Tissue-Specific Isolation of Tagged Arabidopsis Plastids
Clément Boussardon, Clément Boussardon, Olivier Keech, Olivier Keech
Arabidopsis
biotin-streptavidin interaction
editable Golden Gate plasmids
plastids
tissue-specific isolation
Abstract
Plastids are found in all plant cell types. However, most extraction methods to study these organelles are performed at the organ level (e.g., leaf, root, fruit) and do not allow for tissue-specific resolution, which hinders our understanding of their physiology. Therefore, IPTACT (Isolation of Plastids TAgged in specific Cell Types) was developed to isolate plastids in a tissue-specific manner in Arabidopsis thaliana (Arabidopsis). Plastids are biotinylated using one-shot transgenic lines, and tissue specificity is achieved with a suitable promoter as long as such a promoter exists. Cell-specific biotinylated plastids are then isolated with 2.8-µm streptavidin beads. Plastids extracted by IPTACT are suitable for RNA or protein isolation and subsequent tissue-specific OMICs analyses. This method provides the user with a powerful tool to investigate plastidial functions at cell-type resolution. Furthermore, it can easily be combined with studies using diverse genetic backgrounds and/or different developmental or stress conditions. © 2022 The Authors. Current Protocols published by Wiley Periodicals LLC.
Basic Protocol 1 : Promoter cloning and plant selection
Basic Protocol 2 : Isolation of biotinylated plastids
Basic Protocol 3 : Quality control of isolated plastids
INTRODUCTION
In plants, plastids are one of the three genome-containing compartments of the cell, together with the nucleus and mitochondria. Although mostly known for being the site of photosynthesis in photosynthetic tissues, plastids vary in size, shape, internal structures, and metabolic functions (Cayla et al., 2015; Hibberd & Quick, 2002; Majeran, Cai, Sun, & van Wijk, 2005). This has led to an extended nomenclature that defines the types of plastids found in different cell types, i.e., amyloplast, chloroplast, chromoplast, dessicoplast, elaioplast, etioplast, gerontoplast, leucoplast, phenyloplast, proplast, proteinoplast, and even xyloplast (Pinard & Mizrachi, 2018). As a sub-compartment of the cell, their isolation is often required to study them. For decades, methods based on differential centrifugations, and generally associated with Percoll or sucrose gradients, were employed to purify plastids for further experimentation (see, e.g., Emes & England, 1986; Jacobson, 1968; Miflin & Beevers, 1974). These methods are used to analyze the proteomes of etioplast from rice (von Zychlinski et al., 2005); amyloplast from wheat (Balmer, Vensel, DuPont, Buchanan, & Hurkman, 2006); chloroplast from pea (Bayer, Stael, Csaszar, & Teige, 2011); plastid from Arabidopsis thaliana (Arabidopsis) and maize (Huang et al., 2013); chloroplast envelope of pea, Medicago truncatula , and Arabidopsis (Simm et al., 2013); chloroplast from tomato (Tamburino et al., 2017); and chromoplast from tomato and pepper fruits (Barsan, Kuntz, & Pech, 2017; Suzuki et al., 2015), or for sequencing plastid genomes, as shown with Festuca species, for example (Islam, Buttelmann, Chekhovskiy, Kwon, & Saha, 2019). A lot of work was also done with the model plant species Arabidopsis , for which valuable proteomic data were obtained with intact plastids or subfractions of plastids (Chen, Yuan, & Schroder, 2016; Ferro et al., 2002; Froehlich et al., 2003; Hall, Mishra, & Schroder, 2011; Kleffmann et al., 2004; Peltier, Ytterberg, Sun, & van Wijk, 2004; see Current Protocols article: Seigneurin-Berny, Salvi, Joyard, & Rolland, 2008). Although most proteome data for plastids were obtained with leaves and were thus for chloroplasts, a few proteomic studies have focused on plastids from other organs. For example, plastids from Arabidopsis roots were isolated using a Percoll gradient, and organellar root proteins were separated using a 2D-gel approach, which led to identification of 84 proteins by LC/MS (Grabsztunowicz, Rokka, Farooq, Aro, & Mulo, 2020). Taken together, these methods, which have strongly contributed to deepening our understanding of plastid functions, are still valid and highly valuable. However, they generally rely on the isolation of plastids from an entire organ, which does not offer the depth of studying organelle functions at “cell-type” resolution. Indeed, an organ such as a leaf, for example, contains at least four different cell types (i.e., mesophyll, phloem, epidermis, and stomata), which fulfill different functions. In turn, this means that plastids have defined roles that vary with the cell type hosting the organelles. These variations include strong modulations of the transcriptome, proteome, and metabolome (Beltran et al., 2018; Hibberd & Quick, 2002; Majeran et al., 2005).
Recently, we developed a method, tailor-made for Arabidopsis , to isolate mitochondria from different cell types. The method involves the in vivo tagging of the outer membrane mitochondrial protein 64 (OM64) followed by isolation using magnetic beads (Boussardon & Keech, 2022; Boussardon, Przybyla-Toscano, Carrie, & Keech, 2020). The cell-type specificity is achieved by use of tissue-specific promoters, which confers the exclusivity of in vivo biotinylation and subsequent binding to beads. We have now adapted this strategy to plastids in a method named IPTACT (Isolation of Plastids Tagged in specific Cell Types; Boussardon, Carrie, & Keech, in press), which allowed the successful isolation and proteomic study of plastids from mesophyll and vascular cells. To do so, we used a translational fusion leading to expression of the translocon at the outer membrane of chloroplasts 64-I (TOC64) protein in frame with the eGFP and the biotin ligase recognition peptide (BLRP) at its C-terminal end. On the same plasmid, biotin ligase (BirA), allowing the biotinylation of BLRP, was ubiquitously expressed using the UBQ10 constitutive promoter. In the case of mesophyll and vascular cell plastid isolation, TOC64-eGFP-BLRP cassette expression was driven by the CAB3 and SUC2 promoters, respectively. The aim was to study the metabolic functions of plastids from phloem, as little is known about their molecular and physiological roles apart from a potential role in the defense response against abiotic stress. The tissue-specific isolation of plastids allowed the identification of nearly 1700 proteins, among which about 1400 are predicted to be plastidial and about 700 are confirmed according to SUBAcon (SUBA 5; Boussardon et al., 2023; Hooper, Castleden, Tanz, Aryamanesh, & Millar, 2017). We discovered that although both proteomes were globally similar, plastids from vascular tissues had an accumulation of proteins associated with plastoglobuli and jasmonate metabolism as well as enhanced redox turnover (Boussardon et al., in press).
In this article, we describe step-by-step protocols to successfully purify plastids from any cell type or developmental stage in Arabidopsis , providing that an appropriate promoter exists. First, Golden Gate plasmids are edited to introduce the desired promoter, and plants are transformed and verified by PCR and confocal imaging (Basic Protocol 1). Then, biotinylated plastids are isolated using streptavidin magnetic beads (Basic Protocol 2). Finally, the quality of the isolated plastids is checked (Basic Protocol 3). See Figure 1 for an overview of the procedures.
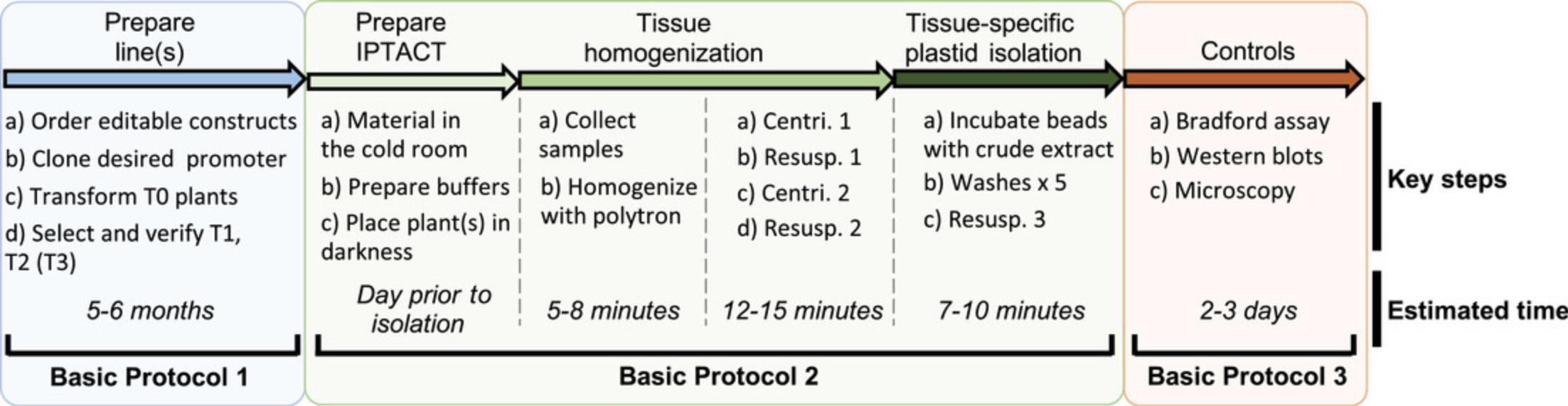
STRATEGIC PLANNING
The user can either design their own constructs or request the already available plasmids from the corresponding author. Presently, the authors can provide a set of three editable plasmids containing different selection markers, i.e., kanamycin, Basta, and hygromycin (Fig. 2). If the user aims to isolate plastids from insertion lines issued by a stock center (e.g., NASC), they should ask for the appropriate selection marker so that it does not compete with the T-DNA selection marker. Transform the plasmids into the DB3.1 ccdB -resistant Escherichia coli strain. The IPTACT strategy relies on replacement of the ccdB cassette, a negative selection marker, using the Bsa I restriction enzyme. Therefore, the promoter should be free of any Bsa I restriction site. Alternatively, the Bsa I site could be removed by PCR mutagenesis, though one should ensure that it does not affect the expression quality.
