A Protocol for Culture and Characterization of Human Induced Pluripotent Stem Cells After Induction
Wei Zheng, Wei Zheng, Yu-Shan Cheng, Yu-Shan Cheng, Miao Xu, Miao Xu, Guibin Chen, Guibin Chen, Jeanette Beers, Jeanette Beers, Catherine Z. Chen, Catherine Z. Chen, Chengyu Liu, Chengyu Liu, Jizhong Zou, Jizhong Zou
human induced pluripotent stem cells
hiPSC culture
hiPSC passaging
hiPSC characterization
hiPSC cryopreservation
teratoma
Abstract
Human induced pluripotent stem cells (hiPSCs) are characterized by unlimited self-renewal and the capability to differentiate into all three germ layers, with the potential to further differentiate into all types of cells and tissues. Human iPSCs retain all genetic information from their original donors and can be developed into disease models to study disease pathophysiology, identify disease phenotypes and biomarkers, and evaluate therapeutic efficacy and toxicity for drug development. Human iPSCs can also be used to develop cell therapies and regenerative medicine. In the last decade, the technologies for hiPSC generation and differentiation have advanced rapidly. Human iPSC culture and propagation are tedious and require careful handling. High-quality hiPSCs are necessary for downstream applications. The methods, techniques, and skills for hiPSC maintenance and characterization are very different from those for immortalized cell lines. It can be a challenge for new laboratory staff, and sometimes even for experienced staff, to properly culture and maintain the high quality of these cells. Here, we describe a comprehensive set of protocols for hiPSC propagation under chemically defined and feeder-free culture conditions. These step-by-step protocols describe in detail all the reagents and experimental procedures needed to culture hiPSCs. The protocols also describe experimental methods for hiPSC characterization, including immunofluorescence staining and flow cytometric analysis with a panel of pluripotency markers, a teratoma formation assay for validation of in vivo pluripotency, and detection of Sendai virus to ensure elimination of the viral vectors. These protocols have been successfully used in our laboratory for hiPSC expansion and propagation, and this article provide a useful reference guide for laboratory staff to work on hiPSC culture. Published 2023. This article is a U.S. Government work and is in the public domain in the USA. Current Protocols published by Wiley Periodicals LLC.
Basic Protocol 1 : Propagation and cryopreservation of hiPSC cultures
Basic Protocol 2 : Recovery of cryopreserved hiPSCs
Basic Protocol 3 : Validation of pluripotency markers via immunocytochemical analysis
Alternate Protocol : Determination of the expression of pluripotency markers via flow cytometry analysis
Basic Protocol 4 : Assessment of pluripotency via in vivo teratoma formation assay
Basic Protocol 5 : Confirmation of Sendai viral vector clearance via RT-PCR
INTRODUCTION
Induced pluripotent stem cells (iPSCs) are generated in vitro by the reprograming of somatic cells via the ectopic expression of a defined set of factors: octamer-binding transcription factor 3/4 (Oct3/4), sex-determining region Y-box 2 (Sox2), Krüppel-like factor 4 (Klf4), and cellular myelocytomatosis (c-Myc) (OSKM; Takahashi et al., 2007; Takahashi & Yamanaka, 2006). When properly maintained in cell culture, iPSCs have capabilities for unlimited passaging in vitro and differentiation into all cell types and tissues. Earlier methods to generate human iPSCs (hiPSCs) used retroviral vectors to transfect host cells with the transcription factor genes, which integrated into the host genome. These reprogramming strategies have disadvantages, including low efficiency and safety concerns for clinical applications. To enhance safety for clinical applications, nonintegrative systems have been developed to deliver the transcription factors via plasmids, proteins, self-replicating vectors, or RNAs. Through these approaches, a variety of somatic cells have been successfully reprogrammed into hiPSCs with improved efficiency. The generation of hiPSCs from somatic cells has opened exciting opportunities not only for basic research but also for therapeutics development. The reported applications of hiPSCs include in vitro disease modeling, drug screening, cell therapy, and regenerative medicine (Inoue et al., 2014; Shi et al., 2017). In particular, the ability to generate patient-specific hiPSCs that retain the patient's genetic information has offered unique opportunities to study disease pathogenesis, identify disease phenotypes and biomarker sets, discover potential drug targets, and model diseases for drug development. Furthermore, the feasibility of differentiating hiPSCs into cells of all three germ layers enables the development of tissues and organs for regenerative medicine. However, several important issues remain to be addressed before hiPSCs can be widely utilized for broad downstream applications. These include the potential for inappropriate cell expansion and maintenance of hiPSCs, resulting in poor-quality hiPSCs with inefficient and inconsistent differentiation potential and/or a predisposition to mutations/abnormalities during long-term cell culture (Inoue et al., 2014; Shi et al., 2017). The ability to obtain and maintain high-quality iPSCs is critical to ensure the success of downstream applications. It is also important to periodically monitor and examine the quality and characteristics of hiPSCs maintained in laboratories for quality control purposes.
Here, we present detailed, step-by-step protocols with specific reagent information for culturing and maintaining high-quality hiPSCs. Basic Protocol 1 outlines procedures to maintain, expand, and cryopreserve hiPSCs. Basic Protocol 2 describes a method to initiate cultures of hiPSCs from frozen stocks. Basic Protocol 3 outlines steps to examine the expression of pluripotency markers using an immunofluorescence assay. The Alternate Protocol describes the examination of the pluripotency markers using flow cytometry. Basic Protocol 4 provides experimental steps for evaluating pluripotency via in vivo teratoma formation assay. For completeness, Basic Protocol 5 provides a method to detect Sendai viral vectors in hiPSCs using a reverse transcription PCR (RT-PCR) approach to validate the clearance of the Sendai virus vectors used in the hiPSC induction.
It is worth noting that the reprogramming process and hiPSC maintenance may cause cell stress, resulting in genomic instability that may include unwanted genomic lesions and chromosomal abnormalities. A karyotyping examination should be routinely performed in the early passages (passages 7-10) and during propagation (every 10-15 passages) to ensure the genetic integrity of the hiPSCs (Fig. 1). hiPSC clones with an abnormal karyotype should be discarded. Meanwhile, as a rule of thumb for all cell cultures, cell line authentication testing (short tandem repeat [STR] profiling) and mycoplasma testing should be regularly conducted during long periods of hiPSC culturing (Fig. 1).
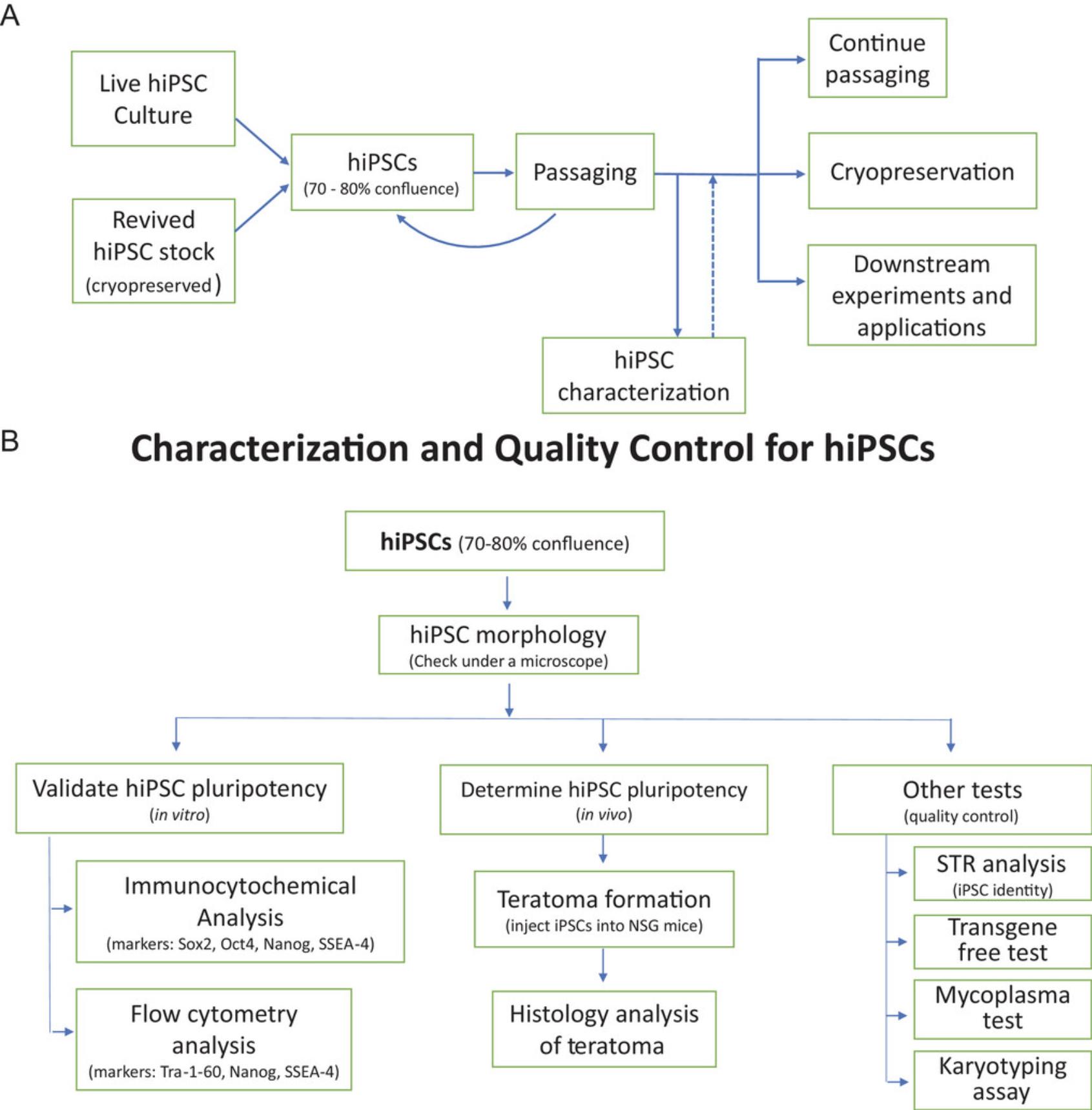
STRATEGIC PLANNING
This protocol is mainly used to culture hiPSCs for cell expansion, cryopreservation, and characterization after they are generated or obtained. Culturing hiPSCs usually takes several months and is time consuming and labor intensive. It is thus very important to start with high-quality hiPSCs. If the original hiPSCs, generated or obtained, are of poor quality, they may not grow well in culture, which wastes time and resources. Before starting the hiPSC culture, the user should have good experience or training in regular cell line culture and be proficient with aseptic technique. Because usually no antibiotics are included in hiPSC culture medium, the user's ability to maintain sterility in cell culture is a prerequisite for carrying out these protocols. In addition, hiPSCs are more fragile than immortalized cell lines and need to be handled with special care and caution. Additionally, the user should read the following protocols and plan the experiments accordingly. All reagents, media, and plasticware for hiPSC culture should be purchased in advance. Equipment and hardware for the cell culture laboratory need to be well maintained and regularly certified. In addition to following the general hiPSC culture protocols provided here, any specific information for the given hiPSC line should be read and incorporated into the protocols if needed. Because a daily medium change is usually required for most hiPSC lines, the user should plan their working schedule accordingly and be sure to change the medium daily on weekdays and at least once over the weekend. We highly recommend using a non-enzymatic method to dissociate hiPSCs from the culture vessels because hiPSCs are fragile and prone to apoptosis. We use Versene solution, a specially formulated EDTA solution, for hiPSC dissociation, as this gently dissociates cells from culture vessels without the use of enzymes. Due to the long cell culture process (at least 1-3 months), hiPSCs may undergo changes that affect their abilities to self-renew or differentiate. Therefore, it is important to characterize each hiPSC line and to validate their possession of the unique features of hiPSCs during propagation. A set of experimental methods for hiPSC characterization and quality control are also included here. For these hiPSC characterization experiments, the user should have knowledge and experience of the assays and purchase all reagents in advance. If some experiments described below cannot be done in the user's laboratory, outsourcing of these experiments to collaborators’ laboratories or commercial laboratories should be considered.
