Towards the Development, Maintenance and Standardized Phenotypic Characterization of Single-Seed-Descent Genetic Resources for Chickpea
Saleh Alseekh, Saleh Alseekh, Alisdair R. Fernie, Alisdair R. Fernie, Elena Bitocchi, Roberto Papa, Karolina Susek, Tania Gioia, Tania Gioia, Giuseppina Logozzo, Giuseppina Logozzo, Lorenzo Rocchetti, Lorenzo Rocchetti, Creola Brezeanu, Creola Brezeanu, Luis Guasch Pereira, Luis Guasch Pereira, Lucía De la Rosa, Lucía De la Rosa, Stefania Marzario, Stefania Marzario, Alice Pieri, Alice Pieri, Douglas R. Cook, Rajeev K. Varshney, Shiv Kumar Agrawal, Aladdin Hamwieh
Abstract
Here we present the approach used to develop the INCREASE “Intelligent Chickpea” Collections, from analysis of the information on the life history and population structure of chickpea germplasm, the availability of genomic and genetic resources, the identification of key phenotypic traits and methodologies to characterize chickpea. We present two phenotypic protocols within H2O20 Project INCREASE to characterize, develop, and maintain chickpea single-seed-descent (SSD) line collections. Such protocols and related genetic resource data from the project will be available for the legume community to apply the standardized approaches to develop Chickpea Intelligent Collections further or for multiplication/seed-increase purposes. © 2022 The Authors. Current Protocols published by Wiley Periodicals LLC.
[Correction added on May 16, 2022, after first online publication: CRUI-CARE funding statement has been added.]
Basic Protocol 1 : Characterization of chickpea seeds for seed-trait descriptors
Basic Protocol 2 : Characterization of chickpea lines for plant-trait descriptors specific for primary seed increase
INTRODUCTION
In recent years, there has been clear recognition of the role of food legumes in the transition to a plant-based diet, along with the importance of their wider use in the fight against climate change and to promote food security and human health (Bellucci et al., 2021). A crucial step to increase the cultivation and consumption of food legumes is the analysis of their genetic resources. Indeed, characterization, maintenance, and use of food legume genetic resources are key steps in breeding new varieties with greater adaptation and improved quality traits and nutritional value. Among food legumes, chickpea (Cicer arietinum) is the second most important food legume (after the common bean) for direct human consumption worldwide (FAOStat 2019, see Internet Resources). Advances in genomics and metabolomics offer new opportunities to characterize the heritable diversity of genetic resources and identify the genetic basis of phenotypic traits for the development of genomic prediction tools and a deeper understanding of Genotype × Environment interactions. Under this scenario, the development of well-described collections based on pure single-seed-descent (SSD) lines where the phenotypic characteristics are linked to a specific genotype offers the potential to manage the genetic resources efficiently and create unique information for access by users. Here, we first outline the main aspects of our knowledge of chickpea's evolutionary history and population structure, along with a description of the germplasm collections conserved worldwide and the available genomic resources. In the second part, we present the concept and protocols for developing “Intelligent Collections” (ICs) for chickpea and their phenotypic characterization during the multiplication/seed-increase steps, as has also been proposed for common bean (Cortinovis et al., 2021), lentil (Guerra-García et al., 2021), and lupin (Kroc et al., 2021).
ORIGIN, DOMESTICATION, DIFFUSION, AND EVOLUTION OUT OF THE CENTERS OF ORIGIN
The genus Cicer comprises 46 species with 10 annuals and 36 perennials, which include a new Cicer species that was recently discovered, and is named Cicer turcicum Toker, Berger & Gokturk (Ladizinsky & Adler, 1976; Toker et al., 2021; van der Maesen et al., 2007).
Following the gene-pool concept introduced by Harlan and De Wet (1971), the different Cicer species can be grouped into (i) the primary gene pool that includes the domesticated C. arietinum and its wild progenitor C. reticulatum ; (ii) the secondary gene pool that is represented by C. echinospermum P.H. Davis; and (iii) the tertiary gene pool that comprises wild Cicer species, as six annuals (C. pinnatifidum Jaub. & Spach.; C. bijugum Rech. f.; C. judaicum Boiss; C. yamashitae Kitam.; C. chorassanicum Popow; C. cuneatum Hochst. ex A. Rich.) and 36 perennials (Ahmad, Slinkard, & Scoles, 1988; Smýkal et al., 2015). Based on similarities of internal transcribed spacers (ITS), Toker et al. (2021) reported that the recently proposed C. turcicum species is closely related to C. echinospermum and suggested that it is likely to be a member of the secondary gene pool; however, crossing experiments need to be carried out to test hybridization with other chickpea species (Toker et al., 2021).
The domesticated species C. arietinum is diploid (2n = 2× = 16) and predominantly selfing, with an estimated genome size of 740 Mpb (Varshney et al., 2013). The wild progenitor C. reticulatum (Ladizinky & Adler 1976) is currently found in a few provinces in southeastern Turkey (von Wettberg et al., 2018). A strong domestication-related genetic bottleneck was observed (von Wettberg et al., 2018).
The Fertile Crescent is considered the center of chickpea domestication (Harlan, 1992). Cultivated chickpea spread further into the Levant and ultimately throughout the Mediterranean basin (southern Europe, northern Africa). Cultivation of chickpea began within the past century in North America and Australia (Berger & Turner 2007). Figure 1 shows the distribution by the origin of the chickpea genetic resources conserved in gene banks (Genesys, see Internet Resources).
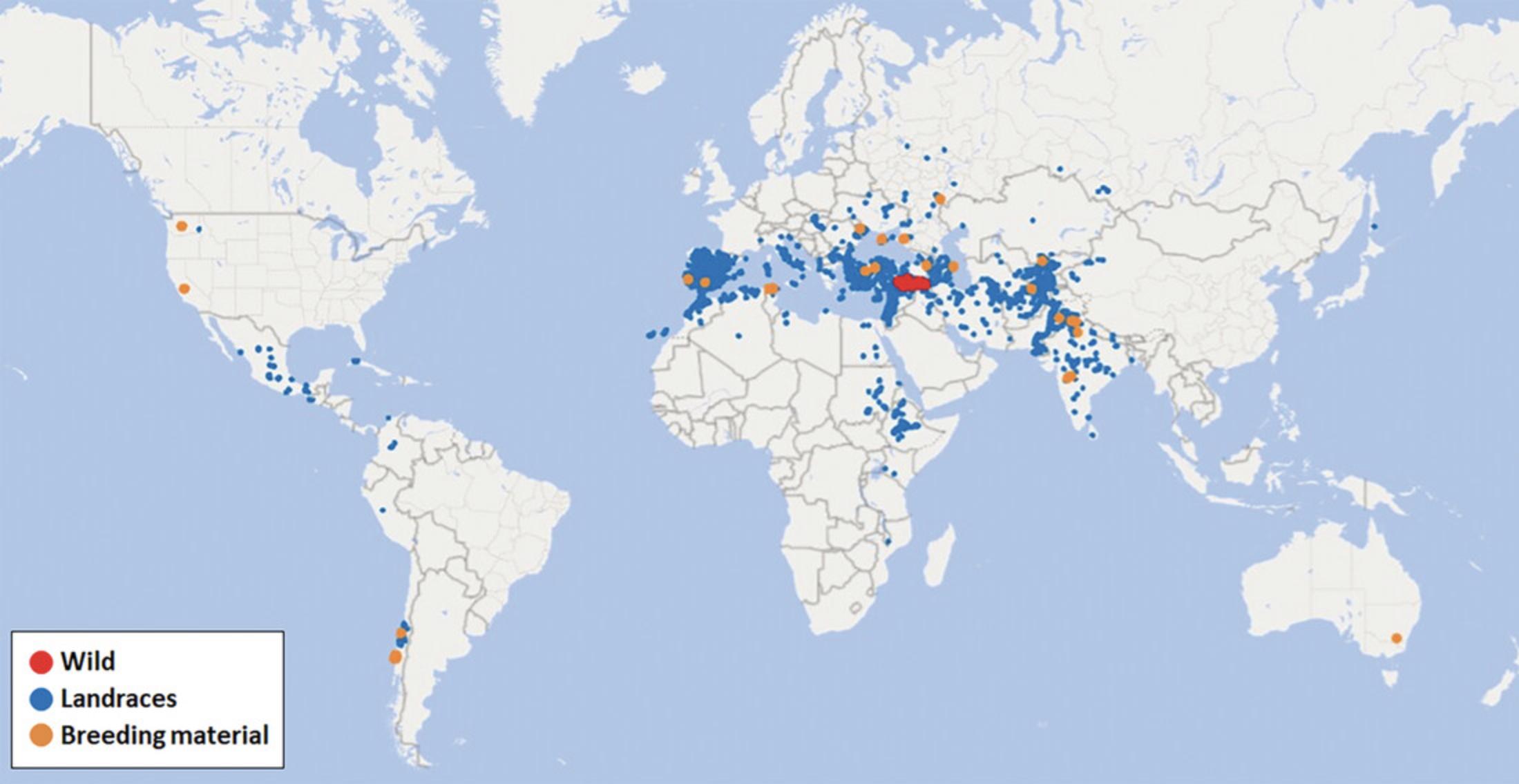
Many domesticated species from the Fertile Crescent are cultivated after autumn germination, which is also the pattern observed among their wild progenitors. The situation with chickpeas is less clear. Breeding has adapted the modern crop to diverse agroclimatic zones, with chickpea grown as a spring and summer crop in Turkey, North Africa, and North America, winter in Australia and India, and post-monsoon fall in Ethiopia. Abbo, Berger, and Turner (2003) suggested that wild Cicer has a fall germination pattern and that the practice of spring cropping was adopted by farmers attempting to avoid the devastating effects of Ascochyta blight. However, von Wettberg et al. (2018) documents examples where wild populations exhibit a pattern of springtime (April-May) vegetative growth, with maturation occurring in early summer (June) as temperatures rise and humidity declines. Berger, Buck, Henzell, and Turner (2005) compared wild and domesticated chickpea genotypes and detected a loss of vernalization responses and other chilling tolerant traits (i.e., pod setting delay with temperatures <16°C) for the cultivated germplasm, showing that selection for different phenological mechanisms across the cultivation range has important adaptive implications (Berger & Turner 2007; Berger et al., 2011).