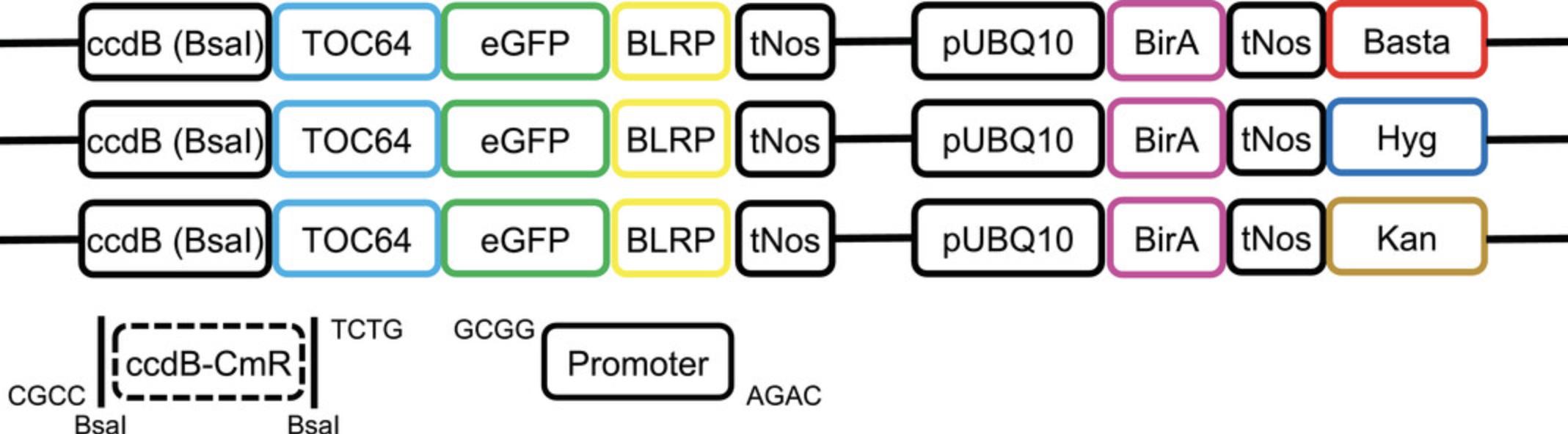
NOTE : Experiments involving PCR require extremely careful technique to prevent contamination.
Basic Protocol 1: PROMOTER CLONING AND PLANT SELECTION
This protocol describes the procedure to prepare lines containing the desired construction. Once the promoter is defined, Bsa I restriction sites are cloned on both ends of the promoter by PCR. When Bsa I restriction enzyme and T4 DNA ligase are used, the promoter replaces the ccdB negative selection cassette. Agrobacterium tumefaciens competent cells are transformed, and A. thaliana ecotypes or mutants are transformed by floral dipping. T1 transformed plants are first selected on kanamycin, Basta, or hygromycin. Then, TOC64-eGFP-BLRP protein fluorescence and tissue localization are confirmed by fluorescence imaging. Although T1 hemizygous selected lines are usable for experimental work, it is recommended to propagate seeds to the T2 and T3 generations.
Materials
-
Freely available Golden Gate construct containing ccdB negative selection cassette (for editable Golden Gate plasmids, backbone based upon pSEVA641; Chiasson et al., 2019)
-
DB3.1 thermocompetent E. coli strain, tolerant to ccdb
-
LB agar plate supplemented with kanamycin (50 µg/ml)
-
Liquid LB supplemented with kanamycin (50 µg/ml)
-
Plasmid extraction kit (EZNA, Omega Bio-tek, cat. no. D6942-00S)
-
dNTP mix (10 mM each; Thermo Fisher, cat. no. R0192)
-
High-fidelity Taq polymerase (e.g., Phusion Taq polymerase, Thermo Fisher, cat. no. F530S), including 5× buffer
-
Nuclease-free water
-
Gel or PCR purification kit (EZNA, Omega Bio-tek, cat. no. D2500-01)
-
10 mM ATP (NEB, cat. no. P0756L)
-
Bsa I (NEB, cat. no. R3733S), including 10× buffer G
-
T4 DNA ligase (NEB, cat. no. M0202S)
-
DH5α thermocompetent E. coli strain
-
Primer on the promoter and a primer on TOC64 (TOC64-rev) or eGFP (eGFP-rev):
- TOC64-rev: AGGAACAGCTGGCAATGTTG
- eGFP-rev: CTTGTACAGCTCGTCCATGC
-
DreamTaq DNA polymerase (Thermo Fisher, cat. no. EP0703), including 10× buffer
-
tumefaciens GV3101 competent cells (pMP90, pSOUP)
-
Liquid LB supplemented with kanamycin (50 µg/ml), gentamycin (10 µg/ml), or rifampicin (25 µg/ml)
-
LB agar plate supplemented with kanamycin (50 µg/ml), gentamycin (10 µg/ml), or rifampicin (25 µg/ml)
-
50% (v/v) glycerol
-
Liquid nitrogen
-
Plant transformation medium (see recipe; make fresh)
-
Arabidopsis ecotype or mutant [here, Columbia (Col-0)]
-
½ MS + 0.1% (w/v) sucrose medium containing right herbicide (50 μg/ml kanamycin, 50 μg/ml hygromycin, or 10 μg/ml Basta)
-
Soil
-
28°C and 37°C incubators
-
15-ml polypropylene centrifuge tubes (Sigma Aldrich, cat. no. CLS430791-500EA)
-
42°C heating block
-
28°C and 37°C shaking incubators (Infors™ Multitron Standard Shaker, Fisher Scientific, cat. no. 11971582)
-
1.5- and 2-ml Eppendorf tubes (Eppendorf Safe-Lock Tubes, Eppendorf, cat. no. 0030120086 and 0030120094)
-
Electroporation cuvette
-
Electroporator (Gene Pulser electroporation system, Bio-Rad, cat. no. 1652660)
-
500-ml Erlenmeyer flasks
-
250-ml bottle (Nalgene, Outnorth, cat. no. A041549)
-
Standard tabletop centrifuge
-
Plastic bags or mini greenhouse domes
-
Growth chambers
-
Confocal microscope (e.g., LSM780, Zeiss)
-
5-ml polypropylene Falcon tubes
-
Additional reagents and equipment for PCR (e.g., thermal cycler system, T100 Thermal Cycler, Bio-Rad, cat. no. 1861096), Sanger sequencing (e.g., Mix2Seq Kit, Eurofins Genomics), and floral dipping (Clough & Bent, 1998)
Promoter cloning and Agrobacterium transformation
1.After obtaining the freely available Golden Gate construct containing the ccdB negative selection cassette, transform DB3.1 thermocompetent E. coli strain using 30 to 50 ng plasmid. Streak bacteria on an LB agar plate supplemented with kanamycin (50 µg/ml) and allow colonies to grow overnight at 37°C. Check colonies by PCR and inoculate 5 ml liquid LB supplemented with kanamycin (50 µg/ml) for pre-culture a 15-ml polypropylene centrifuge tube. Grow bacteria overnight at 37°C and isolate plasmids using plasmid extraction kit. Check concentration with a spectrophotometer.
2.Design primers containing Bsa I restriction sites flanking the promoter region to be cloned:
- 5´ATGAAGACTTTACGGGTCTCAGCGG + 5´ promoter (underlined: BsaI , bold : overhangs)
- 3´ TGAAGACTTCAGAGGTCTCACAGA + 3´ promoter (underlined: BsaI , bold : overhangs).
3.Amplify promoter with Bsa I overhangs using a high-fidelity Taq polymerase by PCR. If a Bsa I site is present in the promoter, edit it by PCR mutagenesis, turning GG** TCTC into GG A**CTC. For mutagenesis, order primers containing the T-to-A polymorphism on both the forward and reverse strands. Perform PCR with the primer containing the 5’ of the promoter (+Bsa I) and the reverse primer with the polymorphism. Perform another PCR with the primer containing the 3’ of the promoter (+Bsa I) and the forward primer with polymorphism. Once both products are obtained, dilute both 1:10 and mix 0.5 µl of each to be used as a matrix for the second PCR. Perform a second PCR using both 5´ and 3´ Bsa I primers and confirm a product of the expected size (for the promoter flanked by Bsa I sites, including a polymorphism) by Sanger sequencing. Perform PCRs as described below:
Reaction mix:
Reagent | Amount for one reaction |
Primer 1 (10 µM) | 1 µl |
Primer 2 (10 µM) | 1 µl |
dNTP mix (10 mM each) | 0.4 µl |
5× buffer | 4 µl |
Taq polymerase (2 U/µl) | 0.2 µl |
Nuclease-free water | 12.4 µl |
DNA matrix | 1 µl |
Reaction conditions:
Initial step: | 3 min | 98°C |
35 cycles: | 10 s | 98°C |
30 s | 60°C | |
30 s/kb | 72°C | |
Final step: | 5 min | 72°C. |
4.Purify PCR product using a gel or PCR purification kit.
5.Perform Golden Gate reaction as follows:
Reaction mix:
Reagent | Amount |
Editable plasmid (step 1) | 100 ng |
Purified PCR product (step 4) | 5 µl |
10× buffer G | 1.5 µl |
10 mM ATP | 1.5 µl |
20 U/µl BsaI | 0.75 µl |
5 U/µl T4 DNA ligase | 0.75 µl |
Nuclease-free water | to 15 µl |
Reaction conditions:
6 cycles: | 10 min | 37°C |
10 min | 16°C | |
Final steps: | 10 min | 37°C |
20 min | 65°C. |
6.Gently mix 50 µl DH5α thermocompetent E. coli strain with 5 µl Golden Gate reaction in a 1.5-ml Eppendorf tube and incubate on ice for 30 min. Apply a thermal shock at 42°C in a heating block for 45 s and transfer on ice for 5 min. Inoculate 650 µl liquid LB supplemented with kanamycin (50 µg/ml) and let grow at 37°C in a shaking incubator for 1 hr. Plate 150 µl on an LB agar plate supplemented with kanamycin (50 µg/ml) and grow overnight at 37°C.