NOTE : All protocols involving animals must be reviewed and approved by the appropriate Animal Care and Use Committee and must follow regulations for the care and use of laboratory animals. Appropriate informed consent is necessary for obtaining and use of human study material.
Basic Protocol 1: PROPAGATION AND CRYOPRESEVATION OF hiPSC CULTURES
Essential 8 (E8) medium was originally developed by the laboratory of James Thomson (Chen et al., 2011) based on the mTeSR1 medium, one of the first chemically defined media for the feeder-free culture of hiPSCs. The medium contains eight essential components required to support the growth and expansion of hiPSCs and human embryonic stem cells (hESCs), providing a simpler medium for pluripotent stem cell propagation.
The propagation and culture of hiPSCs and hESCs with E8 medium requires the use of Matrigel matrix to aid cell attachment and expansion. The Matrigel matrix is a mixture of extracellular matrix proteins derived from Engelbreth-Holm-Swarm (EHS) mouse sarcoma, which includes laminin, collagen IV, heparin sulfate proteoglycans, entactin/nidogen, and several growth factors. Alternative coating matrices that have been reported to effectively support the culture of iPSCs include Geltrex, Vitronectin XF™, and CellAdhere™ Laminin-521.
Human iPSCs are more sensitive to enzymatic dissociation and prone to apoptosis and death, which often leads to poor survival during hiPSC propagation. The use of an EDTA-based enzyme-free dissociation method significantly improves cell survival and replating efficiency during hiPSC culture and propagation (Beers et al., 2012). With standard seeding density, the majority of hiPSC lines reached 70%-80% confluence within 4-5 days and can be continually passaged or frozen down (Cheng et al., 2019; Long et al., 2016). Any spontaneously differentiated cells observed under the microscope should be removed from the culture before passaging.
Cryopreservation is important to safeguard the valuable hiPSC lines. It is recommended that hiPSCs should be cryopreserved at early passages during cell propagation. A large batch of hiPSC frozen stock should be made and stored at −150°C (or liquid nitrogen) once the hiPSCs have been fully characterized. Cryopreserved hiPSCs with intermediate passage numbers are useful back-up resources for future expansion in case of unexpected cell death or contamination; in addition, cryopreserved hiPSCs in frozen in a deep freezer vials can also be immediately used in downstream applications such as RNA-seq analysis and mass-spectrum-based proteomic profiling.
This protocol describes the steps for routine culture and cryopreservation of hiPSCs that have been successfully used in our laboratory for over 100 hiPSC lines in the last 3 years. The hiPSCs are cultured and maintained in a feeder-free condition with chemically defined medium. The cells exhibit characteristics of high-quality hiPSCs based on growth rate, genetic stability, morphology, and phenotypes. They also maintain the ability to differentiate into three germ layer tissues in vivo (Chen et al., 2011).
Materials
-
Matrigel, hESC-Qualified Matrix, LDEV-free (Corning, cat. no. 354277), stored at −80°C
-
DMEM-F12 (Gibco, cat. no. 11320033)
-
Confluent hiPSCs (at 70%-80% confluence; if iPSCs are from stock vials stored in liquid nitrogen or a −150°C deep freezer, go to Basic Protocol 2)
-
Complete E8 medium (see recipe)
-
Versene solution (ThermoFisher Scientific, cat. no. 15040066), stored at 4°C
-
10 mM Y-27632 dihydrochloride (see recipe)
-
CryoStor CS10 (StemCell Technologies, cat. no. 7930), stored at 4°C
-
1.5-ml microcentrifuge tubes, 4°C
-
50-ml conical tube (Falcon, cat. no. 352098), 4°C
-
Costar 6-well clear TC-treated multiple-well plate (Corning, 3506)
-
Laminar flow hood
-
Phase-contrast microscope
-
2-ml Costar aspirating pipets (Corning, cat. no. 9186)
-
15-ml conical tubes
-
Humidified 37°C, 5% CO2 incubator
-
Cryogenic tubes (Corning, cat. no. 430488)
-
Corning CoolCell Containers (cat. no. 432000)
-
Liquid nitrogen cell storage container or −150°C deep freezer
Preparation of Matrigel coating
1.Matrigel arrives in 10-ml bottles with varying concentrations across lots. Check the protein concentration of Matrigel on the label before working with it. Matrigel coating of 6-well plates for hiPSC culture uses 8.7 µg/cm2 of Matrigel (0.1 mg/ml, 1 ml/well to coat 6-well plates). Aliquot the desired volume of Matrigel stock solution (depending on the number of plates used in a typical experiment) into microcentrifuge tubes (e.g., 250 μl/vial) and store them at −20°C.
2.On the day of coating, thaw an aliquot of Matrigel on ice. Add a small volume (300-500 μl) of ice-cold (0°C-4°C) sterile DMEM-F12 medium to dilute the Matrigel aliquots.
3.Transfer the diluted Matrigel aliquot to a prechilled 50-ml conical tube containing a volume of ice-cold DMEM-F12 to obtain a final concentration of 0.1 mg/ml as the Matrigel coating solution. The dilution volume is calculated for each lot of Matrigel based on the protein concentration.
4.Pipet the Matrigel coating solution into the wells of 6-well plates at 1 ml/well. To coat 96-well plates, add 0.1 ml/well Matrigel coating solution.
5.Incubate the 6-well plates with Matrigel coating solution at room temperature for at least 1 h. After incubation, Matrigel-coated plates can be used immediately or stored at 4°C for up to 1 week before use. For storage, seal the plate (containing the Matrigel coating solution) with Parafilm to prevent dehydration. The stored Matrigel-coated plates should be placed in the tissue culture hood prior to use to pre-equilibrate to room temperature.
Dissociation of hiPSCs for passaging
6.Once the hiPSCs reach 70%-80% confluence, examine them under a tissue culture microscope to identify any spontaneously differentiated cells (shown on Fig. 2).
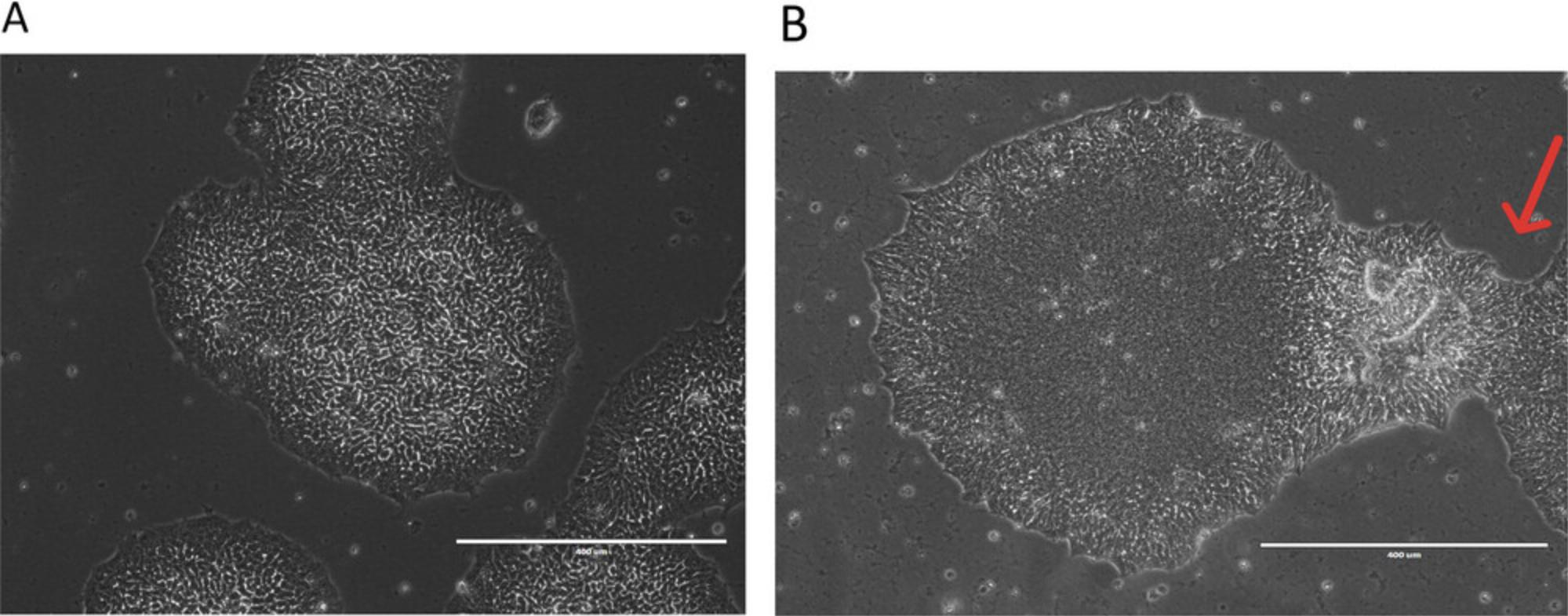
7.Remove differentiated cells by marking them under microscope and scratching them off by using a P20 pipet with pipet tips. This is especially crucial when there is an excessive number of differentiated cells.
8.Remove medium from the wells containing hiPSCs with an aspirating pipet and rinse the wells once quickly with Versene solution (1 ml/well in a 6-well plate).
9.Add Versene solution to the wells (1 ml/well in a 6-well plate). Gently swirl the plate to cover the entire well surface.
10.Incubate the plate at room temperature for 3-4 min. When cells start to separate and round up (forming cell colonies and holes when observed under the microscope), they are ready to be removed from the wells.
11.Carefully aspirate the Versene solution with an aspirating pipet.
12.Prepare an appropriate amount of complete E8 medium containing 10 µM Y-27632 hydrocholoride by adding 10 µl of 10 mM Y-27632 dihydrochloride stock per 10 ml of prewarmed (room-temperature) complete E8 medium. Gently squirt 500 μl to 1 ml/well of this into each well of the 6-well plates. Collect cell aggregates from the plates in a 15-ml conical tube as follows.
