The Isolation of Stress Granules From Plant Material
Monika Kosmacz, Monika Kosmacz, Aleksandra Skirycz, Aleksandra Skirycz
affinity purification
Arabidopsis thaliana
metabolome
plant
proteome
stress granules
stress response
Abstract
Stress granules (SGs) are ubiquitous nonmembrane-bound assemblies of protein and mRNA formed under stress conditions associated with stalled translation. SGs are evolutionarily conserved across eukaryotes. The canonical function of SGs is to selectively protect mRNAs and proteins from unfolding and prevent degradation induced by diverse environmental stresses. Moreover, sequestration into SGs provides an elegant way to regulate protein activities. Disassembly of SGs upon stress recovery is accompanied by the reactivation of protein translation and protein activities. The regulatory importance of SGs has been corroborated by recent studies describing the multiomics analysis of the composition of SGs from yeast, animal, and plant cells. Herein, we describe an isolation protocol of SGs that allows for the identification of proteins, mRNA, and metabolites sequestered into SG cores. Furthermore, the described protocols can be used to isolate other SG-like foci. © 2020 The Authors.
Basic Protocol 1 : Preparation of SG-enriched fraction from plant material
Basic Protocol 2 : Affinity purification to isolate SGs
Basic Protocol 3 : Simultaneous extraction of proteins and metabolites from affinity-purified beads
Basic Protocol 4 : Protein digestion on affinity-purified beads
Basic Protocol 5 : Data analysis
INTRODUCTION
In contrast to animals, plants are sessile organisms that need to use different adaptation strategies to survive unfavorable and often stressful environmental conditions. The formation of stress granules (SGs; nonmembranous cytosolic foci) is one of the mechanisms that help plants to survive stress by providing a way to protect mRNA from degradation (Merret et al., 2013) and, therefore, subsequently moderate the translatome (Khong et al., 2017; Nover, Scharf, & Neumann, 1989; Yan, Yan, Wang, Yan, & Han, 2014). SGs have been shown to also sequester proteins (Takahara & Maeda, 2012) and metabolites (Kosmacz et al., 2019). Recently, the composition of SGs was elucidated in animal and yeast cells (Jain et al., 2016; Khong et al., 2017; Wheeler, Jain, Khong, & Parker, 2017), revealing the complexity of the proteome and translatome of SGs. Many authors have provided evidence that the formation of SGs is a very dynamic process and that the composition of the granules is very complex (Jain et al., 2016; Wheeler, Matheny, Jain, Abrisch, & Parker, 2016). It has been reported, especially for mammalian SGs, that SGs contain a more fluid shell (Jain et al., 2016) belonging to liquid–liquid phase separation complexes, in addition to the stable core. Interestingly, in addition to high dynamics, the formation of SGs in yeast has been described as a two-step process (Cabrera et al., 2020), starting from core nucleation at the protein aggregation center (which may have already started under mild stress) and proceeding to shell assembly. Among the conserved components of SGs, RNA-binding proteins, ATPases, chaperones, and translation initiation factors have been identified (Jain et al., 2016). Although the existence of SGs in plants was first reported in heat-treated Solanum lycopersicum (Nover et al., 1989) in 1989, the proteome and metabolome composition of plant SGs was only recently reported in 2019 (Kosmacz et al., 2019). The presented results indicate the similarities between the structure of plant SGs and mammalian SGs, suggesting SGs in plants are also composed of a stable core and fluid shell. In terms of protein composition, in addition to conserved proteins, SGs contain plant-specific proteins that are involved in plant growth, metabolism, or hormonal signaling. The basic procedure of SG extraction can be divided into a few steps: (1) stress-treated and control material harvesting, (2) execution of differential centrifugation, (3) affinity purification, (4) extraction of molecules, and (5) mass spectrometry analysis (Fig. 1).