Two market classes of chickpea are recognized: desi and kabuli. Desi chickpea is characterized by purple flowers and small dark seeds, predominantly grown in the Indian sub-continent and Ethiopia. Kabuli chickpea is characterized by white flowers and larger, light-colored seeds with a thin seed coat produced and consumed in the Mediterranean Basin, the Middle East, and North America. As with other legumes, anthocyanins and pro-anthocyanidins appear to underlie differences in flower and seed color, as well as seed coat thickness (Penmetsa et al., 2016). Genetic analyses demonstrate that the desi-vs-kabuli dichotomy arose multiple times independently, correlated with loss of function in a key transcriptional regulator (Penmetsa et al., 2016). Population structure analysis of diverse chickpea collections has shown that the identification of these groups is largely correlated with geographic distribution rather than desi and kabuli differentiation (De Giovanni et al., 2017; Penmetsa et al., 2016; Sokolkova et al., 2020; Varshney et al., 2021).
WORLDWIDE GERMPLASM COLLECTIONS
The genetic diversity of chickpea germplasm maintained in gene banks is vital for discovering useful genes for chickpea breeding programs. At the worldwide level, there are about 59 gene banks and more than 65,000 conserved Cicer accessions (Genesys, 2021, accessed June 10, 2021; see Internet Resources). For all germplasm collections, overrepresentations of specific collection sites and duplication of accessions due to exchange between gene banks is common (see the example from Berger, Abbo, & Turner, 2003 for wild Cicer) and suggest much care when developing sampling schemes. The International Crop Research Institute for the Semi-Arid Tropics (ICRISAT) in India holds the largest Cicer collection, with 20,764 accessions, followed by the International Centre for Agricultural Research in Dry Areas (ICARDA) in Lebanon, with 15,368 accessions; the Australian Grains Genebank, Department of Economic Development Jobs Transport and Resources, with 9771 accessions; and the Western Regional Plant Introduction Station (USDA-ARS), with 6732 accessions. Cicer accessions are also maintained by the N.I. Vavilov Institute of Plant Genetic Resources (VIR), one of the oldest gene banks in the world, with 2767 chickpea accessions. The VIR chickpea collection is unique in its preponderance of historic landraces, sampled prior to the advent of intensive modern breeding. Thus, the VIR collection is likely to capture genetic adaptations and functional diversity that reflect historic local and regional practices (Plekhanova et al., 2017), providing opportunities to study post-domestication and diversification processes prior to the Green Revolution (Sokolkova et al., 2020).
The following Cicer species are conserved: 61,770 C. arietinum , 424 C. reticulatum , 244 C. judaicum , 138 C. echinospermum , 171 C. pinnatifidum , 167 C. bijugum , 74 C. microphyllum , and 29 C. cuneatum. For 114 accessions, only the genus (Cicer sp.) was determined (Genesys, 2021, accessed June 10, 2021; see Internet Resources). According to the available biological status data, most of the accessions are classified as landraces (31,149 accessions), followed by breeding/research materials (8347 accessions), and wild (1121 accessions) and advanced/improved (1125 accessions) cultivars. The top six countries of origin of the materials are India (13,101 accessions), Iran (9610 accessions), Syria (6268 accessions), Turkey (5707 accessions), Afghanistan (2259 accessions), and Pakistan (1791 accessions).
GENOMICS OF THE GENETIC RESOURCES
The development of high-throughput sequencing technologies has provided new opportunities for genomic characterization of genetic resources and the application of population genetics approaches and genome-wide analysis to identify the genetic control of phenotypic variance for specific traits. In the last decade, such methods have been largely used for chickpea genetic resources, which created a reference set of genomics data useful for the scientific community and plant breeders (Roorkiwal et al., 2020).
Three reference genomes are available for chickpea: one for the kabuli type (CDC Frontier genotype; Varshney et al., 2013), one for the desi type (ICC 4958 genotype; Parween et al., 2015), and one for a wild C. reticulatum accession (PI489777; Gupta et al., 2017). The three genomes, together with de novo whole-genome sequencing data (aligned to CDC Frontier) from 3171 cultivated and 28 C. reticulatum accessions, were used to guide the assembly of the chickpea pan-genome (Varshney et al., 2021). Draft genome sequences of the wild C. echinospermum S2Drd 065 accession and the wild C. reticulatum Besevler 079 accession are also in the NCBI BioProject database (accession numbers, PRJNA418060, PRJNA418059, respectively), as developed by the D. Cook laboratory (University of California, Davis).
Whole-genome sequencing data of different and wide diversity panels are already available. The work of Thudi et al. (2016) shows the whole-genome sequencing analysis of a set of 129 commercial chickpea varieties that were released between 1948 and 2012 in 14 countries. Resequencing data are also available for a panel of a diverse set of 429 lines that were collected in 45 countries (Varshney et al., 2019). Other whole-genome sequencing data for chickpea genetic resources are in the NCBI BioProject database, including 229 wild Cicer accessions (accession number, PRJNA416007).
From the Australian chickpea breeding program, a small set of 69 chickpea lines that differed in their resistance to Ascochyta blight (Li et al., 2017) and a set of 132 commercial varieties and advanced breeding lines were resequenced (Li et al., 2018).
Several studies that have used chickpea core collections are also available, based on genotyping by sequencing (GBS) data (Pavan et al., 2017; Roorkiwal et al., 2018). Sokolkova et al. (2020) used GBS to genotype a set of 407 landraces sampled from the chickpea VIR collections, which included the domestication and secondary diversity centers. Von Wettberg et al. (2018) collected a wide set of wild chickpea materials (about 1,000 accessions) and characterized these using GBS. Ecological principles were used to guide the collection of these materials across the full range of habitats in which wild chickpea occurs. This research work also increased the supply of wild Cicer accessions compared to the material that had been available over the previous decade (Berger et al., 2003). It provided related GBS data and deep characterization of the accessions (e.g., evolutionary history, ancestral adaptations, the impact of environment on genetic structure, trait values), which has proven useful to improve cultivated chickpea germplasm.
Segregant populations also represent important plant genetic resources, such as bi-parental populations like recombinant inbred lines (RILs) and multiparent populations like nested association mapping (NAM) and multi-parent advanced generation inter-cross (MAGIC). In chickpea, a NAM population of 284 lineages was developed from the crosses of a single early flowering cultivated lineage (ICCV 96029) and 20 wild accessions (Shin et al., 2019). The 284 lineages were composed of 255 F3 families derived from C. arietinum (ICCV 96029), 17 C. reticulatum parents, and 29 F3 families derived from C. arietinum (ICCV 96029) × 3 C. echinospermum wild parents. The combined 284 lineages were genotyped using GBS and phenotyped for agronomic traits (Shin et al., 2019).
Two MAGIC populations are available for chickpea: one that was developed at ICRISAT that includes 1136 F8 RILs derived from eight well-adapted and drought-tolerant desi chickpea cultivars (Samineni et al., 2021), and one that was developed at ICARDA that includes 3053 RILs derived from 12 diverse parents (unpublished data).
DEVELOPMENT, MAINTENANCE, AND CHARACTERIZATION OF CHICKPEA “INCREASE INTELLIGENT” COLLECTIONS
The characterization and maintenance of legume genetic resources are crucial for their exploitation in breeding programs to develop novel varieties adapted to different environments and that show interesting agronomic and quality traits. Traditional methods identify plant genetics resources based on passport data and maintain seed collections at the accession level. Generally, each accession is composed of a mixture of individuals (population) with unknown genotypic information. This approach does not allow phenotypic and genotypic information to be linked. Moreover, this type of conservation based on small effective population sizes when seeds are regenerated can be affected by random genetic drift, adding new selection pressures in the gene-bank fields (Mascher et al., 2019).
