Screening Method for the Identification of Compounds That Activate Pregnane X Receptor
Caitlin Lynch, Caitlin Lynch, Srilatha Sakamuru, Srilatha Sakamuru, Menghang Xia, Menghang Xia
Abstract
The pregnane X receptor (PXR) is a nuclear receptor found mainly in the liver and intestine, whose main function is to regulate the expression of drug-metabolizing enzymes and transporters. Recently, it has been noted that PXR plays critical roles in energy homeostasis, immune response, and cancer. Therefore, identifying chemicals or compounds that can modulate PXR is of great interest, as these can result in downstream toxicity or, alternatively, may have therapeutic potential. Testing one compound at a time for PXR activity would be inefficient and take thousands of hours for large compound libraries. Here, we describe a high-throughput screening method that encompasses plating and treating HepG2-CYP3A4-hPXR cells in a 1536-well plate, as well as reading and interpreting assay (e.g., luciferase reporter gene activity) endpoints. These cells are stably transfected with a human PXR expression vector and CYP3A4 -promoter-driven luciferase reporter vector, allowing the identification of compounds that activate PXR through cytochrome 450 3A4. We also describe how to analyze the data from each assay and explain follow-up steps, namely pharmacological characterization and quantitative polymerase chain reaction (qPCR) assays, which can be performed to confirm results from the original screen. These methods can be used to identify and confirm hPXR activators after completion of a compound screening. Published 2022. This article is a U.S. Government work and is in the public domain in the USA. Current Protocols published by Wiley Periodicals LLC.
Basic Protocol 1 : Establishment of a high-throughput assay to identify hPXR activators
Basic Protocol 2 : Quantitative high-throughput screening a compound library to classify hPXR activators
Basic Protocol 3 : Performing pharmacological characterization and qPCR assays to confirm hPXR activators
INTRODUCTION
Nuclear receptors belong to an important family of proteins that act as transcription factors regulating the expression of genes involved in metabolism, cell growth, and development (Schulman, 2010). In particular, the pregnane X receptor (PXR) controls drug-metabolizing enzymes (DMEs) and plays roles in energy homeostasis, inflammation, and cell proliferation (Kliewer et al., 1998; Kumar & Surapaneni, 2001; Sueyoshi & Negishi, 2001). Given the increasing amounts of chemicals being released into the atmosphere each year, alongside the novel drugs being produced, the development of quick and easy approaches to identify toxic and organism-interacting compounds is becoming increasingly relevant. As such, it has become of great interest to identify compounds that can modulate the induction or activity of PXR.
Low-throughput assays that test only a few compounds at a time are currently being used. It is relatively easy and accurate to transfect PXR expression and CYP3A4 promoter–luciferase vectors into each well of a 6-well, 12-well, or 24-well plate. However, without a high-throughput screening method, it would take years to test every chemical for PXR activation. Recently, a quantitative high-throughput method to identify chemicals that can act as human PXR activators has been reported (Lynch et al., 2021). This assay utilizes a double-stable cell line including the previously mentioned vectors for PXR and CYP3A4. The HepG2-CYP3A4-hPXR cell line (Lin et al., 2008) generates an easy-to-work with and ideal model for screening compounds in a high-throughput manner, producing the results in days, not years.
In this article, we describe how to set up a high-throughput screen, using the previously reported method (Lynch et al., 2021), so that a quick and accurate classification of PXR activators can be made. We also provide steps to perform follow-up studies to ascertain the validity of the high-throughput screening data. By identifying PXR activators, therapeutic applications, potential drug-drug interactions, or other toxic implications can be identified.
To identify and confirm a compound as a PXR activator, the following procedure (Fig. 1) should be followed. Basic Protocol 1 explains how to optimize a high-throughput cell-based luciferase reporter gene assay to identify PXR activators from a large library of compounds. The next logical step is to execute the high-throughput screen itself (Basic Protocol 2). This can be done using 96-, 384-, or 1536-well plates so that many compounds can be thoroughly examined in one efficient assay. Finally, Basic Protocol 3 portrays two different follow-up studies, including a pharmacological assay that can be used to measure the shift in potency, after the addition of a selective PXR antagonist, of the studied compound and a real-time quantitative polymerase chain reaction (RT-qPCR) to measure the induction of PXR's most important drug metabolizing enzyme, CYP3A4. These two assays are performed in order to confirm the compounds identified in Basic Protocol 2 are true PXR activators.
