humidified CO2 incubator at 37°C.
Day 2—Transduction of activated T cells
37.Thaw the lentivirus at room temperature and mix it briefly.
38.Gently remove 667 µl from each well of the 48-well plate, without touching the T cells.
39.Add 5 µg/ml polybrene per well.
40.Add lentivirus at a MOI of 3.
41.Centrifuge the plate f0r 45 min at 800 × g , 32°C, slow acceleration, no break for deceleration.
42.Incubate the cells for 4 hr in a humidified CO2 incubator at 37°C.
43.After 4 hr, add 667 µl fresh T cell medium per well.
44.Add 50 IU/ml IL-2 to each well.
Day 3 and after—Expansion of transduced T cells
Expand T cells for up to 2 weeks. Examine cultures daily, noting cell size and shape.
45.Exchange half of the medium every two days, each time also adding IL-2 to a final concentration of 50 IU/ml the T cells.
46.Count the T cells twice weekly after thorough resuspension. When the cell density exceeds 2 × 106 cells/ml, transfer the cells into a bigger vessel, diluting them to 5 × 105 cells/ml.
Day 6—Removal of Dynabeads
47.Harvest the T cells and transfer them into a 15-ml conical tube.
48.Gently resuspend the T cells in their medium within the tube to remove cells sticking to the Dynabeads.
49.Place the tube in a magnetic stand.
50.After 1 min, remove T cells suspension without touching the Dynabeads and transfer them to the culture vessel and scale up as needed.
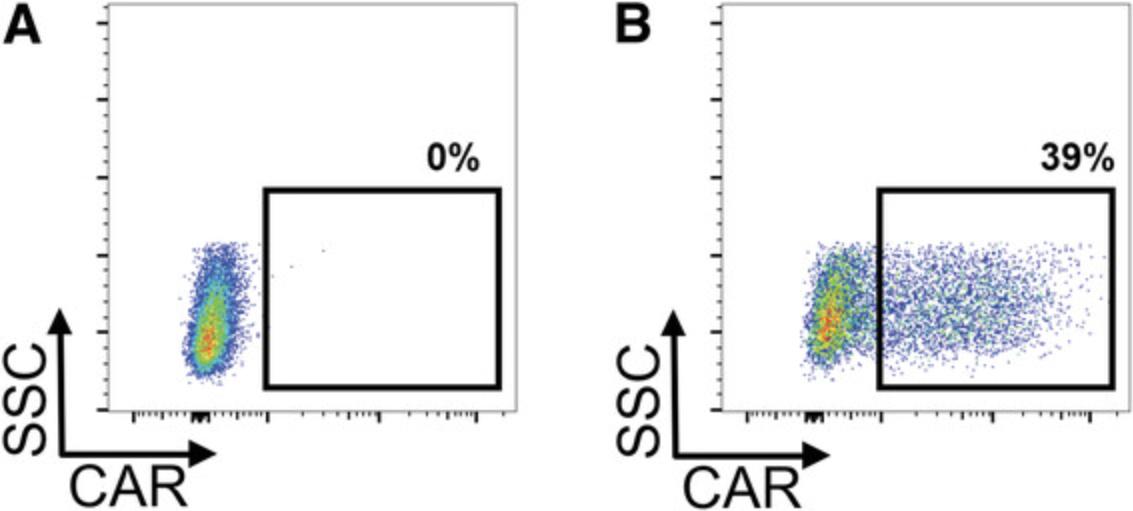
Optional steps
If the expression cassette of the CAR contains a tag (e.g., Myc-tag, FLAG-tag or truncated EGFR), CAR-expressing T cells can be enriched on day 10 or after. Therefore, transduced T cells can be labeled and sorted with a suitable tag-target reaction. The cells are first stained with a tag-specific biotinylated antibody and afterward with anti-Biotin magnetic beads. CAR T cells can be enriched by positive magnetic sorting as denoted at Day 1, 2/3.
Another optional step is the expansion of CAR T cells with feeder cells at day 10 or after. Therefore, T cells are stimulated with an irradiated, antigen-expressing target cell line for at least 10 days or longer. As an example, the CD19+ Jeko-1 cell line can be used to expand CD19-CAR T cells. Irradiation of the feeder cells is mandatory to stop prevent them from overgrowing the T cells.
51.Resuspend CAR T cells by pipetting up and down and count them.