-
Cell aggregates should be appropriately sized for plating (10-30 cells per aggregate). Little or no extra pipetting is required to break up cell clumps after Versene solution treatment.
-
Work with multiple wells at a time, and work quickly to collect cells after adding E8 medium to the well(s).
13.Aspirate the Matrigel coating solution from the Matrigel-coated 6-well plates from step 5 (if removing from 4°C storage, first equilibrate the plates to room temperature for 1 hr). Immediately add 1 ml room-temperature pre-warmed complete E8 medium with 10 µM Y-27632 dihydrochloride to each 6-well plate.
14.Determine the numbers of wells and the split ratio needed to seed the cell aggregates (from step 12) at the desired density into the wells of these plates. The final volume of complete E8 medium should be 2 ml/well in 6-well plates.
15.Move the plate in several figure-eight motions (with slow and gentle movements) to evenly distribute cells across the surface of the wells.
16.Gently place the culture plate in a 37°C, 5% CO2 incubator and incubate the cells overnight.
17.The next day, replace the old medium with 2 ml/well fresh complete E8 medium without Y-27632.Continue to culture the cells, replacing the medium daily with 2 ml/well fresh complete E8 medium without Y-27632, until they reach 70%-80% confluence (usually 4-5 days).
18.The hiPSC colonies are generally ready for passaging, or for use in experiments, after 4-5 days in culture. If cryopreservation is necessary, the hiPSCs should be denser than 80% confluent for this purpose.
-
For hiPSC passage: When the cells reach 70%-80% confluence, repeat steps 6-17.
-
For hiPSC cryopreservation: When the cells reach ∼80% confluence, dissociate them with Versene solution as in steps 6-11. Aspirate the spent medium and then gently add CryoStor™CS10 cell cryopreservation medium (700 µl/well per 6-well plate). Dislodge cells by gently pipetting the medium up and down. Aliquot 350 µl/vial into separate cryogenic vials (hiPSCs from one well of a 6-well plate are stored in two vials). Place the vials in a Corning CoolCell freezing container and store at −80°C at least overnight (or 1-3 days). Then, transfer the vials from the freezing container to a liquid nitrogen tank or −150°C deep freezer for long-term storage.
-
For further experiments: hiPSCs at 70%-80% confluence can be used for experiments such as hiPSC characterization (Basic Protocols3,4, and 5) and other downstream hiPSC applications (e.g., differentiation to neurons, hepatocytes, and cardiomyocytes, and various organoids).
Basic Protocol 2: RECOVERY OF CRYPRESERVED hiPSCs
This protocol describes a centrifugation-free method to recover cryopreserved hiPSCs, which greatly improves hiPSC survival and recovery rate upon thawing. After a vial of frozen hiPSCs is thawed (from a liquid nitrogen tank or −150°C deep freezer) in a 37°C water bath, all the contents in the vial are transferred into one well of a precoated 6-well plate and incubated for 60 min in the presence of 10 μM Y-27632 dihydrochloride. After attachment of hiPSC colonies to the plate is confirmed, the medium is removed and replaced with a fresh complete E8 medium containing 10 µM Y-27632 dihydrochloride.
Materials
-
Frozen hiPSC stock
-
70% (v/v) ethanol
-
Dry ice
-
Matrigel-coated 6-well plate (prepared as described in Basic Protocol 1, steps 1-5), pre-equilibrated to room temperature for 1 hr in the hood
-
Water bath
-
DMEM-F12 (Gibco, cat. no. 11320033)
-
HEPES (Gibco, cat. no. 11330-032)
-
Complete E8 medium (see recipe)
-
Versene solution (ThermoFisher Scientific, cat. no. 15040066), stored at 4°C
-
10 mM Y-27632 dihydrochloride (see recipe)
-
CryoStor CS10 (StemCell Technologies, cat. no. 7930), stored at 4°C
-
1.5-ml microcentrifuge tubes, 4°C
-
50-ml conical tube (Falcon, cat. no. 352098)
-
Costar 6-well clear TC-treated multiple well plate (Corning, 3506)
-
Laminar-flow hood
-
Phase-contrast microscope
-
2-ml Costar aspirating pipets (Corning, cat. no. 9186)
-
15-ml conical tubes
-
Humidified 37°C, 5% CO2 incubator
-
Cryogenic tubes (Corning, cat. no. 430488)
-
Corning CoolCell Containers (cat. no. 432000)
-
Liquid nitrogen cell storage container or −150°C deep freezer
1.Remove a cryopreserved vial of hiPSCs (prepared in Basic Protocol 1, step 18, or purchased) from liquid nitrogen storage or −150°C freezer and immediately place in a 37°C water bath (keeping the vial on dry ice while transferring it from the freezer to the 37°C water bath).
2.Thaw the cells by gently swirling the vial in the 37°C water bath until only a small piece of ice remains in the vial (just before it thaws completely).
3.Transfer the vial to a laminar flow hood and wipe its surface with 70% (v/v) ethanol before opening. If the vial is labeled with a marker, record the information listed on the label before wiping because 70% ethanol may completely erase the written information.
4.Aspirate the Matrigel-containing solution from a Matrigel-coated 6-well plate (pre-equilibrated to room temperature), and immediately add 5 ml/well of room-temperature prewarmed complete E8 medium with 10 μM Y-27632 dihydrochloride (prepared immediately before use by adding 10 µl of 10 mM Y-27632 dihydrochloride stock to 10 ml complete E8 medium).
5.Transfer the thawed hiPSCs from the vial to the prepared 6-well plate dropwise, evenly distributing them into the complete E8 medium in the well.
6.Move the plate in several figure-eight motions (with slow and gentle movements) to distribute cells evenly across the surface of the bottom of 6-well plates.
7.Gently place the 6-well plate into a 37°C, 5% CO2 incubator and incubate the cells for 1 hr.
8.Examine cell attachment under a microscope to make sure most cells have attached to the well. If not, extend the incubation time for an additional 30 min (hiPSCs may have variations in attachment rates).
9.Carefully remove the supernatant (containing freezing medium) from the plate and add 5 ml/well of room-temperature prewarmed fresh complete E8 medium with 10 µM Y-27632 dihydrochloride.
10.Place the plate in the 37°C, 5% CO2 incubator and incubate the cells overnight.
11.The next day, remove the medium (containing Y-27632 dihydrochloride) and replace with 2 ml/well complete E8 medium without Y-27632 dihydrochloride. Return the plate to the 37°C, 5% CO2 incubator.
12.After the second day post thawing, remove the old medium and feed the hiPSCs with 2 ml/well fresh complete E8 medium daily. Monitor cells and assess growth until they reach 70%-80% confluence (∼2-5 days after thawing), when they are ready for splitting and passaging.
13.Continue hiPSC culture and passaging as described in Basic Protocol 1, step 6-18.
Basic Protocol 3: VALIDATION OF hiPSC PLURIPOTENCY VIA IMMUNOCYTOCHEMICAL ANALYSIS
Human iPSCs possess unique properties of self-renewal and differentiation into all of the cell types in the human body. However, spontaneous differentiation occurs frequently during hiPSC propagation and, if left unchecked, can reduce hiPSC self-renewal and/or differentiation into the intended cell types. Phenotypic characterization of hiPSCs using a panel of pluripotency markers is a standard method to efficiently qualify hiPSCs. This protocol describes how to evaluate the expression of pluripotency markers in hiPSCs using immunocytochemistry. The hiPSCs are plated, stained, and analyzed using a microscope in 96-well-plate format. Immunocytochemistry provides visualization of pluripotency markers in individual cells within a colony, allowing the subcellular localization of each marker to be assessed. This analysis should be performed after the removal of transgenes (usually after 10 passages) and before downstream applications such as differentiation into neurons or liver organoids.
Materials
-
Matrigel, hESC-Qualified Matrix, LDEV-free (Corning, cat. no. 354277), stored at −80°C
-
DMEM-F12 (Gibco, cat. no. 11320033)
-
Confluent hiPSCs in 6-well plates (from Basic Protocol 1, step 18c)
-
Complete E8 medium (see recipe)
-
Versene solution (ThermoFisher Scientific, cat. no. 15040066), stored at 4°C
-
10 mM Y-27632 dihydrochloride (see recipe)
-
32% (w/v) paraformaldehyde (Electron Microscopy Sciences, 15714-S)
-
DPBS with calcium and magnesium (ThermoFisher Scientific, 14040141)
-
Triton X-100 (Sigma-Aldrich, T8787)
-
Blocking solution (Cell Staining Buffer, BioLegend, 420201)
-
Appropriate primary and secondary antibodies
-
Hoechst 33342 (Invitrogen, cat. no. H1399)
-
1.5-ml microcentrifuge tubes, 4°C
-
50-ml conical tube (Falcon, cat. no. 352098), 4°C
-
Costar 6-well clear TC-treated multiple-well plate (Corning, 3506)
-
96-well plate (Greiner Bio-one, 655090)
-
Laminar flow hood
-
Phase-contrast microscope
-
Humidified 37°C, 5% CO2 incubator
-
2-ml Costar aspirating pipets (Corning, cat. no. 9186)
Day 1
1.Prepare a Matrigel-coated 96-well plate by adapting Basic Protocol 1, steps 1-5, adjusting the volumes by adding 100 μl/well of a diluted Matrigel coating solution consisting of 0.1 mg/ml Matrigel in DMEM-F12 medium. Incubate at room temperature for 1 hr in the hood.
2.Dissociate hiPSC colonies using Versene solution following Basic Protocol 1, steps 6-12.Combine the cells from one well of a 6-well plate with 5.5 ml complete E8 medium supplemented with 10 µM Y-27632 dihydrochloride (prepared immediately before use by adding 10 µl of 10 mM Y-27632 dihydrochloride stock to 10 ml complete E8 medium) to obtain a total volume of 6 ml of dissociated hiPSCs.
3.Remove the Matrigel coating solution from the 96-well plate (from step 1) and seed 100 µl/well of the diluted hiPSC suspension into the Matrigel-coated 96-well plate. The number of wells to be seeded with hiPSCs is dependent on the experimental needs.
4.Examine under the microscope to confirm the hiPSC seeding and incubate in a 37°C, 5% CO2 incubator overnight.
Day 2
5.Change the medium by removing the old medium and adding 100 µl/well of room-temperature prewarmed complete E8 medium without Y27632.
Day 3
6.Check the cells under the microscope to ensure that they are 30%-50% confluent. If that is the case, remove medium and fix the cells by adding 100 µl/well of 4% paraformaldehyde diluted in DPBS with calcium and magnesium. Incubate the 96-well plate for 15 min at room temperature.
7.After hiPSC fixation, wash the cells three times with 100 µl/well of DPBS with calcium and magnesium (leave the DPBS in the wells for 5 min each time), and remove all the solution from each well after cell wash.
8.Permeabilize cells by adding 100 μl/well of 0.5% Triton X-100 in DPBS with calcium and magnesium. Incubate 15 min at room temperature.