7.Select positive clones by colony PCR using a primer on the promoter and a primer on TOC64 (TOC64-rev) or eGFP (eGFP-rev) and then extract plasmids:
Reaction mix:
Reagent | Amount for one reaction |
Primer 1 (10 µM) | 0.5 µl |
Primer 2 (10 µM) | 0.5 µl |
dNTP mix (10 mM each) | 0.25 µl |
10× buffer | 1.25 µl |
DreamTaq polymerase (5 U/µl) | 0.1 µl |
Nuclease-free water | to 12.5 µl |
Reaction conditions:
Initial step: | 5 min | 95°C |
35 cycles: | 30 s | 95°C |
30 s | 57-60°C | |
1 min/kb | 72°C | |
Final step: | 5 min | 72°C. |
8.Mix 100 ng plasmid with 100 µl A. tumefaciens GV3101 competent cells in a 1.5-ml Eppendorf tube. Load mix into an electroporation cuvette and set electroporator to 1.5 kV, 200 Ω resistance, and 25 µF capacitance, with a time constant of 4 ms. Quickly inoculate 650 µl liquid LB supplemented with kanamycin (50 µg/ml), gentamycin (10 µg/ml), or rifampicin (25 µg/ml) in a 2-ml Eppendorf tube and let culture grow for 2 hr at 28°C in a shaking incubator. Streak on an LB agar plate supplemented with kanamycin (50 µg/ml), gentamycin (10 µg/ml), or rifampicin (25 µg/ml). Select positive clones by colony PCR after 2 to 4 days of incubation at 28°C.
9.Make a glycerol stock by mixing 700 µl overnight culture and 300 µl of 50% glycerol in a 2-ml Eppendorf tube, flash-freeze in liquid nitrogen, and store at −80°C.
Plant transformation and selection of positive plants
10.Inoculate 1 ml liquid LB supplemented with kanamycin (50 µg/ml), gentamycin (10 µg/ml), or rifampicin (25 µg/ml) with a positive clone in a 2-ml Eppendorf tube. Incubate overnight at 28°C in a shaking incubator. Then, inoculate an overnight culture by pouring 1 ml pre-culture into 200 ml liquid LB supplemented with kanamycin (50 µg/ml), gentamycin (10 µg/ml), or rifampicin (25 µg/ml) in a 500-ml Erlenmeyer flask and incubate at 28°C, 200 rpm, in a shaking incubator.
11.Centrifuge culture in a 250-ml bottle for 30 min at 5000 × g , remove supernatant, and resuspend in 200 ml plant transformation medium.
12.Transform desired Arabidopsis mutant or ecotype by floral dipping (e.g., in a 250-ml Erlenmeyer flask) for 2 min (Clough & Bent, 1998). Cover plants with a plastic bag or a mini greenhouse dome for 2 days and keep under long-day conditions. Then, transfer plants into growth chambers under long-day conditions. Harvest T1 seeds 1 month after transformation. Store seeds at room temperature for 1 week to lift dormancy and then proceed to step 13.
13.Select T1 plantlets on ½ MS + 0.1% sucrose medium containing the right herbicide (50 μg/ml kanamycin, 50 μg/ml hygromycin, or 10 μg/ml Basta) for 1 week.
14.Transfer transgenic plantlets into soil and grow under either a short-day photoperiod (SD: light 8 hr at 22°C, dark 16 hr at 17°C) or a long-day photoperiod (LD: light 16 hr at 22°C, dark 8 hr at 17°C).
15.Confirm fluorescence and tissue localization of TOC64-eGFP-BLRP protein by imaging with a confocal microscope.
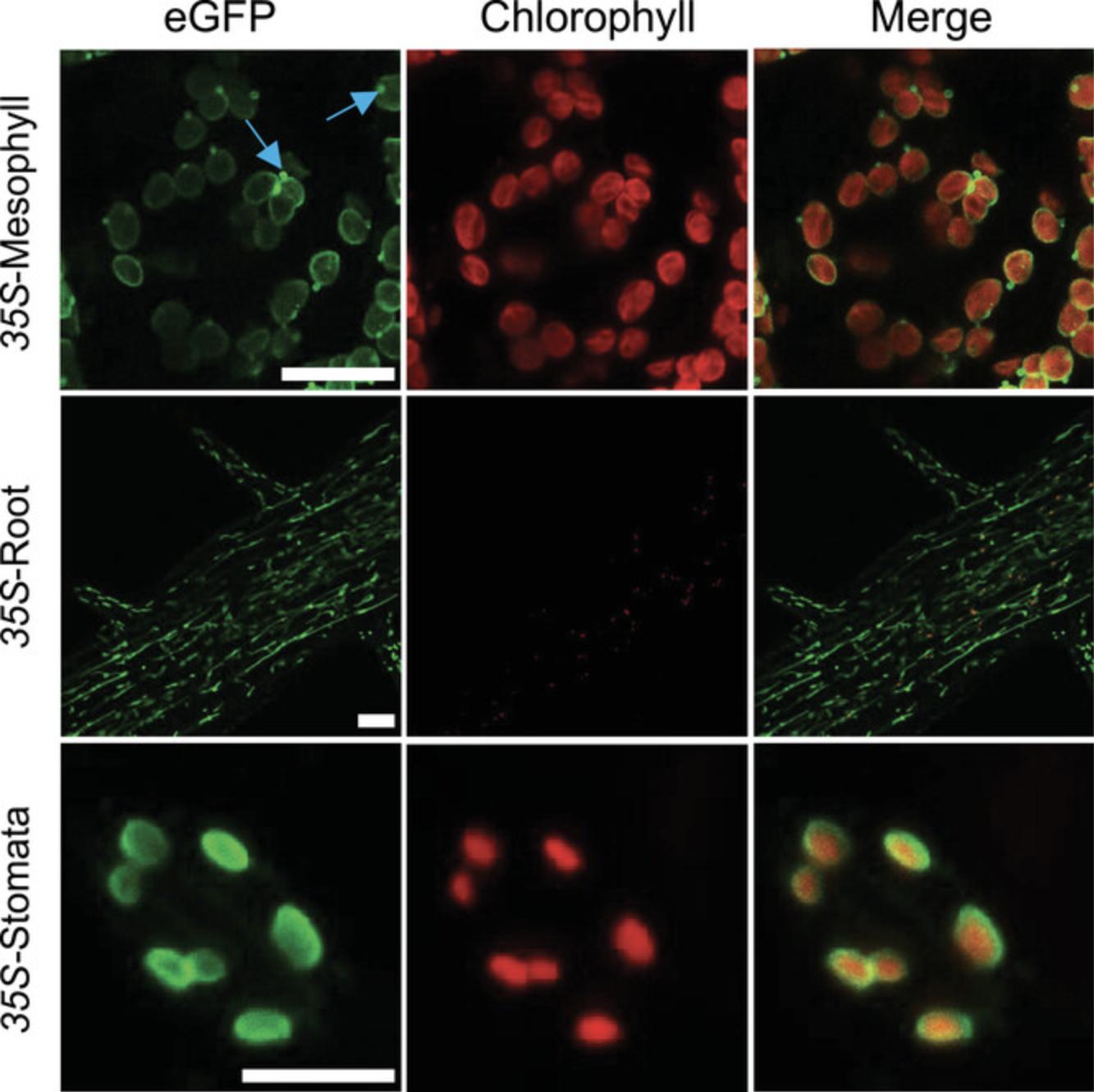
16.Keep positive plants and obtain T2 segregating seeds. Select plantlets on ½ MS + 0.1% sucrose medium containing the right herbicide (75% plantlets resistant) and check again by PCR and confocal imaging. Keep best T2 plants and collect T3 seeds. Select plantlets on ½ MS + 0.1% sucrose medium containing the right herbicide.
Basic Protocol 2: ISOLATION OF BIOTINYLATED PLASTIDS
This protocol describes the procedure to isolate tissue-specific plastids from plants expressing TOC64-eGFP-BLRP in the desired tissue from Basic Protocol 1.It is recommended to perform the experiment in a cold room set at 6°C and under a green light. When samples are collected, tissues are homogenized, and different centrifugation steps are performed to obtain a crude extract. This extract is enriched in intact plastids but still contains a high amount of contaminants. Then, the crude extract is put in contact with 2.8-µm streptavidin magnetic beads. As plastids are biotinylated, they will interact with the magnetic beads. Non-biotinylated plastids are discarded when the supernatant is discarded. Washes are performed to remove contaminants trapped on the beads. Pure, tissue-specific plastids are ready for quality control (Basic Protocol 3) and further experimentation.
Materials
-
T2 or T3 IPTACT plants (see Basic Protocol 1)
-
Isolation buffer (see recipe; make fresh if possible; Aronsson & Jarvis, 2002)
-
HMS buffer (see recipe; Aronsson & Jarvis, 2002)
-
Streptavidin magnetic beads (Dynabeads M-280 Streptavidin, Thermo Fisher, cat. no. 11205D)
-
Cold room, ideally with green light (TL-D Colored 36W Green 1SL/25, Philips, cat. no. 928048501705)
-
Plastic funnel (Nalgene PPCO Powder Funnels 65 mm diameter, Thermo Fisher, cat. no. 4252-0065)
-
200-ml glass beaker
-
Miracloth or 20-µm nylon mesh
-
Polytron (used in our work but discontinued: Kinematica Polytron™ PT 10/35 Homogenizer with PCU-11 Controller; available online: Kinematica Polytron™ PT 2500 E, Fisher Scientific, cat. no. 10785812)
-
50-ml plastic beaker
-
Refrigerated centrifuge (Beckman Avanti J-20XP, GMI, cat. no. 8043-30-1171), 4°C
-
50-ml polycarbonate centrifugation tubes (29 × 104 mm; Beckman Coulter, cat. no. 363647)
-
Paint brush
-
2-ml Eppendorf tubes
-
Vortex (Vortex Genie2 120V, Stellar Scientific, cat. no. SI-0236)
-
Magnet (Miltenyi Biotec, MiniMACS Separator)
-
Tweezers (optional)
-
Stand (e.g., a tube rack)
Tissue homogenization
1.Transfer T2 or T3 IPTACT plants to darkness the day prior to extraction.
