A Basic Guide to the Propagation and Manipulation of the Clubroot Pathogen, Plasmodiophora brassicae
Rasha Salih, Rasha Salih, Muhammad Asim Javed, Muhammad Asim Javed, Anne-Sophie Brochu, Anne-Sophie Brochu, Priyavashini Prakash, Priyavashini Prakash, Jean-Dominic Côté, Jean-Dominic Côté, Edel Pérez-López, Edel Pérez-López
Abstract
Clubroot caused by the obligate parasite Plasmodiophora brassicae is a devastating disease affecting the canola industry worldwide. The socio-economic impact of clubroot can be significant, particularly in regions where Brassica crops are a major agricultural commodity. The disease can cause significant crop losses, leading to reduced yield and income for farmers. Extensive studies have been conducted to understand the biology and genetics of the pathogens and develop more effective management strategies. However, the basic procedures used for pathogen storage and virulence analysis have not been assembled or discussed in detail. As a result, there are discrepancies among the different protocols used today. The aim of this article is to provide a comprehensive and easily accessible resource for researchers who are interested in replicating or building upon the methods used in the study of the clubroot pathogen. Here, we discuss in detail the methods used for P. brassicae spore isolation, inoculation, quantification, propagation, and molecular techniques such as DNA extraction and PCR. © 2024 The Authors. Current Protocols published by Wiley Periodicals LLC.
Basic Protocol 1 : Extraction of Plasmodiophora brassicae resting spores and propagation
Support Protocol 1 : Evans blue staining to identify resting spore viability
Support Protocol 2 : Storage of Plasmodiophora brassicae
Basic Protocol 2 : Generation of single spore isolates from P. brassicae field isolates
Basic Protocol 3 : Phenotyping of Plasmodiophora brassicae isolates
Basic Protocol 4 : Genomic DNA extraction from Plasmodiophora brassicae resting spores
Basic Protocol 5 : Molecular detection of Plasmodiophora brassicae
INTRODUCTION
The clubroot pathogen Plasmodiophora brassicae is a member of a group of plant-pathogenic protists known as plasmodiophorids (Schwelm et al., 2017). The genus Plasmodiophora was originally established by Woronin, who initially considered them a subset of Myxomycetes (Woronin, 1877). Myxomycetes are categorized as fungi, and although both share a chitinous cell wall in their resting spores (Muirhead & Pérez-López, 2022), plasmodiophorids exhibit distinct characteristics such as cruciform nuclear division, secondary zoospores with two flagella, plasmodia with multiple nuclei, resting spores with a single nucleus, and the absence of filamentous growth, traits clearly setting plasmodiophorids apart from fungi and oomycetes (Bulman et al., 2011).
Clubroot disease primarily affects cruciferous plants, leading to the formation of galls or clubs in the roots of susceptible hosts (Javed et al., 2023). This disease poses a significant global threat to agricultural production and has serious implications for global food security (Wu & Pérez-López, 2023). To tackle this issue, numerous countries worldwide are actively devising strategies to manage and control clubroot disease. Cultural practices such as crop rotation and sanitation are being implemented to minimize the disease's spread and impact (Hwang et al., 2012; Javed et al., 2023). However, the most effective current management strategy involves the development of resistant plant varieties capable of withstanding P. brassicae infection (Ochoa et al., 2023). Nonetheless, it is important to note that new clubroot pathogen isolates have demonstrated the ability to overcome various clubroot-resistant profiles in commercial canola cultivars (Hwang et al., 2019).
The clubroot pathogen is a soil-borne, biotrophic, obligate parasite, necessitating a living host to complete its life cycle (Kageyama & Asano, 2009). These traits have hindered advancements in biology, genomics, and the development of novel clubroot-resistant cultivars (Javed et al., 2023). From a practical point of view, handling the clubroot pathogen is also very challenging due to its unculturable nature. The clubroot pathogen must be propagated and conserved in plant tissue, and although various methods have been developed for storage, generating single spore isolates, and propagation (Castlebury et al., 1994; Jones et al., 1982; Lv et al., 2021; MacFarlane, 1970; Tinggal & Webster, 1981; Xue et al., 2008), these methods have not been standardized or compiled.
A distinct physiological response of brassicas to the clubroot pathogen became apparent in the early stages of clubroot research. This response laid the foundation for categorizing P. brassicae isolates as races (Ayers, 1957; Buczacki et al., 1975; Williams, 1966), which are now referred to as pathotypes (Somé et al., 1996; Strelkov et al., 2018). Pathotyping the physiological reactions of different brassica cultivars to various P. brassicae isolates, as well as molecular phenotyping of the isolates themselves, plays a pivotal role in breeding clubroot resistance and selecting suitable germplasm for growers (Pang et al., 2020; Salih et al., 2023; Somé et al., 1996; Strelkov et al., 2018; Williams, 1966). However, challenges remain within the clubroot community, including the phenotyping process itself and basic tasks like DNA extraction from resting spores.
In this article, we have compiled the essential protocols required for working with the clubroot pathogen. Each protocol offers comprehensive instructions for key procedures, including storing, extracting resting spores, and generating single spore isolates. Additionally, we have incorporated a DNA extraction procedure and a PCR protocol for detecting P. brassicae. Our objective is to accelerate clubroot research in newly established labs that are delving into the biology, genomics, and management of the clubroot pathogen. We also aim to standardize procedures across laboratories and ensure reproducibility.
CAUTION: Plasmodiophora brassicae is a Biosafety Level 1 pathogen. Follow all appropriate guidelines and regulations for the use and handling of pathogenic microorganisms.
Basic Protocol 1: EXTRACTION OF Plasmodiophora brassicae RESTING SPORES AND PROPAGATION
As an obligate biotrophic parasite, P. brassicae requires a living host to grow and multiply (Javed et al., 2023). The pathogen's long-lasting resting spores have a half-life of 4 years, although some reports indicate resting spores remain viable for up to 20 years (Wang et al., 2022). During periods of dormancy, the resting spores are resistant to adverse environmental conditions and can survive without a host (Zahr et al., 2021).
Among the various protocols available for extracting P. brassicae resting spores, the methodology employed in this article is one that has been repeated extensively, consistently yielding reliable results (Hossain et al., 2021; Muirhead & Pérez-López, 2022; Pérez-López et al., 2020; Salih et al., 2023). The resting spores are extracted from infected clubroot galls, as these galls contain the highest concentration of inoculum. Maintaining P. brassicae spore suspensions necessitates multiple rounds of spore propagation and extraction. For this process, cruciferous plant seedlings are infected with the desired P. brassicae isolate. When symptoms become apparent at 35 to 40 days post-inoculation (dpi), the galls are extracted and subsequently employed for further experiments (Fig. 1A).