9.Aspirate the solutions and wash the cells three times with 100 µl/well of DPBS with calcium and magnesium, each for 5 min (leave the DPBS in wells for 5 min).
10.Aspirate the solution, add 100 μl/well of blocking solution, and incubate the plate for 1 hr at room temperature.
11.Aspirate the blocking solution and add a single primary antibody at 100 µl/well (diluted in blocking solution at the appropriate concentration for the antibody listed in Table 1). Use 100 µl/well of blocking solution without antibody in negative control wells. Usually, three wells are needed for each antibody dilution and three wells for the negative control in each experiment.
Usage of antibody | Antibody host species | Dilution | Company, cat. no., RRID |
---|---|---|---|
Primary antibody | Mouse anti-SOX2 | 1:50 | R&D Systems, MAB2018, AB_358009 |
Mouse anti-SSEA4 | 1:100 | Cell Signaling Technology, 4755, AB_1264259 | |
Rabbit anti-NANOG | 1:100 | Cell Signaling Technology, 4903, AB_10559205 | |
Rabbit anti-OCT4A | 1:100 | ThermoFisher Scientific, A13998, AB_2534182 | |
Secondary antibody | Donkey anti-mouse IgG (Alexa Fluor 488) | 1:400 | ThermoFisher Scientific, A21202, AB_141607 |
Donkey anti-rabbit IgG (Alexa Fluor 594) | 1:400 | ThermoFisher Scientific, A21207, AB_141637 |
-
Note: If different antibodies are used, the antibody dilutions must be tested and optimized.
12.Incubate the plate overnight at 4°C.
Day 4
13.Aspirate the solution and wash the cells three times with 100 μl/well of DPBS with calcium and magnesium, each for 5 min (leave the DPBS in wells for 5 min).
14.Aspirate the wash buffer, add secondary antibody diluted in blocking solution at the appropriate concentration (see Table 1), and incubate at room temperature in the dark for 1 hr.
15.Aspirate the solution and wash the cells three times with 100 μl/well of DPBS with calcium and magnesium, each for 5 min (leave the DPBS in wells for 5 min).
16.Add 100 μl/well of 1 µg/ml Hoechst 33342 in DPBS with calcium and magnesium and incubate for 15 min at room temperature in the dark.
17.Aspirate the solution and wash the cells three times with 100 μl/well of DPBS with calcium and magnesium, each for 5 min (leave the DPBS in wells for 5 min).
18.Add 100 µl of DPBS with calcium and magnesium to each well.
19.Image cells with the appropriate filters (10× objective, 6 images per well).
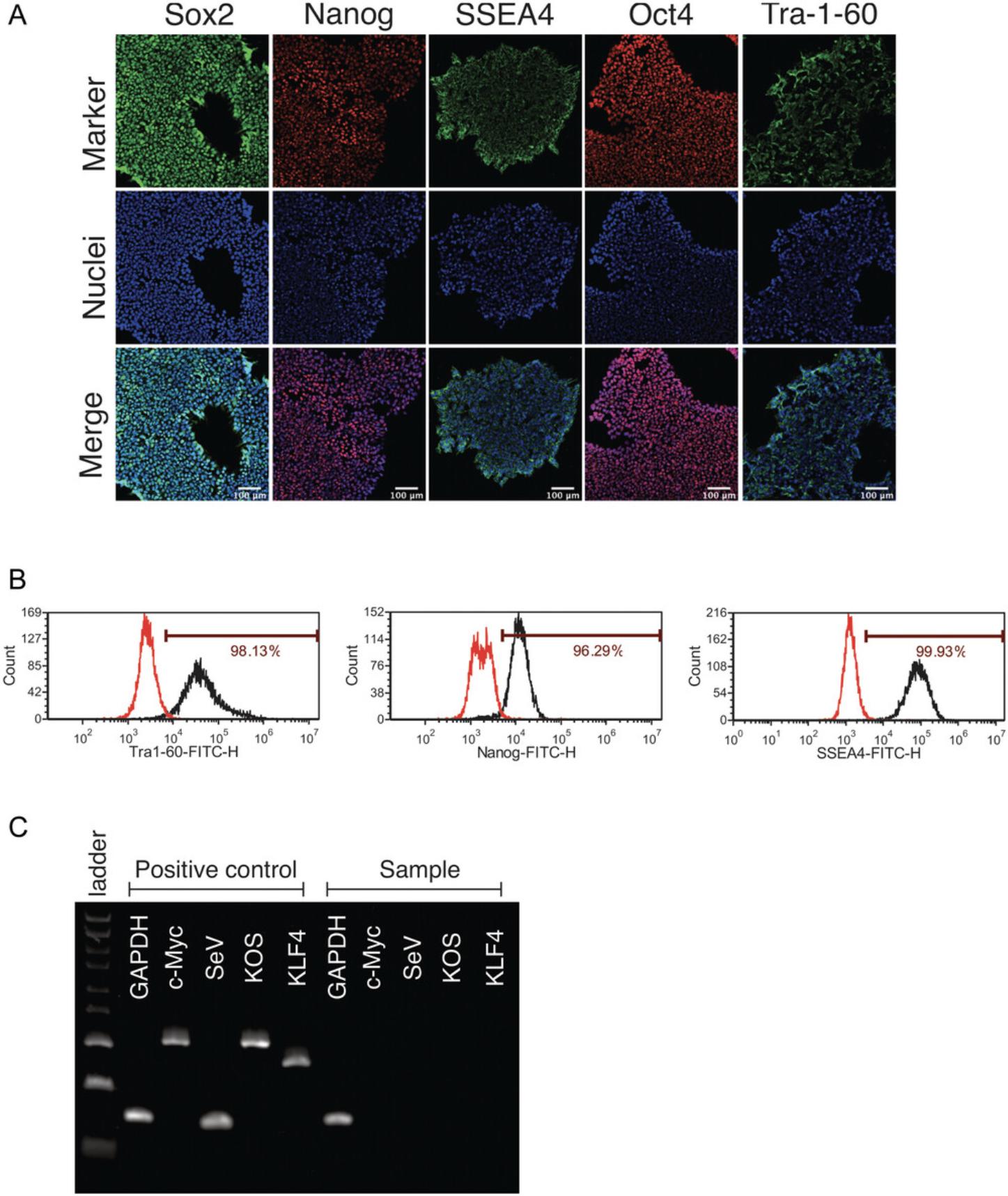
Alternate Protocol: DETERMINATION OF THE EXPRESSION OF PLURIPOTENCY MARKERS VIA FLOW CYTOMETRY ANALYSIS
Flow cytometry is an alternative way to quantitatively examine the expression of pluripotency markers in hiPSCs. This method can quantitate pluripotency marker expression in cell populations, and it provides more quantitative data analysis compared than immunocytochemistry, whereas the immunocytochemistry analysis using fluorescence microscopy provides qualitative information such as subcellular localizations of the markers. The following protocol outlines the steps and reagents for flow cytometry analysis. Cells in single-cell suspension are required for flow cytometry, and thus an enzymatic dissociation method is used.
Materials
-
Confluent hiPSCs (70%-80% confluency) in 6-well plates (from Basic Protocol 1, step 18c)
-
TrypLE Express Enzyme (ThermoFisher Scientific, 12605010)
-
DPBS with calcium and magnesium (ThermoFisher Scientific, 14040141)
-
32% (w/v) paraformaldehyde (Electron Microscopy Sciences, 15714-S)
-
Permeabilization buffer
-
Appropriate fluorophore-conjugated primary antibody (Table 2)
-
Appropriate nonspecific rabbit or mouse IgG (Table 2)
-
Fetal bovine serum (FBS; vendors may vary depending on availability, e.g., HyClone Characterized FBS, US Origin, SH30071.03IH30-45)
-
Tween-20 (Sigma-Aldrich, P9416)
-
DPBS without calcium or magnesium (ThermoFisher Scientific, cat. no. 14190144)
-
1.5-ml microcentrifuge tubes, 4°C
-
2-ml Costar aspirating pipets (Corning, cat. no. 9186)
-
Flow cytometer (Agilent, NovoCyte Penteon)
Antibody | Species | Dilution | Company, cat. no. and RRID |
---|---|---|---|
Anti-Tra-1-60-DyLight 488 | Mouse IgM | 1:50 | ThermoFisher Scientific, MA1–023-D488X, AB_2536700 |
Anti-Nanog-Alexa Fluor 488 | Rabbit IgG | 1:50 | Millipore, FCABS352A4, AB_10807973 |
Anti-SSEA-4-Alexa Fluor 488 | Mouse IgG3 | 1:50 | ThermoFisher Scientific, 53–8843-41, AB_10597752 |
Mouse-IgM-DyLight 488 | Mouse IgM | 1:50 | ThermoFisher Scientific, MA1–194-D488, AB_2536969 |
Rabbit IgG-Alexa Fluor 488 | Rabbit IgG | 1:50 | Cell Signaling, 4340S, AB_10694568 |
Mouse IgG3-FITC | Mouse IgG3 | 1:50 | ThermoFisher Scientific, 11–4742-42, AB_2043894 |
1.Dissociate hiPSCs using the standard cell dissociation method described in Basic Protocol 1 (steps 6-11), except with 1 ml/well of TryPLE EXpress enzyme solution instead of Versene solution for cell dissociation.
2.Collect the cells from one well into a 1.5-ml microcentrifuge tube, centrifuge 5 min at 200 × g to pellet the cells, room temperature, and wash once with 1 ml of DPBS without calcium and magnesium.
3.Aspirate supernatant from the 1.5-ml tube.
4.Fix the cells: Add 0.5 ml/tube of 4% paraformaldehyde diluted in DPBS without calcium and magnesium to resuspend the cell pellet, and incubate for 10 min at room temperature.
5.Wash the cells twice with 1 ml/tube of DPBS without calcium and magnesium, by centrifuging 5 min at 200 × g , room temperature, to pellet cells.
6.Resuspend cells with 0.5 ml/tube of permeabilization buffer and incubate for 10 min at room temperature.
7.Centrifuge at 200 × g , room temperature, and then aspirate the permeabilization buffer from each tube.
8.Add 0.5 ml/tube of the desired fluorophore-conjugated antibody and use a nonspecific rabbit or mouse IgG as a negative control (see Table 2).
9.Wrap each vial with aluminum foil to avoid light exposure and incubate for 1 hr at 4°C on a shaker rotating at 20 rpm.
10.Wash the cells twice with 1 ml DPBS without calcium and magnesium by centrifuging at 200 × g to pellet cells.
11.Analyze stained cells using a flow cytometer at the appropriate wavelengths based on the antibody labeling. Collect at least 10,000 events from the cell gate.