2.Pre-cool buffers and equipment by leaving them in a cold room (set at 6°C) the day prior to isolation.
3.Install a plastic funnel on a 200-ml glass beaker and place Miracloth or 20-µm nylon mesh on funnel.
4.Pre-wet another piece of Miracloth or mesh with isolation buffer and create a “pillow” of isolation buffer in the 200-ml glass beaker.
5.Collect plant material in the early morning in the cold room.
6.Homogenize plants using a polytron (speed of 4 out of 11 (∼40%), 2-s pulses, repeat four times) in 20 ml isolation buffer in a 50-ml plastic beaker.
7.Filter homogenate through the double layer of Miracloth or 20-µm nylon mesh prepared in steps 3 and 4.
8.Homogenize debris retained in the Miracloth in 20 ml isolation buffer and filter two times.
9.Centrifuge homogenate in a 50-ml polycarbonate centrifugation tube for 5 min at 1000 × g , 4°C.
10.Discard supernatant and gently resuspend pellet in 20 ml HMS buffer using a paint brush.
11.Repeat step 9.
12.Remove supernatant and gently resuspend pellet in 4 ml HMS buffer using a brush, obtaining the crude extract, and transfer to 2-ml Eppendorf tubes.
Preparation of magnetic beads
13.Vortex vial with streptavidin magnetic beads for 30 s for resuspension.
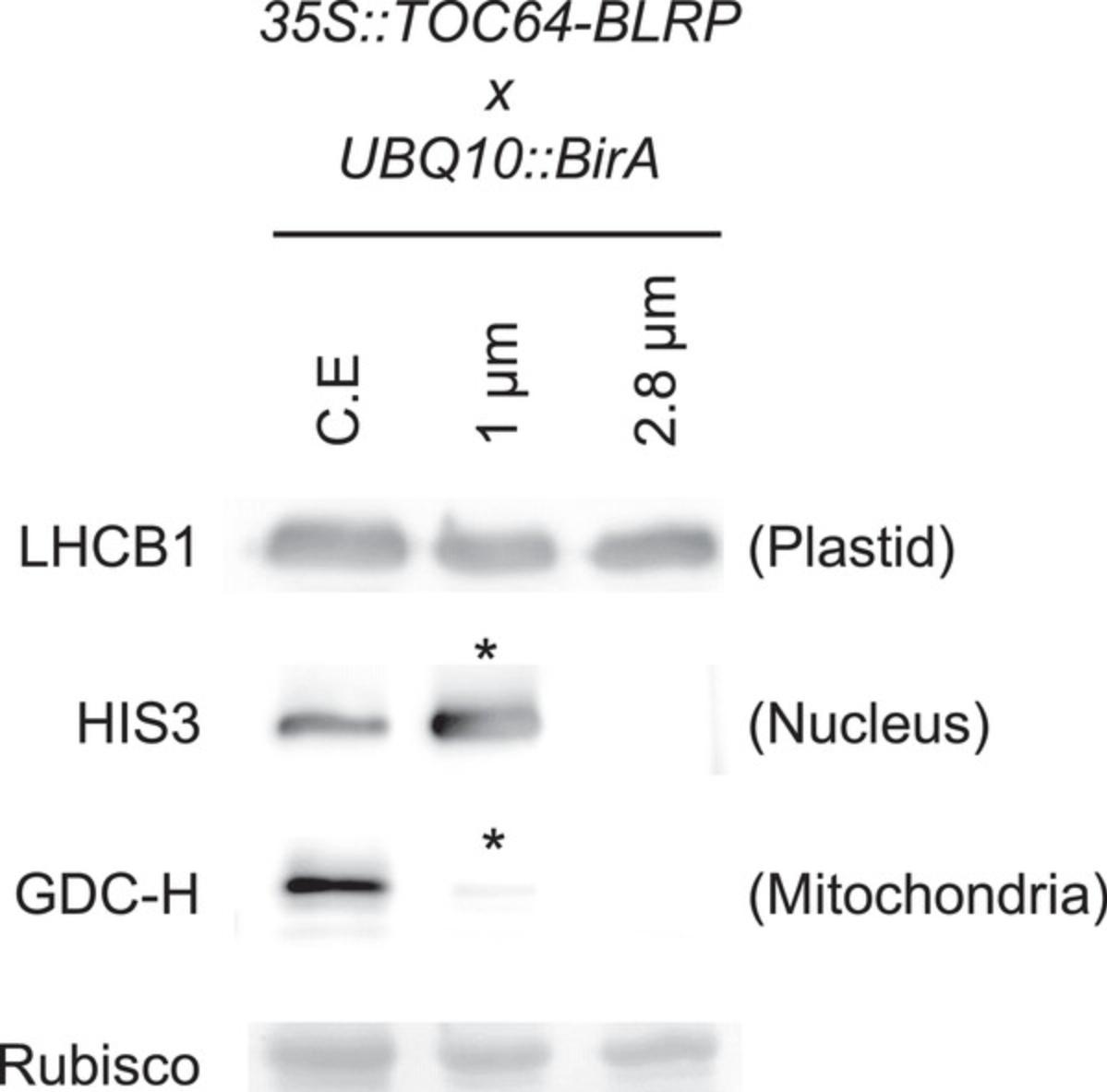
14.Aliquot 30 µl beads into a 2-ml Eppendorf tube.
15.Add 1 volume (30 µl) of HMS buffer and gently resuspend beads by pipetting until the solution is homogeneous.
16.Separate beads with a magnet for 1 min.
17.Discard supernatant and rinse with HMS buffer (twice the bead volume, i.e., 60 µl here). Repeat three times total.
18.Discard supernatant and finally resuspend beads with 30 µl HMS buffer.
Tissue-specific plastid isolation
19.Mix 1.7 ml crude extract (step 12) with magnetic beads (step 18).
20.Hold tube, with a closed cap, with index finger and thumb or tweezers and invert tube for 1 min, making sure that the sample does not warm up.
21.Place tube upright on a stand (e.g., a tube rack) and apply magnet (the magnet should touch the tube). Separate beads with the magnet for 2 min and gently collect supernatant by pipetting.
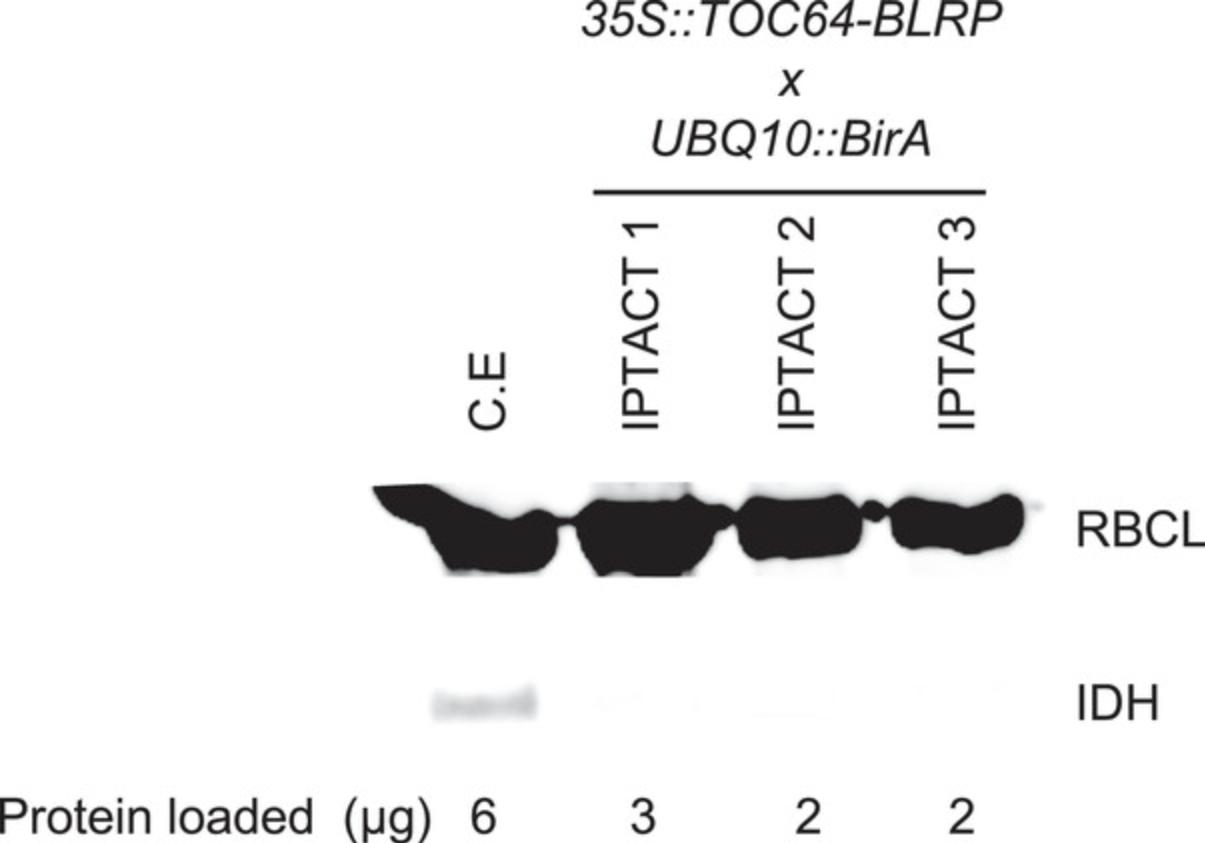
22.Resuspend beads in 800 µl HMS buffer by manual inversion and apply magnet for 1 min.