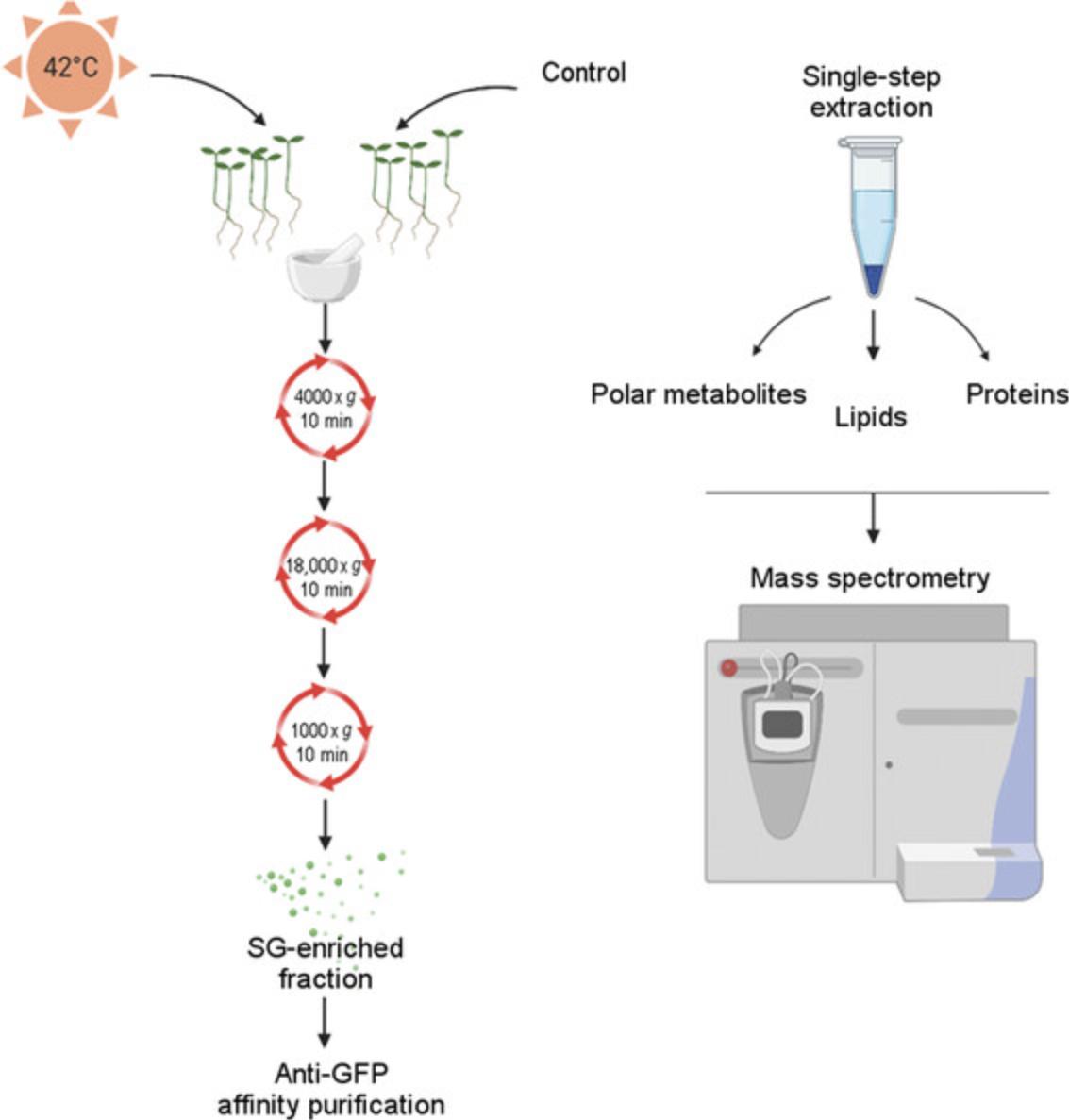
Basic Protocol 1: PREPARATION OF SG-ENRICHED FRACTION FROM PLANT MATERIAL
To extract SGs from plant cells, the formation of SGs must be induced first. In our case, plants expressing a green fluorescent protein (GFP)-tagged stress granule marker (e.g., Rbp47b) treated with heat stress (at 42°C for 30 min) are used as the starting material. The extraction of intact SGs requires a procedure that will not interfere with their integrity. The first step in the extraction of SGs is the preparation of an SG-enriched fraction, which is then used as the starting input for the affinity purification protocol (Basic Protocol 2). Frozen plant material, after mechanical grinding and the addition of a lysis buffer, is then homogenized and prepared for differential centrifugation. The first step of centrifugation separates the supernatant from the pellet of cellular debris, membranes, and larger aggregates, which are kept for the next steps of fast centrifugation. Then, a slow centrifugation step is used to release SGs from the pellet.