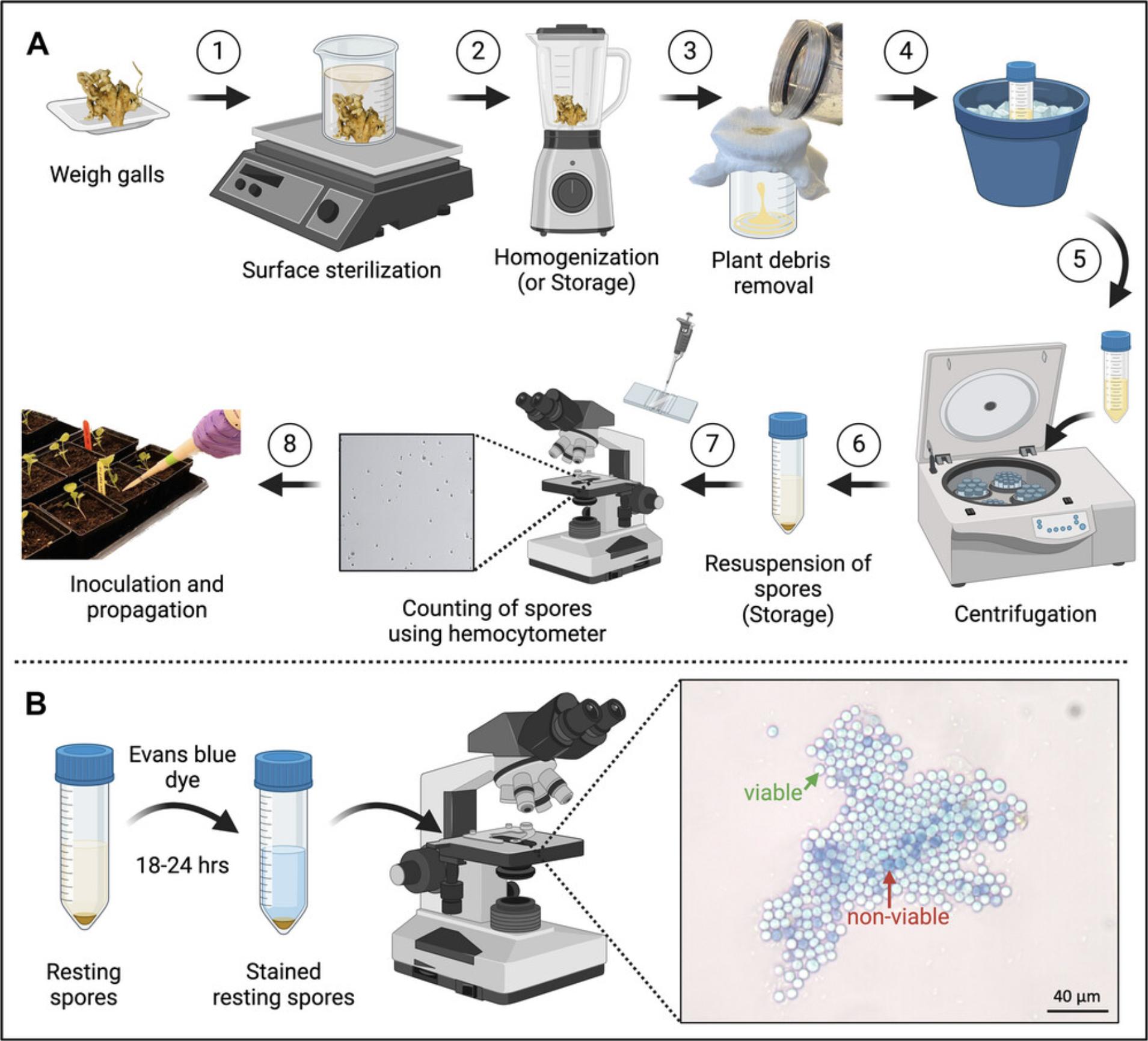
Materials
-
Clubroot galls (e.g., galls obtained from a clubroot-infested canola field in Québec and propagated in clubroot susceptible canola cultivar Westar)
-
0.25% (v/v) Tween 20 (e.g., VWR, cat. no. 9005-64-5) (in water) for surface sterilization of clubroot galls and removing root debris
-
70% (v/v) ethanol (in water) for decontaminating the tabletop, equipment, and surface sterilization of clubroot galls
-
10% (w/v) sucrose (e.g., Millipore Sigma, cat. no. 57-50-1) (in water)
-
Autoclaved double distilled water
-
10-day-old canola Westar seedlings
-
Autoclaved cheese cloth (e.g., Thomas Scientific, cat. no. 2904B25)
-
High-capacity balance (e.g., Sartorius, Cubis II, cat. no. MCA20201S0-S00)
-
Single-channel micropipettes, volume 0.5-10 μl, 20-200 μl, and 100-1000 μl (e.g., Bio-Rad, cat. nos. 1660505EDU, 1660507EDU, and 1660508EDU)
-
Pipette tips, volume 0.5-10 μl, 20-200 μl, and 100-1000 μl (e.g., Thermo Scientific, cat. nos. 9400303 and 9401030, Invitrogen, cat. no. AM12650)
-
600-ml glass beakers (e.g., Fisher Scientific, PYREX cat. no. 02-540M)
-
Weighing dishes (e.g., Fisherbrand Disposable Polystyrene Weighing Dishes, cat. no. S40293)
-
1000-ml measuring cylinder (e.g., Fisherbrand cat. no. 03-007-44)
-
Magnetic stirrer plate (e.g., Fisherbrand Ultra-Thin Magnetic Stirrer)
-
Magnetic stir bars (e.g., VWR Spinbar Magnetic Stir Bars, Octagon, cat. nos. 58948-091, 58948-116, and 58948-218)
-
Forceps (e.g., Fisherbrand General-Purpose Broad-Tipped Forceps, cat. no.10-300)
-
Timer (e.g., Bio-Rad Multi-Function Countdown Timer, cat. no. 305553)
-
Blender (e.g., NutriBullet, cat. no. NB50100C)
-
Conical centrifuge tubes (e.g., Fisherbrand, Corning Falcon 50 ml Conical Centrifuge Tubes, cat. no.14-432-22)
-
Standard hemocytometer (e.g., Weber scientific, cat. no. 3048-12)
-
Light microscope (e.g., Omax, cat. no. SKU: MD827Z30-A195K-A185A)
-
Ice bucket (e.g., 4 L, Fisherbrand, cat. no. 03395162)
-
Ice maker (e.g., Fisherbrand, CurranTaylor Scotsman NUGGET, cat. no. 09540001)
-
Centrifuge (e.g., Thermo Scientific Megafuge 8 Small Benchtop Centrifuge Series cat. no. 75007210)
-
Conical centrifuge tube rack (e.g., Fisherbrand, cat. no. 21-200-285)
-
Glass slides (e.g., Fisherbrand Bev-L-Edge Slides cat. no. 12-549-5C)
-
Cover slips (e.g., Fisherbrand, cat. no. S17525B)
1.Weigh (e.g., 4 g clubbed canola roots) and submerge the root in a glass beaker containing 0.25% Tween 20 (v/v), add a magnetic stir bar, and transfer to a magnetic stirrer for 6 min.
2.Using forceps, remove the gall from the beaker into a clean weighing dish and discard the remaining solution. Place the galls in a clean beaker and submerge them into 70% (v/v) ethanol. Add a magnetic stir bar and transfer to a magnetic stirrer for 6 min.
3.Using forceps, remove the gall from the beaker into a clean weighing dish and discard the remaining solution. Place the galls in a clean beaker and submerge in ddH2O. Add a magnetic stir bar and transfer to a magnetic stirrer for 6 min.
4.Repeat step 3 once.
5.Place the surface-sterilized galls in the sterile glass jar of a blender. Add 10% sucrose (w/v) and grind the galls until a homogenized mixture is formed. The duration depends on whether a mortar or blender is used.
6.Pass the resulting emulsion through an autoclaved eight-layered cheesecloth attached to a glass beaker (the liquid suspension must be maintained on ice).
7.Transfer the passthrough to a 50-ml conical centrifuge tube and centrifugate the solution at 300 rpm and 4°C for 6 min. Transfer the supernatant to a clean 50-ml conical centrifuge tube.
8.Centrifuge the supernatant at 4500 rpm and 4°C for 10 min to separate the pellet containing P. brassicae resting spores. Discard the supernatant by decanting.
9.Wash the resulting pellet twice with 3-5 ml ddH2O, centrifuge at 4500 rpm and 4°C for 10 min, and re-suspend in ddH2O.
10.Place 10 μl of the stained spores onto a sterile glass slide, cover with a cover slip, and count under a light microscope at 40× magnification.
Support Protocol 1: EVANS BLUE STAINING TO IDENTIFY RESTING SPORE VIABILITY
Evans blue staining is a standard method for assessing cell viability (Gaff & Okong'O-Ogala, 1971). This assay has been used to identify viable and non-viable resting spores, as non-viable spores stain blue and viable spores remain colorless (Gaff & Okong'O-Ogala, 1971). This assay has been reported and modified by several groups (Harding et al., 2019); however, we use the most straightforward approach to assess the viability of a spore suspension stored at −20°C (Fig. 1B) (Wang et al., 2022).
Materials
-
Evans blue (≥85% dye content) 10 mg/ml (VWR, cat. no. BT134155-10G)
-
P. brassicae resting spore suspension
-
1.5- and 2-ml microcentrifuge tubes (e.g., Thermo Scientific, cat. nos. 3451, 3453)
-
Glass slides (e.g., Fisherbrand Bev-L-Edge Slides cat. no. 12-549-5C)
-
Cover slips (e.g., Fisherbrand, cat. no. S17525B)
-
Single-channel micropipettes, volume 0.5-10 μl, 20-200 μl, and 100-1000 μl (e.g., Bio-Rad, cat. nos. 1660505EDU, 1660507EDU, and 1660508EDU)
-
Pipette tips, volume 0.5-10 μl, 20-200 μl, and 100-1000 μl (e.g., Thermo Scientific, cat. nos. 9400303 and 9401030, Invitrogen, cat. no. AM12650)
-
Light microscope (e.g., Omax, cat. no. SKU: MD827Z30-A195K-A185A)
1.In a 1.5-ml microcentrifuge tube, mix 500 μl resting spore suspension with 500 μl of 10 mg/ml Evans blue dye.
2.Mix thoroughly and incubate at room temperature for 18 to 24 hr.
Support Protocol 2: STORAGE OF Plasmodiophora brassicae
The primary source of P. brassicae resting spores is the galls induced by the pathogen in the roots of susceptible hosts. Resting spores can be extracted and stored in autoclaved distilled water at −20°C. Galls can be surface-sterilized and stored at −20°C for short-term use and can be preserved at −80°C for long-term use (Fig. 1). Spores stored using these techniques have been shown to remain viable even after a year at −20°C, highlighting the effectiveness of the methodology presented below.
Materials
-
0.25% (v/v) Tween 20 (e.g., VWR, cat. no. 9005-64-5) (in water) for surface sterilization of clubroot galls and removing root debris
-
70% (v/v) ethanol (in water) for decontaminating the tabletop and equipment and for surface-sterilization of clubroot galls
-
Autoclaved double distilled water
-
Resting spore solution of Plasmodiophora brassicae (see protocol)
-
Clubroot galls
-
1.5- and 2-ml microcentrifuge tubes (e.g., Thermo Scientific, cat. nos. 3451, 3453)
-
Single-channel micropipettes, volume 0.5-10 μl, 20-200 μl, and 100-1000 μl (e.g., Bio-Rad, cat. nos. 1660505EDU, 1660507EDU, and 1660508EDU)
-
Pipette tips, volume 0.5-10 μl, 20-200 μl, and 100-1000 μl (e.g., Thermo Scientific, cat. nos. 9400303 and 9401030, Invitrogen, cat. no. AM12650)
-
600-ml glass beakers (e.g., Fisher Scientific, PYREX cat. no. 02-540M)
-
Weighing dishes (Fisherbrand Disposable Polystyrene Weighing Dishes cat. no. S40293)
-
1000-ml measuring cylinder (e.g., Fisherbrand cat. no. 03-007-44)
-
Magnetic stirrer plate (e.g., Fisherbrand Ultra-Thin Magnetic Stirrer)
-
Magnetic stir bars (e.g., VWR Spinbar Magnetic Stir Bars, Octagon, cat. nos. 58948-091, 58948-116, and 58948-218)
-
Forceps (e.g., Fisherbrand General-Purpose Broad-Tipped Forceps, cat. no.10-300)
-
Timer (Bio-Rad Multi-Function Countdown Timer, cat. no. 305553)
-
Zipper seal bags (e.g., Dimensions 17.7 cm × 18.8 cm, Ziploc)
-
Permanent markers (e.g., Sharpie)
-
Cardboard cryogenic storage boxes to store microcentrifuge tubes containing P. brassicae resting spores (e.g., Thomas Scientific, cat. no. 1159R84)
-
TSX Series High-Performance −20°C Manual Defrost Freezer (e.g., Thermo Scientific, cat. no. TSX2320FV)
1.For resting spore storage, use a 1000-μl micropipette to transfer a total of 1500 μl or a desired volume of resting spore suspension into 2- or 1.5-ml microcentrifuge tubes and label accordingly. Place tubes in a cryogenic storage box and store at −20°C.