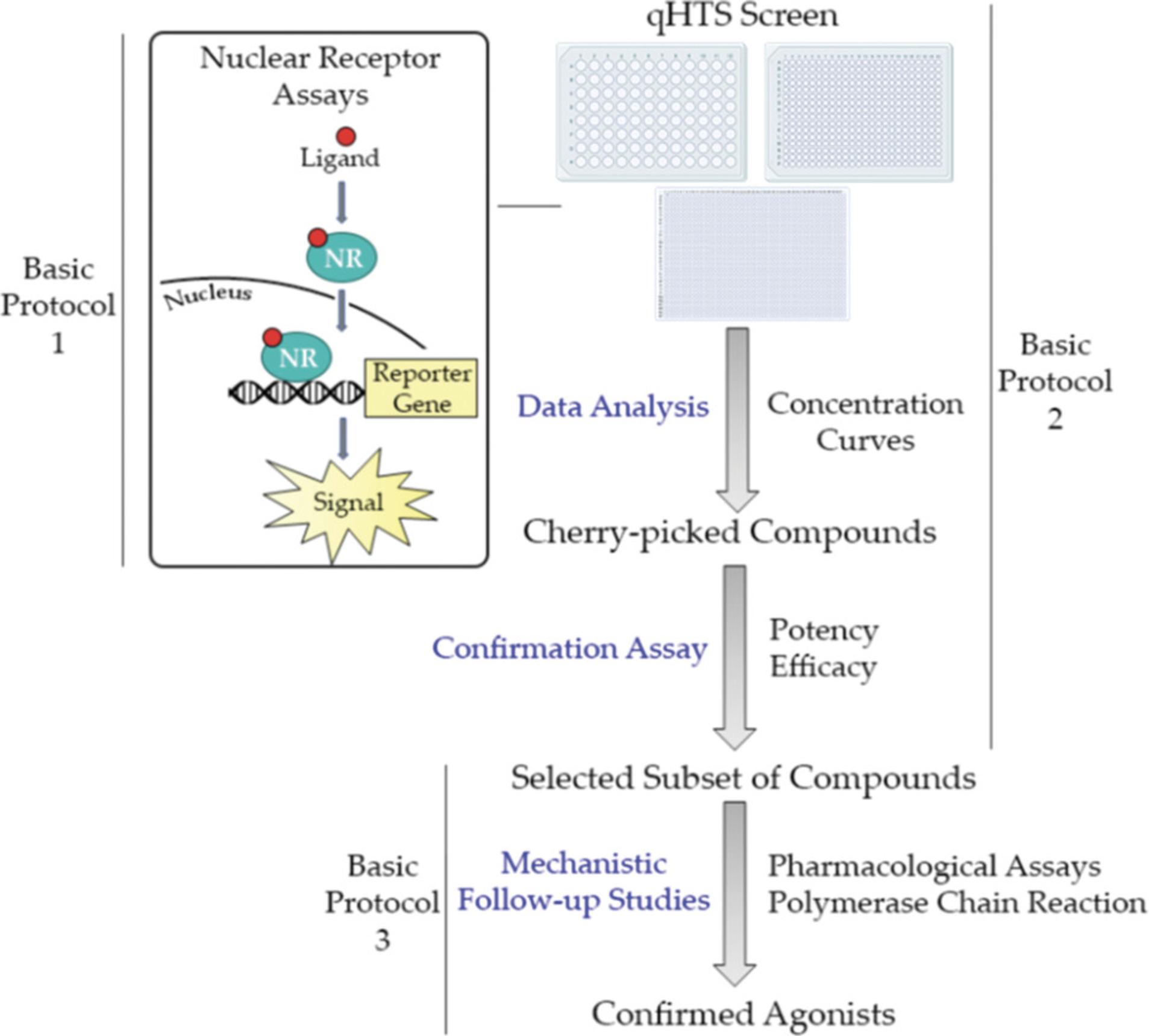
Basic Protocol 1: ESTABLISHMENT OF A HIGH-THROUGHPUT ASSAY TO IDENTIFY hPXR ACTIVATORS
Optimization of a new assay can be time-consuming and sometimes challenging; to overcome these difficulties, the following protocol describes a step-by-step methodology for generating an in vitro high-throughput assay, employing luciferase reporter gene technology, to identify PXR activators. The first steps describe thawing and culturing a double-stable cell line that includes an hPXR expression vector and CYP3A4-luciferase plasmid (Lin et al., 2008). A double-stable cell line is preferred so that transient transfection does not need to be performed; eliminating this decreases the experiment time and creates more consistent data by generating a uniform population of cells containing both vectors in each well of the assay plate. Every laboratory needs to optimize the assay by testing a different number of cells/well, positive control concentration, and/or equipment settings. Therefore, an optimization step to address each of these items is necessary. Here, we show how to test multiple concentrations of cells/well and explain how to determine the optimal conditions for the assay. Once the optimization is complete, the assay will be ready for use in a high-throughput manner and ready to screen hundreds or thousands of compounds in one experiment.