Basic Protocol 4: ASSESSMENT OF PLURIPOTENCY VIA IN VIVO TERATOMA FORMATION
By definition, pluripotency refers to a cell's ability to differentiate into all cell types of the body. Cell morphology, growth property, karyotype, and epigenetic markers may provide some clues to whether the cells are likely to be pluripotent, but they are indirect and unreliable. Random differentiation through embryoid body formation or directed differentiation into specific lineages can in theory address this question, but such in vitro culture methods are prone to false conclusions due to artificial effects caused by inconsistent reagents or poor technical skills. Conversely, injecting hiPSCs into immunocompromised mice to test their ability to form teratomas is a simple and reliable method to find out whether a particular hiPSC line is capable of differentiating into a variety of tissues, including cell types that originate from all three germ layers (ectoderm, mesoderm, and endoderm) during normal embryonic development.
In addition to confirming whether a particular cell line is truly pluripotent in a qualitative manner, teratoma formation assay can also provide semiquantitative insights into a cell line's differentiation propensity. Not all hiPSC lines have equal differentiation tendency toward all three germ layers, as the original source of the hiPSCs and the reprogramming method can significantly influence differentiation propensity. An abundance of tissues from one particular germ layer formed in teratomas may hint at whether the hiPSC line is a suitable choice for in vitro differentiation into the intended cell type for further studies or disease modeling.
Because teratoma assays involve the use of laboratory animals, these experiments need to be approved by the Institutional Animal Care and Use Committee (IAUCU).
Materials
-
hiPSCs (∼80% confluence) in 6-well plates (see Strategic Planning)
-
Complete E8 medium (see recipe) supplemented with 25 mM HEPES (Gibco, cat. no. 11330-032), pH 7.4
-
Matrigel, hESC-Qualified Matrix, LDEV-free (Corning, cat. no. 354277), stored at −80°C
-
Two 8- to 26-week-old NSG immunocompromised mice (Jackson Laboratory, stock no. 005557; both male and female mice can be used)
-
Isoflurane liquid (Baxter Forane cat. no. 10019-360-40)
-
10% Neutral Buffered Formalin (BDH cat. no. BDH0502-4LP)
-
Tissue culture microscope
-
2-ml Costar aspirating pipets (Corning, cat. no. 9186)
-
1.5-ml microcentrifuge tubes, sterile
-
Centrifuge (Beckman Allegra X-14R)
-
Cell counter (Nexcelom Auto T4)
-
Isoflurane vaporizer (SurgiVet Classic T3TM Vaporizer)
-
1-ml tuberculin syringe (BD REF-309659)
-
25 G × 5/8 in. hypodermic needle (BD REF-305122)
-
Scissors (Roboz RS-6808)
-
Forceps (Roboz RS-5159)
-
50-ml conical tubes
1.Harvest 70%-80% confluency hiPSCs from one 10-cm culture dish or all six wells of a 6-well plate using Versene, as described in Basic Protocol 1, step 6-12.
2.Pellet the cells for 5 min at 300 × g , room temperature. Aspirate the supernatant and resuspend the pelleted cells in 500 μl of complete E8 medium with 25 mM HEPES (pH 7.4).
3.Count cells and transfer 8 × 106 cells into a sterile microcentrifuge tube. Adjust the total volume to 400 μl by adding additional E8 medium with 25 mM HEPES (pH 7.4). Cool the cell suspension on ice.
4.Thaw an aliquot of Matrigel on ice. Mix 200 μl of Matrigel with the cold cell suspension so that the final Matrigel concentration is 33% (v/v). Store the tube on ice.
5.Turn on the gas tank regulator to supply pure oxygen to the isoflurane vaporizer. Set the isoflurane concentration to 4%-5%.
6.Place two NSG mice into the induction chamber of the vaporizer.
7.Attach a 25-G needle to a 1-ml sterile syringe and draw the entire 600 μl of cell suspension from step 4 into the syringe.
8.Take one anesthetized mouse out of the isoflurane chamber. Lay the mouse on its back and place its muzzle inside the nose cone of the isoflurane vaporizer.
9.Quickly inject 0.1 ml of cells subcutaneously into the groin area of one of the mouse's hind legs and then inject 0.1 ml of cells into the groin area of the other hind leg. Place the injected mouse back into its cage.
10.Take the second mouse out of the chamber and repeat the injection procedures described in steps 8 and 9.
11.Turn off the isoflurane vaporizer and oxygen tank gas regulator.
12.Mark the cage card with cell line information and injection date. Injected mice should wake up in a few minutes. Return the cage to the animal room.
13.Check the injected mice on the next day and then on a weekly basis. When the tumors reach ∼1 cm in diameter, monitor the mice every other day to make sure that the tumors do not exceed the size limit (usually 2 cm diameter) specified by the Animal Care and Use Committee and the specific animal facility.
14.When one or both tumors nearly reach the size limit, euthanize the mouse by CO2 asphyxiation followed by cervical dislocation.
15.Lay the euthanized mouse on its back and spray 70% ethanol on the ventral side to wet the fur. Use a pair of sharp scissors to make a large cut (∼2 cm long) in the skin of the abdomen area. Use gloved fingers to grasp each side of the cut and tear the skin apart to expose the teratomas, which should sit between the skin and muscle body wall (Fig. 4).
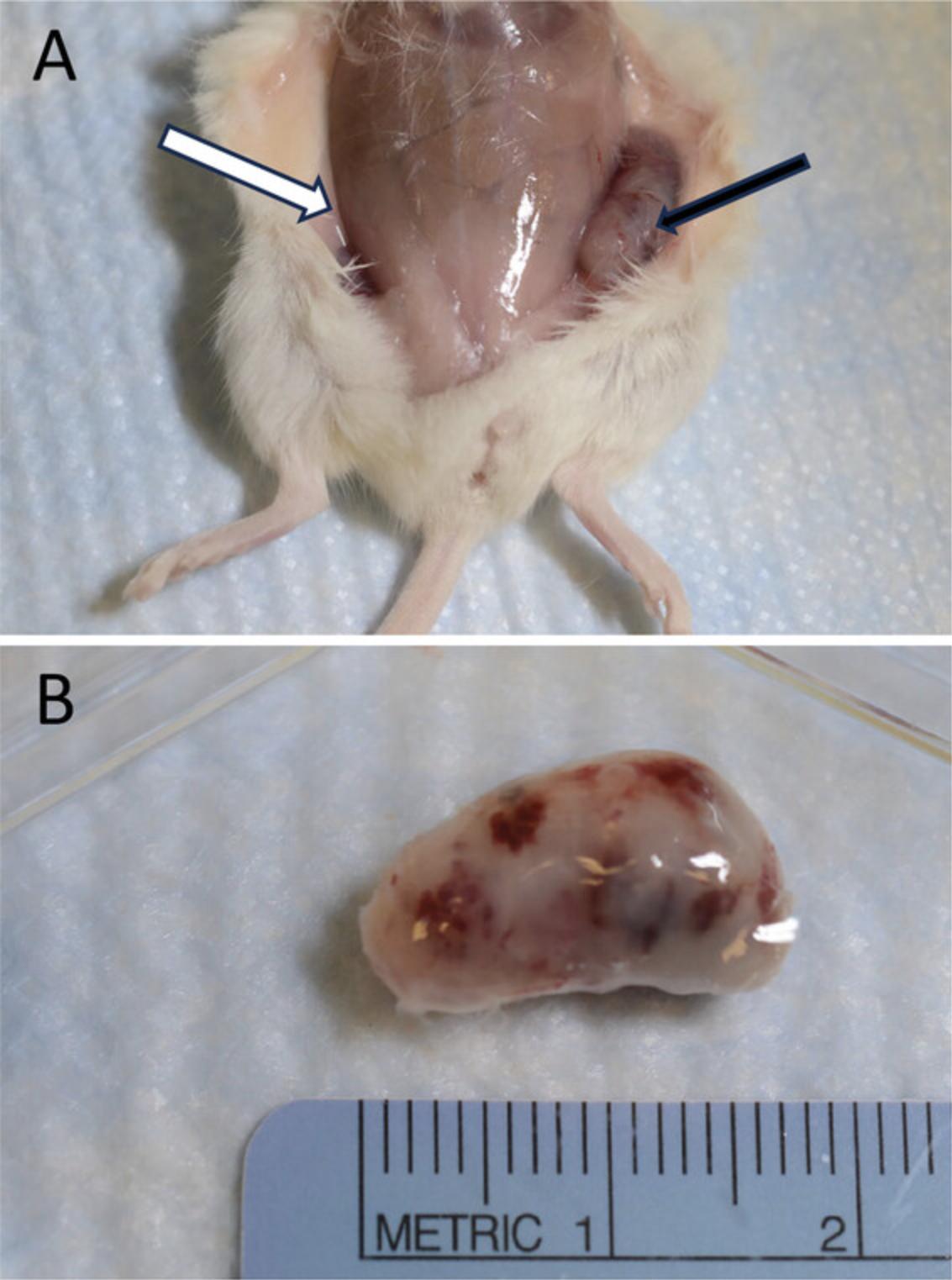
16.Use a pair of iris forceps and a pair of scissors to carefully remove the teratomas and fix them in 10% Neutral Buffered Formalin in 50-ml conical tubes.
17.Embed the fixed teratomas in paraffin, section them to make 5-μm-thick slides, and then stain with H&E (hematoxylin and eosin) following standard procedures; or send them to a contract histology lab to perform these procedures.
18.Examine the stained slides under a light microscope. Alternatively, the entire slide can be scanned using a digital slide scanner, such as the NanoZoomer scanner made by Hamamatsu, Inc. The goal is to look for tissues derived from various germ layers.
19.Truly pluripotent hiPSCs should give rise to tissues derived from all three germ layers. As shown in Fig 5, the tissue most often used to represent ectoderm is the neural epithelium, the most reliable and obvious tissue for mesoderm is cartilage or bone, and the most typical endodermal tissue is the gut, such as intestine. Most properly derived hiPSC lines can give rise to all three germ layer tissues, but their differentiation propensities are often biased toward one or two layers, resulting in large differences in tissue abundances between germ layers. This may be caused by epigenetic memories retained from the donor cell type used for hiPSC reprogramming, partial differentiation due to improper culturing conditions, or mishandling before or during the hiPSC injection process. When a particular cell line fails to yield tissues from all three germ layers, it is recommended that the teratoma assay be repeated with a different hiPSC clone from the same reprograming event. If no other clones are available, subcloning the original hiPSCs may yield good subclones through the elimination of partially differentiated cells or cells that have accumulated deleterious mutations (such as abnormal karyotypes) during the culture process.