Recently, the strategy of developing and using SSD purified accessions has been applied in several projects (e.g., AGILE, BEAN_ADAPT, BRESOV, BRIDGE); indeed, SSD lines offer the possibility to associate phenotypes to a unique genotype, thus promoting genetic resources conservation and their use in pre-breeding programs. With the purpose of conservation, management, and making the best use of food-legume genetic resources, the European Union Horizon 2020 has funded the Project INCREASE—Intelligent Collections of Food Legumes Genetic Resources for European Agrofood Systems (Bellucci et al., 2021) (https://www.pulsesincrease.eu/).
The main aim of INCREASE is to enhance the genotypic and phenotypic characterization of genetic resources of four important food legumes linked to the European food tradition: chickpea, common bean, lentil, and lupin. The INCREASE approach involves the development of nested-core collections of different sizes based on genetically purified accessions (Bellucci et al., 2021). The collections are designed to represent the worldwide diversity of each legume crop. Their genetic and phenotypic characterization at different levels is also planned based on the collection size. Linking genotypic and phenotypic data will allow identification of the genes and/or genomic regions responsible for important adaptive and agronomic traits and implement genomic selection models to predict phenotypes based on genetic data (Bellucci et al., 2021).
Currently, for chickpea, two INCREASE collections have been established: the larger Reference core (R-CORE) collection that comprises a total of 3276 accessions (mainly C. arietinum , but also including wild Cicer species of its gene pools); and the Training CORE (T-CORE) collection, defined by maximizing the geographic distribution and the phenotypic diversity based on characterizations already available. The T-CORE, which represents a subset of the R-CORE, comprises 450 C. arietinum genotypes that are mostly (392 lines) derived from the European and Mediterranean Association Panel (EMCAP) collection (480 lines) developed at the Polytechnic University of Marche (Ancona, Italy) (Rocchetti et al., 2020).
The procedures applied by the INCREASE project to develop, maintain, and characterize SSD lines of each legume species included in INCREASE are summarized in Figure 2. Thus, the development and characterization of SSD lines of chickpea Intelligent Collections will follow the same workflow (i.e., selfing cycles under controlled conditions, assignment of Digital Object Identifiers, DNA extraction, phenotypic characterization, transfer of information to the INCREASE database).
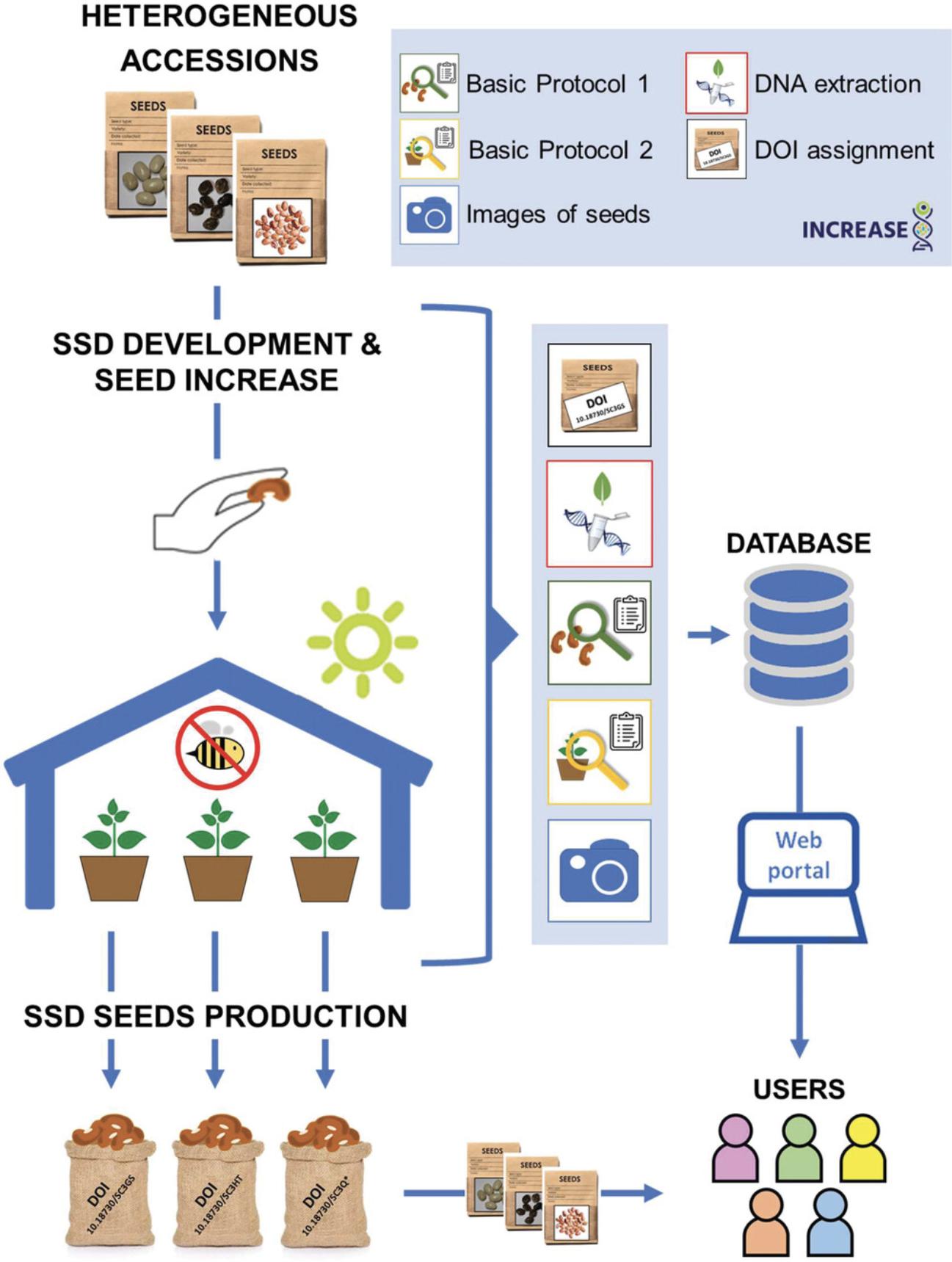
Specific protocols to characterize the seeds before and after the selfing cycles (including images of the seeds) and the plants during cultivation under controlled conditions have been established and described for common bean (Cortinovis et al., 2021), lentil (Guerra-García et al., 2021), and lupin (Kroc et al., 2021); here, we describe the specific protocols to be applied for characterization of chickpea SSD lines during the process of development and maintenance of seeds (i.e., the so-called Primary Seed Increase within INCREASE; see Cortinovis et al., 2021). These protocols for chickpea were designed based on highly hereditable seed and plant traits that can be used for phenotypic characterization and to monitor the SSD seed-multiplication process (i.e., check for potential human errors during the different seed-increase cycles). The protocols are based on IBPGR/ICRISAT/ICARDA 1993 Descriptors for chickpea (C. arietinum L.), Chickpea Crop Ontology, https://www.cropontology.org/, and other specific traits newly defined in INCREASE. The source of each descriptor is indicated in brackets after each one.
Basic Protocol 1: CHARACTERIZATION OF CHICKPEA SEEDS FOR SEED-TRAIT DESCRIPTORS
This protocol was developed to characterize seeds before the start of any selfing multiplication cycles carried out under controlled conditions. The protocol allows the characterization of the original seed phenotype from heterogeneous accessions and the seeds obtained in subsequent cycles. The data obtained will also be used as a validation tool for the accuracy of the entire process (i.e., identification of potential human/technical errors).
Materials
-
Seeds
-
Ruler
-
Analytical balance
-
Spreadsheet for data collection
1.Seed weight (g) – (Crop Ontology Code ID CO_338:0000026). Count 100 well-developed seeds harvested when plants are completely dried. If this number is not possible, count and weigh a sample of 10 seeds for each line (if more than 10 seeds are available, three samples of 10 randomly selected seeds should be weighed, and the values averaged).