23.Discard supernatant and repeat step 22 four times.
24.Gently resuspend beads in 80 µl HMS buffer and store on ice (or freeze at −80°C) until further use (see Basic Protocol 3).
Basic Protocol 3: QUALITY CONTROL OF ISOLATED PLASTIDS
After obtaining plastids bound to beads (Basic Protocol 2), it is necessary to assess the quality of the extraction. It is highly probable that the user will obtain tissue-specific plastids at this stage, but purity and integrity need to be determined. Therefore, using one IPTACT extract, a set of experiments is proposed in this protocol. First, protein concentration is estimated, as there is a linear correlation between the number of plastids isolated and the protein content. Then, IPTACT samples are checked on immunoblots using antibodies directed against (i) the plastid's envelope and stroma and (ii) other cell compartments. This protocol defines the purity level of the samples. Finally, the integrity of the samples is assessed by observation under a confocal microscope.
Materials
-
Isolated plastids coupled to beads (see Basic Protocol 2)
-
Hypotonic buffer (see recipe), for plastid bursting (Bag, Schroder, Jansson, & Farci, 2021)
-
Bradford reagent (Bio-Rad, cat. no. 5000006)
-
2× Laemmli buffer (supplemented with 200 mM DTT; see recipe)
-
Crude extract (see Basic Protocol 2, step 12)
-
Antibodies raised against plastids, mitochondria, and nucleus:
- Anti-plastid stroma antibody (e.g., α-RBCL, Agrisera, cat. no. AS03 037)
- Anti-plastid envelope antibody (e.g., α-TOC34, Agrisera, cat. no. AS07 235)
- Anti-mitochondrial matrix (e.g., α-IDH, Agrisera, cat. no. AS06 203A)
- Anti-nucleus (e.g., α-HIS3, Agrisera, cat. no. AS10 710)
-
Antibodies raised against other cell compartments (optional but recommended):
- Anti-endoplasmic reticulum (e.g., α-SEC12; Zheng et al., 2002)
- Anti-peroxisomes (e.g., α-CAT, Agrisera, cat. no. AS09 501)
- Anti-cytosol (e.g., α-UGPase, Agrisera, cat. no. AS05 086)
- Anti-vacuoles (e.g., α-ɣTIP; Rojo, Zouhar, Kovaleva, Hong, & Raikhel, 2003)
-
Vortex
-
Magnet (Miltenyi Biotec, MiniMACS Separator)
-
95°C heating block
-
Confocal laser microscope (e.g., LSM780, Zeiss)
-
ImageJ software (https://imagej.nih.gov/ij/)
-
Additional reagents and equipment for Bradford assay
Protein quantity assessment
1.Aliquot 5 µl isolated plastids coupled to beads and add 4 volumes of hypotonic buffer.
2.Vortex and let sit for 20 min at room temperature.
3.Perform a quick spin, separate beads with a magnet for 2 min, and gently collect supernatant.
4.Use supernatant and Bradford reagent to perform a Bradford assay.
Purity assessment
5.Aliquot 1 to 3 µg proteins and mix with 1 volume of 2× Laemmli buffer (supplemented with 200 mM DTT).
6.Incubate at 95°C for 15 min in a heating block and store on ice until further use.
7.Perform a western blot on the IPTACT samples and an aliquot of the crude extract using antibodies raised against plastids, mitochondria, and nucleus and, optionally, antibodies raised against other cell compartments.
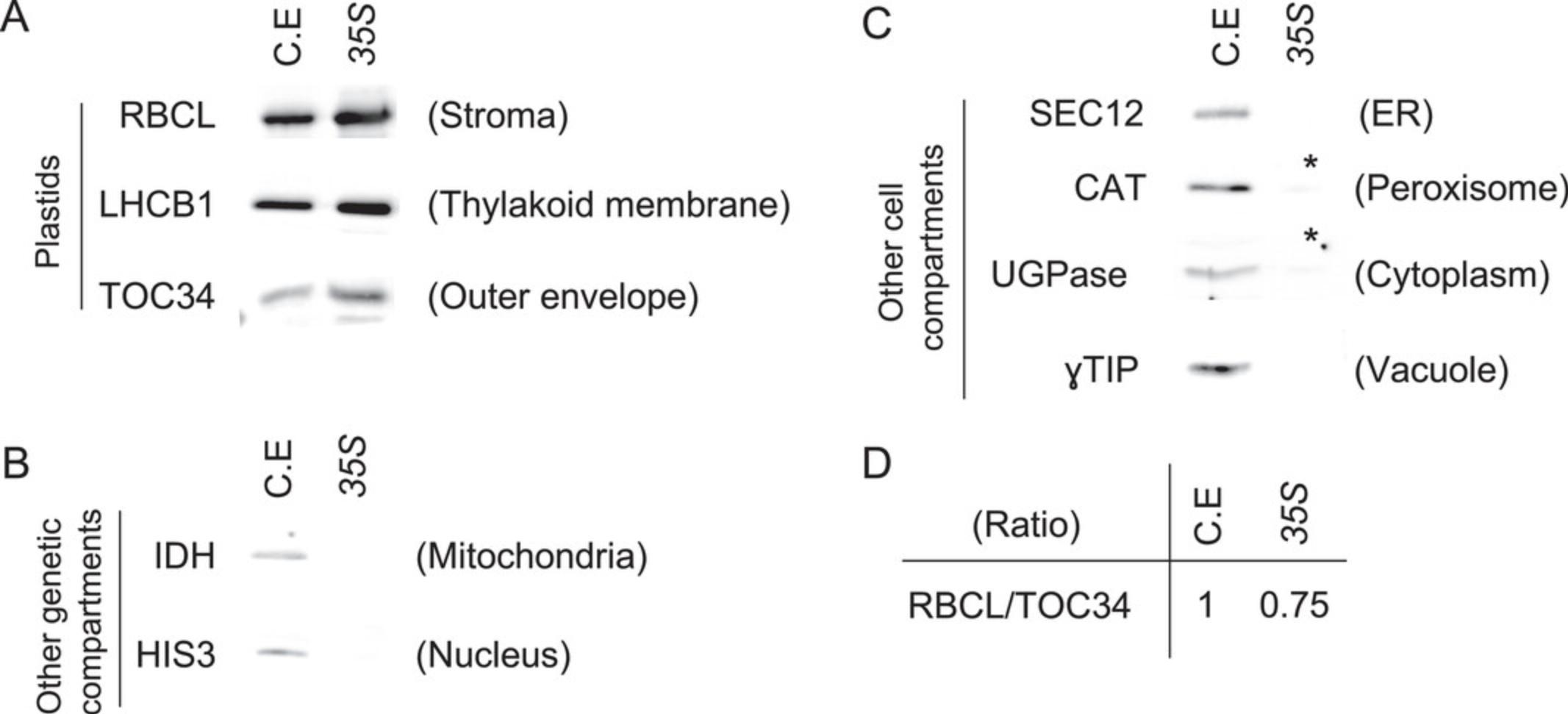
Microscopy integrity assessment
8.Aliquot 1 µl for confocal microscopy of IPTACT samples.
9.Under a confocal microscope, detect signals according to the following excitation/emission wavelengths: eGFP (488 nm/495 to 535 nm) and chloroplast autofluorescence (633 nm/660 to 720 nm). Analyze images using ImageJ software.
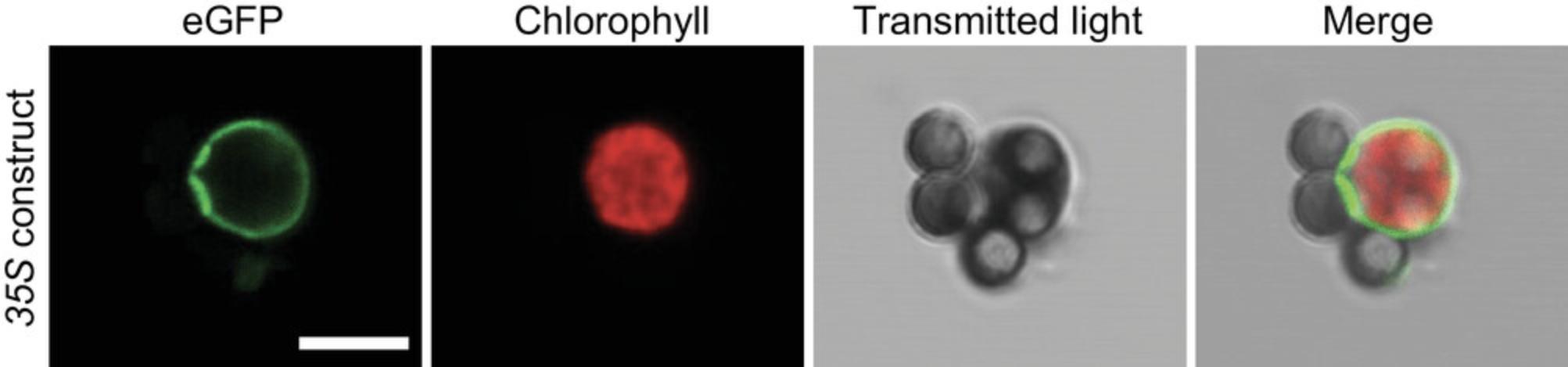
REAGENTS AND SOLUTIONS
HMS buffer (Aronsson & Jarvis, 2002)
Chemical | Final concentration | Weight for 100-ml solution |
D-sorbitol | 0.3 M | 5.465 g |
MgSO4 7*H2O | 0.003 M | 0.074 g |
HEPES | 0.05 M | 1.192 g |
Adjust pH with KOH to 7.6. Store at 4°C for up to 1 month; pH should be checked before isolation. Better to use fresh.