For further analysis of the components of SGs, it is essential to include specific controls. For example, the use of plants expressing a tag only (35S:GFP) or expressing a different protein that is known to not be localized into SGs (forms different kinds of aggregates) might help to exclude unspecific binders (false positives).
Materials
-
Frozen plant material
-
Lysis buffer (see recipe)
-
Acetone
-
Grinding mill (e.g., Retsch, cat. no. 207450001)
-
5-mm stainless steel grinding balls (e.g., Retsch, cat. no. 05.368.0034)
-
Liquid nitrogen
-
Mortar and pestle
-
15-ml conical tube
-
Refrigerated centrifuge (e.g., Multifuge #1S-R with Sorvall rotor)
-
Vortex (e.g., Scientific Industries, cat. no. SI-T236)
-
2-ml microcentrifuge tube
-
Refrigerated microcentrifuge
-
Vacuum concentrator (e.g., SpeedVac)
1.Grind frozen plant material using a grinding mill and 5-mm stainless steel grinding balls at 30 Hz for 1 min. Repeat this step two times if needed. Cool samples in liquid nitrogen between grinding steps to avoid thawing.
2.To 2 g sample, add 2 ml lysis buffer (multiply by three to have three replicates), and homogenize using mortar and pestle.
3.Transfer to 15-ml conical tube, and centrifuge 10 min at 4000 × g , 4°C.
4.Remove supernatant and dilute pellets of each sample with 1 ml lysis buffer by pipetting up and down a few times. Vortex for a few seconds.
5.Transfer to 2-ml microcentrifuge tube, and centrifuge 10 min at 18,000 × g , 4°C.
6.Repeat step 4.
7.Centrifuge 10 min at 18,000 × g , 4°C, and then repeat step 4.
8.Centrifuge 10 min at 1000 × g , 4°C.
9.Take 50 μl SG-enriched fraction for protein extraction to obtain a reference input for the affinity purification step. Briefly, add 150 μl cold (−20°C) acetone to SG-enriched sample if needed, and then precipitate for 2 hr at −20°C. Centrifuge 10 min at 18,000 × g, 4°C. Dry remaining protein pellets in a vacuum concentrator for about 2 hr, and store at −80°C for up to 6 months.
Microscopic analysis to ensure the presence of SGs in the SG-enriched fraction
Before proceeding to the next purification step, the presence of SGs in the lysate should be observed under a confocal microscope. About 10 µl of the fraction can be transferred to the top of a glass slide to visualize the GFP signal using excitation of a 488-nm laser and emission between 500 and 520 nm.
Basic Protocol 2: AFFINITY PURIFICATION TO ISOLATE SGs
Affinity purification using anti-GFP antibodies allows fishing for a GFP-tagged bait and protein-metabolite-RNA complexes specific for SGs.
Materials
-
Dynabeads Protein A (e.g., Thermo Fisher, cat. no. 10002D)
-
Diethyl pyrocarbonate (DEPC)-treated phosphate-buffered saline (PBS; see recipe)
-
Lysis buffer (see recipe)
-
SG fraction (see Basic Protocol 1)
-
RNasin plus RNase inhibitor (e.g., Promega, cat. no. N2615)
-
Anti-GFP rabbit IgG antibody (e.g., Thermo Fisher Scientific, cat. no. A11122)
-
Tube rotator
-
Magnetic rack
-
Centrifuge
1.Equilibrate 100 μl Dynabeads Protein A per sample with DEPC-treated PBS for 1 hr at room temperature. Rotate beads on tube rotator. For example, for six samples equilibrate 600 μl Dynabeads with 600 μl PBS.
2.Separate beads using the magnet, and wash once with DEPC-treated PBS for 5 min at 4°C.
3.Rinse three times with lysis buffer for 5 min at 4°C.
4.To 370 μl SG fraction, add RNasin (1:100 final dilution) and 30 μl equilibrated Dynabeads.
5.Incubate on tube rotator for 15 min at room temperature.
6.Separate beads using the magnet, and place supernatant in a new tube.
7.Incubate supernatant with 12.5 μl anti-GFP rabbit IgG antibody for 60 min on the tube rotator for 1 hr at room temperature.
8.Remove excess anti-GFP antibody by centrifuging 15 min at 14,000 × g , 4°C.
9.Remove supernatant and resuspend pellet with 500 μl lysis buffer supplemented with 5 μl RNasin.