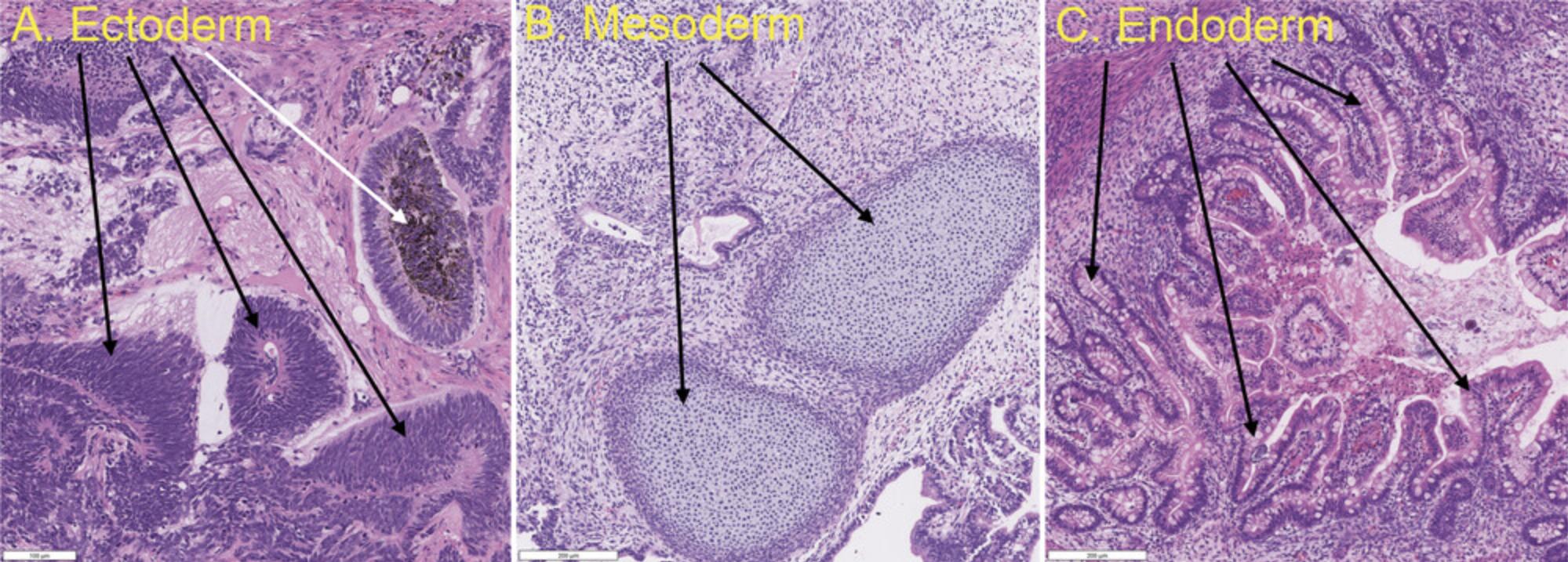
Basic Protocol 5: CONFIRMATON OF SENDAI VIRAL VECTOR CLEARANCE VIA RT-PCR
The reprogramming of somatic cells via the Sendai virus delivery system has become a common approach to efficiently generate hiPSCs. The advantages of the Sendai virus reprogramming system include safety for humans, as no human diseases are linked to this virus; broad cellular tropism, as it binds to ubiquitous sialic acid as the cellular receptor to enter human cells; and a non-integrating viral replication mechanism that does not alter the host genome. Sendai-virus-mediated hiPSC induction has been shown to maintain genomic integrity with minimal level of genetic aberration. However, it still takes a few months for transgenes to be cleared from the reprogrammed cells. Validation of the clearance of reprogramming materials from hiPSCs is an important quality control for establishing hiPSC lines. Reverse transcriptase polymerase chain reactions (RT-PCR) with primers binding to the reprogramming factors is considered one of the best methods for detecting the transgenes. In this protocol, an RT-PCR analysis is used to confirm that no residual transgenes of viral origin remain in the hiPSCs when the non-integrative Sendai virus delivery system was used to generate hiPSCs. The original human somatic cells that were freshly transduced with the Sendai virus system are used as a positive control for this experiment. It is important to use hiPSCs that have cleared genetic materials from iPSC induction for other downstream applications such as differentiation into neurons or organoids.
Materials
- Somatic cells (e.g., fibroblasts)
- Complete somatic cell culture medium (e.g., DMEM/10% FBS for fibroblasts)
- CytoTune™-iPS 2.0 Sendai Reprogramming Kit (ThermoFisher Scientific, A16517)
- TrypLE Express Enzyme (ThermoFisher Scientific, 12605010)
- DPBS without calcium and magnesium (ThermoFisher Scientific, cat. no. 14190144)
- Confluent hiPSCs in 6-well plates (from Basic Protocol 1, step 18c)
- Qiagen RNeasy Plus Mini Kits (Qiagen, 74034) or other RNA isolation protocols
- SuperScript™ III First-Strand Synthesis SuperMix (ThermoFisher Scientific, 18080400) or other reverse transcription kit
- Platinum II Hot-Start PCR Master Mix (ThermoFisher Scientific, 14000012) or other PCR cocktail
- PCR primers (Table 3)
- E-Gel Agarose Gels with SYBR Safe DNA Gel Stain, 1% (ThermoFisher Scientific, A45202)
- E-Gel 1 Kb Plus DNA Ladder (ThermoFisher Scientific, 10488090)
- E-Gel Sample Loading Buffer, 1X (ThermoFisher Scientific, 10482055)
- Humidified 37°C, 5% CO2 incubator
- Tissue culture microscope
- 2-ml Costar aspirating pipets (Corning, cat. no. 9186)
- 15-ml conical tube
- PCR thermocycler
- Invitrogen E-Gel iBase and E-Gel Safe Imager (Fisher Scientific, 10001123)
- G: Box Chemi-XX6 gel doc system (Syngene)
- NanoDrop 2000 Spectrophotometer (ThermoFisher Scientific)
Target | Forward and reverse primers (5′-3′) | |
---|---|---|
Reference genes | GAPDH/197bp |
Fw: GGAGCGAGATCCCTCCAAAAT Rv: GGCTGTTGTCATACTTCTCATGG |
SeV-specific primers | SeV/181bp |
Fw: GGA TCA CTA GGT GAT ATC GAG C Rv: ACC AGA CAA GAG TTT AAG AGA TAT GTA TC |
SeV-specific primers | KOS/528bp |
Fw: ATG CAC CGC TAC GAC GTG AGC GC Rv: ACC TTG ACA ATC CTG ATG TGG |
SeV-specific primers | Klf4/410bp |
Fw: TTC CTG CAT GCC AGA GGA GCC C Rv: AAT GTA TCG AAG GTG CTC AA |
SeV-specific primers | C-Myc/523bp |
Fw: TAA CTG ACT AGC AGG CTT GTC G Rv: TCC ACA TAC AGT CCT GGA TGA TGA TG |
Preparation of positive control samples
Day –2
1.Seed human fibroblasts into a well of a 6-well plate so that the cells reach 30%-60% confluence on the day planned for the experiment (day 0).
Day 0
2.Change the culture medium and add CytoTune™-iPS 2.0 Sendai reprogramming vectors at the appropriate multiplicity of infection (MOI) following the manufacturer's instructions.
3.Incubate the cells overnight in a 37°C, 5% CO2 incubator.
Day 1
4.Aspirate medium containing Sendai reprogramming vectors in the 6-well plate. Add 1 ml fresh complete fibroblast medium and return to the incubator.
5.Change medium every other day (1 ml/well).
Day 4
6.Dissociate cells using standard cell dissociation method (Basic Protocol 1, steps 6-11), except with 1 ml/well of TryPLE EXpress enzyme solution instead of Versene solution for cell dissociation. Collect the final 1 ml/well cells into a 1.5-ml microcentrifuge tube.
7.Pellet the cells via centrifugation for 5 min at 200 × g , room temperature. Remove the supernatant and wash the cell pellet once with 1 ml of DPBS without calcium and magnesium.
8.Pellet cells again via centrifugation for 5 min at 200 × g. Remove the supernatant. The cell pellet can be used immediately for the experiment (continue to step 12 below) or stored at −80°C for later use.
RT-PCR for reprogramming vectors
9.Collect hiPSCs from four confluent wells of a 6-well plate (70%-80% confluence, following the Basic Protocol 1) into a 15-ml conical tube by the enzymatic dissociation method using 1 ml/well of TrypLE Express solution. Pellet cells via centrifugation for 5 min at 200 × g.
10.Resuspend the cell pellet with 1 ml DPBS without calcium and magnesium. Transfer the cell suspension into a 1.5-ml microcentrifuge tube. Pellet cells via centrifugation for 5 min at 200 × g. Wash the cell pellet once with 0.5 ml DPBS without calcium and magnesium.
11.Pellet cells via centrifugation for 5 min at 200 × g and remove the supernatant. The cell pallet can be used immediately for the experiment in the following step or stored at −80°C for later use.
12.Extract total RNA from the hiPSC sample (from Basic Protocol 1, step 18c) and from the Sendai-virus-transfected fibroblast pellet from step 10 of this protocol using a Qiagen RNeasy Plus Mini Kit by following the manufacturer's instructions. Quantitate the RNA concentrations with a Nanodrop 2000 spectrophotometer, which provides a ratio of A 260 nm/A 280 nm and RNA concentration (μg/ml) detected in samples. The typical A 260/A 280 ratio is 1.8-2.2 and the typical RNA yield is in the range of 2.4-4.0 µg/ml.
13.Use 0.5 µg of total RNA to synthesize cDNA in a 20-µl reaction using the SuperScript™ III First-Strand Synthesis SuperMix kit or other cDNA reverse transcription kit, following the manufacturer's instructions.
14.Add 1 µl of the resulting cDNA above to 20 μl/tube of Platinum II Hot-Start PCR Master Mix for PCR. Prepare 5 reactions for each test sample, including one reaction of internal control (GAPDH) and four reactions using the primers listed in Table 3.
15.Run PCR using the following conditions on a Mastercycler pro S (Eppendorf) Thermal Cycler instrument:
Initial step: | 2 min | 94°C |
30 cycles: | 14 s | 94°C |
15 s | 60°C | |
15 s | 68°C. |
16.Mix 10 µl of each PCR product with 10 µl of 2× loading buffer, and load each sample in one lane of a 1% agarose E-Gel Agarose gel.
17.Load 25 µl of 1 Kb DNA ladder to a lane of each gel.
18.Run DNA gel with E-Gel 0.8%-2% Program, and then stain with the SYBR Safe DNA Gel Stain to visualize the PCR products. Analyze PCR products using an imager (e.g., G: Box Chemi-XX6 gel doc system by Syngene). A typical gel picture is shown in Figure 3c.
REAGENTS AND SOLUTIONS
Complete E8 medium
From a Essential 8 Flex Medium kit (ThermoFisher Scientific, cat. no. A2858501), thaw 10 ml of 50× supplement (stored in −80°C) in a 4°C refrigerator overnight, and then add it to 490 ml E8 medium. Store up to 2 weeks at 2°C-8°C.
Y-27632 dihydrochloride, 10 mM
Dissolve Y-27632 dihydrochloride powder (Tocris, catalog number 1254) in distilled water (dH2O) to a 10 mM solution. Divide into aliquots and store up to 1 year at −80°C.