52.Take 5 × 105 CAR T cells and centrifuge for 10 min at 300 × g , room temperature.
53.Resuspend the CAR T cells in 10 ml T cell medium and put them in a 25-cm2 flask.
54.Harvest feeder cells and count them.
55.Take 1 × 107 feeder cells and centrifuge for 10 min at 300 × g , room temperature.
56.Resuspend feeder cells in T cell medium.
57.Irradiate feeder cell line to 80 Gy.
58.Resuspend the feeder cells by pipetting up and down and count them.
59.Take 3.5 × 106 feeder cells and centrifuge for 10 min at 300 × g , room temperature.
60.Resuspend the pellet in 10 ml T cell medium and add them to CAR T cells.
COMMENTARY
Background Information
In the last decade, CAR T cells have revolutionized the field of hematologic malignancies and first CAR T cell products have now been approved by FDA and EMA. As research in this field constantly progresses, the approaches used for CAR T cell generation are continually changing. The protocol which is finally chosen for CAR T cell production depends on different factors, such as the type of CAR, CAR T cell usage after production (pre-clinical or clinical), and the used T cell subset. The present protocol has been tested on a variety of different types of T cells, including CD3+ bulk T cells, CD4+ and CD8+ T cells (as described above), as well as subsets of these, comprising Effector or Central Memory, Naïve, as well as Stem Memory T cells (TSCM), for which only different isolation kits have to be used in protocol step Day 1, 2/3 while the rest of protocols can be performed unchanged. There are various possibilities, how to activate T cells, how to perform the gene transfer, or how to expand the T cells. This protocol was chosen as it describes an easy, robust, and widely applicable way to generate CAR T cells.
Critical Parameters
CAR T cells have to be considered as “living drug.” Therefore, their production, expansion, and also their functionality, do not only depend on the protocol that is used for manufacturing. There might also be some donor-dependent variation, which might have influence on T cell activation, viability, or state of T cell differentiation. Especially when using patient-derived blood as starting material, the underlying disease and medical treatment might influence CAR T cell expansion and function.
Troubleshooting
Low transduction efficiency
The achieved transduction rate depends on different factors: The state of T cell activation at the time point of transduction and the quality of the virus batch might be the most critical factors. There is also some variance when performing T cell transductions on different donors. If transduction rates stay constantly low, T cell activation at the time point of viral transduction should be verified (e.g., by FACS staining for CD25, CD69). In addition, higher MOIs can be applied for transducing the T cells (up to a MOI of 10).
Low T cell numbers
If T cell numbers are already low at the beginning of the culture, something might have gone wrong during the T cell sort. Therefore, it is helpful to check the composition of PBMC before magnetic T cell sorting and to calculate how many T cells can be expected after sorting. Stick to the protocol that is provided by the vendor (Miltenyi) and monitor the temperature of the reagents and cells.
It also has to be considered that some cells might die during lentiviral transduction, especially if MOIs are increased.
If T cells are not satisfyingly expanding after successful gene transfer, this could be due to a low degree of stimulation. Check if the amount of Dynabeads was calculated correctly. As Interleukin-2 has only a relatively short shelf life when not being frozen, we recommend to freeze small working aliquots to avoid repeated freeze-thaw cycles and long-term storage in a non-frozen state. In some donors, earlier removal of CD3/CD28 Dynabeads leads to higher cell counts.
Understanding Results
When the respective steps are conducted properly, the user can expect to get a >90% pure T cell population after magnetic T cell isolation (exemplary data shown in Fig. 1). Typical ranges for transgene-positive T cells 7 days after lentiviral transduction at an MOI of 3 are 40%-80% for CD4+ T cells and 20%-50% for CD8+ T cells, which prove to be more resistant to gene transfer (exemplary data shown in Fig. 2).
Time Considerations
The whole process of CAR T cells generation, including T cell isolation, stimulation, lentiviral transduction,, and expansion, takes approximately 10-14 days, depending on the expansion kinetics of the T cells that are donor-dependent. If the derived CAR T cells should undergo a second round of expansion to increase the number of cells, an additional 10-14 days have to be added.