10.Incubate resuspension with 60 μl equilibrated Dynabeads for 15 min at room temperature on the tube rotator.
11.Wash three times for 5 min with lysis buffer at 4°C.
12.Remove supernatant and proceed to Basic Protocol 3 to extract proteins and metabolites from the beads.
Basic Protocol 3: SIMULTANEOUS EXTRACTION OF PROTEINS AND METABOLITES FROM AFFINITY-PURIFIED BEADS
To extract lipids, polar metabolites, and proteins, we use a single-step extraction protocol where a complex extraction buffer containing solvents with different polarities is used (Giavalisco et al., 2011). In that way, we separate three independent fractions: organic upper phase containing lipids (nonpolar metabolites), lower polar phase containing polar primary and secondary metabolites, and pellet containing protein and starch. For our interest, lipids, secondary metabolites, and proteins are further analyzed using liquid chromatography–mass spectrometry (LC/MS).
Materials
-
Beads with attached SGs (see Basic Protocol 2)
-
Extraction buffer 1 (see recipe)
-
Extraction buffer 2 (see recipe)
-
Vortex mixer
-
Refrigerated shaking incubator
-
Ultrasonic bath (e.g., Bandelin, cat. no. RK 31)
-
Centrifuge
-
1.5-ml microcentrifuge tube
-
Vacuum concentrator (e.g., SpeedVac)
-
Vacuum pump
1.To beads, add 1 ml precooled (−20°C) extraction buffer 1 and vortex vigorously.
2.Incubate for 10 min at 4°C with shaking.
3.Perform a short, 10-min sonication treatment by partially submerging tubes in an ultrasonic bath to ensure metabolite extraction from the beads.
4.Add 0.5 ml extraction buffer 2, and mix well by vortexing.
5.Centrifuge samples 5 min at 18,000 × g , room temperature.
6.Transfer 500 µl upper (organic) phase to a fresh 1.5-ml tube, and dry in a vacuum concentrator overnight.
7.Remove the rest of the organic phase with a vacuum pump together with the interphase.
8.Transfer 500 µl lower (polar) phase to a fresh 1.5-ml tube, and dry using a vacuum concentrator overnight.
9.Remove the rest of the polar phase, and air-dry the remaining protein pellet. Freeze pellets at −80°C for up to 6 months, or continue with protein digestion (Basic Protocol 4).
Basic Protocol 4: PROTEIN DIGESTION ON AFFINITY-PURIFIED BEADS
Before MS analysis of enriched SG proteins can be performed, proteins need to be digested using trypsin and desalted. This protocol requires 2 days of work and is crucial in order to identify enriched proteins specific for SGs.
Materials
-
Beads with attached dried protein pellets (see Basic Protocol 3)
-
Denaturation buffer (see recipe)
-
100 mM dithiothreitol (DTT)
-
300 mM iodoacetamide
-
Trypsin/Lys-C Mix (e.g., Promega, cat. no. V5071)
-
50 mM ammonium bicarbonate, pH 8 (adjust pH using acetic acid)
-
Trifluoroacetic acid (TFA; e.g., Biosolve, cat. no. 202341)
-
100% methanol
-
0.1% (v/v) TFA/80% (v/v) acetonitrile
-
0.1% (v/v) TFA/60% (v/v) acetonitrile
-
37°C incubator
-
Sep-Pak C18 column (e.g., Teknokroma, cat. no. TR-F034000)
-
2-ml microcentrifuge tube
-
Vacuum concentrator (e.g., SpeedVac)
Bead defrosting and trypsin digestion
1.Solubilize beads with attached proteins in 50 μl denaturation buffer.
2.Reduce cysteine residues by adding 5 μl of 100 μM DTT, and incubate for 30 min at room temperature.
3.Perform alkylation of samples by adding 5 μl of 300 mM iodoacetamide, and incubate for 20 min at room temperature in the dark.
4.Digest reduced and alkalized proteins with Trypsin/Lys-C Mix according to the manufacturer's instructions. Add Trypsin/Lys-C Mix to achieve a protein:protease ratio of 25:1.
5.Dilute urea concentration to 4 M with 50 mM ammonium bicarbonate, pH 8, and incubate for 3 to 4 hr at 37°C. For example, for 70 μl sample (with 4 M urea), add 15 μl of 50 mM ammonium bicarbonate.