COMMENTARY
Background Information
Pluripotent cells are cells that have the capability to differentiate to all somatic lineages in the human body. They have been found to exist transiently in the inner cell mass (ICM) of the blastocyte, where they can be collected and used to generate embryonic stem cell (ESC) lines whose pluripotency can be successfully maintained in culture. ESCs that are propagated in a defined cell culture environment show a transcriptional profile and epigenetic states resembling those of pluripotent epiblast cells (Hanna et al., 2010b; Nichols and Smith, 2012; Weinberger et al., 2016). An additional source of pluripotent cells is the direct reprograming of somatic cells into pluripotency in vitro via the ectopic expression of defined transcription factors, generating induced pluripotent stem cells (iPSCs; Takahashi et al., 2007; Takahashi and Yamanaka, 2006). Advances in human iPSC (hiPSC)-related technologies have offered great opportunities for stem-cell-based therapies, tissue/organ regeneration and replacement, and disease modeling. Patient-derived hiPSCs preserve the genetic background and disease-related gene mutations of the donors and have been successfully used as in vitro disease models for studies of disease pathophysiology and evaluation of drug efficacy for drug development. Modeling diseases using human cells (e.g., neurons, hepatocytes, and cardiomyocytes) and organoids (e.g., brain organoids, liver organoids etc.) derived from hiPSCs has advantages over animal models as many disease phonotypes are not recapitulated by animal models due to species differences (Farkhondeh et al., 2019). Patient-derived hiPSCs could be especially useful for modeling genetic diseases, as there are currently over 7000 known genetic diseases, including many rare diseases for which proper disease models are lacking. The original process for hiPSC generation and expansion requires the use of mouse or human fibroblasts as a feeder layer to support hiPSC growth. The preparation of feeder cells is labor intensive and contributes to significant batch variations, resulting in inconsistent hiPSC expansion. More recently, feeder-free culture systems for hiPSC generation and expansion have been developed, consisting of Matrigel or other extracellular matrix (ECM) proteins and completely defined stem cell culture medium. These systems have been widely applied to maintain and culture hiPSCs and hESCs. Thus, it is important to update hiPSC culture protocols periodically to ensure a reliable and consistent process of culturing and maintaining hiPSCs in laboratories for downstream applications such as cell therapy development and disease modeling. In order to find the optimal culture condition for hiPSCs, we have evaluated and optimized parameters including matrix, cell culture medium, cell seeding density, dissociation reagent, and other related reagents as discussed in each section of this article.
Culturing hESCs and hiPSCs is very challenging work that requires experienced staff with excellent cell culture skill and gentle hands. Poor post-thaw recovery rate, spontaneous differentiation, contamination with bacteria, fungi, and/or mycoplasma, and low reproducibility are some common issues encountered with hiPSC culture. In addition to proper staff training for hiPSC culture work, many details and tips are included in these protocols describing how to overcome these obstacles in hiPSC culture process. The low cryopreservation efficiency of hiPSCs has recently been improved through supplementation with ROCK inhibitor in the cryopreservation medium and revival medium, although extensive technical improvements and excellent technical skills are also required to achieve high recovery rates from cryopreserved stocks. Eliminating centrifugation of hiPSCs after thawing increase hiPSC recovery rate as it reduces cell stress. The critical standards for high-quality hiPSCs are still in development by researchers in the field. The quality of hiPSCs can be influenced by many factors, including culture materials, culture conditions, culture manipulation techniques, and operator's handling skills. We have included in our protocols the parameters defining high-quality hiPSCs, which are measured by colony morphology observed by microscopy, expression of pluripotency markers determined by immunofluorescence assay and flow cytometry analysis, transgene-/vector-free condition assayed by RT-PCR, and cell identity assessed by STR (to ensure that the identity of the hiPSCs is the same as that of the original somatic cells). The combination of these parameters, cytogenetic analysis, and potential for three-germ-layer differentiation in vivo or in vitro should provide valuable information to examine the quality and integrity of hiPSCs in long-term culture, which can easily last weeks to months.
In addition to confirming in a more qualitative manner whether a particular cell line is truly pluripotent for differentiation into three layers, the teratoma formation assay can provide semi-quantitative insights into a cell line's differentiation propensity. Not all hiPSC lines have an equal tendency for differentiation into all three germ layers because the original source of the hiPSCs and the reprogramming method can significantly influence iPSC differentiation propensity. Therefore, the abundance of tissues from one particular germ layer in teratomas may hint at whether the hiPSC line is a suitable choice for in vitro differentiation into particular types of cells, tissues, or organs.
Critical Parameters
Experiences with cell culture, sterile technique, and gentle handling are needed for staff who work on hiPSC culture. Careful monitoring of cell morphology is an important task during the hiPSC culture process. Pluripotency is a crucial property of hiPSCs that enables them to maintain unlimited self-renewal and differentiate into all the cell types of interest for various downstream applications. However, hiPSCs can spontaneously differentiate into other types of cells during culture and passaging. Proper maintenance of hiPSCs in culture is critical for sustaining their self-renewal ability and efficient differentiation into three germ layers. Moreover, careful monitoring of cell morphology and removal of differentiated cells are critical for the maintenance of pluripotency for downstream applications after long-term culture.
In particular, because passaging hiPSC cells at an appropriate cell density (confluency) and time is critical during hiPSC culture and propagation, daily evaluation of the hiPSC culture via microscopy is necessary to monitor the morphology and confluence of hiPSCs. Because each hiPSC line may exhibit a different growth rate, the split ratio of a given hiPSC line needs to be adjusted at each passage based on the appearance of the colonies (confluency) and number of days needed to reach 70%-80% confluence. Usually, the split ratio of hiPSCs during passaging is between 1:6 and 1:10. If the hiPSC colonies are becoming too dense or too large, a split ratio of 1:10 or higher is needed. The cells should be passaged every 4-5 days according to the appearance of the colonies in terms of size and density. Importantly, any spontaneously differentiated cells should be removed before passaging.
Quality control steps for hiPSC cultures are discussed in the Understanding Results section below.
Troubleshooting
Table 4 lists common problems, their causes, and potential solutions for these protocols.
Problem | Possible cause(s) | Solution(s) |
---|---|---|
Transgenes detectable in hiPSC clone | Transgene(s) have become randomly integrated into the genome. | Generate fresh iPSCs from somatic cells. |
Unexpected differentiation of hiPSCs during culture and passaging (>10% of the population is differentiating) | Differentiated cells were accidentally carried over. | Remove differentiated area prior to passaging, restart from a different hiPSC clone, or generate fresh hiPSCs from patient samples. |
An unstable clone was selected from the hiPSC generation, leading to spontaneous differentiation. | ||
A disease-related gene mutation occurred (in hiPSCs generated from patient cells). | Generate fresh hiPSCs from a different patient sample with different mutations. | |
hiPSCs were kept in Y-27632 dihydrochloride for too long (>24 hr). | Make sure hiPSCs are not cultured with Y-27632 dihydrochloride for a long period. | |
Insufficient cell recovery after thawing | Inappropriate freezing and thawing process was used. | Optimize steps and culture materials options for the freezing, thawing, and seeding of hiPSCs on feeder-free, ECM-coated cell culture plates. |
hiPSCs grow slowly | Cell numbers are too low; cell aggregates are too small, or single cells, during cell passaging. | As the hiPSCs grow to ∼70%-80% confluency, make sure that they remain in the log growth phase and are split at a 1:2-1:6 ratio. Avoid harsh pipetting that disrupts the clone into single cells or tiny aggregates during passaging. A cell aggregate size of 50-200 µm is normally recommended. |
Contamination from bacteria, fungi, or mycoplasma occurs | Poor aseptic technique was followed, such as using contaminated pipet tips, flasks, medium, or water. | Discard the hiPSCs and use a fresh vial of hiPSC stock to restart the culture. We do not recommend attempting cleanup mycoplasma infections in hiPSCs because the “cleanup” is usually incomplete and the mycoplasma infection will reoccur after a few passages. |
Massive cell death and loss occur after several passages (most often 5-7 passages after hiPSC generation from somatic cells) | Original iPSC colonies were of poor quality. | Consider selecting a different hiPSC colony to culture, or generating fresh hiPSCs. |
No teratoma growth 12 weeks after injection | hiPSCs were cultured under non-optimal conditions before injection, allowed to become over-confluent in culture, cultured in bad (e.g., not freshly made) medium, or not fed or split on time. | Repeat the injection using cells in rapidly growing phase (50%-70% confluency). |
Cells were mishandled during harvesting or injection (e.g., too much time passed between harvest and injection); 25 mM HEPES (pH 7.4) was omitted from medium, so the pH of the cell suspension was not properly maintained when cells were outside of the CO2 incubator; the high-density cell suspension was not kept on ice, which can cause depletion of metabolites due to higher metabolic rate at room temperature. | Repeat the injection using proper handling methods. | |
Too few cells were successfully injected (e.g., a cell counting error occurred or cells leaked out from injection site); needle was too thin or too thick (causing cell lysis or leakage, respectively). | Repeat the injection using the correct number of cells and size of needle. | |
hiPSC line has lost pluripotency and therefore does not form teratomas when injected subcutaneously. | Choose a different hiPSC clone. If no alternative clones are available, repeat the injection using more mice and more cells. Try injecting into alternative sites, such as intramuscularly or in the kidney capsule or testicular capsule, which require fewer cells and provide different niches. | |
Karyotyping results show incomplete pluripotency markers or abnormal chromosome morphology | Original iPSC colonies were of poor quality. | Discard hiPSC colony and select a fresh colony from the same hiPSC pool after hiPSC generation. Consider cytogenetic analysis by karyotyping to check chromosome integrity in original donor cells. If the original donor cells (somatic cells) show abnormal chromosome integrity, the patient sample should not be used again. |
Teratomas are largely cysts with very little solid tissues, or solid tumors are homogenous with very little well differentiated cell types | Cell line is not pluripotent or is contaminated with transformed cancerous cells; or certain genes used for reprograming were not fully inactivated, which promotes proliferation and inhibits differentiation. | Choose a different hiPSC clone. If no alternative clones are available, inject more mice (5-6) using properly handled cells to confirm whether the cell line is indeed incapable of differentiating into various cell types. |
Teratomas contain abundant tissues from certain germ layers but few tissues from other germ layer(s) | Cells retain epigenetic memory from donor cells or incomplete hiPSC reprograming; cells carry genetic defects that inhibit differentiation into certain cell lineages or promote differentiation into other cell lineages; the analyzed tumor happens to be abundant in certain tissue types by chance (as cell differentiation in teratomas is largely random and sporadic). | Choose different hiPSC clones, if available. Otherwise, repeat the injection using more mice to confirm that the line is biased towards differentiating into a certain tissue type. If this is confirmed, this cell line could be preferable or non-preferable depending on the purpose of your study. |
Understanding Results
Sample images of hiPSCs and results of hiPSC characterization are shown in Figures 2 and 3.More results of hiPSC culture, passaging, and characterization are also described in detail in our previous papers (Li et al., 2020; Xu et al., 2020). The following special points are useful for understanding the results.