NOTE : This protocol must be completed in a biological safety cabinet, to keep contamination to a minimum.
Materials
- Eagle's Minimum Essential Medium (EMEM; ATCC, cat. no. 30-2003)
- HyClone Characterized Fetal Bovine Serum (FBS; Cytiva, cat. no. SH30071.03)
- 10,000 U/ml Penicillin-Streptomycin (Pen-Strep; ThermoFisher Scientific, cat. no. 15140122)
- HepG2-CYP3A4-hPXR stable cell line (Dr. Taosheng Chen, Department of Chemical Biology and Therapeutics, St. Jude Children's Research Hospital; Lin et al., 2008)
- 70% (v/v) ethanol
- PXR culture medium (see recipe), prewarmed to 37°C
- 0.25% trypsin-EDTA with phenol red (ThermoFisher Scientific, cat. no. 25200056)
- Phosphate-buffered saline (PBS), pH 7.4, calcium chloride and magnesium chloride free (ThermoFisher Scientific, cat. no. 10010072)
- PXR assay medium (see recipe), prewarmed to 37°C
- Dimethyl sulfoxide (DMSO; Millipore Sigma, cat. no. 472301, CAS no. 67-68-5)
- Rifampicin (MilliporeSigma, cat. no. R3501, CAS no. 13292-46-1)
- Tetraoctylammonium bromide (Millipore Sigma, cat. no. 294136, CAS no. 14866-33-2)
- ONE-Glo Luciferase Assay System (Promega, cat. no. E6130)
- Biological safety cabinet (Labconco, cat. no. 302610101, or equivalent)
- Vacuum filter system, 500 ml (Corning Life Sciences, cat. no. 431205)
- Isotemp water bath (Fisher Scientific, cat. no. FSGPD20) or equivalent
- 15-ml tubes (ThermoFisher Scientific, cat. no. 339651, or equivalent)
- 200-µl micropipettor (Rainin, cat. no. 17008652, or equivalent)
- 200-µl pipet tips (Rainin, cat. no. 30389268)
- 10-ml pipets (Corning Life Sciences, cat. no. 357551, or equivalent)
- Centrifuge (ThermoFisher Scientific, cat. no. 75004521, or equivalent)
- Pipettor (Integra Biosciences, cat. no. 155019, or equivalent)
- 225-cm2 (T-225) cell culture flasks (Corning Life Sciences, cat. no. 431082), Millicell HY 5-layer T-1000 cell culture flasks (Millipore Sigma, cat. no. PFHYS1008), or 175-cm2 collagen-I-coated flasks (ThermoFisher Scientific, cat. no. 132708)
- CO2 incubator (ThermoFisher Scientific, cat. no. 3310, or equivalent)
- Tissue culture microscope (Carl Zeiss Microscopy, LLC, cat. no. 491237-0013-000, or equivalent)
- Cell counter (Nexcelom Biosciences, cat. no. Cellometer Auto T4-IQOQ, or equivalent)
- White/solid 1536-well flat-bottom cell-culture-treated plate (Greiner Bio-One, cat. no. 789173-F)
- BioRAPTR2 Flying Reagent Liquid Dispenser (Let's Go Robotics, Inc., model no. BR2) or equivalent
- Microplate lids (Wako Automation, cat. no. 08-3241-0005)
- Clear 1536-well compound plates (Greiner Bio-One, cat. no. 789270-C)
- Pintool Station (Wako Automation, cat. no. Pintool1144-3100 PLUS VELOCITY) or equivalent
- ViewLux uHTS Microplate Imager 1430-0010A (Perkin Elmer, cat. no. 26149) or equivalent
- GraphPad Prism Software (or equivalent)
Thawing HepG2-CYP3A4-hPXR cells
1.In the biological safety cabinet, set up a 500-ml vacuum filter system. Prepare thaw medium by combining 500 ml EMEM, 50 ml FBS, and 5 ml Pen-Strep and filter the liquid.