2.Seed shape (Crop Ontology Code ID CO_338:0000019). Take at least five seeds from each accession (heterogeneous materials) or line (previously developed SSD lines), and through visual observation, evaluate and classify the seed shape according to the following categories (Fig. 3):
- 1 = Angular (ram's head)
- 2 = Pea-shaped
- 3 = Irregular (owl's head)
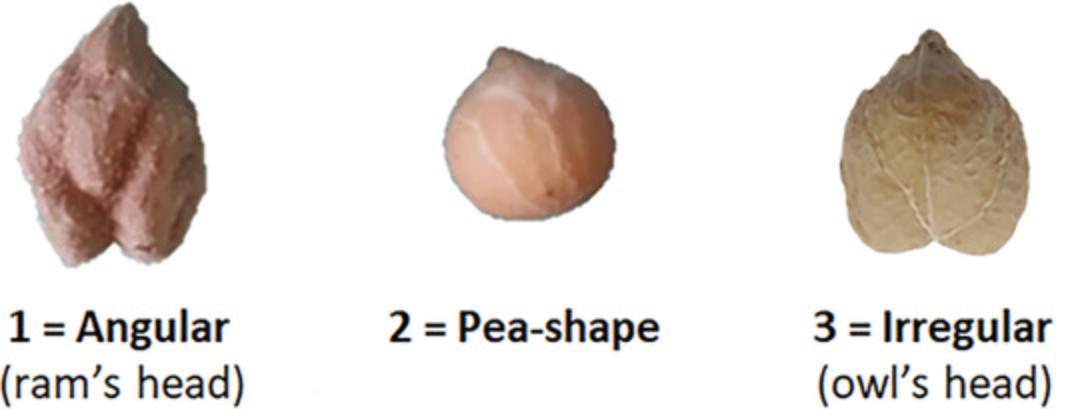
3.Seed surface (Crop Ontology Code ID CO_338:0000021). Take at least five seeds (the same used to evaluate seed shape) from each accession (heterogeneous materials) or line (previously developed SSD lines), and through visual observation, evaluate and classify the seed surface according to the following categories (Fig. 4):
- 1 = Smooth
- 2 = Rough
- 3 = Tuberculate
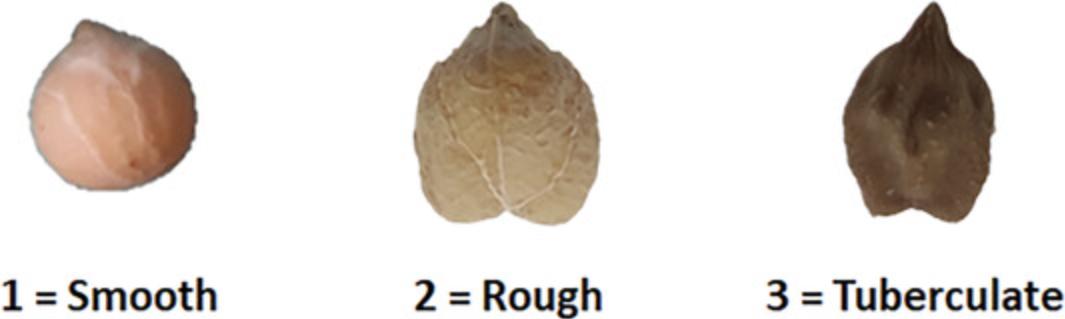
4.Seed color (INCREASE descriptor). Take at least five seeds (the same used to evaluate seed shape and surface) from each accession (heterogeneous materials) or line (previously developed SSD lines), and through visual observation, evaluate and classify the seed color of mature seeds stored not longer than 5 months, according to the following categories (Fig. 5):
- 1 = Black
- 2 = Brown
- 3 = Reddish
- 4 = Grayish
- 5 = Yellow
- 6 = Green
- 7 = Beige or cream
- 8 = White or whitish
- 9 = Other
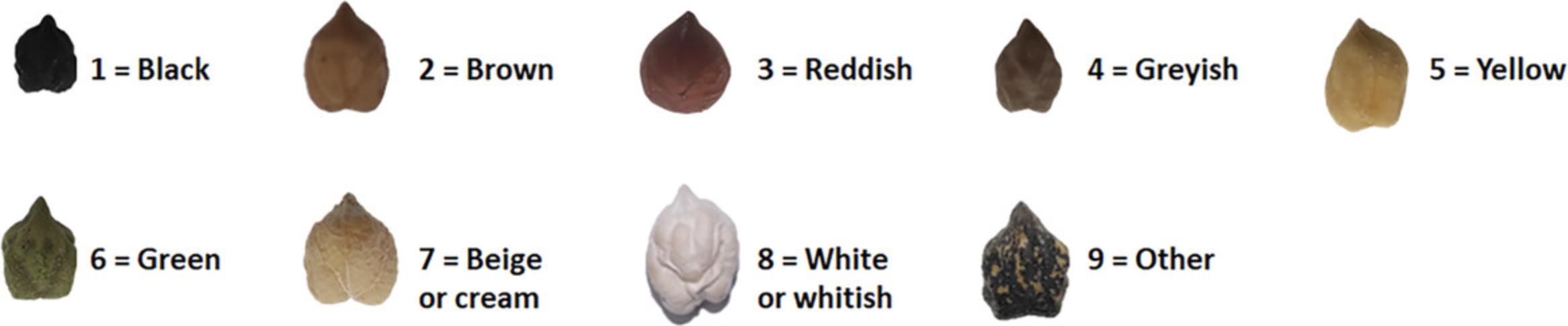
5.Dotted seed coat (Crop Ontology Code ID CO_338:0000022). Visually assess the presence or absence of dots on the seed coat (Fig. 6):
- 1 = Absent
- 2 = Present
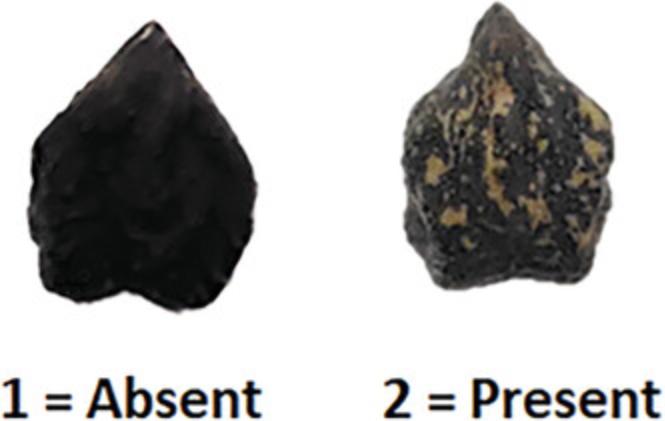
6.Seed imaging. Take images of chickpea seeds before and after each multiplication cycle following the SEED imaging protocol established within the INCREASE project for all four legume species. The protocol is reported in Basic Protocol 2 and Cortinovis et al. (2021). An example of images obtained for chickpea SSD lines is given in Figure 7.
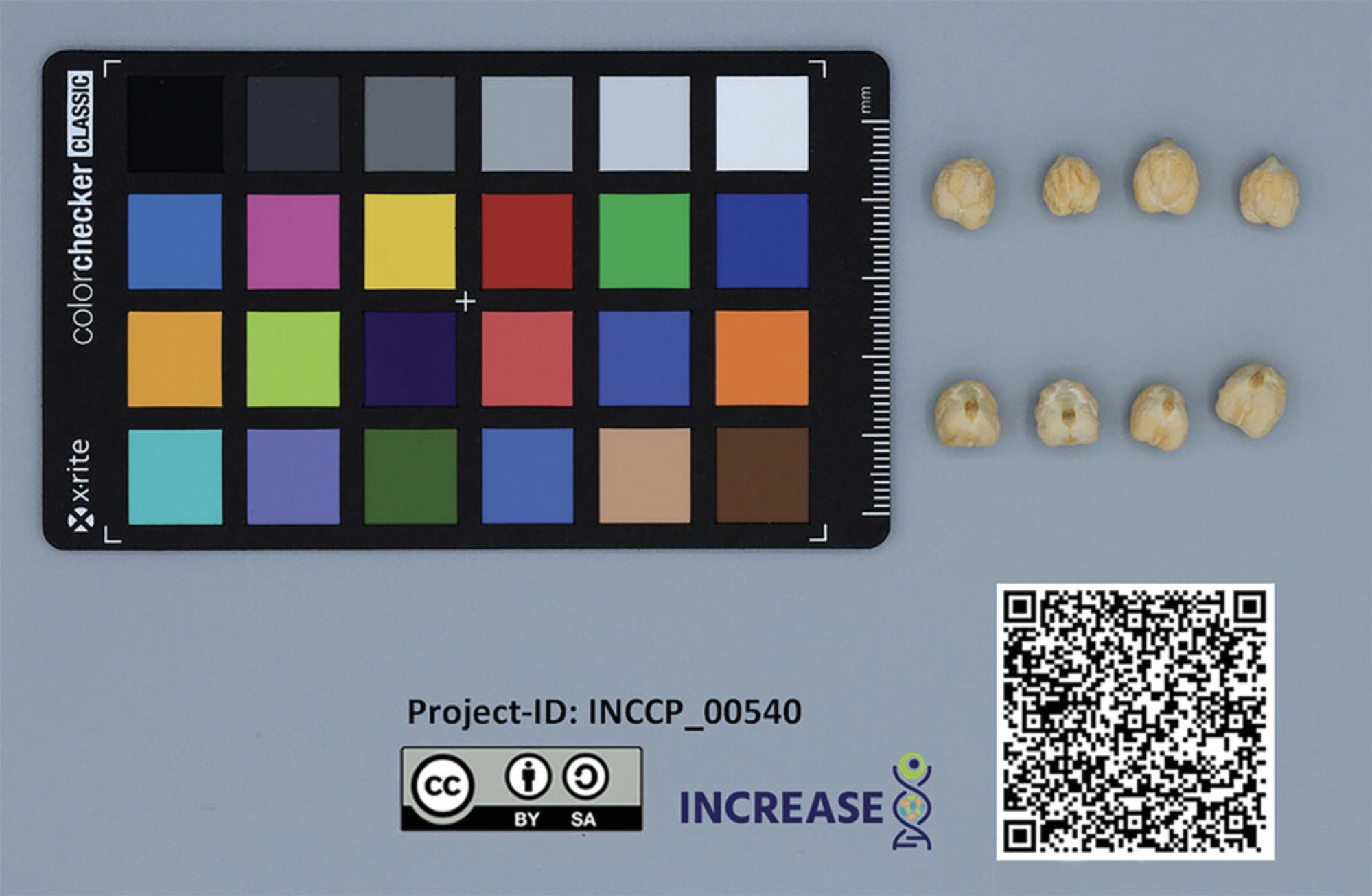
Basic Protocol 2: CHARACTERIZATION OF CHICKPEA LINES FOR PLANT-TRAIT DESCRIPTORS SPECIFIC FOR PRIMARY SEED INCREASE
This protocol was developed to characterize plant and seed traits for each line during the seed multiplication cycle (Primary Seed Increase). Plants must be grown in a greenhouse or growth chamber to ensure insect-free conditions and avoid cross-pollination.