6.Dilute samples to 1 M urea with 50 mM ammonium bicarbonate, pH 8.For example, for 85 μl sample (with 4 M urea), add 255 μl of 50 mM ammonium bicarbonate. Incubate samples overnight at 37°C.
7.Acidify samples with TFA to a final concentration of 1% (v/v) TFA and pH <3.
8.Store samples at −20°C for up to 6 months, or continue with desalting (step 9).
Peptide desalting
9.Desalt peptides using Sep-Pack C18 columns. To do so, first activate column with 1 ml of 100% methanol. Then start vacuum to allow methanol to flow through the column.
10.Wet column with 1 ml of 0.1% TFA/80% acetonitrile. Start vacuum to allow TFA/acetonitrile to flow through the column.
11.Wash column twice with 1 ml of 0.1% TFA, and use vacuum to allow TFA to flow through the column.
12.Load sample on the prepared column. Start vacuum to allow sample to flow through the column.
13.Wash filter column with 1 ml of 0.1% TFA. Start vacuum and discard flow-through.
14.Put clean 2-ml microcentrifuge tubes under columns, and add 600 µl of 0.1% TFA/60% acetonitrile to each column. Activate vacuum and collect flow-through.
15.Dry peptides on a vacuum concentrator.
16.Store dried, desalted peptides at −20°C for up to 6 months, or use directly for LC/MS analysis.
Basic Protocol 5: DATA ANALYSIS
To define proteins associated with SGs, multiple criteria might be used for data analysis. First, because SGs are present only under heat stress conditions but not under control conditions, proteins should be considered if they are either present in the heat-stressed sample and absent in the control or are significantly enriched at least twice in heat-stressed samples in comparison to the control. Second, (here depends on the hypothesis) exclusion can be performed for proteins that are known to be localized in chloroplasts or mitochondria, as those proteins are unlikely to be localized in cytosolic SGs. Third, comparison should be done of the list of SG proteins with the list of proteins enriched in sample affinity purified for GFP (35S:GFP) to exclude unspecific binders to GFP only. Fourth, depending on the question, comparison can be performed of the SG protein list with conserved SG proteins from yeast and human. Analysis can be performed of different SG-specific protein domains, such as RNA-binding or prion-like domains, and ATPase activity.
Data analysis depends not only on the hypothesis and protein used as bait but also on controls used in the experiment. Think about the negative protein list that might be used in your case to clean the final list of SG proteins from false-positive proteins.
REAGENTS AND BUFFERS
Denaturation buffer
- 50 mM ammonium bicarbonate, pH 8 (e.g., Sigma-Aldrich, cat. no. 9830; adjust pH using acetic acid)
- 2 M thiourea (e.g., Sigma-Aldrich, cat. no. T8656)
- 6 M urea (e.g., Sigma-Aldrich, cat. no. U5128)
- Prepare fresh before use
Weigh urea and thiourea powder in a conical tube, and then dissolve powders by adding ammonium bicarbonate buffer. Mix tube well by moving up and down.
Extraction buffer 1
- 1 vol. methanol, UPLC grade (e.g., BioSolve, cat. no. 136806)
- 3 vol. methyl tert-butyl ether, UPLC grade (e.g., BioSolve, cat. no. 138906)
- 1 vol. water, UPLC grade (e.g., BioSolve, cat. no. 232106)
- Precool at −20°C for 1 hr before use
- Store leftover buffer at 4°C for up to 1 month
Extraction buffer 2
- 1 vol. methanol, UPLC grade (e.g., BioSolve, cat. no. 136806)
- 3 vol. water, UPLC grade (e.g., BioSolve, cat. no. 232106)
- Precool at 4°C for 1 hr before use
- Store leftover buffer at 4°C for up to 1 month
Lysis buffer
- 50 mM Tris⋅HCl, pH 7.4
- 100 mM potassium acetate
- 2 mM magnesium acetate
- 5 mM DTT (e.g., Sigma-Aldrich, cat. no. D0632)
- 1 mM phenylmethylsulfonyl fluoride (e.g., Sigma-Aldrich, cat. no. P7626)
- 0.1 mM sodium orthovanadate (e.g., Sigma-Aldrich, cat. no. S6580)
- 1 mM sodium fluoride (e.g., Sigma-Aldrich, cat. no. S6776)
- 1× protease inhibitor cocktail (e.g., Sigma-Aldrich, cat. no. P9599)
- 1 U/μl RNasin plus RNase inhibitor (Promega, cat. no. N2615)
- Prepare fresh before use
Add all inhibitors immediately before the experiment. Keep it on ice.