2.For gall storage, place the clubroot galls in a glass beaker, add water and an appropriate volume of Tween 20 (e.g., for 300 ml water, add 75 μl Tween 20). Insert a magnetic stir bar and place on the magnetic stirrer for 6 min.
3.Using forceps, transfer the galls to a clean weighing dish and discard the Tween 20.
4.Add 70% (v/v) ethanol to the beaker and replace the galls, insert a magnetic stir bar, and place on the magnetic stirrer for 6 min.
5.Transfer the galls to a sterile weighing dish and discard the ethanol.
6.Put the clean galls back into the clean beaker, add double-distilled water and a magnetic stir bar, and place the beaker back on the magnetic stirrer for 6 min.
7.Repeat Step 6 once.
8.Discard the water and transfer the galls to a sterile double zip seal bag, label accordingly, and place at −20°C for long-term storage.
Basic Protocol 2: GENERATION OF SINGLE SPORE ISOLATES FROM Plasmodiophora brassicae FIELD ISOLATES
One of the most significant limitations associated with clubroot disease management is the emergence of novel P. brassicae pathotypes with distinct virulence profiles. These pathotypes pose a challenge to the utilization of genetic resistance as the preferred strategy for managing clubroot, highlighting the need for a deeper understanding of genetic diversity in the field and the mechanisms employed by these new isolates to overcome resistance (Wu & Pérez-López, 2023). To achieve this, generating single spore isolates (SSIs) is crucial. These isolates can be subsequently propagated and phenotyped. For instance, they can be used to rapidly test new brassica varieties for breeding purposes or employed in genome-wide association studies to identify new clubroot-resistance genes or the interactive partners within the clubroot pathogen that triggers resistance (Javed et al., 2023; Wu & Pérez-López, 2023). Several methods have been put forward and are in use to generate SSIs (Jones et al., 1982; Lv et al., 2021; Tinggal & Webster, 1981; Voorrips, 1996; Xue et al., 2008). After trying several variations of some of these protocols, we have successfully implemented the methodology developed by Lv et al. (2021) with minor modifications (Fig. 2).
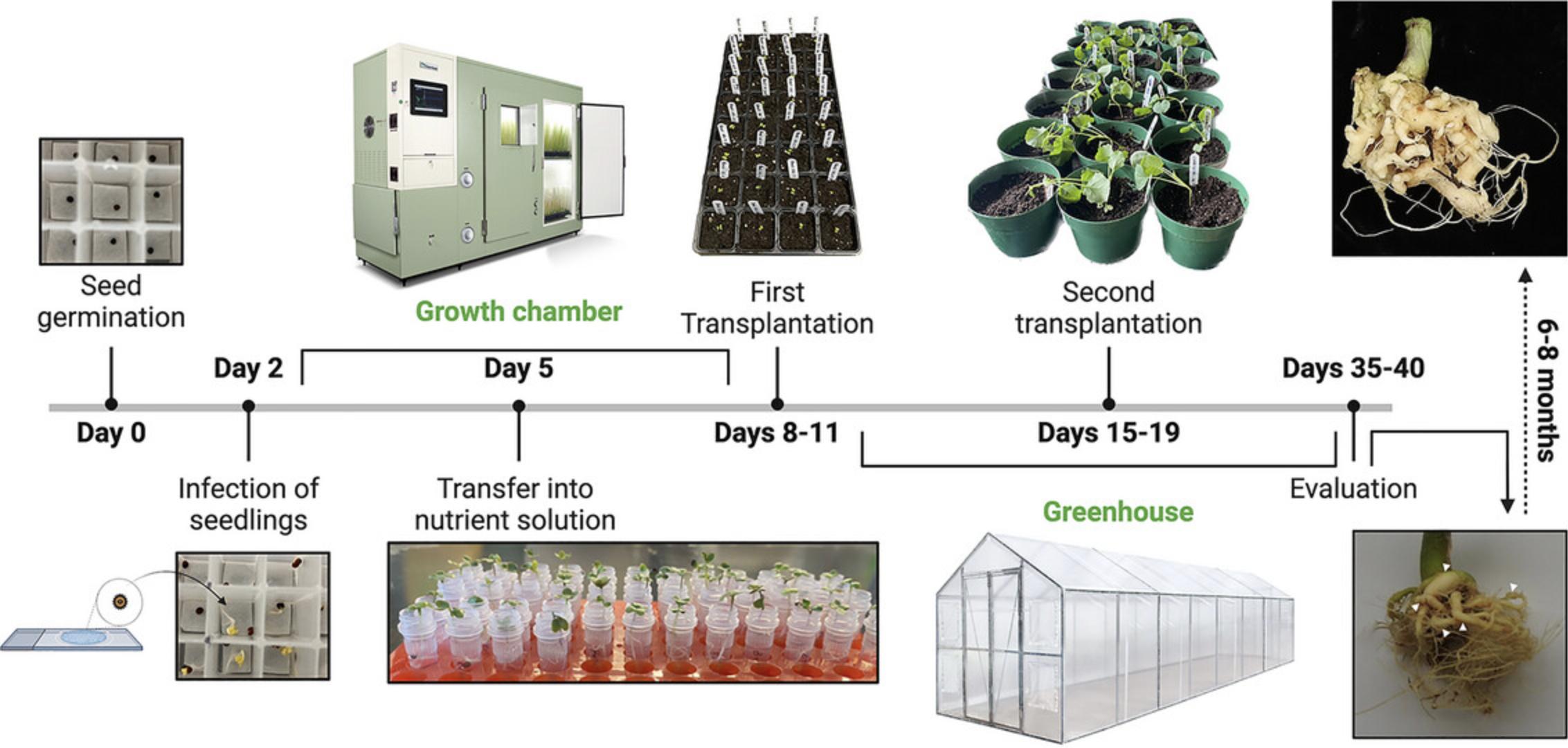
Materials
-
Westar canola seeds (or seeds of any host plant)
-
Nutrient solution (20-20-20 + Epsom salt, MgSO4.7H2O, mix)
-
VERANDA Container Mix (Fafard, https://www.fafard.ca/)
-
Pro-Mix BX mycorrhizae lose soil (PRO-MIX, https://www.pthorticulture.com)
-
1% (w/v) bacteriological agar (in water) (e.g., Sigma-Aldrich, cat. no. A5306-250G)
-
Cryobox (e.g., Thermo Scientific, cat. no. 330821)
-
Sterile Whatman filter paper (e.g., Scienceware cat. no. Z177601)
-
Aluminum foil (e.g., Thomas Scientific, cat. no. 1181K82)
-
Forceps (e.g., Fisherbrand General-Purpose Broad-Tipped Forceps, cat. no.10-300)
-
Weighing dishes (e.g., Fisherbrand Disposable Polystyrene Weighing Dishes cat. no. S40293)
-
1.5- and 2-ml microcentrifuge tubes (e.g., Thermo Scientific, cat. nos. 3451, 3453)
-
Glass slides (e.g., Fisherbrand Bev-L-Edge Slides cat. no. 12-549-5C)
-
Light microscope (e.g., Omax, cat. no. SKU: MD827Z30-A195K-A185A)
-
Transfer pipette (e.g., Uline, model no. S-24320)
-
Glass slides (e.g., Fisherbrand Bev-L-Edge Slides cat. no. 12-549-5C)
-
Single-channel micropipettes, volume 0.5-10 μl, 20-200 μl, and 100-1000 μl (e.g., Bio-Rad, cat. nos. 1660505EDU, 1660507EDU, and 1660508EDU)
-
Pipette tips, volume 0.5-10 μl, 20-200 μl, and 100-1000 μl (e.g., Thermo Scientific, cat. nos. 9400303 and 9401030, Invitrogen, cat. no. AM12650)
-
32-cell seedling trays (e.g., Boots-trap-farmer, https://indoorfarmer.ca)
-
Humidity domes with vents (e.g., Boots-trap-farmer, https://indoorfarmer.ca)
-
Seedling pot (e.g., Boots-trap-farmer, https://indoorfarmer.ca)
1.Place surface-sterilized Westar canola seeds in a compartment of a cryobox with wet filter paper on the bottom.
2.Wrap the cryobox with aluminum foil to ensure darkness and place at room temperature until infection.
3.After two days, infect each seedling with a single spore of P. brassicae. For infection, please follow these steps:
4.Prepare 1% bacteriological agar and place 80 μl of the solution on a sterile glass slide.