Data are differentiated according to two levels of priority: Priority 1 (Mandatory Traits) and Priority 2 (Non-Mandatory Traits).
Materials
-
Seeds
-
Ruler
-
Analytical balance
-
Spreadsheet for data collection
Priority 1, Mandatory Traits
1.Days to emergence (INCREASE Descriptor). Record the number of days from sowing to the stage when seedlings have emerged.
2.Time to flowering (Crop Ontology Code ID CO_338:0000266). Record the number of days from sowing to the stage when plants have at least one open flower (standard petal is visible).
3.Plant pigmentation (Crop Ontology Code ID CO_338:000015). Observe the anthocyanin coloration at the base of the ramification, and score according to the following three categories (Fig. 8):
- 1 = No anthocyanin
- 2 = Low anthocyanin
- 3 = High anthocyanin
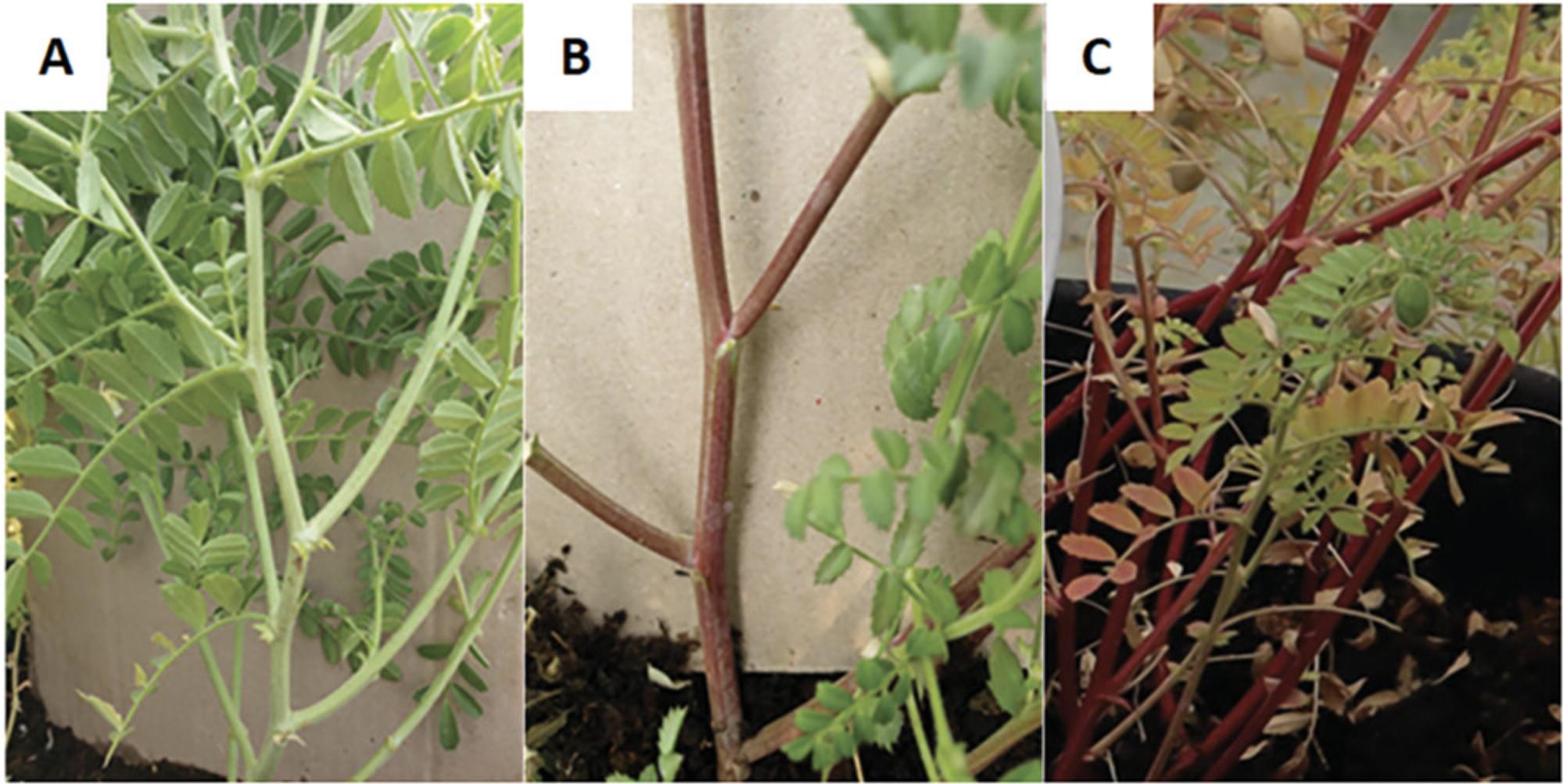
4.Leaf type (Crop Ontology Code ID CO_338:0000066). Visually observe before maturity and score as (Fig. 9):
- 1 = Normal (uni-imparipinnate)
- 2 = Simple (leaf lamina not differentiated into leaflet and rachis)
- 3 = Multipinnate (leaf lamina differentiated more than once)
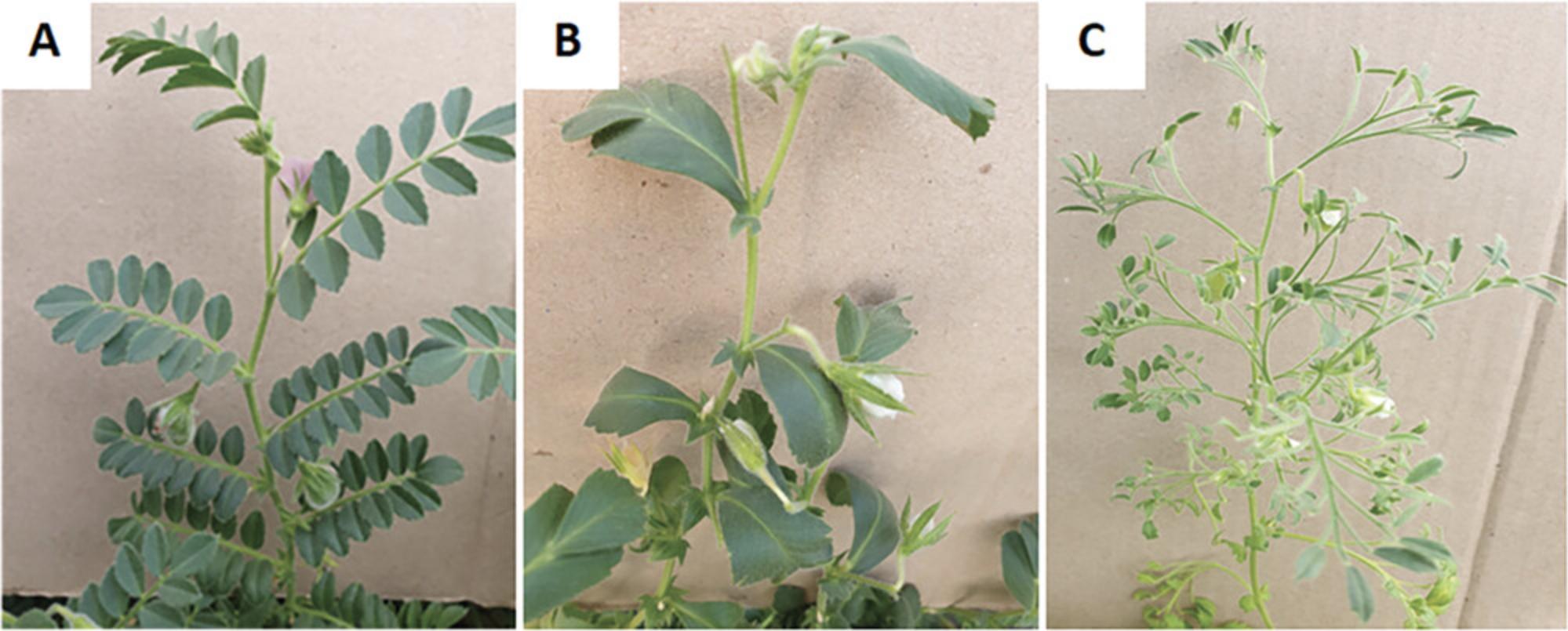
5.Number of leaflets per fully developed leaf (Crop Ontology Code ID CO_338: 0000079). Observe at the filling stage, select three fully developed leaves from the median part of the plant, count the leaflets, and report the average.