Hypotonic buffer (Bag et al., 2021)
Chemical | Final concentration | Volume/weight for 10-ml solution |
0.5M HEPES-KOH pH7.5 | 0.05 M | 5 ml |
MgCl2 6*H2O | 0.005 M | 0.0102 g |
NaF | 0.01 M | 0.004 g |
Store ≤1 month at room temperature.
CAUTION: NaF (CAS no. 7681-49-4) must be added under a fume hood. It must be manipulated with care as it causes irritation (Category 2, H315, H319) and acute toxicity (Category 3, H301).
Isolation buffer (Aronsson & Jarvis, 2002)
Chemical | Final concentration | Weight for 100-ml solution |
D-sorbitol | 0.3 M | 5.465 g |
MgCl2 6*H2O | 0.005 M | 0.102 g |
EGTA | 0.005 M | 0.190 g |
EDTA 2*H2O | 0.005 M | 0.186 g |
HEPES | 0.02 M | 0.477 g |
NaHCO3 | 0.01 M | 0.084 g |
Adjust pH with KOH to 8. Prepare fresh immediately before use (preferable) or store ≤1 month at 4°C. Check pH before isolation.
Laemmli buffer, 2×
Chemical | Final concentration | Volume/weight for 20-ml solution |
20% SDS | 4% | 4 ml |
100% glycerol | 20% | 4 ml |
Bromophenol blue | 0.004% | 0.8 mg |
1 M Tris·HCl, pH 6.8 | 0.125 M | 2.5 ml |
Store ≤1 year at −20°C. Immediately before use, add 1/10 volume of 2 M DTT (final concentration: 0.2 M).
Plant transformation medium
Chemical | Final concentration | Volume/weight for 200-ml solution |
Sucrose | 5% | 10 g |
Silwet L-77 | 0.0002% | 40 µl |
Prepare fresh immediately before use.
COMMENTARY
Background Information
Background
Isolation of plastids is essential to accurately study molecular regulation associated with these organelles. To maintain integrity of the envelope, extraction and washing buffers should contain sorbitol (or another osmoticum), a buffer such as HEPES, and several other chemical compounds that maintain membrane integrity and physiological functionalities (e.g., EDTA, EGTA). These buffers are necessary to keep organelles in good condition. For decades, purity of plastid samples was achieved by employing sucrose or Percoll gradients. Usually taking 1 hr, these methods allow the isolation of intact chloroplasts in a quite simple manner, with one- or two-step gradients from 5 to 10 g tissues (Aronsson & Jarvis, 2002; van Wijk, Peltier, & Giacomelli, 2007). However, to achieve tissue specificity, it is necessary to establish alternative methods.
One method is to manually separate the different tissues using razor blades, as shown for mitochondria (Clement, Moison, Soulay, Reisdorf-Cren, & Masclaux-Daubresse, 2018), or to directly select chloroplasts using laser-assisted microdissection (Meimberg et al., 2003). In the past decade, methods relying on genetically encoded tags have emerged. In 2007, Truernit and Hibberd described a method using enhancer trap lines involving chloroplasts from individual cell types labeled by placing a YFP on the outer membrane (Truernit & Hibberd, 2007). A huge step forward was achieved in a publication presenting the INTACT strategy, allowing the isolation of nuclei through biotinylation of the envelope (Deal & Henikoff, 2011). A similar strategy was used to isolate mitochondria from HeLa cells and plant tissues, the latest version being named IMTACT (Boussardon et al., 2020; Chen, Freinkman, Wang, Birsoy, & Sabatini, 2016). Isolation of plastids using the same principle (IPTACT) was the next logical step, as plastids were the last genetic compartment for which the biotinylation method was not adapted (Boussardon et al., 2022). As plastid integrity, activity, purity, and tissue specificity are achieved, a broad range of OMICs or physiological experiments are foreseen.
Advantages and disadvantages
The IPTACT method has the strong advantage of relying on editable plasmids containing a ccdB cassette. By a simple Golden Gate reaction, this negative selection cassette is replaced by any promoter of interest. Thus, this method is only limited by the specificity of the promoter used. The set of editable plasmids has been thought to be user friendly. In fact, the plant selection marker can be hygromycin, kanamycin, or Basta, allowing the expression cassette to be inserted into different genetic backgrounds. As markers are genetically encoded, there is no need to dissect samples, and hence, no mechanical stress is induced prior to homogenization. Furthermore, IPTACT is a fast (∼30 min from tissue collection to the end of isolation), affordable (estimated at 8 USD per reaction), and easy-to-achieve (common lab materials used) procedure.
The main drawback of this method results from its strength: plastids are bound to beads. Biotin and streptavidin have an extraordinarily high affinity, so it is particularly difficult to elute plastids from beads without damaging them. This notably leads to some issues when conducting physiological experiments. Experiments such as gas exchange in liquid phase using a Clark-type O2 electrode rely on continuous stirring of the sample. As stirring is achieved by a magnet, plastids linked to beads are inevitably attracted to the bottom of the chamber and crushed by the revolutions of stirring bar. Additionally, beads greatly influence spectrophotometry experiments, as they have been observed to fluoresce within a broad range of spectra and to distort the result of the Bradford assay at 595 nm.
In addition, although the procedure by itself is fast (∼30 min), plants need to be transformed and propagated at least until the T2 generation. In fact, T1 seeds have a ∼1% chance of being transformed (hemizygous state) and should be maintained carefully for segregation observations. Thus, one should plan ahead for the experiment. Moreover, as the IPTACT construct is inserted in the nuclear genome, it can potentially affect essential genes’ expression. Hence, it is recommended to work with a set of independent lines. Finally, we opted to drive the expression of the biotin ligase (BirA) by the UBQ10 promoter. Even though this promoter is ubiquitous, it might not be expressed in very specific tissues or developmental stages, for example, the first steps of pollen development. In these rare cases, the TOC64-eGFP-BLRP construct would therefore not be biotinylated, and the isolation procedure would not be suitable.
Comparison with other published methods
Here, we aim to benchmark the IPTACT approach relative to other methods for the isolation of plastids. However, we want to acknowledge many of these methods for their intrinsic qualities, and without these techniques, IPTACT would not have been possible. IPTACT is a complementary strategy that should be considered if tissue specificity, purity of the sample, or the amount of starting material is at stake. For instance, gradient protocols usually need more starting material (∼10 g), compared to the <1g required for IPTACT. In other methods, materials from more than one plant are often pooled to achieve gradient chloroplast isolation. From IPTACT, a single plant can provide enough material for one reaction, enabling plant-to-plant comparison. Gradient protocols allow the extraction of a massive amount of plastids (possibly organ specific); however, tissue specificity is hardly achieved.
More similar to IPTACT, enhancer trap lines of Arabidopsis were also used to tag plastids in specific cells (Truernit & Hibberd, 2007). However, the major difference from IPTACT is that the transgene, allowing plastids to be tagged, is randomly inserted in the genome (the endogenous enhancer element giving the cell specificity). Thus, cell type–specific plastid tagging is more controlled in IPTACT than in enhancer trap lines.
Finally, the fluorescence-activated cell sorting (FACS) method is also suitable for the tissue-specific isolation of plastids and was successfully used to compare plastids from mesophyll and epidermal cells (Beltran et al., 2018). However, one needs to purchase a cell sorter and calibrate it for chloroplast isolation. Additionally, if targeting plastids and not chloroplasts, an insert with a fluorescent protein, or at least use of a fluorescent marker, would be necessary. We believe that if the FACS method is not established in the user's lab, the IPTACT method is more user friendly, less costly, and less time consuming.
Other approaches considered
The method presented here resulted from testing a few alternatives, and here, we will present a few arguments supporting our choice of the method detailed above. The 2.8-µm beads (Basic Protocol 2) appear to be the best choice for plastid isolation. Plastid capture might be limited to the surface of the beads, so adjusting the bead size to the average plastid's size (4 µm) could lead to improved yields and purity. Conversely, plastids isolated with 1-µm beads are contaminated with other cell compartments. With that being said, we did not manage to isolate plastids using 4-µm beads. Additionally, it was shown that mitochondria with an HA tag could be extracted with anti-HA magnetic beads or with a Twin-Strep tag could be extracted with Strep-Tactin beads (Kuhnert et al., 2020; Niehaus et al., 2020). Although not exempted of drawbacks, we opted for the biotin streptavidin interaction as we wanted to maximize the plastids/beads interaction.
For the buffers (isolation and HMS buffers; Basic Protocol 2), the method published in Aronsson & Jarvis (2002) is used. Another set of buffers was also tested with success; however, it gave slightly more contaminants in our hands (van Wijk et al., 2007; isolation: 0.33 M D-sorbitol, 2 mM EDTA, 0.05 M HEPES, 0.05% BSA, 5 mM ascorbic acid, and 5 mM cysteine in KOH, pH 8; wash: 0.05 M HEPES, 2 mM EDTA, and 0.33 M D-sorbitol in KOH, pH 8). As proposed in some methods (Binder, Jagendorf, & Ngo, 1978; Shimaoka, Yokota, & Miyake, 2000), we added different concentrations of sodium (NaCl), though with no apparent gain.
The equipment used for homogenization (Basic Protocol 2) was carefully selected. A polytron (13-mm-diameter rotor) is preferred to a blender, as it results in a higher proportion of intact plastids. Use of a 50-ml plastic beaker is also important, as increasing the beaker volume leads to an increased number of broken plastids.
Finally, we previously repeatedly obtained peroxisome contamination. To circumvent this issue, we increased the number of pulses (from 3 to 4 pulses of 2 s) and worked under green light. A study showed that peroxisomes strongly bind to plastids within seconds under blue and red light (Oikawa et al., 2015). In contrast, peroxisomes have limited contact area with plastids under darkness and green light, thereby reducing interaction. Adding these steps leads to more robust results and increased purity of the sample.