5.While the agar solidifies, dilute a P. brassicae spore suspension in distilled water and adjust the spore concentration to approximately 2 × 103 spores/ml using a hemacytometer.
6.Carefully place 0.5 μl of the diluted spore suspension on the center of the solidified agar.
7.Scan the agar block using a microscope at 40× magnification to locate a single spore that is well-separated from the others.
8.Mark the position of the single spore using a marker pen on the back of the glass slide.
9.Carefully excise the section of the agar block containing the single spore with a sterile scalpel.
10.Place the agar section containing the single spore inverted on the root hairs of the germinated seed so that the spore side touches the roots.
11.Keep the infected seedlings in the cryobox in the dark for 24 hr and transfer to a growth chamber under 12 hr light/12 hr dark, 22°C day, 16°C night, and 60% humidity conditions until the seedlings outgrow the cryobox.
12.Once the seedlings outgrow the cryoboxes (approximately 2 to 3 days of growth), transfer them and the agar blocks containing a single spore to 2-ml tubes, one seedling per tube, filled with distilled water.
13.After one day, replace the distilled water with nutrient solution every other day.
14.From 6-8 dpi, transfer the infected seedlings into compact soil (VERANDA container mix) in a 32-cell seedling tray with one plant per well.
15.Place the tray in the greenhouse covered with a humidity control dome at 25°C and maintain 60% to 70% humidity.
16.At the 5th to 6th true-leaf stage, transplant into individual seedling pots containing PROMIX-BX-Mychorrizae non-pasteurized loose soil in the greenhouse at the above-mentioned conditions.
17.Fertilize the plants with 3 ml nutrient solution every week.
18.At 35-40 dpi, evaluate the plants and observe the roots for any symptoms of clubroot, such as galls in the roots, stunning, and yellowing; harvest and store at −20°C.
Basic Protocol 3: PHENOTYPING OF Plasmodiophora brassicae ISOLATES
Phenotyping P. brassicae isolates serves the purpose of studying and describing the response of different hosts to the pathogen, aiming to comprehend its biology (Buczacki et al., 1975; Pang et al., 2020; Salih et al., 2023; Somé et al., 1996; Strelkov et al., 2018; Williams, 1966). This gathered information aids in revealing differences in virulence and host range. Moreover, phenotyping allows the identification of genetic or environmental factors that impact the pathogen's growth and development, contributing to the formulation of effective management strategies for mitigating infections in Brassica crops. In a recent study published by our group in collaboration with the canola industry, we characterized several inbred homozygous (IH) canola lines using a hydroponic-based phenotyping system and the classic substrate inoculation method (Salih et al., 2023). Here, we elaborate on the substrate inoculation method because it allows phenotyping of the response of different hosts to the clubroot pathogen and facilitates propagation of the inoculum for further analyses.
Materials
-
Westar canola seeds, IH2, and IH4 canola lines seeds
-
70% (v/v) ethanol (in water) for decontaminating the surfaces
-
P. brassicae resting spores (single spore isolate TH2O_05)
-
Nutrient solution (20-20-20 + Epsom salt, MgSO4.7H2O, mix)
-
Single-channel micropipette, volume 100-1000 μl (e.g., Bio-Rad, cat. no. 1660508EDU)
-
VERANDA Container Mix (Fafard, https://www.fafard.ca/)
-
Pro-Mix BX mycorrhizae lose soil (PRO-MIX®, https://www.pthorticulture.com)
-
Autoclaved vermiculite (e.g., Holiday Vermiculite, https://www.perlitecanada.com/)
-
Microgreen tray/seedling tray (e.g., Boots-trap-farmer)
-
32-cell seedling trays (3-in pots) (e.g., Boots-trap-farmer, https://indoorfarmer.ca)
-
Humidity domes with vents (e.g., Boots-trap-farmer, https://indoorfarmer.ca)
-
Seedling pot (8-in pots) (e.g., Boots-trap-farmer, https://indoorfarmer.ca)
1.Surface-sterilize with 70% ethanol and fill the microgreen tray/seedling tray with three rows of autoclaved vermiculite.
2.Place Westar, IH2, and IH4 canola seeds well apart from each other in the vermiculite. Add 10-20 ml water until the vermiculite is completely damped for the seeds to germinate.
3.Cover the starter tray with a humidity dome (vent opened) and place in a growth chamber for 7-10 days under the following conditions: 12 hr light/12 hr dark, 24°C day and 16°C night, 60% humidity.
4.Once the seedlings are ready to be inoculated, transfer to a 32-cell seedling tray containing VERANDA compact soil, one seedling per cell, and add 3 ml nutrient solution.
5.Place in the greenhouse under long day conditions, 23°C and 30% to 50% relative humidity.
6.Two days after transplanting, infect with P. brassicae resting spore suspension. Use a 100-1000 μl micropipette to slowly add 2 ml of 2 × 108 spores/ml suspension to each plant on the soil at the base of the stem (Fig. 1).
7.From 5 to 8 dpi, the plants can be transplanted into individual seedling pots containing Pro-Mix BX mycorrhizae non-pasteurized loose soil and remain in the greenhouse under the above-mentioned conditions.
8.Fertilize each plant once per week with 3 ml nutrient solution.