6.Flower color: the ground color of the standard petal (Crop Ontology Code ID CO_338:0000017). Visually observe freshly opened flowers. An example is shown in Figure 10.
- 1 = Dark pink
- 2 = Pink
- 3= Dark blue
- 4= Blue
- 5 =White

7.Number of flowers per peduncle (Crop Ontology Code ID CO_338:0000054). Observe the number of flowers of the majority of peduncles:
- 1 = Single pod per peduncle
- 2 = Twin pods (at least 10% of the peduncles bear two pods)
8.Plant growth habit (Crop Ontology Code ID CO_338:0000011). Visually assess at mid-pod filling stage (the use of a protractor is strongly recommended), according to the angle of the primary branches (Fig. 11):
- 1 = Erect (0° to 15° from the vertical)
- 2 = Semi-erect (16° to 25° from the vertical)
- 3 = Semi-spreading (26° to 60° from the vertical)
- 4 = Spreading (61° to 80° from the vertical)
- 5 = Prostrate (branches flat on the ground)
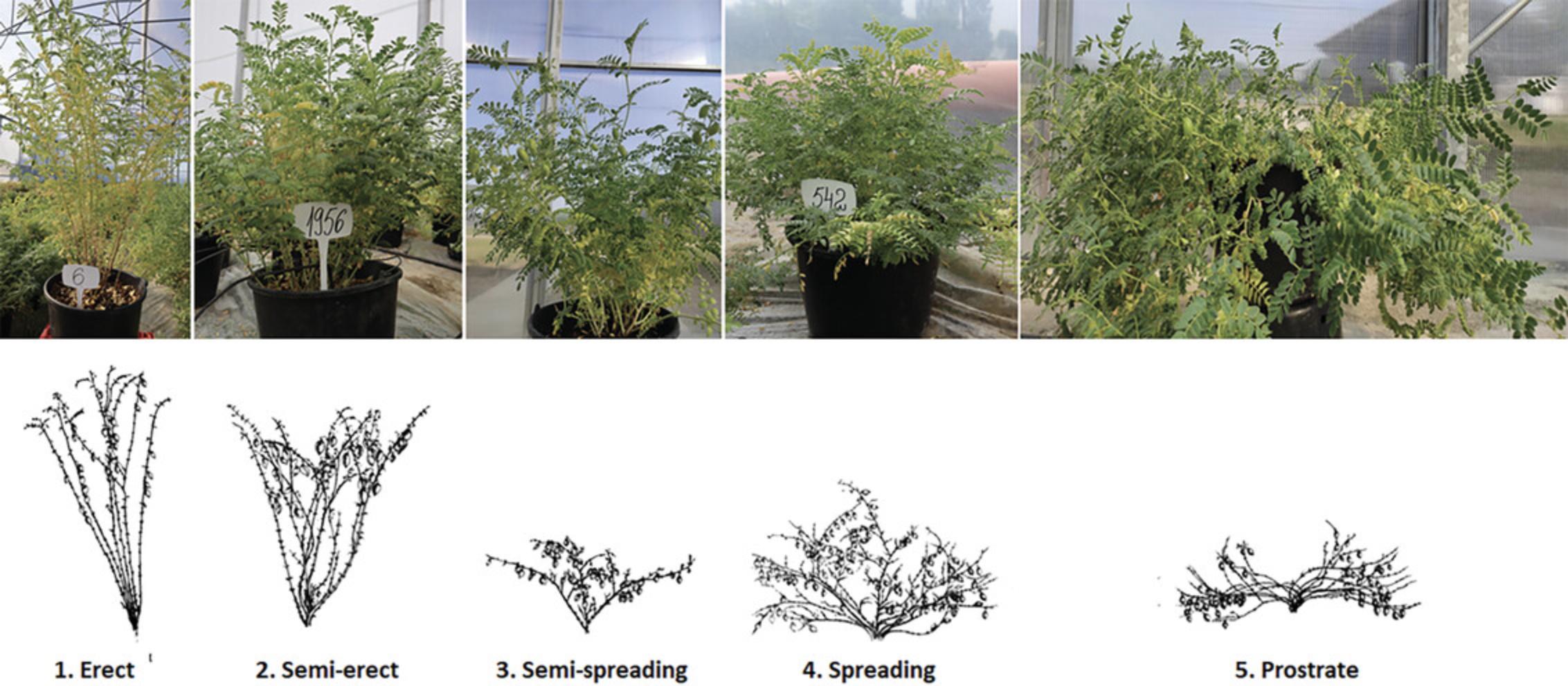
9.Time to maturity (Crop Ontology Code ID CO_338:0000044). Record the number of days from sowing to the stage when 90% of the pods are golden-brown and ready to be harvested.
10.Seeds per pod (IBPGR Descriptor). Record the mean of the number of seeds in 5 randomly selected pods.
11.Seeds per plant (Crop Ontology Code ID CO_338:0000052). Count the total number of seeds harvested from each plant, excluding immature, broken, or infected seeds.
12.Seed mass per plant (Crop Ontology Code ID CO_338:0000025). When the plants are completely dried, harvest the seeds and record the total weight (g) of all of the seeds from each plant.
13.Seed weight (Crop Ontology Code ID CO_338:0000026). Count and weigh 100 well-developed seeds, excluding immature, broken, or infected seeds. If this is not possible, count and weigh a sample of 10 seeds maximum and adjust the proportion to 100 seeds.
14.Biotic stress present (INCREASE descriptor). Record the presence of any biotic stress, and if possible, describe it or make notes.
- 1 = No biotic stress present
- 2 = Biotic stress present
- 3 = Unsure
Priority 2, Non-Mandatory Traits
15.Days until plants have 50% mature pods (INCREASE descriptor). Record the number of days from sowing to the stage when 50% of the plants have mature pods (i.e., the pods are dry and ready to be harvested).
16.Plant pubescence (Crop Ontology Code ID CO_338:0000080). Visually observe the intensity of trichomes on the shoot and score according to the following categories (Fig. 11):
- 1 = Low pubescence
- 2 = Moderate pubescence
- 3 = High pubescence
17.Pod length (Crop Ontology Code ID CO_338:0000076). Measure the pod length at the mid-pod filling stage. Select three pods at random, measure the lengths, and report the average (mm).
18.Pod dehiscence (IBPGR Descriptor). Record a week after maturity, by visual observation of pod dehiscence:
- 1 = No dehiscent
- 2 = <10% dehiscent
- 3 = >10% dehiscent
19.Pods per plant (Crop Ontology Code ID CO_338:0000004). Calculate the average number of pods from 3 to 5 randomly selected plants.
20.Pests and disease susceptibility (INCREASE descriptor): Record the presence of the specific biotic stress when present.
-
Insects:
-
Aphids
-
Leaf miners
-
Weevils
-
Pod borers
-
Storage bruchid beetles
-
b.Fungi:
-
Rust
-
Ascochyta blight
-
Vascular wilt
-
Gray mold
COMMENTARY
Conclusions
Here we have provided the phenotypic protocols to be applied to chickpea seeds and plants during the development and maintenance of SSD lines. The protocols were developed within the H2O20 Project INCREASE, and they are designed to improve the characterization of legume genetic resources and promote their exploitation for breeding. The protocols ensure a valuable phenotypic evaluation of chickpea materials based on highly hereditable seed and plant traits that can also be used as a validation system to monitor the maintenance and seed increase of SSD purified accessions. Such SSD lines are, indeed, crucial to identify marker-trait associations and to predict phenotypes based on genotypic data.
Within INCREASE, the data will be integrated into a centralized data system. Each SSD line will be linked to genotypic and phenotypic data produced during the project and will be freely accessible by users. Also, after the end of INCREASE, these materials can be used by research institutes and gene banks, and they can apply these protocols to increase the information present in the database.