Critical Parameters
The success of this approach relies mainly on the expression of the TOC64-eGFP-BLRP construct. Thus, the choice of the promoter in Basic Protocol 1 is critical to properly select plastids in the right tissue or at the right developmental stage. The promoter should be carefully selected based on the literature, expression data, and, if possible, complementary in vivo localization. Additionally, it has been observed that lines containing a constitutive promoter could exhibit silencing, especially at the homozygous stage. We strongly recommend checking the eGFP signal using confocal microscopy. Establishment of the IPTACT lines (Basic Protocol 1) is the foundation for successful plastid isolation (Basic Protocol 2).
The reliability and reproducibility of IPTACT also depend on the user's organization. All material must be prepared in advance (e.g., pre-cooling, placing the funnel on the beaker, preparing tubes), which leads to a considerable gain of time as well as an improved yield in Basic Protocol 2.
Although slightly counterintuitive, it is important to use <1 g plant material for the experiment in Basic Protocol 2, step 5. Starting with too much plant material results in a very dense crude extract, which in turn increases the risk of contaminants being trapped between the 2.8-µm beads during the isolation procedure. A more fluid extract is recommended.
After the second centrifugation, the pellet will subsequently be in contact with beads. It is necessary that this pellet is well resuspended (see Basic Protocol 2, step 12). If sticky clumps remain, these will stick to the samples even after five washes, as they will be physically attracted to the side of the tube when the magnet is applied. As there is a huge amount of plastids in the crude extract, the user is encouraged to maximize the resuspension step, even though it may turn out to be at the expense of plastid intactness.
Finally, the incubation with magnetic beads must be gentle from the crude extract step to the last resuspension (see Basic Protocol 2, steps 19 to 24). Here, the strong streptavidin-biotin interaction is an advantage, as it happens within seconds in the sample. Thus, although manufacturers’ recommendations for incubation time are between 15 and 60 min, we observed that 1 min of incubation is sufficient for bead-plastid association. As plastids are relatively fragile structures, gentle hand mixing with low air space (<400 µl) in the tube is important to avoid damaging the samples.
Troubleshooting
Table 1 lists common problems when performing IPTACT and possible causes and solutions.
Problem | Possible cause | Solution |
---|---|---|
Cannot propagate editable plasmids | CCDB protein is a DNA gyrase poison causing cell death | Use DB3.1 ccdB-tolerant competent cells |
Golden Gate reaction does not work | Degradation of the overhangs | Use fresh PCR products and store samples at 4°C (short term) |
BsaI inactivated | BsaI is prone to deactivation if repeatedly frozen and thawed, so aliquot BsaI and use it fresh | |
Impossible to detect eGFP signal | Gene silencing, more commonly observed for homozygous constitutive promoters | Work with hemizygous lines at the T2 generation (segregation) and screen 5-10 independent lines |
High contamination | Peroxisomes interact with plastids | Perform experiment under green light |
Tissues are not ground well | Increase number of pulses and check integrity of the Miracloth | |
Crude extract too dense | Use ≤1 g plant material, as too-concentrated samples lead to clumps and trapped contaminants among beads | |
Crude extract not resuspended well enough | Fully resuspend pellet using a brush and, if necessary, let crude extract settle for 1 min before collecting the supernatant | |
Not enough washing steps | Add one or two more washing steps | |
Low concentration | Starch crushes plastids while homogenizing samples | Put plants in the dark the day before the experiment |
Too-harsh homogenization; overheating of the samples | Limit to 2-s pulses; work in a cold room or with samples on ice | |
Pellet resuspension too harsh | Pellet should be gently resuspended using a clean brush, and a gentle circular movement should be used | |
Incubation with beads too long | Plastids are quite fragile, so gently incubate beads with the crude extract for 1 min | |
Mechanical degradation of plastids | Try to avoid resuspension by pipetting and use 1-ml cut tips only if necessary |
Understanding Results
IPTACT applied to 1g leaves, and 30 µl beads should yield up to 40 µg proteins, depending on the promoter used. Around 70% to 80% intact plastids should be obtained when comparing plastid stroma and envelope immunoblots. In addition, extraction from leaves containing a 35S -driven insert yielded to 2 µg RNA. The user can consider those values as standards, but the yield can be improved by increasing the number of beads or pooling samples. For controls, the immunoblot profile should be nearly immaculate compared to the crude extract.
Time Considerations
Considering that a new promoter must be cloned and plants transformed, the procedure detailed in Basic Protocol 1 should take 5 to 6 months. However, as we already generated several constitutive, tissue-specific T3 lines, the experimental time could potentially be much shorter. In another research group, generation of the first lines would also take some time, but subsequent iterations of the method might become less consuming because several constructs, stable lines, and so forth would become available.
Note that the experimental time also depends on the genetic background and the developmental stages that need to be considered for a given experiment.
The day prior to isolation, plants should be put in the dark. The plastid isolation procedure (Basic Protocol 2) described here can be completed in ∼30 min from homogenizing to isolation. Without rushing it, the protocol should be done as quickly as possible to prevent plastid degradation.
Some quality control should be performed on freshly extracted plastids (Basic Protocol 3). Plastid integrity should be assessed (at least with microscopy) on the day of the extraction. Protein quantification and immunoblot controls could be postponed to the next day or week providing that samples are aliquoted upon isolation. However, this could be achieved in a couple of days.
Acknowledgments
This work was financially supported by the KVA (BS2021-0001 to CB), the T4F program, and the SSF (FFF20-0008). UPSC is supported by grants from the Knut and Alice Wallenberg Foundation and the Swedish Governmental Agency for Innovation Systems (VINNOVA).
Apologies to our colleagues whose work could not be cited in the introduction due to space limitations.
Author Contributions
Clément Boussardon : Methodology, writing and editing; Olivier Keech : Supervision, writing and editing.
Conflict of Interest
The authors declare no conflict of interest.
Open Research
Data Availability Statement
The data, tools, and material (or their source) that support the protocols are available from the corresponding author upon reasonable request.
Literature Cited
- Aronsson, H., & Jarvis, P. (2002). A simple method for isolating import-competent Arabidopsis chloroplasts. FEBS Letters , 529(2-3), 215–220. doi: 10.1016/s0014-5793(02)03342-2
- Bag, P., Schroder, W. P., Jansson, S., & Farci, D. (2021). Solubilization method for isolation of photosynthetic mega- and super-complexes from conifer thylakoids. Bio-Protocol , 11(17), e4144. doi: 10.21769/BioProtoc.4144
- Balmer, Y., Vensel, W. H., DuPont, F. M., Buchanan, B. B., & Hurkman, W. J. (2006). Proteome of amyloplasts isolated from developing wheat endosperm presents evidence of broad metabolic capability. Journal of Experimental Botany , 57(7), 1591–1602. doi: 10.1093/jxb/erj156
- Barsan, C., Kuntz, M., & Pech, J. C. (2017). Isolation of chromoplasts and suborganellar compartments from tomato and bell pepper fruit. Methods in Molecular Biology , 1511, 61–71. doi: 10.1007/978-1-4939-6533-5_5
- Bayer, R. G., Stael, S., Csaszar, E., & Teige, M. (2011). Mining the soluble chloroplast proteome by affinity chromatography. Proteomics , 11(7), 1287–1299. doi: 10.1002/pmic.201000495
- Beltran, J., Wamboldt, Y., Sanchez, R., LaBrant, E. W., Kundariya, H., Virdi, K. S., … Mackenzie, S. A. (2018). Specialized plastids trigger tissue-specific signaling for systemic stress response in plants. Plant Physiology , 178(2), 672–683. doi: 10.1104/pp.18.00804
- Binder, A., Jagendorf, A., & Ngo, E. (1978). Isolation and composition of the subunits of spinach chloroplast coupling factor protein. Journal of Biological Chemistry , 253(9), 3094–3100.
- Boussardon, C., Carrie, C., & Keech, O. (in press). Comparing plastid proteomes points towards a higher plastidial redox turnover in vascular tissues than in mesophyll cells. Journal of Experimental Botany.