General quality control assays
Variations between cell lines are expected in hiPSC culture. Standardized quality control measures for hiPSC culture can minimize variability and increase reproducibility. The morphology of hiPSCs in culture is the most easily monitored indicator of cell quality, as it can be observed under a common microscope. Typically, hiPSCs appear as relatively round colonies with defined borders and tightly packed cells that have a high ratio of nucleus to cytoplasm (larger nuclear area than cytoplasm in typical cells). hiPSCs are characterized by specific pluripotency cell surface markers (SSEA-4 and TRA-1-60) and key transcription factors regulating the pluripotency network (SOX2, OCT4, and Nanog). Those cell surface markers and transcription factors should be homogeneously expressed in nearly all cells in the hiPSC colonies. In addition, high-quality hiPSCs exhibit no evidence of transgenes (i.e., the genes and gene delivery vectors used in generating them from somatic cells are absent).
Teratoma assay
The teratoma assay, which tests whether hiPSCs can differentiate to all three germ layers, is commonly used to evaluate the differentiation potential of hiPSCs in vivo. An hiPSC-derived teratoma formed in an immunodeficient mouse is usually a heterogeneous tumor composed of differentiated cells representative of all three germ layers. Teratomas are validated by H&E staining and through the detection of specific markers for endoderm, mesoderm, and ectoderm germ layers (Fig. 5).
Other hiPSC quality control experiments
The five basic protocols detailed above are important for hiPSC culture and characterization. The additional experiments listed in Figure 1b for hiPSC quality control are more general, and usually can be outsourced to core facilities or contract research organization (CRO) using live hiPSCs (Basic Protocol 1, step 18c) or cryopreserved hiPSCs (Basic Protocol 2, step 12).
- 1.Karyotyping experiment. This assay is used to check the chromosome integrity in hiPSCs after culturing. If chromosomal abnormality is found, the hiPSCs should be discarded. An example of a CRO that performs G-banding karyotype analysis is the WiCell Research Institute (Madison, WI, USA), which we have used.
- 2.Short tandem repeat (STR) DNA profile analysis. The STR profiling assay is commonly used to examine whether a cell line in culture is same as the original cell line, as unexpected cell line contamination or mislabeling can occur during a long-term cell culture. Both the hiPSCs and the original somatic cells (e.g., patient fibroblasts) used to generate the hiPSC line are needed for the STR assay to assess whether the hiPSCs in culture match the original donor cells. If the STR profiles of the two cell lines are not match (due to contamination or mislabeling), the hiPSCs must be discarded. We have contracted STR experiments to WiCell Research Institute (Madison, WI, USA).
- 3.Mycoplasma test. Mycoplasma can be detected and analyzed using an assay kit, and should be routinely tested for cell culture. For example, the MycoAlert Kit (Lonza, cat. no. LT07-318) can be used by following manufacturer's instruction. A B/A ration of >1.2 indicates mycoplasma-positive status; 0.9-1.2 indicates ambiguous result; and <0.9 indicates mycoplasma-negative status.
Time Considerations
- 1.Propagation and cryopreservation of hiPSCs take 10-15 min. However, once seeded, hiPSC cultures take 4-5 days to reach 70%-80% confluence for the next passage (Basic Protocol 1). Thawing cells take ∼1 hr: 15-30 sec to thaw a cryovial and 1 hr for cell attachment (Basic Protocol 2).
- 2.It takes ∼2 days before the hiPSCs are ready for immunostaining. Cell fixing and washing take ∼30 min. Cell permeabilization after fixation takes 30 min. Blocking cells takes 1 hr at room temperature. Staining with primary antibodies requires an overnight incubation. Staining with secondary antibodies takes 1.5 hr, including three cell wash steps. Nuclear staining takes 30 min (Basic Protocol 3).
- 3.It takes ∼15-30 min to prepare cell pellets for flow cytometric analysis. Cell fixing and washing take ∼20 min. Cell permeabilizing takes 10 min. Antibodies staining takes 1 hr. Final cell washing take 10 min before detection (Alternate Protocol).
- 4.For the teratoma formation assay, hiPSC dissociation, spin-down, cell counting, and injection into two immunocompromised mice can usually be done within 1-2 hr. For most cell lines, teratomas can reach the maximum allowed size (usually 2 cm diameter, but this can vary based on the approved animal protocol) within 6-12 weeks post injection. Euthanizing mice and harvesting teratomas can usually be done in 20 min, but it is likely that the two mice will need to be euthanized at different times due to differences in tumor growth rates. Teratomas are usually fixed in 10% formalin overnight before being sent to a CRO for sectioning and staining, which often takes 2-3 weeks. Examining stained slides (usually three slides from different regions of each tumor) and taking photographs of representative germ layer tissues usually take 30-60 min (Basic Protocol 4).
- 5.It takes 7 days to prepare positive control samples for the Sendai virus vector assay to confirm elimination of virus vector and transgenes introduced during iPSC generation, including 2 days for culturing fibroblasts and 5 days for iPSC induction. RNA extraction requires 25-30 min. PCR sample preparation and amplification take 1.5-2 hr, and an additional 30-60 min is required for detection via gel electrophoresis (Basic Protocol 5).
Acknowledgments
This work was supported by the Intramural Research Programs of the National Center for Advancing Translational Sciences (NCATS), US National Institutes of Health.
Author Contributions
Yu-Shan Cheng : Writing—initial draft, methodology, validation, writing—review and editing. Miao Xu : Data curation, methodology. Guibin Chen : Data curation, methodology, validation, visualization, writing—review and editing. Jeanette Beers : Methodology. Catherine Chen : Validation, writing—review and editing. Chengyu Liu : Methodology, writing—review and editing. Jizhong Zou : Methodology, Project administration, writing—review and editing. Wei Zheng : Conceptualization, funding acquisition, investigation, methodology, project administration, resources, supervision, writing—review and editing.
Conflict of Interest
The authors declare no conflict of interest.
Open Research
Data Availability Statement
No new data were created or analyzed during the writing of this protocol.
Literature Cited
- Beers, J., Gulbranson, D. R., George, N., Siniscalchi, L. I., Jones, J., Thomson, J. A., & Chen, G. (2012). Passaging and colony expansion of human pluripotent stem cells by enzyme-free dissociation in chemically defined culture conditions. Nature Protocols , 7(11), 2029–2040. https://doi.org/10.1038/nprot.2012.130
- R. Behringer, M. Gertsenstein, K.V. Nagy, & A. Nagy (Eds.) (2014). Manipulating the Mouse Embryo, 4th edition. Cold Spring Harbor Laboratory Press.
- Chen, G., Gulbranson, D. R., Hou, Z., Bolin, J. M., Ruotti, V., Probasco, M. D., Smuga-Otto, K., Howden, S. E., Diol, N. R., Propson, N. E., Wagner, R., Lee, G. O., Antosiewicz-Bourget, J., Teng, J. M. C., & Thomson, J. A. (2011). Chemically defined conditions for human iPSC derivation and culture. Nature Methods , 8(5), 424–429. https://doi.org/10.1038/nmeth.1593
- Cheng, Y. S., Li, R., Baskfield, A., Beers, J., Zou, J., Liu, C., & Zheng, W. (2019). A human induced pluripotent stem cell line (TRNDi007-B) from an infantile onset Pompe patient carrying p.R854X mutation in the GAA gene. Stem Cell Research , 37, 101435. https://doi.org/10.1016/j.scr.2019.101435
- Farkhondeh, A., Li, R., Gorshkov, K., Chen, K. G., Might, M., Rodems, S., Lo, D. C., & Zheng, W. (2019). Induced pluripotent stem cells for neural drug discovery. Drug Discovery Today , 24(4), 992–999. https://doi.org/10.1016/j.drudis.2019.01.007
- Hanna, J. H., Saha, K., & Jaenisch, R. (2010b). Pluripotency and cellular reprogramming: Facts, hypotheses, unresolved issues. Cell , 143, 508–525. https://doi.org/10.1016/j.cell.2010.10.008
- Inoue, H., Nagata, N., Kurokawa, H., & Yamanaka, S. (2014). iPS cells: A game changer for future medicine. The EMBO Journal , 33(5), 409–417. https://doi.org/10.1002/embj.201387098
- Li, R., Pradhan, M., Xu, M., Roeder, A., Beers, J., Zou, J., Liu, C., Porter, F. D., & Zheng, W. (2020). An induced pluripotent stem cell line (TRNDi001-D) from a Niemann-Pick disease type C1 (NPC1) patient carrying a homozygous p. I1061T (c. 3182T>C) mutation in the NPC1 gene. Stem Cell Research , 44, 101737. https://doi.org/10.1016/j.scr.2020.101737
- Long, Y., Xu, M., Li, R., Dai, S., Beers, J., Chen, G., Soheilian, F., Baxa, U., Wang, M., Marugan, J. J., Muro, S., Li, Z., Brady, R., & Zheng, W. (2016). Induced pluripotent stem cells for disease modeling and evaluation of therapeutics for Niemann-Pick disease type A. Stem Cells Translational Medicine , 5(12), 1644–1655. https://doi.org/10.5966/sctm.2015-0373
- Nichols, J., & Smith, A. (2012). Pluripotency in the embryo and in culture. Cold Spring Harbor Perspectives in Biology , 4, a008128. https://doi.org/10.1101/cshperspect.a008128
- Shi, Y., Inoue, H., Wu, J. C., & Yamanaka, S. (2017). Induced pluripotent stem cell technology: A decade of progress. Nature Reviews Drug Discovery , 16(2), 115–130. https://doi.org/10.1038/nrd.2016.245
- Takahashi, K., Tanabe, K., Ohnuki, M., Narita, M., Ichisaka, T., Tomoda, K., & Yamanaka, S. (2007). Induction of pluripotent stem cells from adult human fibroblasts by defined factors. Cell , 131, 861–72. https://doi.org/10.1016/j.cell.2007.11.019
- Takahashi, K., & Yamanaka, S. (2006). Induction of pluripotent stem cells from mouse embryonic and adult fibroblast cultures by defined factors. Cell , 126, 663–76. https://doi.org/10.1016/j.cell.2006.07.024
- Weinberger, L., Ayyash, M., Novershtern, N., & Hanna, J. H. (2016). Dynamic stem cell states: Naive to primed pluripotency in rodents and humans. Nature Reviews Molecular Cell Biology , 17, 155–169. https://doi.org/10.1038/nrm.2015.28
- Xu, X., Pradhan, M., Xu, M., Cheng, Y. S., Beers, J., Linask, K. L., Lin, Y., Zheng, W., & Zou, J. (2020). Four induced pluripotent stem cell lines (TRNDi021-C, TRNDi023-D, TRNDi024-D and TRNDi025-A) generated from fibroblasts of four healthy individuals. Stem Cell Research , 49, 102011. https://doi.org/10.1016/j.scr.2020.102011
Citing Literature
Number of times cited according to CrossRef: 1
- Hao Yang, Yuan Yang, Zijun Lu, Joe Z Zhang, Simultaneous Optical Imaging of Action Potentials and Calcium Transients in Human Induced Pluripotent Stem Cell–Derived Cardiomyocytes, Current Protocols, 10.1002/cpz1.1101, 4 , 7, (2024).