9.Evaluate canola plants for clubroot symptoms at 35 to 41 dpi by removing the pants from the soil and examining the roots (Fig. 3A), although well-formed galls can be observed in susceptible hosts as early as 28 dpi (Fig. 4).
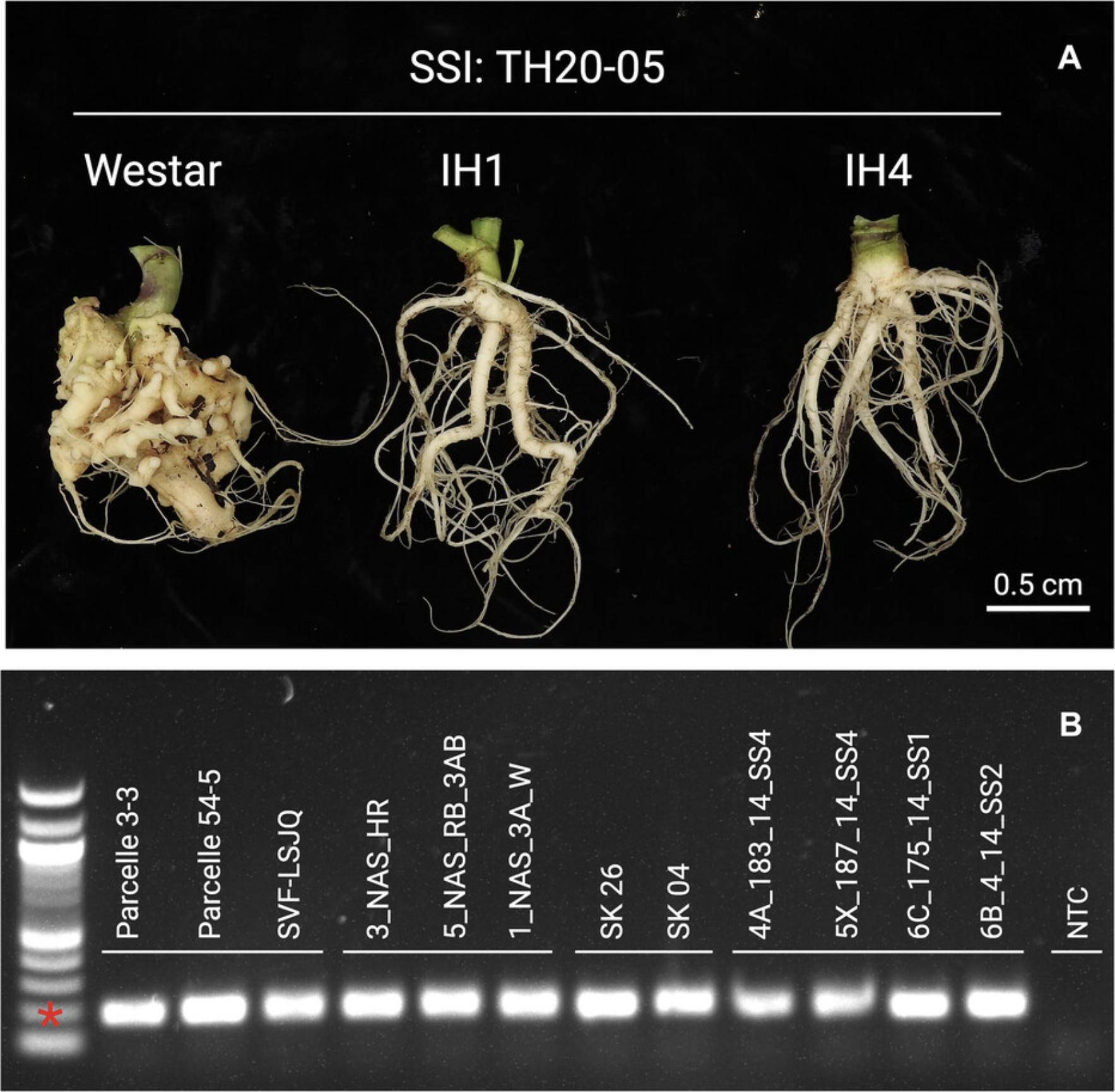
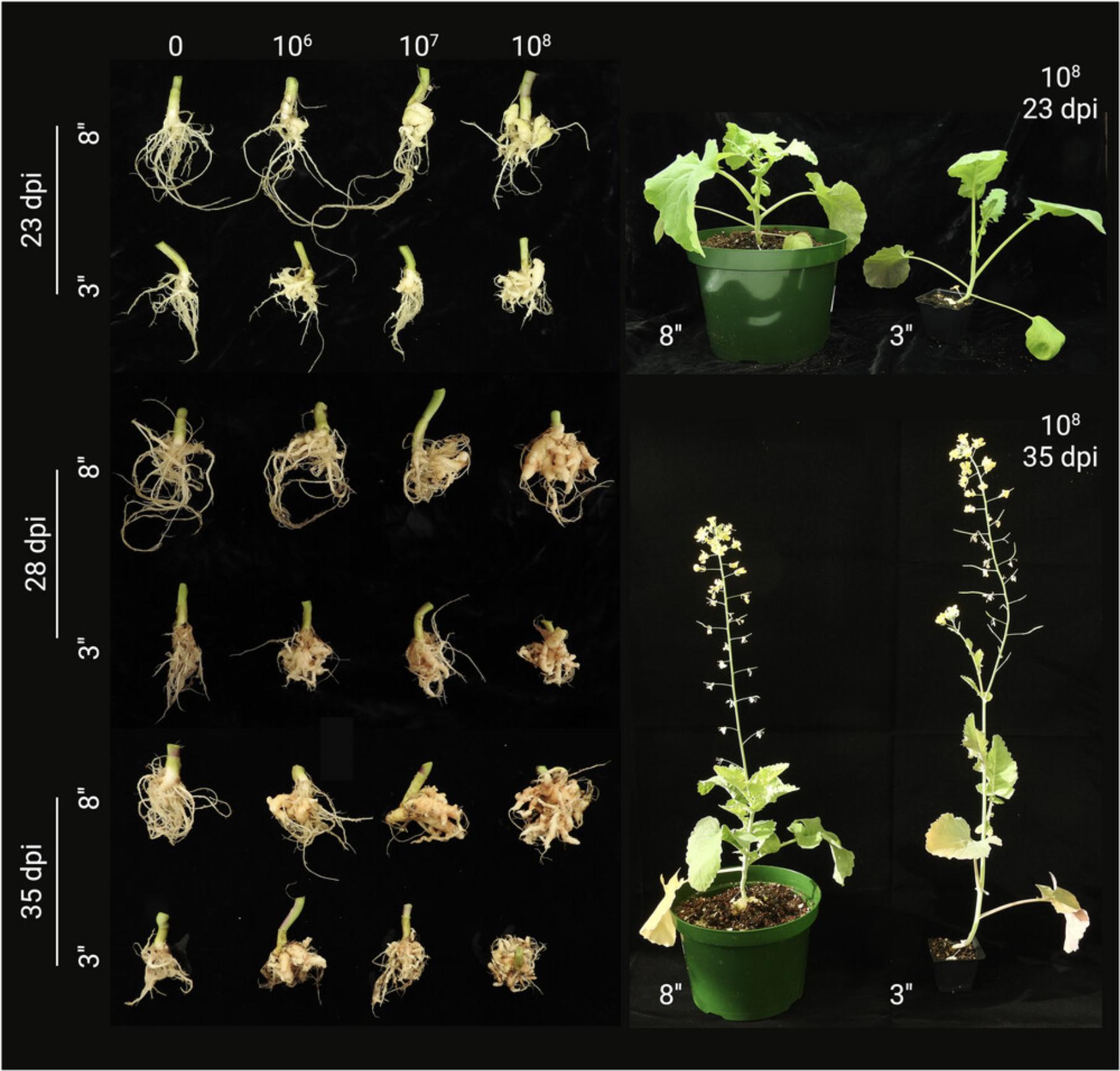
10.Using a 0 to 3 clubroot disease severity scale, rate the canola plants to calculate the disease index: 0, no clubroot symptoms or healthy plant; 1, small clubs or thickening with nodules in the lateral roots; 2, small clubs on the main root; and 3, severe galling in the roots (Figs. 2 and 3).
Basic Protocol 4: GENOMIC DNA EXTRACTION FROM Plasmodiophora brassicae RESTING SPORES
P. brassicae genomic DNA can be obtained from the resting spores. However, extraction of the clubroot pathogen DNA is challenging due to the expected plant DNA contamination. In principle, any method for extracting plant DNA can be used. In our laboratory, we implemented an acetyl trimethyl ammonium bromide (CTAB)-based protocol, but several modifications have been incorporated to enhance the ratio of clubroot pathogen DNA to plant DNA.
Materials
-
CTAB buffer (CTAB 2%, Tris·HCl 100 mM, EDTA 20 mM, NaCl 1.4 M)
-
Cold ethanol 70% (v/v) ethanol (in water) (kept in ice)
-
Cold isopropanol (2-propanol) (kept in ice)
-
Cold 24:1 (v/v) chloroform: isoamyl alcohol
-
Resting spore suspension at 2 × 108 spores/ml
-
2.8-mm Ceramic beads (e.g., OMNI International, cat. no. SKU:19-646)
-
Sterile distilled water
-
1.5- and 2-ml microcentrifuge tubes (e.g., Thermo Scientific, cat. nos. 3451, 3453)
-
Single-channel micropipettes, volume 0.5-10 μl, 20-200 μl, and 100-1000 μl (e.g., Bio-Rad, cat. nos. 1660505EDU, 1660507EDU, and 1660508EDU)
-
Pipette tips, volume 0.5-10 μl, 20-200 μl, and 100-1000 μl (e.g., Thermo Scientific, cat. nos. 9400303 and 9401030, Invitrogen, cat. no. AM12650)
-
Permanent markers (e.g., Sharpie)
-
Microtube rack (e.g., Bio Plas, cat. no. 22-313630)
-
Microbiological Incubator (e.g., Fisherbrand, cat. no. 150152633)
-
Omni grinder/Multi sample Homogenizer (e.g., Fisherbrand, cat. no. 15-340-163)
-
Timer (e.g., Bio-Rad Multi-Function Countdown Timer, cat. no. 305553)
-
Forceps (e.g., Fisherbrand General-Purpose Broad-Tipped Forceps, cat. no.10-300)
-
500-ml glass bottle with lid (e.g., Pyrex, cat. no. 1743E05)
-
Vortex mixer (e.g., Fisherbrand, cat. no. 02-215-414)
-
Mini centrifuge (e.g., Scilogex, cat. no. 1205R41)
-
Water bath (e.g., Thermo Scientific, cat. no. TSGP02)
-
Centrifuge (e.g., Thermo Scientific Megafuge 8 Small Benchtop Centrifuge Series cat. no. 75007210)
-
Fluorometer (e.g., Invitrogen Qubit 4 Fluorometer cat. no. Q33238)
-
DNA quantification buffer and standards (e.g., Qubit dsDNA Quantification Assay Kit cat. no. Q32850)
1.Transfer 1.5 ml P. brassicae resting spore suspension (2 × 108 spores/ml) to a 2-ml microcentrifuge tube and centrifuge at 14,000 × g and room temperature for 5 min.
2.Discard the supernatant by decanting and add 2 ceramic beads (2.8 mm diameter) and 600 μl CTAB buffer heated to 60-65°C.
3.Grind with the Omni grinder under the following conditions to break the spore cell wall.
1.S = 6 m/s 2.T = 2 × 40 s 3.Pause = 30 s
4.Add 500 μl CTAB buffer and vortex well, then spin down in the mini centrifuge.
5.Incubate the tubes at 65°C for 30 min, and centrifuge at 14,000 × g and room temperature for 10 min.
6.Take approximately 700 μl of the supernatant and transfer to a clean 1.5-ml tube. Add an equal volume of 24:1 (v/v) chloroform: isoamyl alcohol, and mix well by vortexing.
7.Centrifuge at 14,000 × g and room temperature for 10 min.
8.Transfer the upper aqueous phase to a new tube (approximately 500 μl).
9.Add 0.7× volume ice-cold isopropanol and mix well by inverting the tube 10 times.
10.Incubate at −20°C for 30 min.
11.Centrifuge the samples at 14,000 × g and 4°C for 5 min to recover the genomic DNA. Remove the supernatant by decanting slowly.
12.Carefully add 500 μl of ice-cold 70% ethanol.
13.Centrifuge at 14,000 × g for 5 min at 4°C, although room temperature should work fine given the stability of genomic DNA.
14.Discard the supernatant by decanting and repeat steps 12-14.
15.Place the tubes upside down on the bench for ∼10 min to allow the residual ethanol to evaporate.
16.Resuspend the pellet in 20-100 μl sterile distilled water and mix well to dissolve the DNA.
17.Quantify DNA concentration using the Qubit DNA quantification kit and fluorometer according to the manufacturer's recommendations to be sure the DNA concentration is as expected.
18.Store the samples at −20°C.
Basic Protocol 5: MOLECULAR DETECTION OF Plasmodiophora brassicae
Although clubroot symptoms are clear and easy to recognize when plants are infected with a large number of resting spores (Fig. 1A), these are hardly perceivable when the pathogen titer is low (Fig. 2B). In these scenarios, we must resort to molecular methods such as PCR (Javed et al., 2023). Among several other molecular targets, the 18S subunit of the ribosomal DNA has been identified as suitable for studying the evolutionary relationships between P. brassicae isolates from different geographic locations (Faggian et al., 1999). However, the 18S rDNA operon has been also used as a target to quantify and detect the clubroot pathogen (Lemarié et al., 2015; Padrón et al., 2022).