2.Warm thaw medium to 37°C using a water bath.
3.In the biological safety cabinet, put 9 ml warmed thaw medium into a 15-ml tube.
4.Take a single vial of frozen HepG2-CYP3A4-hPXR cells and place in a 37°C water bath for ∼3 min or until the mix starts to thaw.
5.Wipe the vial with 70% ethanol and then, in the safety cabinet, pour the cell solution into the 9 ml of warmed thaw medium. Remove any remaining liquid in the vial and transfer into the 15-ml tube, using a 200-µl micropipettor or similar.
6.Pipet up and down five to ten times with a 10-ml pipet to break up clumps of cells.
7.Centrifuge cells 4 min at 900 rpm (100 × g), room temperature.
8.Pour off supernatant from cell pellet into a waste bin and add 10 ml fresh prewarmed thaw medium. Pipet cells up and down at least ten times using a 10-ml pipet. Pour this cell suspension into a 175-cm2 collagen-I-coated flask and swirl around slightly.
9.Incubate at 37°C, 5% CO2, for a few days, or until cells are at least 80% confluent.
Culturing HepG2-CYP3A4-hPXR cells
10.Warm up PXR culture medium in a 37°C water bath. Let trypsin solution warm up to room temperature.
11.Pour off used medium from the HepG2-CYP3A4-hPXR cells flask into a waste bin.
12.Add 10 ml PBS to the flask and swirl around. Pour off PBS into waste bin. Repeat two times.
13.Add 6 ml trypsin solution to the flask and swirl around to cover the entire flask. Place the flask in the incubator for 3-5 min until cells start to detach from flask.
14.Add 4 ml prewarmed PXR culture medium to the flask. Swirl or pipet liquid around to release and gather as many cells as possible. Suck up cells and medium and transfer into a 50-ml tube.
15.Centrifuge 4 min at 900 rpm (100 × g), room temperature.
16.Pour off supernatant and resuspend cells in 10 ml fresh prewarmed PXR culture medium. Pipet up and down at least ten times to break up clumps.
17.Acquire a fresh 175-cm2 collagen-I-coated flask and pour 40 ml fresh prewarmed PXR culture medium into the flask.
18.Count the cells and transfer ∼20 million cells into the flask. Place flask in the 37°C, 5% CO2 incubator and incubate until the cells become ∼80% confluent.
19.Culture cells for at least another passage by repeating steps 11-19.This is to ensure that the highest number of the correct cells are present.
Performing optimization assay
20.Warm assay medium to 37°C using a water bath.
21.Repeat steps 12-17 using prewarmed PXR assay medium instead of culture medium. Count the newly made cell suspension.
22.Generate 5.0 × 105 cells/ml and 9.0 × 105 cells/ml solutions using PXR assay medium.
23.Plate 5 µl of each suspension into the first four columns of white 1536-well plates using a liquid dispenser.
24.Place a microplate lid on top of each assay plate and incubate the plates at 37°C, 5% CO2 for ∼4-5 hr.
25.Create a positive control compound plate for the assay using DMSO, rifampicin, and tetraoctylammonium bromide in the first four columns of a fresh clear compound plate. Store at −20°C when not in use.