Acknowledgments
We would like to thank Miriam Alb, PhD, for critical reading of the manuscript. M. H. is a member of the Young Scholar Program (Junges Kolleg) and Extraordinary Member of the Bavarian Academy of Sciences (Bayerische Akademie der Wissenschaften). This work was supported by a grant from German Cancer Aid (Deutsche Krebshilfe e. V., Max Eder Program, grant no. 70110313), the Deutsche Forschungsgemeinschaft (project number 324392634, TRR 221), and the European Union (Horizon 2020 research and innovation programme, grant agreement No 733297 - EURE-CART and No 754658 - CARAMBA).
Literature Cited
- Birkholz, K., Hombach, A., Krug, C., Reuter, S., Kershaw, M., Kampgen, E., … Dorrie, J. (2009). Transfer of mRNA encoding recombinant immunoreceptors reprograms CD4+ and CD8+ T cells for use in the adoptive immunotherapy of cancer. Gene Therapy , 16(5), 596–604. doi: 10.1038/gt.2008.189.
- Bridgeman, J. S., Hawkins, R. E., Bagley, S., Blaylock, M., Holland, M., & Gilham, D. E. (2010). The optimal antigen response of chimeric antigen receptors harboring the CD3zeta transmembrane domain is dependent upon incorporation of the receptor into the endogenous TCR/CD3 complex. Journal of Immunology , 184(12), 6938–6949. doi: 10.4049/jimmunol.0901766.
- Charrot, S., & Hallam, S. (2019). CAR-T Cells: Future perspectives. HemaSphere , 3(2), e188. doi: 10.1097/hs9.0000000000000188.
- Gogishvili, T., Danhof, S., Prommersberger, S., Rydzek, J., Schreder, M., Brede, C., … Hudecek, M. (2017). SLAMF7-CAR T cells eliminate myeloma and confer selective fratricide of SLAMF7(+) normal lymphocytes. Blood , 130(26), 2838–2847. doi: 10.1182/blood-2017-04-778423.
- Guedan, S., Posey, A. D., Jr., Shaw, C., Wing, A., Da, T., Patel, P. R., … June, C. H. (2018). Enhancing CAR T cell persistence through ICOS and 4-1BB costimulation. JCI Insight , 3(1), e96976. doi: 10.1172/jci.insight.96976.
- Hudecek, M., Lupo-Stanghellini, M. T., Kosasih, P. L., Sommermeyer, D., Jensen, M. C., Rader, C., & Riddell, S. R. (2013). Receptor affinity and extracellular domain modifications affect tumor recognition by ROR1-specific chimeric antigen receptor T cells. Clinical Cancer Research , 19(12), 3153–3164. doi: 10.1158/1078-0432.CCR-13-0330.
- Hudecek, M., Schmitt, T. M., Baskar, S., Lupo-Stanghellini, M. T., Nishida, T., Yamamoto, T. N., … Riddell, S. R. (2010). The B-cell tumor-associated antigen ROR1 can be targeted with T cells modified to express a ROR1-specific chimeric antigen receptor. Blood , 116(22), 4532–4541. doi: 10.1182/blood-2010-05-283309.
- Hudecek, M., Sommermeyer, D., Kosasih, P. L., Silva-Benedict, A., Liu, L., Rader, C., … Riddell, S. R. (2015). The nonsignaling extracellular spacer domain of chimeric antigen receptors is decisive for in vivo antitumor activity. Cancer Immunology Research , 3(2), 125–135. doi: 10.1158/2326-6066.CIR-14-0127.
- Jetani, H., Garcia-Cadenas, I., Nerreter, T., Thomas, S., Rydzek, J., Meijide, J. B., … Hudecek, M. (2018). CAR T-cells targeting FLT3 have potent activity against FLT3(-)ITD(+) AML and act synergistically with the FLT3-inhibitor crenolanib. Leukemia , 32(5), 1168–1179. doi: 10.1038/s41375-018-0009-0.
- June, C. H., & Sadelain, M. (2018). Chimeric Antigen Receptor Therapy. New England Journal of Medicine , 379(1), 64–73. doi: 10.1056/NEJMra1706169.
- Lana, M. G., & Strauss, B. E. (2020). Production of lentivirus for the establishment of CAR-T cells. Methods in Molecular Biology , 2086, 61–67. doi: 10.1007/978-1-0716-0146-4_4.
- Maude, S. L., Laetsch, T. W., Buechner, J., Rives, S., Boyer, M., Bittencourt, H., … Grupp, S. A. (2018). Tisagenlecleucel in children and young adults with B-cell lymphoblastic leukemia. The New England Journal of Medicine , 378(5), 439–448. doi: 10.1056/NEJMoa1709866.