PBS, 10×
- 80 g NaCl
- 2.0 g KCl
- 14.4 g Na2HPO4
- 2.4 g KH2PO4
- 800 ml distilled water
- Adjust pH to 7.4 with HCl
- Bring to 1 liter with distilled water
- Store at room temperature for up to 6 months
Before use, prepare a 1× working solution by diluting 10× PBS 1:10 with water.
To prepare DEPC-treated PBS, add 1 ml DEPC (e.g., Sigma-Aldrich, cat. no. D5758) to 1× PBS, and autoclave before use.
COMMENTARY
Background Information
SGs are cytosolic aggregates of proteins, mRNA, and metabolites formed when translation initiation is limited (Buchan & Parker, 2009). SGs were proposed to play a role in neurodegenerative disorders as it was shown that casual disease mutations might increase the formation of SGs or their persistence (Ramaswami, Taylor, & Parker, 2013). In all studied organisms where SGs were described, the formation of SGs was shown to be a very dynamic process and always stress inducible (Kedersha et al., 2005; Protter & Parker, 2016; Youn et al., 2019). Most of the knowledge that we have was collected on human and yeast cells. Therefore, there is still space in the field of SGs in plants. Recent studies showed that SGs in plants are formed under heat (Gutierrez-Beltran, Moschou, Smertenko, & Bozhkov, 2015; Hamada et al., 2018; Kosmacz et al., 2018; Kosmacz et al., 2019), low oxygen (Sorenson & Bailey-Serres, 2014), and salt stress (Frei dit Frey et al., 2010; Yan et al., 2014). Overexpression of the key component of SGs, UBP1, has been reported to improve resistance to high temperatures (Nguyen et al., 2016) suggesting that SGs indeed are very important for response to stress and modulation of stress response. Identification of the components of SGs might lead to understanding the complexity of stress signaling mechanism, which then can lead to improvement of a plant's resistance to different environmental stresses.
Critical Parameters and Troubleshooting
There are many parameters and steps of the protocol that must be well adjusted before performing an experiment to enable success of the protocol.
Preparation of native lysate for extraction of SGs : To avoid protein degradation, it is important to be concerned about the temperature of the whole procedure. Keep samples cold (on ice unless stated), and include protease inhibitors in the lysis buffer. In addition to inhibitors and the proper temperature, it is important to adjust pH and salt concentration in the buffer in order to keep the composition of native complexes untouched.
Amount of starting material : The yield of SGs in the lysate might differ depending on the starting material used to perform the extraction. Factors such as developmental stage of the plant, strength of stress used for induction of SGs, lysis of the cells, or amount of buffer used for extraction can affect the amount of SGs in the final stage. Therefore, it is advisable to follow GFP signal under a microscope at each stage of the protocol when performing it for the first time.
Extraction of proteins and metabolites from beads : As explained, the protocol for metabolite and protein extraction allows the simultaneous extraction of metabolites and proteins from one sample. However, some metabolites cannot be extracted with our method due to their specific properties (e.g., ATP, hormones, sugar phosphates). To extract those molecules from the beads, different extraction methods have to be considered.
Time Considerations
For SG extraction, around 5 hr is required. Time necessary for simultaneous extraction of proteins and metabolites using single-step extraction depends on the number of samples to process. Simultaneous extraction of proteins and metabolites in a single batch of around 10 samples requires around 1.5 to 2 hr. After extraction, samples are dried using a vacuum concentrator (SpeedVac) for several hours or overnight. Dried samples can be stored at −20°C or −80°C for several weeks. Protein digestion and desalting require 2 days of work.
Acknowledgments
The authors thank Prof. Lothar Wilmitzer for his support and advice, Anna Michaelis for measuring metabolite samples, and Dr. Ewelina Sokolowska for measuring proteomic samples. Open access funding enabled and organized by Projekt DEAL.
Author Contributions
Monika Kosmacz : Conceptualization; methodology; validation; visualization; writing-original draft. Aleksandra Skirycz : Conceptualization; formal analysis; project administration; resources; supervision; writing-review & editing.