Materials
-
Forward primer (10 µM) - Pb18sF: 5'-AAACAACGAGTCAGCTTGAATGC-3'
-
Reverse primers (10 µM) - Pb18sR - 5'-AGGACTTGGCTGCGGATCAC-3'
-
P. brassicae gDNA
-
Deoxyribonucleotide triphosphate (dNTP) mix (e.g., Thermo Scientific, cat. no. R0181)
-
Phusion High-Fidelity DNA Polymerase (e.g., Thermo Scientific, cat. no. F530L)
-
Thermal cycler (e.g., Bio-Rad T100 Thermal Cycler, cat. no 1861096)
-
Preparation rack, 96-well (e.g., Thermo Scientific, cat. no. PRK0963)
-
PCR tubes (e.g., VWR, 8 strip PCR, cat. no. 53509-304)
-
1.5- and 2-ml microcentrifuge tubes (e.g., Thermo Scientific, cat. nos. 3451, 3453)
-
Single-channel micropipettes, volume 0.5-10 μl, 20-200 μl, and 100-1000 μl (e.g., Bio-Rad, cat. nos. 1660505EDU, 1660507EDU, and 1660508EDU)
-
Pipette tips, volume 0.5-10 μl, 20-200 μl, and 100-1000 μl (e.g., Thermo Scientific, cat. nos. 9400303 and 9401030, Invitrogen, cat. no. AM12650)
-
Ice bucket (e.g., 4 L, Fisherbrand, cat. no. 03395162)
-
Ice maker (e.g., Fisherbrand, CurranTaylor Scotsman NUGGET, cat. no. 09540001)
-
Centrifuge (e.g., Thermo Scientific Megafuge 8 Small Benchtop Centrifuge Series cat. no. 75007210)
-
Vortex mixer (e.g., Fisherbrand, cat. no. 02-215-414)
-
Fluorometer (e.g., Invitrogen Qubit 4 Fluorometer cat. no. Q33238)
-
Qubit dsDNA Quantification Broad Range Kit (Thermo Scientific, cat. no. Q32850)
-
Agarose (e.g., Thermo Scientific, UltraPure Agarose, cat. no. 16500500)
-
DNA stain (e.g., Thermo Scientific, SYBR Safe DNA Gel Stain, cat. no. S33102)
-
DNA ladder (e.g., Thermo Scientific, GeneRuler 100 bp Plus DNA Ladder, cat. no. SM0321)
-
TBE buffer (Prepare yourself or buy, e.g., Thermo Scientific, TBE Buffer 1×, cat. no. YBP24304)
1.Thaw all frozen components on ice. Mix thoroughly and centrifuge, and keep everything on ice.
2.Prepare the master mix: for a 20 µl reaction, add 11.4 µl nuclease-free H2O, 4 µl of 5× Phusion HF buffer, 1 µl forward primer Pb18sF (10 µM), 1 µl reverse primer Pb18sR (10 µM), 0.6 µl DMSO (optional, it can increase the efficiency of the polymerase reaction. If not added, increase H2O volume to 12 µl), 0.2 µl Phusion DNA polymerase (2 U/µl), and 2 µl P. brassicae gDNA (∼1 ng/µl).
3.Dispense the master mix into PCR tubes (18 µl/tube) and add template. Spin the tubes to remove any bubbles and ensure the reaction mixture is at the bottom of the tube.
4.Program the thermal cycler: 1 min at 95°C (1×); 30 s at 95°C, 30 s at 61°C, and 20 s at 72°C (×35); 5 min at 72°C (1×); and hold at 4°C.
5.Visualize the expected ∼200 bp PCR product using a 1.5 % agarose gel. Weigh 1 g agarose for 100 ml of TBE 0.5×, melt it in the microwave, and when it is warm, add 5 µl SYBR Safe 10,000×. Include the 100 bp plus DNA ladder in one of the gel lanes as a reference (Fig. 3B).
COMMENTARY
Background Information
The earliest experiments involving P. brassicae focused on identifying and studying the pathogen's characteristics and its impact on Brassica crops (Cook & Swartz, 1930; Woronin, 1878). This encompassed the examination of infection symptoms, the two phases of the pathogen's life cycle, and strategies for managing infections in agricultural lands (Ayers, 1944; Karling, 1968; McFarlane, 1970). Researchers have developed various host differential systems to assess the virulence of P. brassicae isolates. This approach aids in implementing appropriate management strategies and speeding up the breeding and selection of resistant crops (Buczacki et al., 1975; Somé et al., 1996; Strelkov et al., 2018; Williams, 1966). The foundation of most experiments involving the clubroot pathogen depends on the quality and quantity of resting spores, the generation of SSIs, and the quality of the pathogen DNA.
Common techniques for preserving clubroot samples include drying and freezing (Fennell, 1960). Scientific literature discussing various storage methods for clubbed roots and P. brassicae spore suspensions is limited. However, there is evidence supporting the viability of resting spores after 2 years of storage at −20°C (Zahr et al., 2021). Multiple freeze-thaw cycles could adversely affect spore viability in galls, a problem avoided by using dried roots, which are typically stored at 4°C for short-term usage (Harding et al., 2019). Numerous techniques are available to assess the viability of P. brassicae spores, particularly for samples stored over extended periods. This step is crucial before using long-term stored samples. Evans blue staining is a reliable method for estimating spore viability. The azo dye penetrates damaged or destabilized membranes, staining the cytoplasm of dead cells blue, while viable spores remain colorless (Fig. 1B) (Harding et al., 2019; Wang et al., 2022). In addition, qPCR-based assays coupled with propidium monoazide (PMA), a membrane-impermeable dye, are also employed to estimate viability (Al-Daoud et al., 2017).
The extraction and purification of viable P. brassicae resting spores from host tissue dates to the 1990s (Castlebury et al., 1994). Most of these protocols are optimized to eliminate plant debris and other contaminants. The objective is to obtain a viable resting spore suspension suitable for DNA extraction and long-term storage or inoculation. Many spore extraction methods involve extensive purification steps, such as subjecting the spore pellet to density gradients (Cao et al., 2006). Additionally, filter sterilization is another method for spore extraction. Fresh clubroot samples are homogenized and filtered multiple times through layered cheesecloth until all debris is removed (Pérez-López et al., 2020; Salih et al., 2023; Zamani-Noor, 2016). The method outlined in this article encompasses all these steps, optimizing the yield of resting spores from clubbed roots (Fig. 1A).
Several agar-based methods have been developed to generate P. brassicae single spore isolates (Jones et al., 1982; Tinggal & Webster, 1981; Xue et al., 2018). These methods have undergone numerous modifications. The initial liquid paraffin-based method had a very low success rate, with only two infected plants observed out of 250 (Buczacki, 1977). Recently, Xue et al. (2018) proposed a root dip method with an infection rate ranging from 0%-16%, while other methods using petri dishes with agar or wetted filter reported a success rate of 20% to 30% (Askarian et al., 2020; Jones et al., 1982). Acknowledging the limitations of these protocols, Lv et al. (2021) introduced an improved technique, where plants are initially grown in a cryobox and then infected with a slice of agar containing a single spore, significantly enhancing efficiency. In our lab, this protocol has shown infection success rates ranging from 35% to 50% (Fig. 2).
Obtaining high-quality genomic DNA from P. brassicae has been a persistent challenge. Since the pathogen cannot be cultured outside the host plant, the extracted DNA always contains host DNA and other saprophytic microbes, potentially interfering with subsequent steps. Despite these challenges, protocols have been developed to extract high-quality DNA. Many column-based methods are in use (Daval et al., 2020). However, compared to other methodologies, the modified CTAB extraction method presented here has consistently yielded higher DNA concentrations (Doyle & Doyle, 1990; Sedaghatkish et al., 2019).
Critical Parameters and Troubleshooting
Several critical factors influence the protocol presented here and require special attention. These are listed below. We also provide a troubleshooting guide for the generation of SSIs and the maintenance and propagation of galls (Table 1).
Problem | Possible cause | Solution |
---|---|---|
Low infectivity of resting spores | Freezing and thawing |
Keep two sets of galls for each isolate: a working gall, and a backup gall always at −20°C. Avoid using frozen spore suspension, as virulence will be reduced. Prepare fresh suspensions or store at 4°C and use within 7 days. |
Long storage | Keep frozen galls at −20°C no longer 3-4 months. Propagate and replace with fresh galls to avoid reduced infectivity. | |
Small galls during propagation | Size of the pots | Use 8” pots or larger for propagation to allow root development and larger galls |
Low spore concentration | Use spore concentrations ≥108 spores/ml to ensure large galls | |
Evaluation time | Wait ≥35 days post-inoculation to evaluate plants and obtain large galls | |
Low DNA concentration | Low resting spore concentration | Extract DNA from ≥108 spores/ml |
CTAB buffer was not preheated to 60-65°C | Preheat CTAB buffer at 65°C before mixing with resting spores | |
Short incubation at 65°C | Incubate ground spores with CTAB buffer for at least 30 min at 65°C; incubation can be extended to 60 min if necessary. |
Clubroot galls stored long-term at 4°C can easily become contaminated with other microorganisms, leading to unreliable data. Another factor to consider is the potential impact of multiple freeze-thaw cycles on spore viability. Cross-contamination is a common issue during spore extraction. Ensure that your workstation is properly surface-sterilized. If you need to extract spores from multiple clubroot samples, take precautions to disinfect all glassware, blenders, forceps, and other tools. Remember to change your gloves between each sample.