- Moco, P. D., de Abreu Neto, M. S., Fantacini, D. M. C., & Picanco-Castro, V. (2020). Optimized production of lentiviral vectors for CAR-T cell. Methods in Molecular Biology , 2086, 69–76. doi: 10.1007/978-1-0716-0146-4_5.
- Neelapu, S. S., Locke, F. L., Bartlett, N. L., Lekakis, L. J., Miklos, D. B., Jacobson, C. A., … Go, W. Y. (2017). Axicabtagene ciloleucel CAR T-cell therapy in refractory large B-cell lymphoma. The New England Journal of Medicine , 377(26), 2531–2544. doi: 10.1056/NEJMoa1707447.
- Peng, H., Nerreter, T., Chang, J., Qi, J., Li, X., Karunadharma, P., … Rader, C. (2017). Mining Naive Rabbit antibody repertoires by Phage display for monoclonal antibodies of therapeutic utility. Journal of Molecular Biology , 429(19), 2954–2973. doi: 10.1016/j.jmb.2017.08.003.
- Prommersberger, S., Jetani, H., Danhof, S., Monjezi, R., Nerreter, T., Beckmann, J., … Hudecek, M. (2018). Novel targets and technologies for CAR-T cells in multiple myeloma and acute myeloid leukemia. Current Research in Translational Medicine , 66(2), 37–38. doi: 10.1016/j.retram.2018.03.006.
- Sommermeyer, D., Hudecek, M., Kosasih, P. L., Gogishvili, T., Maloney, D. G., Turtle, C. J., & Riddell, S. R. (2016). Chimeric antigen receptor-modified T cells derived from defined CD8+ and CD4+ subsets confer superior antitumor reactivity in vivo. Leukemia , 30(2), 492–500. doi: 10.1038/leu.2015.247.
- Turtle, C. J., Hanafi, L. A., Berger, C., Gooley, T. A., Cherian, S., Hudecek, M., … Maloney, D. G. (2016). CD19 CAR-T cells of defined CD4+:CD8+ composition in adult B cell ALL patients. Journal of Clinical Investigation , 126(6), 2123–2138. doi: 10.1172/JCI85309.
- Turtle, C. J., Hanafi, L. A., Berger, C., Hudecek, M., Pender, B., Robinson, E., … Maloney, D. G. (2016). Immunotherapy of non-Hodgkin's lymphoma with a defined ratio of CD8+ and CD4+ CD19-specific chimeric antigen receptor-modified T cells. Science Translational Medicine , 8(355), 355ra116. doi: 10.1126/scitranslmed.aaf8621.
- Wang, X., Chang, W. C., Wong, C. W., Colcher, D., Sherman, M., Ostberg, J. R., … Jensen, M. C. (2011). A transgene-encoded cell surface polypeptide for selection, in vivo tracking, and ablation of engineered cells. Blood , 118(5), 1255–1263. doi: 10.1182/blood-2011-02-337360.
Citing Literature
Number of times cited according to CrossRef: 11
- Florian Märkl, Christoph Schultheiß, Murtaza Ali, Shih-Shih Chen, Marina Zintchenko, Lukas Egli, Juliane Mietz, Obinna Chijioke, Lisa Paschold, Sebastijan Spajic, Anne Holtermann, Janina Dörr, Sophia Stock, Andreas Zingg, Heinz Läubli, Ignazio Piseddu, David Anz, Marcus Dühren-von Minden, Tianjiao Zhang, Thomas Nerreter, Michael Hudecek, Susana Minguet, Nicholas Chiorazzi, Sebastian Kobold, Mascha Binder, Mutation-specific CAR T cells as precision therapy for IGLV3-21R110 expressing high-risk chronic lymphocytic leukemia, Nature Communications, 10.1038/s41467-024-45378-w, 15 , 1, (2024).
- Katrin Schoenfeld, Jan Habermann, Philipp Wendel, Julia Harwardt, Evelyn Ullrich, Harald Kolmar, T cell receptor-directed antibody-drug conjugates for the treatment of T cell-derived cancers, Molecular Therapy: Oncology, 10.1016/j.omton.2024.200850, 32 , 3, (200850), (2024).