Literature Cited
- Buchan, J. R., & Parker, R. (2009). Eukaryotic stress granules: The ins and outs of translation. Molecular Cell , 36, 932–941. doi: 10.1016/j.molcel.2009.11.020.
- Cabrera, M., Boronat, S., Marte, L., Vega, M., Perez, P., Ayte, J., & Hidalgo, E. (2020). Chaperone-facilitated aggregation of thermo-sensitive proteins shields them from degradation during heat stress. Cell Reports , 30, 2430–2443. doi: 10.1016/j.celrep.2020.01.077.
- Frei dit Frey, N., Muller, P., Jammes, F., Kizis, D., Leung, J., Perrot-Rechenmann, C., & Bianchi, M. W. (2010). The RNA binding protein Tudor-SN is essential for stress tolerance and stabilizes levels of stress-responsive mRNAs encoding secreted proteins in Arabidopsis. Plant Cell , 22, 1575–1591. doi: 10.1105/tpc.109.070680.
- Giavalisco, P., Li, Y., Matthes, A., Eckhardt, A., Hubberten, H. M., Hesse, H., … Willmitzer, L. (2011). Elemental formula annotation of polar and lipophilic metabolites using 13C, 15N and 34S isotope labelling, in combination with high-resolution mass spectrometry. Plant Journal , 68, 364–376. doi: 10.1111/j.1365-313X.2011.04682.x.
- Gutierrez-Beltran, E., Moschou, P. N., Smertenko, A. P., & Bozhkov, P. V. (2015). Tudor staphylococcal nuclease links formation of stress granules and processing bodies with mRNA catabolism in Arabidopsis. Plant Cell , 27, 926–943. doi: 10.1105/tpc.114.134494.
- Hamada, T., Yako, M., Minegishi, M., Sato, M., Kamei, Y., Yanagawa, Y., … Hara-Nishimura, I. (2018). Stress granule formation is induced by a threshold temperature rather than a temperature difference in Arabidopsis. Journal of Cell Science , 131, jcs216051. doi: 10.1242/jcs.216051.
- Jain, S., Wheeler, J. R., Walters, R. W., Agrawal, A., Barsic, A., & Parker, R. (2016). ATPase-modulated stress granules contain a diverse proteome and substructure. Cell , 164, 487–498. doi: 10.1016/j.cell.2015.12.038.
- Kedersha, N., Stoecklin, G., Ayodele, M., Yacono, P., Lykke-Andersen, J., Fritzler, M. J., … Anderson, P. (2005). Stress granules and processing bodies are dynamically linked sites of mRNP remodeling. Journal of Cell Biology , 169, 871–884. doi: 10.1083/jcb.200502088.
- Khong, A., Matheny, T., Jain, S., Mitchell, S. F., Wheeler, J. R., & Parker, R. (2017). The stress granule transcriptome reveals principles of mRNA accumulation in stress granules. Molecular Cell , 68, 808–820. doi: 10.1016/j.molcel.2017.10.015.
- Kosmacz, M., Gorka, M., Schmidt, S., Luzarowski, M., Moreno, J. C., Szlachetko, J., … Skirycz, A. (2019). Protein and metabolite composition of Arabidopsis stress granules. New Phytologist , 222, 1420–1433. doi: 10.1111/nph.15690.
- Kosmacz, M., Luzarowski, M., Kerber, O., Leniak, E., Gutierrez-Beltran, E., Moreno, J. C., … Skirycz, A. (2018). Interaction of 2′,3′-cAMP with Rbp47b plays a role in stress granule formation. Plant Physiology , 177, 411–421. doi: 10.1104/pp.18.00285.
- Merret, R., Descombin, J., Juan, Y. T., Favory, J. J., Carpentier, M. C., Chaparro, C., … Bousquet-Antonelli, C. (2013). XRN4 and LARP1 are required for a heat-triggered mRNA decay pathway involved in plant acclimation and survival during thermal stress. Cell Reports , 5, 1279–1293. doi: 10.1016/j.celrep.2013.11.019.
- Nguyen, C. C., Nakaminami, K., Matsui, A., Kobayashi, S., Kurihara, Y., Toyooka, K., … Seki, M. (2016). Oligouridylate binding protein 1b plays an integral role in plant heat stress tolerance. Frontiers in Plant Science , 7, 853. doi: 10.3389/fpls.2016.00853.