- Boussardon, C., & Keech, O. (2022). Cell type-specific isolation of mitochondria in Arabidopsis. Methods in Molecular Biology , 2363, 13–23. doi: 10.1007/978-1-0716-1653-6_2
- Boussardon, C., Przybyla-Toscano, J., Carrie, C., & Keech, O. (2020). Tissue-specific isolation of Arabidopsis/plant mitochondria - IMTACT (isolation of mitochondria tagged in specific cell types). Plant Journal , 103(1), 459–473. doi: 10.1111/tpj.14723
- Cayla, T., Batailler, B., Le Hir, R., Revers, F., Anstead, J. A., Thompson, G. A., … Dinant, S. (2015). Live imaging of companion cells and sieve elements in Arabidopsis leaves. PLOS ONE , 10(2), e0118122. doi: 10.1371/journal.pone.0118122
- Chen, W. W., Freinkman, E., Wang, T., Birsoy, K., & Sabatini, D. M. (2016). Absolute quantification of matrix metabolites reveals the dynamics of mitochondrial metabolism. Cell , 166(5), 1324–1337.e1311. doi: 10.1016/j.cell.2016.07.040
- Chen, Y. E., Yuan, S., & Schroder, W. P. (2016). Comparison of methods for extracting thylakoid membranes of Arabidopsis plants. Physiologia Plantarum , 156(1), 3–12. doi: 10.1111/ppl.12384
- Chiasson, D., Giménez-Oya, V., , Bircheneder, M., Bachmaier, S., Studtrucker, T., Ryan, J., & Parniske, M. (2019). A unified multi-kingdom Golden Gate cloning platform. Scientific Reports , 9, 10131. doi: 10.1038/s41598-019-46171-2
- Clement, G., Moison, M., Soulay, F., Reisdorf-Cren, M., & Masclaux-Daubresse, C. (2018). Metabolomics of laminae and midvein during leaf senescence and source-sink metabolite management in Brassica napus L. leaves. Journal of Experimental Botany , 69(4), 891–903. doi: 10.1093/jxb/erx253
- Clough, S. J., & Bent, A. F. (1998). Floral dip: A simplified method for Agrobacterium-mediated transformation of Arabidopsis thaliana. Plant Journal , 16(6), 735–743. doi: 10.1046/j.1365-313-1998.00343.x
- Deal, R. B., & Henikoff, S. (2011). The INTACT method for cell type-specific gene expression and chromatin profiling in Arabidopsis thaliana. Nature Protocols , 6(1), 56–68. doi: 10.1038/nprot.2010.175
- Emes, M. J., & England, S. (1986). Purification of plastids from higher-plant roots. Planta , 168(2), 161–166. doi: 10.1007/BF00402959
- Ferro, M., Salvi, D., Riviere-Rolland, H., Vermat, T., Seigneurin-Berny, D., Grunwald, D., … Rolland, N. (2002). Integral membrane proteins of the chloroplast envelope: Identification and subcellular localization of new transporters. Proceedings of the National Academy of Sciences of the United States of America , 99(17), 11487–11492. doi: 10.1073/pnas.172390399
- Froehlich, J. E., Wilkerson, C. G., Ray, W. K., McAndrew, R. S., Osteryoung, K. W., Gage, D. A., & Phinney, B. S. (2003). Proteomic study of the Arabidopsis thaliana chloroplastic envelope membrane utilizing alternatives to traditional two-dimensional electrophoresis. Journal of Proteome Research , 2(4), 413–425. doi: 10.1021/pr034025j
- Grabsztunowicz, M., Rokka, A., Farooq, I., Aro, E. M., & Mulo, P. (2020). Gel-based proteomic map of Arabidopsis thaliana root plastids and mitochondria. BMC Plant Biology , 20(1), 413. doi: 10.1186/s12870-020-02635-6
- Hall, M., Mishra, Y., & Schroder, W. P. (2011). Preparation of stroma, thylakoid membrane, and lumen fractions from Arabidopsis thaliana chloroplasts for proteomic analysis. Methods in Molecular Biology , 775, 207–222. doi: 10.1007/978-1-61779-237-3_11
- Hibberd, J. M., & Quick, W. P. (2002). Characteristics of C4 photosynthesis in stems and petioles of C3 flowering plants. Nature , 415(6870), 451–454. doi: 10.1038/415451a
- Hooper, C. M., Castleden, I. R., Tanz, S. K., Aryamanesh, N., & Millar, A. H. (2017). SUBA4: The interactive data analysis centre for Arabidopsis subcellular protein locations. Nucleic Acids Research , 45(D1), D1064–D1074. doi: 10.1093/nar/gkw1041
- Huang, M., Friso, G., Nishimura, K., Qu, X., Olinares, P. D., Majeran, W., … van Wijk, K. J. (2013). Construction of plastid reference proteomes for maize and Arabidopsis and evaluation of their orthologous relationships; the concept of orthoproteomics. Journal of Proteome Research , 12(1), 491–504. doi: 10.1021/pr300952g
- Islam, M. S., Buttelmann, G. L., Chekhovskiy, K., Kwon, T., & Saha, M. C. (2019). Isolation of intact chloroplast for sequencing plastid genomes of five festuca species. Plants-Basel , 8(12), 606. doi: 10.3390/plants8120606
- Jacobson, A. B. (1968). A procedure for isolation of proplastids from etiolated maize leaves. Journal of Cell Biology , 38(1), 238–&. doi: 10.1083/jcb.38.1.238
- Kleffmann, T., Russenberger, D., von Zychlinski, A., Christopher, W., Sjolander, K., Gruissem, W., & Baginsky, S. (2004). The Arabidopsis thaliana chloroplast proteome reveals pathway abundance and novel protein functions. Current Biology , 14(5), 354–362. doi: 10.1016/j.cub.2004.02.039
- Kuhnert, F., Stefanski, A., Overbeck, N., Drews, L., Reichert, A. S., Stuhler, K., & Weber, A. P. M. (2020). Rapid single-step affinity purification of HA-tagged plant mitochondria. Plant Physiology , 182(2), 692–706. doi: 10.1104/pp.19.00732
- Machettira, A. B., Gross, L. E., Tillmann, B., Weis, B. L., Englich, G., Sommer, M. S., … Schleiff, E. (2011). Protein-induced modulation of chloroplast membrane morphology. Frontiers in Plant Science , 2, 118. doi: 10.3389/fpls.2011.00118
- Majeran, W., Cai, Y., Sun, Q., & van Wijk, K. J. (2005). Functional differentiation of bundle sheath and mesophyll maize chloroplasts determined by comparative proteomics. Plant Cell , 17(11), 3111–3140. doi: 10.1105/tpc.105.035519
- Meimberg, H., Thalhammer, S., Brachmann, A., Muller, B., Eichacker, L. A., Heckl, W. M., & Heubl, G. (2003). Selection of chloroplasts by laser microbeam microdissection for single-chloroplast PCR. Biotechniques , 34(6), 1238–1243. doi: 10.2144/03346rr01
- Miflin, B. J., & Beevers, H. (1974). Isolation of intact plastids from a range of plant-tissues. Plant Physiology , 53(6), 870–874. doi: 10.1104/pp.53.6.870
- Niehaus, M., Straube, H., Kunzler, P., Rugen, N., Hegermann, J., Giavalisco, P., … Herde, M. (2020). Rapid affinity purification of tagged plant mitochondria (Mito-AP) for metabolome and proteome analyses. Plant Physiology , 182(3), 1194–1210. doi: 10.1104/pp.19.00736
- Oikawa, K., Matsunaga, S., Mano, S., Kondo, M., Yamada, K., Hayashi, M., … Nishimura, M. (2015). Physical interaction between peroxisomes and chloroplasts elucidated by in situ laser analysis. Nature Plants , 1(4), 15035. doi: 10.1038/nplants.2015.35
- Peltier, J. B., Ytterberg, A. J., Sun, Q., & van Wijk, K. J. (2004). New functions of the thylakoid membrane proteome of Arabidopsis thaliana revealed by a simple, fast, and versatile fractionation strategy. Journal of Biological Chemistry , 279(47), 49367–49383. doi: 10.1074/jbc.M406763200
- Pinard, D., & Mizrachi, E. (2018). Unsung and understudied: Plastids involved in secondary growth. Current Opinion in Plant Biology , 42, 30–36. doi: 10.1016/j.pbi.2018.01.011
- Rojo, E., Zouhar, J., Kovaleva, V., Hong, S., & Raikhel, N. V. (2003). The AtC-VPS protein complex is localized to the tonoplast and the prevacuolar compartment in Arabidopsis. Molecular Biology of the Cell , 14(2), 361–369. doi: 10.1091/mbc.e02-08-0509
- Seigneurin-Berny, D., Salvi, D., Joyard, J., & Rolland, N. (2008). Purification of intact chloroplasts from Arabidopsis and spinach leaves by isopycnic centrifugation. Current Protocols in Cell Biology , 40(1), 3.30.1–3.30.14. doi: 10.1002/0471143030.cb0330s40
- Shimaoka, T., Yokota, A., & Miyake, C. (2000). Purification and characterization of chloroplast dehydroascorbate reductase from spinach leaves. Plant & Cell Physiology, 41(10), 1110–1118. doi: 10.1093/pcp/pcd035
- Simm, S., Papasotiriou, D. G., Ibrahim, M., Leisegang, M. S., Muller, B., Schorge, T., … Schleiff, E. (2013). Defining the core proteome of the chloroplast envelope membranes. Frontiers in Plant Science , 4, 11. doi: 10.3389/fpls.2013.00011
- Suzuki, M., Takahashi, S., Kondo, T., Dohra, H., Ito, Y., Kiriiwa, Y., … Motohashi, R. (2015). Plastid proteomic analysis in tomato fruit development. PLOS ONE , 10(9), e0137266. doi: 10.1371/journal.pone.0137266
- Tamburino, R., Vitale, M., Ruggiero, A., Sassi, M., Sannino, L., Arena, S., … Scotti, N. (2017). Chloroplast proteome response to drought stress and recovery in tomato (Solanum lycopersicum L.). BMC Plant Biology , 17(1), 40. doi: 10.1186/s12870-017-0971-0
- Truernit, E., & Hibberd, J. M. (2007). Immunogenic tagging of chloroplasts allows their isolation from defined cell types. Plant Journal , 50(5), 926–932. doi: 10.1111/j.1365-313X.2007.03113.x
- van Wijk, K. J., Peltier, J. B., & Giacomelli, L. (2007). Isolation of chloroplast proteins from Arabidopsis thaliana for proteome analysis. Methods in Molecular Biology , 355, 43–48. doi: 10.1385/1-59745-227-0:43
- von Zychlinski, A., Kleffmann, T., Krishnamurthy, N., Sjolander, K., Baginsky, S., & Gruissem, W. (2005). Proteome analysis of the rice etioplast: Metabolic and regulatory networks and novel protein functions. Molecular & Cellular Proteomics, 4(8), 1072–1084. doi: 10.1074/mcp.M500018-MCP200
- Zheng, H., Bednarek, S. Y., Sanderfoot, A. A., Alonso, J., Ecker, J. R., & Raikhel, N. V. (2002). NPSN11 is a cell plate-associated SNARE protein that interacts with the syntaxin KNOLLE. Plant Physiology , 129(2), 530–539. doi: 10.1104/pp.003970
Citing Literature
Number of times cited according to CrossRef: 1
- Clément Boussardon, Chris Carrie, Olivier Keech, Comparison of plastid proteomes points towards a higher plastidial redox turnover in vascular tissues than in mesophyll cells, Journal of Experimental Botany, 10.1093/jxb/erad133, (2023).