Acknowledgments
This study was conducted as part of the INCREASE project, funded from the European Union Horizon 2020 Research and Innovation program under grant agreement no. 862862.
Open Access Funding provided by Universita Politecnica delle Marche within the CRUI-CARE Agreement.
Author Contributions
Lorenzo Rocchetti : Initial draft, review, and editing. Tania Gioia : Validation, initial draft, review, and editing. Giuseppina Logozzo : Validation, initial draft, review, and editing. Creola Brezeanu : Validation, initial draft, review, and editing. Luis Guash Pereira : Validation, initial draft, review, and editing. Lucía De la Rosa : Validation, initial draft, review, and editing. Stefania Marzario : Validation, initial draft, review, and editing. Alice Pieri : Validation, initial draft, review, and editing. Alisdair R. Fernie : Validation, initial draft, review, and editing. Saleh Alseekh : Validation, initial draft, review, and editing. Karolina Susek : Validation, initial draft, review, and editing. Douglas R. Cook : Validation, initial draft, review, and editing. Rajeev K. Varshney : Validation, initial draft, review, and editing. Shiv Kumar Agrawal : Validation, initial draft, review, and editing. Aladdin Hamwieh : Validation, initial draft, review, and editing. Elena Bitocchi : Validation, initial draft, review, and editing. Roberto Papa : Supervision, initial draft, review, and editing.
Conflict of Interest
The authors declare no conflict of interest.
Open Research
Data Availability Statement
Data sharing is not applicable—no new data generated.
Literature Cited
- Abbo, S., Berger, J., & Turner, N. C. (2003). Evolution of cultivated chickpea: Four bottlenecks limit diversity and constrain adaptation. Functional Plant Biology , 30(10), 1081–1087. doi: 10.1071/FP03084.
- Ahmad, F., Slinkard, A. E., & Scoles, G. J. (1988). Investigations into the barrier (s) to interspecific hybridization between Cicer arietinum L. and eight other annual Cicer species. Plant Breeding , 100(3), 193–198. doi: 10.1111/j.1439-0523.1988.tb00240.x.
- Bellucci, E., Mario Aguilar, O., Alseekh, S., Bett, K., Brezeanu, C., Cook, D., … Papa, R. (2021). The INCREASE project: Intelligent collections of food-legume genetic resources for European agrofood systems. The Plant Journal, Early-View , 108(3), 646–660. doi: 10.1111/tpj.15472.
- Berger, J., Abbo, S., & Turner, N. C. (2003). Ecogeography of annual wild Cicer species: The poor state of the world collection. Crop Science , 43(3), 1076–1090. doi: 10.2135/cropsci2003.1076.
- Berger, J. D., Buck, R., Henzell, J. M., & Turner, N. C. (2005). Evolution in the genus Cicer —vernalisation response and low temperature pod set in chickpea (C. arietinum L.) and its annual wild relatives. Australian Journal of Agricultural Research , 56(11), 1191–1200. doi: 10.1071/AR05089.
- Berger, J. D., & Turner, N. C. (2007). The ecology of chickpea. In S. S. Yadav, R. J. Redden, W. Chen, B. Sharma (Eds.) Chickpea Breeding and Management, (pp. 47–71). Wallingford, UK: CAB International, CAB eBooks. doi: 10.1079/9781845932138.003.
- Berger, J. D., Milroy, S. P., Turner, N. C., Siddique, K. H. M., Imtiaz, M., & Malhotra, R. (2011). Chickpea evolution has selected for contrasting phenological mechanisms among different habitats. Euphytica , 180(1), 1–15. doi: 10.1007/s10681-011-0391-4.
- Cortinovis, G., Oppermann, M., Neumann, K., Graner, A., Gioia, T., Marsella, M., … Bitocchi, E. (2021). Towards the development, maintenance, and standardized phenotypic characterization of single-seed-descent genetic resources for common bean. Current Protocols , 1(5), e133. doi: 10.1002/cpz1.133.
- De Giovanni, C., Pavan, S., Taranto, F., Di Rienzo, V., Miazzi, M. M., Marcotrigiano, A. R., … Lotti, C. (2017). Genetic variation of a global germplasm collection of chickpea (Cicer arietinum L.) including Italian accessions at risk of genetic erosion. Physiology and Molecular Biology of Plants , 23(1), 197–205. doi: 10.1007/s12298-016-0397-4.
- Guerra-García, A., Gioia, T., von Wettberg, E., Logozzo, G., Papa, R., Bitocchi, E., & Bett, K. E. (2021). Intelligent characterization of lentil genetic resources: Evolutionary history, genetic diversity of germplasm, and the need for well-represented collections. Current Protocols , 1(5), e134. doi: 10.1002/cpz1.134.
- Gupta, S., Nawaz, K., Parween, S., Roy, R., Sahu, K., Kumar Pole, A., … Chattopadhyay, D. (2017). Draft genome sequence of Cicer reticulatum L., the wild progenitor of chickpea, provides a resource for agronomic trait improvement. DNA Research , 24(1), 1–10.
- Harlan, J. R., & de Wet, J. M. (1971). Toward a rational classification of cultivated plants. Taxon , 20(4), 509–517. doi: 10.2307/1218252.
- Harlan, J. R. (1992). Crops and man ( 2nd ed.). Madison, WI: American Society of Agronomy.
- IBPGR, ICRISAT, & ICARDA. (1993). Descriptors for chickpea (Cicer arietinum L.). International Board for Plant Genetic Resources, Rome, Italy; International Crops Research Institute for the Semi-Arid Tropics, Patancheru, India, and International Center for Agricultural Research in the Dry Areas, Aleppo, Syria.
- Kroc, M., Tomaszewska, M., Czepiel, K., Bitocchi, E., Oppermann, M., Neumann, K., … Susek, K. (2021). Towards development, maintenance, and standardized phenotypic characterization of single-seed-descent genetic resources for lupins. Current Protocols , 1(7), e191. doi: 10.1002/cpz1.191.
- Ladizinsky, G., & Adler, A. (1976). Genetic relationships among the annual species of Cicer L. Theoretical and Applied Genetics , 48(4), 197–203. doi: 10.1007/BF00527371.
- Li, Y., Ruperao, P., Batley, J., Edwards, D., Davidson, J., Hobson, K., & Sutton, T. (2017). Genome analysis identified novel candidate genes for ascochyta blight resistance in chickpea using whole genome re-sequencing data. Frontiers in Plant Science , 8, 359. doi: 10.3389/fpls.2017.00359.
- Li, Y., Ruperao, P., Batley, J., Edwards, D., Khan, T., Colmer, T. D., … Sutton, T. (2018). Investigating drought tolerance in chickpea using genome-wide association mapping and genomic selection based on whole-genome resequencing data. Frontiers in Plant Science , 9, 190. doi: 10.3389/fpls.2018.00190.
- Mascher, M., Schreiber, M., Scholz, U., Graner, A., Reif, J. C., & Stein, N. (2019). Genebank genomics bridges the gap between the conservation of crop diversity and plant breeding. Nature Genetics , 51(7), 1076–1081. doi: 10.1038/s41588-019-0443-6.
- Parween, S., Nawaz, K., Roy, R., Pole, A. K., Suresh, B. V., Misra, G., … Chattopadhyay, D. (2015). An advanced draft genome assembly of a desi type chickpea (Cicer arietinum L.). Scientific Reports , 5(1), 1–14. doi: 10.1038/srep12806.
- Pavan, S., Lotti, C., Marcotrigiano, A. R., Mazzeo, R., Bardaro, N., Bracuto, V., … Ricciardi, L. (2017). A distinct genetic cluster in cultivated chickpea as revealed by genome-wide marker discovery and genotyping. The Plant Genome , 10(2). doi: 10.3835/plantgenome2016.11.0115.
- Penmetsa, R. V., Carrasquilla-Garcia, N., Bergmann, E. M., Vance, L., Castro, B., Kassa, M. T., … Cook, D. R. (2016). Multiple post-domestication origins of kabuli chickpea through allelic variation in a diversification-associated transcription factor. New Phytologist , 211(4), 1440–1451. doi: 10.1111/nph.14010.
- Plekhanova, E., Vishnyakova, M. A., Bulyntsev, S., Chang, P. L., Carrasquilla-Garcia, N., Negash, K., … Nuzhdin, S. V. (2017). Genomic and phenotypic analysis of Vavilov's historic landraces reveals the impact of environment and genomic islands of agronomic traits. Scientific Reports , 7(1), 1–12. doi: 10.1038/s41598-017-05087-5.
- Rocchetti, L., Bellucci, E., Cortinovis, G., Di Vittori, V., Lanzavecchia, G., Frascarelli, G., … Papa, R. (2020). The development of a European and Mediterranean Chickpea Association Panel (EMCAP). Agronomy , 10(9), 1417. doi: 10.3390/agronomy10091417.