To ensure success during the generation of SSIs, it is important to check the viability of the seeds before placing them in the cryobox. Since the infection rate is less than 50%, it's advisable to maximize the number of plants used. You can assess seed viability by placing them in a beaker of water and allowing them to soak for 15 min. Discard any seeds that float, as they are unlikely to germinate. Furthermore, make sure that the filter paper lining the bottom of the compartments in the cryobox remains moist until the seedlings are removed.
Phenotyping of the clubroot pathogen relies on the pathogen's virulence and the host's resistance. It is crucial to ensure that the plants are healthy and well-fertilized before infection, as stressed plants can lead to non-conclusive phenotypes. For example, a study conducted by Gazengel et al. (2021) reported that nitrogen deficiency in Brassica napus can result in reduced disease symptoms and pathogen content in the host tissues. It is equally important to have an adequate inoculum density to observe infection. Multiple studies have consistently shown that a spore concentration of 107 or 108 spores/ml leads to the development of pronounced disease symptoms (Fig. 4).
Understanding the Results
When assessing if the plants have been infected by the single spores, it is important to pay close attention to the roots and the presence of small galls or thickening of the main root (Fig. 2). These symptoms may go unnoticed by unexperienced eyes but are clear evidence of the infection and generation of SSIs. After this step, several rounds of propagation will follow. We recommend making microscopic observations during each round to verify the presence of resting spores and, if possible, assess the viability of the spores using the Evans Blue staining described above.
Similarly, during the propagation and phenotyping of the clubroot pathogen, it is important to include a susceptible control during each experiment (Fig. 4). It is well known that factors like temperature, humidity, pH, and soil microbiome can affect the development of clubroot disease (Daval et al., 2020; Javed et al., 2023). This is why the inclusion of a susceptible host will contribute to a better understanding of the results when (i) replicates are not consistent among them; (ii) the size of galls is smaller than expected; and (iii) virulent P. brassicae isolates show reduced virulence or are avirulent against other previously susceptible lines, varieties, or hosts.
Extraction of genomic DNA from the clubroot pathogen has been performed since the 1980s (Bernatzky and Tanksley, 1986) with various protocols, some of which are very similar to the one described (Schwelm et al., 2015; Sedaghatkish et al., 2019). However, commercial kits such as the Nucleospin Plant II Kit (MACHEREY-NAGEL, cat. no. 740772.10) have been successfully used by other research groups (Daval et al., 2020). We have tried this kit, and the quality and quantity of the DNA obtained are lower than those achieved with the CTAB-based method described here.
Time Considerations
The generation of P. brassicae SSIs is challenging, with an infection rate of less than 50%. It is also extremely time-consuming, taking 30-40 days until the observation of root thickening and small galls (Fig. 2). From this point, the generation of large galls can take 6-8 months (Fig. 2). Although spore extraction takes no longer than 30 min, propagation of the pathogen for storage or phenotyping will take 45-55 days from host germination to symptom evaluation (Fig. 4).
DNA extraction from P. brassicae resting spores can be performed in 2.5 hr; however, overnight precipitation at −20°C (total processing time 18-20 hr) will increase yield.
Author Contributions
Rasha Salih : Conceptualization; investigation; methodology; writing original draft. Muhammad Asim Javed : Investigation; methodology. Anne-Sophie Brochu : Investigation; methodology. Priyavashini Prakash : Investigation; methodology. Jean-Dominic Côté : Investigation; methodology. Edel Pérez-López : Conceptualization; funding acquisition; methodology; editing original draft.
Acknowledgments
This project was supported by NSERC Discovery program, grant RGPIN-2021-02518; the Canola Agronomic Research Program grant 2021.4; and Mitacs for supporting Priyavashini Prakash scholarship through the program Globalink. We would like to thank Coreen Franke and Stephen Strelkov for their support with canola seeds and P. brassicae single spore isolates, respectively.
Conflict of Interest
Authors declare no conflict of interest.
Open Research
Data Availability Statement
This study generated no data, so nothing was deposited in databases or collections. DNA or resting spores of the clubroot pathogen are available from the corresponding author upon request.
Literature Cited
- Al-Daoud, F., Gossen, B. D., Robson, J., & McDonald, M. R. (2017). Propidium monoazide improves quantification of resting spores of Plasmodiophora brassicae with qPCR. Plant Disease , 101, 442–447. https://doi.org/10.1094/PDIS-05-16-0715-RE
- Askarian, H., Akhavan, A., Manolii, V. P., Cao, T., Hwang, S. F., & Strelkov, S. E. (2020). Virulence spectrum of single-spore and field isolates of Plasmodiophora brassicae able to overcome resistance in canola (Brassica napus). Plant Disease , 105, 43–52. https://doi.org/10.1094/PDIS-03-20-0471-RE
- Ayers, G. W. (1957). Races of Plasmodiophora brassicae. Canadian Journal of Botany , 35, 923–932. https://doi.org/10.1139/b57-076
- Buczacki, S. T., Toxopeus, H., Mattusch, P., Johnston, T. D., Dixon, G. R., & Hobolth, L. A. (1975). Study of physiologic specialization in Plasmodiophora brassicae : Proposals for attempted rationalization through an international approach. Transactions of the British Mycological Society , 65, 295–303. https://doi.org/10.1016/S0007-1536(75)80013-1
- Buczacki, S. T. (1977). Root infections from single resting spores of Plasmodiophora brassicae. Transactions of the British Mycological Society , 69, 328–329. https://doi.org/10.1016/S0007-1536(77)80059-4
- Bulman, S., Candy, J. M., Fiers, M., Lister, R., Conner, A. J., & Eady, C. C. (2011). Genomics of biotrophic, plant-infecting plasmodiophorids using in vitro dual culture. Protist , 162, 449–461. https://doi.org/10.1016/j.protis.2010.09.004
- Cao, T., Tewari, J., & Strelkov, S. E. (2006). Molecular detection of Plasmodiophora brassicae , causal agent of clubroot of crucifers, in plant and soil. Plant Disease , 91(1), 80–87. https://doi.org/10.1094/PD-91-0080
- Castlebury, L. A., Maddox, J. V., & Glawe, D. A. (1994). A technique for the extraction and purification of viable Plasmodiophora brassicae resting spores from host root tissue. Mycology , 86(3), 458–460. https://doi.org/10.1080/00275514.1994.12026435
- Cook, W. R. I., & Swartz, E. J. (1930). The life history, cytology and method of infection of Plasmodiophora brassicae Woron., the cause of finger and toe disease of cabbage and other crucifers. Philosophical Transactions of the Royal Society B , 218, 283–314.
- Daval, S., Gazengel, K., Belcour, A., Linglin, J., Guillerm-Erckelboudt, A. Y., Sarniguet, A., Manzanares-Dauleux, M. J., Lebreton, L., & Mougel, C. (2020). Soil microbiota influences clubroot disease by modulating Plasmodiophora brassicae and Brassica napus transcriptomes. Microbial Biotechnology , 13(5), 1648–1672. https://doi.org/10.1111/1751-7915.13634
- Doyle, J. J., & Doyle, J. L. (1990). Isolation of plant DNA from fresh tissue. Focus , 12, 13–5.
- Faggian, R., Bulman, S. R., Lawrie, A. C., & Porter, I. J. (1999). Specific polymerase chain reaction primers for the detection of Plasmodiophora brassicae in soil and water. Phytopathology , 89(5), 392–397. https://doi.org/10.1094/PHYTO.1999.89.5.392
- Fennell, D. (1960). Conservation of fungus cultures. The Botanical Review , 26, 80–141. https://doi.org/10.1007/BF02860481
- Gaff, D. F., & Okong'o-Ogola, O. (1971). The use of non-permeating pigments for testing the survival of cells. Journal of Experimental Botany , 22, 756–758. https://doi.org/10.1093/jxb/22.3.756
- Gazengel, K., Aigu, Y., Lariagon, C., Humeau, M., Gravot, A., Manzanares-Dauleux, M. J., & Daval, S. (2021). Nitrogen supply and host-plant genotype modulate the transcriptomic profile of Plasmodiophora brassicae. Frontiers in Microbiology , 12, 701067. https://doi.org/10.3389/fmicb.2021.701067
- Harding, M. W., Hill, T. B., Yang, Y., Daniels, G. C., Hwang, S. F., Strelkov, S. E., Howard, R. J., & Feng, J. (2019). An improved Evans blue staining method for consistent, accurate assessment of Plasmodiophora brassicae resting spore viability. Plant Disease , 103(9), 2330–2336. https://doi.org/10.1094/PDIS-05-18-0855-RE
- Hossain, M. M., Pérez-López, E., Todd, C. D., Wei, Y., & Bonham-Smith, P. C. (2021). Endomembrane-targeting Plasmodiophora brassicae effectors modulate PAMP triggered immune responses in plants. Frontiers in Microbiology , 12, 651279. https://doi.org/10.3389/fmicb.2021.651279
- Hwang, S. F., Strelkov, S. E., Feng, J. I. E., Gossen, B. D., & Howard, R. J. (2012). Plasmodiophora brassicae : A review of an emerging pathogen of the Canadian canola (Brassica napus) crop. Molecular Plant Pathology , 13(2), 105–113. https://doi.org/10.1111/j.1364-3703.2011.00729.x
- Hwang, S. F., Ahmed, H. U., Zhou, Q., Fu, H., Turnbull, G. D., Fredua-Agyeman, R., Strelkov, S., Gossen, B., & Peng, G. (2019). Influence of resistant cultivars and crop intervals on clubroot of canola. Canadian Journal of Plant Science , 99, 862–872. https://doi.org/10.1139/cjps-2019-0018
- Javed, M. A., Schwelm, A., Zamani-Noor, N., Salih, R., Silvestre-Vano, M., Wu, J., Gonzalez Garcia, M., Heick, T. M., Luo, C., Prakash, P., & Pérez-López, E. (2022). The clubroot pathogen Plasmodiophora brassicae : A profile update. Molecular Plant Pathology , 24, 89–106. https://doi.org/10.1111/mpp.13283
- Jones, D. R., Ingram, D. S., & Dixon, G. R. (1982). Characterization of isolates derived from single resting spores of Plasmodiophora brassicae and studies of their interaction. Plant Pathology , 31, 239–246. https://doi.org/10.1111/j.1365-3059.1982.tb01274.x
- Kageyama, K., & Asano, T. (2009). Life cycle of Plasmodiophora brassicae. Journal of Plant Growth Regulation , 28, 203–211. https://doi.org/10.1007/s00344-009-9101-z
- Karling, J. S. (1968). The Plasmodiophorales. Hafner Publishing Company.