- Nover, L., Scharf, K. D., & Neumann, D. (1989). Cytoplasmic heat shock granules are formed from precursor particles and are associated with a specific set of mRNAs. Molecular and Cellular Biology , 9, 1298–1308. doi: 10.1128/MCB.9.3.1298.
- Protter, D. S., & Parker, R. (2016). Principles and properties of stress granules. Trends in Cell Biology , 26, 668–679. doi: 10.1016/j.tcb.2016.05.004.
- Ramaswami, M., Taylor, J. P., & Parker, R. (2013). Altered ribostasis: RNA-protein granules in degenerative disorders. Cell , 154, 727–736. doi: 10.1016/j.cell.2013.07.038.
- Sorenson, R., & Bailey-Serres, J. (2014). Selective mRNA sequestration by oligouridylate-binding protein 1 contributes to translational control during hypoxia in Arabidopsis. Proceedings of the National Academy of Sciences of the United States of America , 111, 2373–2378. doi: 10.1073/pnas.1314851111.
- Takahara, T., & Maeda, T. (2012). Transient sequestration of TORC1 into stress granules during heat stress. Molecular Cell , 47, 242–252. doi: 10.1016/j.molcel.2012.05.019.
- Wheeler, J. R., Jain, S., Khong, A., & Parker, R. (2017). Isolation of yeast and mammalian stress granule cores. Methods , 126, 12–17. doi: 10.1016/j.ymeth.2017.04.020.
- Wheeler, J. R., Matheny, T., Jain, S., Abrisch, R., & Parker, R. (2016). Distinct stages in stress granule assembly and disassembly. eLife , 5, e18413. doi: 10.7554/eLife.18413.
- Yan, C., Yan, Z., Wang, Y., Yan, X., & Han, Y. (2014). Tudor-SN, a component of stress granules, regulates growth under salt stress by modulating GA20ox3 mRNA levels in Arabidopsis. Journal of Experimental Botany , 65, 5933–5944. doi: 10.1093/jxb/eru334.
- Youn, J. Y., Dyakov, B. J. A., Zhang, J., Knight, J. D. R., Vernon, R. M., Forman-Kay, J. D., & Gingras, A. C. (2019). Properties of stress granule and P-body proteomes. Molecular Cell , 76, 286–294. doi: 10.1016/j.molcel.2019.09.014.
Citing Literature
Number of times cited according to CrossRef: 5
- Itzell Hernández‐Sánchez, Tobias Rindfleisch, Jessica Alpers, Martin Dulle, Christopher J. Garvey, Patrick Knox‐Brown, Markus S. Miettinen, Gergely Nagy, Julio M. Pusterla, Agata Rekas, Keyun Shou, Andreas M. Stadler, Dirk Walther, Martin Wolff, Ellen Zuther, Anja Thalhammer, Functional in vitro diversity of an intrinsically disordered plant protein during freeze–thawing is encoded by its structural plasticity, Protein Science, 10.1002/pro.4989, 33 , 5, (2024).
- Huijuan Tan, Wentao Luo, Wei Yan, Jie Liu, Yalikunjiang Aizezi, Ruixue Cui, Ruijun Tian, Jinbiao Ma, Hongwei Guo, Phase separation of SGS3 drives siRNA body formation and promotes endogenous gene silencing, Cell Reports, 10.1016/j.celrep.2022.111985, 42 , 1, (111985), (2023).
- Monika Chodasiewicz, J. C. Jang, Emilio Gutierrez-Beltran, Editorial: Biology of Stress Granules in Plants, Frontiers in Plant Science, 10.3389/fpls.2022.938654, 13 , (2022).
- Aleksandra Skirycz, Alisdair R Fernie, Past accomplishments and future challenges of the multi-omics characterization of leaf growth, Plant Physiology, 10.1093/plphys/kiac136, 189 , 2, (473-489), (2022).
- Monika Chodasiewicz, Olga Kerber, Michal Gorka, Juan C Moreno, Israel Maruri-Lopez, Romina I Minen, Arun Sampathkumar, Andrew D L Nelson, Aleksandra Skirycz, 2′,3′-cAMP treatment mimics the stress molecular response in Arabidopsis thaliana , Plant Physiology, 10.1093/plphys/kiac013, 188 , 4, (1966-1978), (2022).