- Roorkiwal, M., Jarquin, D., Singh, M. K., Gaur, P. M., Bharadwaj, C., Rathore, A., … Varshney, R. K. (2018). Genomic-enabled prediction models using multi-environment trials to estimate the effect of genotype×environment interaction on prediction accuracy in chickpea. Scientific Reports , 8(1), 1–11. doi: 10.1038/s41598-018-30027-2.
- Roorkiwal, M., Bharadwaj, C., Barmukh, R., Dixit, G. P., Thudi, M., Gaur, P. M., … Varshney, R. K. (2020). Integrating genomics for chickpea improvement: Achievements and opportunities. Theoretical and Applied Genetics , 133(5), 1703–1720. doi: 10.1007/s00122-020-03584-2.
- Samineni, S., Sajja, S. B., Mondal, B., Chand, U., Thudi, M., Varshney, R. K., & Gaur, P. M. (2021). MAGIC lines in chickpea: Development and exploitation of genetic diversity. Euphytica , 217(7), 1–12. doi: 10.1007/s10681-021-02874-0.
- Shin, M. G., Bulyntsev, S. V., Chang, P. L., Korbu, L. B., Carrasquila-Garcia, N., Vishnyakova, M. A., … Nuzhdin, S. V. (2019). Multi-trait analysis of domestication genes in Cicer arietinum – Cicer reticulatum hybrids with a multidimensional approach: Modeling wide crosses for crop improvement. Plant Science , 285, 122–131. doi: 10.1016/j.plantsci.2019.04.018.
- Smýkal, P., Coyne, C. J., Ambrose, M. J., Maxted, N., Schaefer, H., Blair, M. W., … Varshney, R. K. (2015). Legume crops phylogeny and genetic diversity for science and breeding. Critical Reviews in Plant Sciences , 34(1-3), 43–104. doi: 10.1080/07352689.2014.897904.
- Sokolkova, A., Bulyntsev, S. V., Chang, P. L., Carrasquilla-Garcia, N., Igolkina, A. A., Noujdina, N. V., … Samsonova, M. G. (2020). Genomic analysis of Vavilov's historic chickpea landraces reveals footprints of environmental and human selection. International Journal of Molecular Sciences , 21(11), 3952. doi: 10.3390/ijms21113952.
- Thudi, M., Chitikineni, A., Liu, X., He, W., Roorkiwal, M., Yang, W., … Varshney, R. K. (2016). Recent breeding programs enhanced genetic diversity in both desi and kabuli varieties of chickpea (Cicer arietinum L.). Scientific Reports , 6(1), 1–10. doi: 10.1038/srep38636.
- Toker, C., Berger, J., Eker, T., Sari, D., Sari, H., Gokturk, R. S., … von Wettberg, E. J. (2021). Cicer turcicum : A new Cicer species and its potential to improve chickpea. Frontiers in Plant Science , 12, 587. doi: 10.3389/fpls.2021.662891.
- van der Maesen, L. J. G., Maxted, N., Javadi, F., Coles, S., & Davies, A. M. R. (2007). Taxonomy of the genus Cicer revisited, In S. S. Yadav, R. J. Redden, W. Chen, B. Sharma (Eds.) Chickpea breeding and management (pp. 14–46). Wallingford, UK: CAB International, CAB eBooks. doi: 10.1079/9781845932138.002.
- Varshney, R. K., Song, C., Saxena, R. K., Azam, S., Yu, S., Sharpe, A. G., … Cook, D. R. (2013). Draft genome sequence of chickpea (Cicer arietinum) provides a resource for trait improvement. Nature Biotechnology , 31(3), 240–246. doi: 10.1038/nbt.2491.
- Varshney, R. K., Thudi, M., Roorkiwal, M., He, W., Upadhyaya, H. D., Yang, W., … Liu, X. (2019). Resequencing of 429 chickpea accessions from 45 countries provides insights into genome diversity, domestication and agronomic traits. Nature Genetics , 51(5), 857–864. doi: 10.1038/s41588-019-0401-3.
- Varshney, R. K., Roorkiwal, M., Sun, S., Bajaj, P., Chitikineni, A., Thudi, M., … Liu, X. (2021). A chickpea genetic variation map based on the sequencing of 3,366 genomes. Nature , 599, 622–627. doi: 10.1038/s41586-021-04066-1.
- von Wettberg, E. J., Chang, P. L., Başdemir, F., Carrasquila-Garcia, N., Korbu, L. B., Moenga, S. M., … Cook, D. R. (2018). Ecology and genomics of an important crop wild relative as a prelude to agricultural innovation. Nature Communications , 9(1), 1–13. doi: 10.1038/s41467-018-02867-z.
Internet Resources
FAOstat (2019) Food and Agriculture Organization of the United Nations, 2019. Production: Crops.
Genesys (2021). Genesys Global Portal on Plant Genetic Resources. Online platform hosting information about Plant Genetic Resources for Food and Agriculture (PGRFA) conserved in gene banks worldwide.
Crop Ontology, developed by several groups of the Consultative Group on International Agricultural Research (CGIAR), provides descriptions of different crop traits.
BioProject is a collection of biological data related to a single initiative, originating from a single organization or from a consortium.
INCREASE Project website
Citing Literature
Number of times cited according to CrossRef: 9
- Madita Lauterberg, Henning Tschiersch, Yusheng Zhao, Markus Kuhlmann, Ingo Mücke, Roberto Papa, Elena Bitocchi, Kerstin Neumann, Implementation of theoretical non-photochemical quenching (NPQ(T)) to investigate NPQ of chickpea under drought stress with High-throughput Phenotyping, Scientific Reports, 10.1038/s41598-024-63372-6, 14 , 1, (2024).
- Palermo Nadia, Buffagni Valentina, Vurro Filippo, Impollonia Giorgio, Pignone Domenico, Janni Michela, T Nguyen Henry, Elena Dembech, Marmiroli Nelson, From landraces to haplotypes, exploiting a genomic and phenomic approach to identify heat tolerant genotypes within durum wheat landraces., Environmental and Experimental Botany, 10.1016/j.envexpbot.2024.105986, (105986), (2024).
- Weidong Chen, Lyndon Porter, Cool-season grain legumes, Agrios' Plant Pathology, 10.1016/B978-0-12-822429-8.00025-X, (703-713), (2024).
- Simone Papalini, Valerio Di Vittori, Alice Pieri, Marina Allegrezza, Giulia Frascarelli, Laura Nanni, Elena Bitocchi, Elisa Bellucci, Tania Gioia, Luis Guasch Pereira, Karolina Susek, Maud Tenaillon, Kerstin Neumann, Roberto Papa, Challenges and Opportunities behind the Use of Herbaria in Paleogenomics Studies, Plants, 10.3390/plants12193452, 12 , 19, (3452), (2023).
- Madita Lauterberg, Henning Tschiersch, Roberto Papa, Elena Bitocchi, Kerstin Neumann, Engaging Precision Phenotyping to Scrutinize Vegetative Drought Tolerance and Recovery in Chickpea Plant Genetic Resources, Plants, 10.3390/plants12152866, 12 , 15, (2866), (2023).
- Jens Keilwagen, Heike Lehnert, Ekaterina D. Badaeva, Hakan Özkan, Shivali Sharma, Peter Civáň, Benjamin Kilian, Finding needles in a haystack: identification of inter-specific introgressions in wheat genebank collections using low-coverage sequencing data, Frontiers in Plant Science, 10.3389/fpls.2023.1166854, 14 , (2023).
- Mustafa Bulut, Regina Wendenburg, Elena Bitocchi, Elisa Bellucci, Magdalena Kroc, Tania Gioia, Karolina Susek, Roberto Papa, Alisdair R. Fernie, Saleh Alseekh, A comprehensive metabolomics and lipidomics atlas for the legumes common bean, chickpea, lentil and lupin, The Plant Journal, 10.1111/tpj.16329, 116 , 4, (1152-1171), (2023).
- Francesca Brescia, Fabiano Sillo, Elisabetta Franchi, Ilaria Pietrini, Vincenzo Montesano, Giovanni Marino, Matthew Haworth, Elisa Zampieri, Danilo Fusini, Martino Schillaci, Roberto Papa, Chiara Santamarina, Federico Vita, Walter Chitarra, Luca Nerva, Giannantonio Petruzzelli, Carmelo Mennone, Mauro Centritto, Raffaella Balestrini, The ‘microbiome counterattack’: Insights on the soil and root‐associated microbiome in diverse chickpea and lentil genotypes after an erratic rainfall event, Environmental Microbiology Reports, 10.1111/1758-2229.13167, 15 , 6, (459-483), (2023).
- Dürdane MART, Chickpea (Cicer arietinum L.): A Current Review, MAS Journal of Applied Sciences, 10.52520/masjaps.v7i2id188, 7 , 2, (372-379), (2022).