- Lemarié, S., Robert-Seilaniantz, A., Lariagon, C., Lemoine, J., Marnet, N., Levrel, A., Jubault, M., Manzanares-Dauleux, M. J., & Gravot, A. (2015). Camalexin contributes to the partial resistance of Arabidopsis thaliana to the biotrophic soilborne protist Plasmodiophora brassicae. Frontiers in Plant Sciences , 6, 539.
- Lv, M., Liu, Y., Wu, Y., Zhang, J., Liu, X., Ji, R., & Feng, H. (2021). An improved technique for isolation and characterization of single-spore isolates of Plasmodiophora brassicae. Plant Disease , 105(12), 3932–3938. https://doi.org/10.1094/PDIS-03-21-0480-RE
- Macfarlane, I. (1970). Germination of resting spores of Plasmodiophora brassicae. Transactions of the British Mycological Society , 55(1), 97–112. https://doi.org/10.1016/S0007-1536(70)80100-0
- Muirhead, K., & Pérez-López, E. (2022). Plasmodiophora brassicae CBM18 proteins bind chitin and suppress chitin-triggered immunity. PhytoFrontiers , 2, 21–29. https://doi.org/10.1094/PHYTOFR-04-21-0032-R
- Ochoa, J. C., Mukhopadhyay, S., Bieluszewski, T., Jędryczka, M., Malinowski, R., & Truman, W. (2023). Natural variation in Arabidopsis responses to Plasmodiophora brassicae reveals an essential role for Resistance to Plasmodiophora brasssicae 1 (RPB1). The Plant Journal , 116(5), 1421–1440. https://doi.org/10.1111/tpj.16438
- Padrón-Rodríguez, L., Cabrera, C. R. C., Coello, N. G. S., Luna-Rodríguez, M., & Pérez-López, E. (2022). Plasmodiophora brassicae in Mexico: From anecdote to fact. Plant Disease , 106, 1832–1836. https://doi.org/10.1094/PDIS-11-21-2607-RE
- Pang, W., Liang, Y., Zhan, Z., Li, X., & Piao, Z. (2020). Development of a Sinitic clubroot differential set for the pathotype classification of Plasmodiophora brassicae. Frontiers in Plant Sciences , 11, 568771. https://doi.org/10.3389/fpls.2020.568771
- Pérez-López, E., Hossain, M. M., Tu, J., Waldner, M., Todd, C. D., Kusalik, A. J., Wei, Y., & Bonham-Smith, P. C. (2020). Transcriptome analysis identifies Plasmodiophora brassicae secondary infection effector candidates. Journal of Eukaryotic Microbiology , 67, 337–351. https://doi.org/10.1111/jeu.12784
- Salih, R., Brochu, A.-S., Labbe, C., Strelkov, S. E., Franke, C., Bélanger, R. R., & Pérez-López, E. (2023). A hydroponic-based bioassay to facilitate Plasmodiophora brassicae phenotyping. Plant Disease , 108(1), 131–138. https://doi.org/10.1094/PDIS-05-23-0959-RE
- Schwelm, A., Fogelqvist, J., Knaust, A., Julke, S., Lilja, T., Bonilla-Rosso, G., Karlsson, M., Shevchenko, A., Dhandapani, V., Choi, S. R., Kim, H. G., Park, J. Y., Lim, Y. P., Ludwig-Muller, J., & Dixelius, C. (2015). The Plasmodiophora brassicae genome reveals insights in its life cycle and ancestry of chitin synthases. Scientific Reports , 5(1), 11153. https://doi.org/10.1038/srep11153
- Schwelm, A., & Neuhauser, S. (2017) Letter to the editor: “Detection of ribosomal DNA sequence polymorphisms in the protist Plasmodiophora brassicae for the identification of geographical isolates”. International Journal of Molecular Sciences , 18, 1454. https://doi.org/10.3390/ijms18071454
- Sedaghatkish, A., Gossen, B. D., Yu, F., Torkamaneh, D., & McDonald, M. R. (2019). Whole-genome DNA similarity and population structure of Plasmodiophora brassicae strains from Canada. BMC Genomics , 20(1), 1–14. https://doi.org/10.1186/s12864-019-6118-y
- Somé, A., Manzanares, M. J., Laurens, F., Baron, F., Thomas, G., & Rouxel, F. (1996). Variation for virulence on Brassica napus L. amongst Plasmodiophora brassicae collections from France and derived single-spore isolates. Plant Pathology , 45, 432–439. https://doi.org/10.1046/j.1365-3059.1996.d01-155.x
- Strelkov, S. E., Hwang, S. F., Manolii, V. P., Cao, T., Fredua-Agyeman, R., Harding, M. W., Peng, G., Gossen, B. D., Mcdonald, M. R., & Feindel, D. (2018). Virulence and pathotype classification of Plasmodiophora brassicae populations collected from clubroot resistant canola (Brassica napus) in Canada. Canadian Journal of Plant Pathology , 40, 284–298. https://doi.org/10.1080/07060661.2018.1459851
- Tinggal, S. H., & Webster, J. (1981). Technique for single spore infection by Plasmodiophora brassicae. Transactions of the British Mycological Society , 87, 179–190.
- Voorrips, R. E. (1996). Plasmodiophora brassicae : Aspects of pathogenesis and resistance in Brassica oleracea. Euphytica , 83(2), 139–146. https://doi.org/10.1007/BF01678041
- Voorrips, R. E., & Visser, D. L. (1993). Examination of resistance to clubroot in accessions of Brassica oleracea using a glasshouse seedling test. Netherlands Journal of Plant Pathology , 99, 269–276. https://doi.org/10.1007/BF01974308
- Wang, Y., Koopmann, B., & von Tiedemann, A. (2022). Methods for Assessment of viability and germination of Plasmodiophora brassicae resting spores. Frontiers in Microbiology , 12, 4212.
- Williams, P. H. (1966). A system for the determination of races of Plasmodiophora brassicae that infect cabbage and rutabaga. Phytopathology , 56, 624–626.
- Woronin, M. S. (1877). Plasmodiophora brassicae , der Organismus, der die unter dem Namen Hernie bekannte Krankheit der Kohlpflanzen verursacht. Arbeiten der Sankt Petersburger Naturforschender Gesellschaft , 8, 169–201.
- Wu, J., & Pérez-López, E. (2023). A multilayer strategy is needed to uncover the clubroot pathogen mysteries. Physiological and Molecular Plant Pathology , 124, 101971. https://doi.org/10.1016/j.pmpp.2023.101971
- Xue, S., Cao, T., Howard, R. J., Hwang, S. F., & Strelkov, S. E. (2008). Isolation and variation in virulence of single-spore isolates of Plasmodiophora brassicae from Canada. Plant Disease , 92, 456–462. https://doi.org/10.1094/PDIS-92-3-0456
- Zahr, K., Sarkes, A., Yang, Y., Ahmed, H., Zhou, Q., Feindel, D., Harding, M. W., & Feng, J. (2021). Plasmodiophora brassicae in its environment: Effects of temperature and light on resting spore survival in soil. Phytopathology , 111(10), 1743–1750. https://doi.org/10.1094/PHYTO-09-20-0415-R
- Zamani-Noor, N. (2016). Variation in pathotypes and virulence of Plasmodiophora brassicae populations in Germany. Plant Pathology , 66(2), 316–324. https://doi.org/10.1111/ppa.12573
Key References
- Javed, M. A., Schwelm, A., Zamani-Noor, N., Salih, R., Silvestre-Vano, M., Wu, J., Gonzalez Garcia, M., Heick, T. M., Luo, C., Prakash, P., & Pérez-López, E. (2022). The clubroot pathogen Plasmodiophora brassicae : A profile update. Molecular Plant Pathology , 24, 89–106. https://doi.org/10.1111/mpp.13283
- Updated P. brassicae profile with all the relevant research generated in the last decade, aiming for a better understanding of the clubroot pathogen, its biology, management, and detection.
- Lv, M., Liu, Y., Wu, Y., Zhang, J., Liu, X., Ji, R., & Feng, H. (2021). An improved technique for isolation and characterization of single-spore isolates of Plasmodiophora brassicae. Plant Disease , 105(12), 3932–3938. https://doi.org/10.1094/PDIS-03-21-0480-RE
- Improved methodology for generating P. brassicae SSIs. This method increased the success rate of the process and was implemented in our lab as a high-throughput protocol generating hundreds of SSIs.