- Dale J. Stibbs, Pedro Silva Couto, Yasuhiro Takeuchi, Qasim A. Rafiq, Nigel B. Jackson, Andrea C.M.E. Rayat, Continuous manufacturing of lentiviral vectors using a stable producer cell line in a fixed-bed bioreactor, Molecular Therapy - Methods & Clinical Development, 10.1016/j.omtm.2024.101209, 32 , 1, (101209), (2024).
- Mina Hosseini, Behnia Akbari, Ahmad Reza Shahverdi, Jamshid Hadjati, Mohammad Ali Faramarzi, Hamid Reza Mirzaei, Mohammad Hossein Yazdi, Generation of Murine Chimeric Antigen Receptor‐Modified T Cells for In Vivo Studies in Syngeneic Tumor Models, Current Protocols, 10.1002/cpz1.1107, 4 , 8, (2024).
- Jun Long, Yian Wang, Xianjie Jiang, Junshang Ge, Mingfen Chen, Boshu Zheng, Rong Wang, Meifeng Wang, Meifang Xu, Qi Ke, Jie Wang, Nanomaterials Boost CAR‐T Therapy for Solid Tumors, Advanced Healthcare Materials, 10.1002/adhm.202304615, 13 , 20, (2024).
- Ashanti Concepción Uscanga-Palomeque, Ana Karina Chávez-Escamilla, Cynthia Aracely Alvizo-Báez, Santiago Saavedra-Alonso, Luis Daniel Terrazas-Armendáriz, Reyes S. Tamez-Guerra, Cristina Rodríguez-Padilla, Juan Manuel Alcocer-González, CAR-T Cell Therapy: From the Shop to Cancer Therapy, International Journal of Molecular Sciences, 10.3390/ijms242115688, 24 , 21, (15688), (2023).
- Haokun Yuan, Xiaomei Wu, Hongwei Liu, Lung-Ji Chang, Lentiviral Gene Therapy of Chronic Granulomatous Disease: Functional Assessment of Universal and Tissue-Specific Promoters, Human Gene Therapy, 10.1089/hum.2022.140, 34 , 1-2, (19-29), (2023).
- Changjiang Guo, Han Chen, Jie Yu, Hui Lu, Qing Xia, Xiaojuan Li, Xiali Guo, Tong Wang, Lingtong Zhi, Zhiyuan Niu, Wuling Zhu, Engagement of an optimized lentiviral vector enhances the expression and cytotoxicity of CAR in human NK cells, Molecular Immunology, 10.1016/j.molimm.2023.01.010, 155 , (91-99), (2023).
- Nuala Trainor, Kelly A. Purpura, Kevin Middleton, Karen Fargo, Lauren Hails, Michele Vicentini-Hogan, Chase McRobie, Raelyn Daniels, Phil Densham, Paul Gardin, Michael Fouks, Hadar Brayer, Rivka Gal Malka, Anastasia Rodin, Tal Ogen, Michal J. Besser, Tim Smith, David Leonard, Adam Bryan, Automated production of gene-modified chimeric antigen receptor T cells using the Cocoon Platform, Cytotherapy, 10.1016/j.jcyt.2023.07.012, 25 , 12, (1349-1360), (2023).
- Rodrigo Vazquez-Lombardi, Johanna S. Jung, Fabrice S. Schlatter, Anna Mei, Natalia Rodrigues Mantuano, Florian Bieberich, Kai-Lin Hong, Jakub Kucharczyk, Edo Kapetanovic, Erik Aznauryan, Cédric R. Weber, Alfred Zippelius, Heinz Läubli, Sai T. Reddy, High-throughput T cell receptor engineering by functional screening identifies candidates with enhanced potency and specificity, Immunity, 10.1016/j.immuni.2022.09.004, 55 , 10, (1953-1966.e10), (2022).
- Yifei Hu, Guoshuai Cao, Xiufen Chen, Xiaodan Huang, Nicholas Asby, Nicholas Ankenbruck, Ali Rahman, Ashima Thusu, Yanran He, Peter A. Riedell, Michael R. Bishop, Hans Schreiber, Justin P. Kline, Jun Huang, Antigen multimers: Specific, sensitive, precise, and multifunctional high-avidity CAR-staining reagents, Matter, 10.1016/j.matt.2021.09.027, 4 , 12, (3917-3940), (2021).