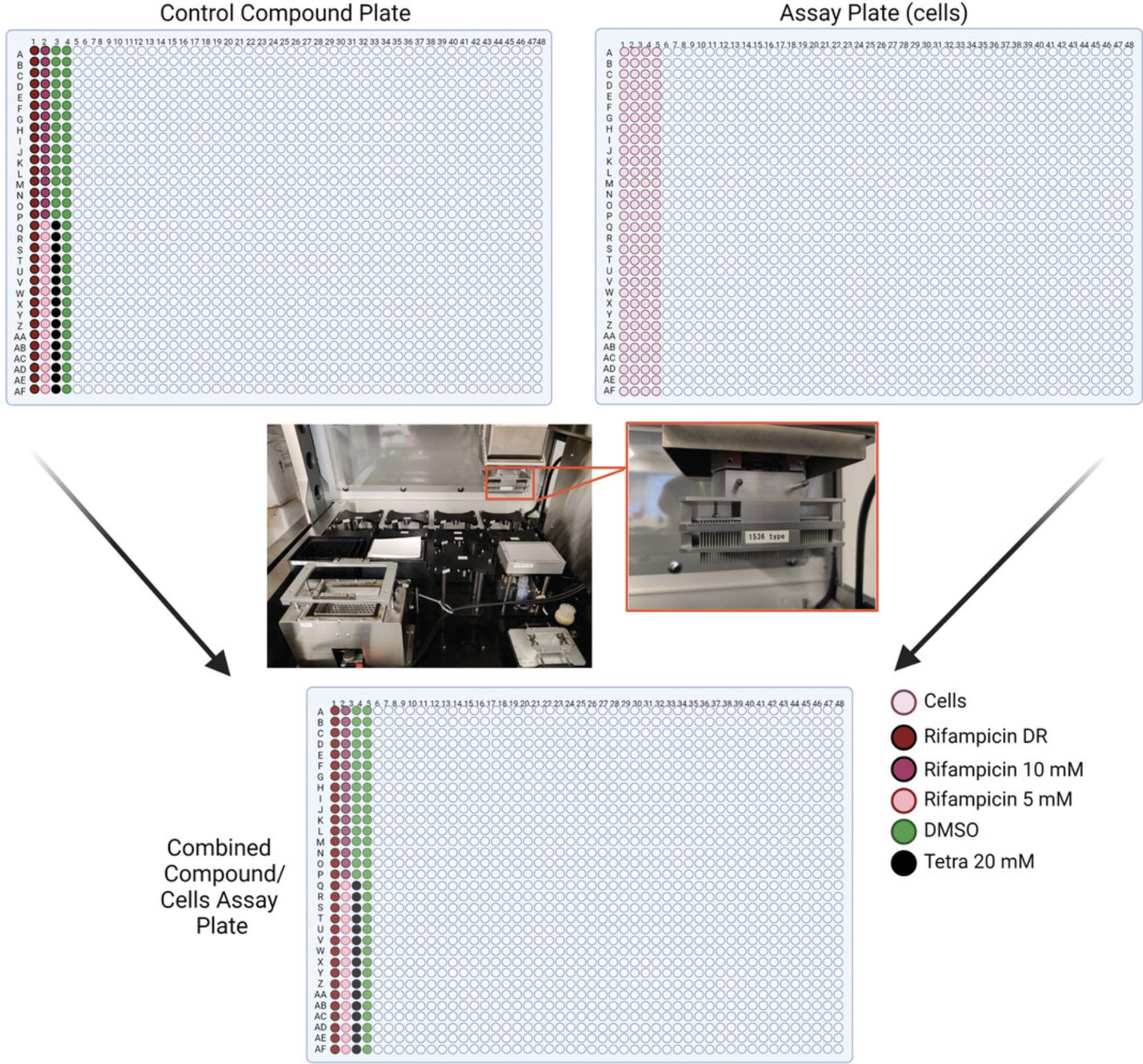
26.Transfer 23 nl from each well of the positive control plate to the cell plate using a Wako Pintool station. Incubate at 37°C, 5% CO2 for 24 hr.
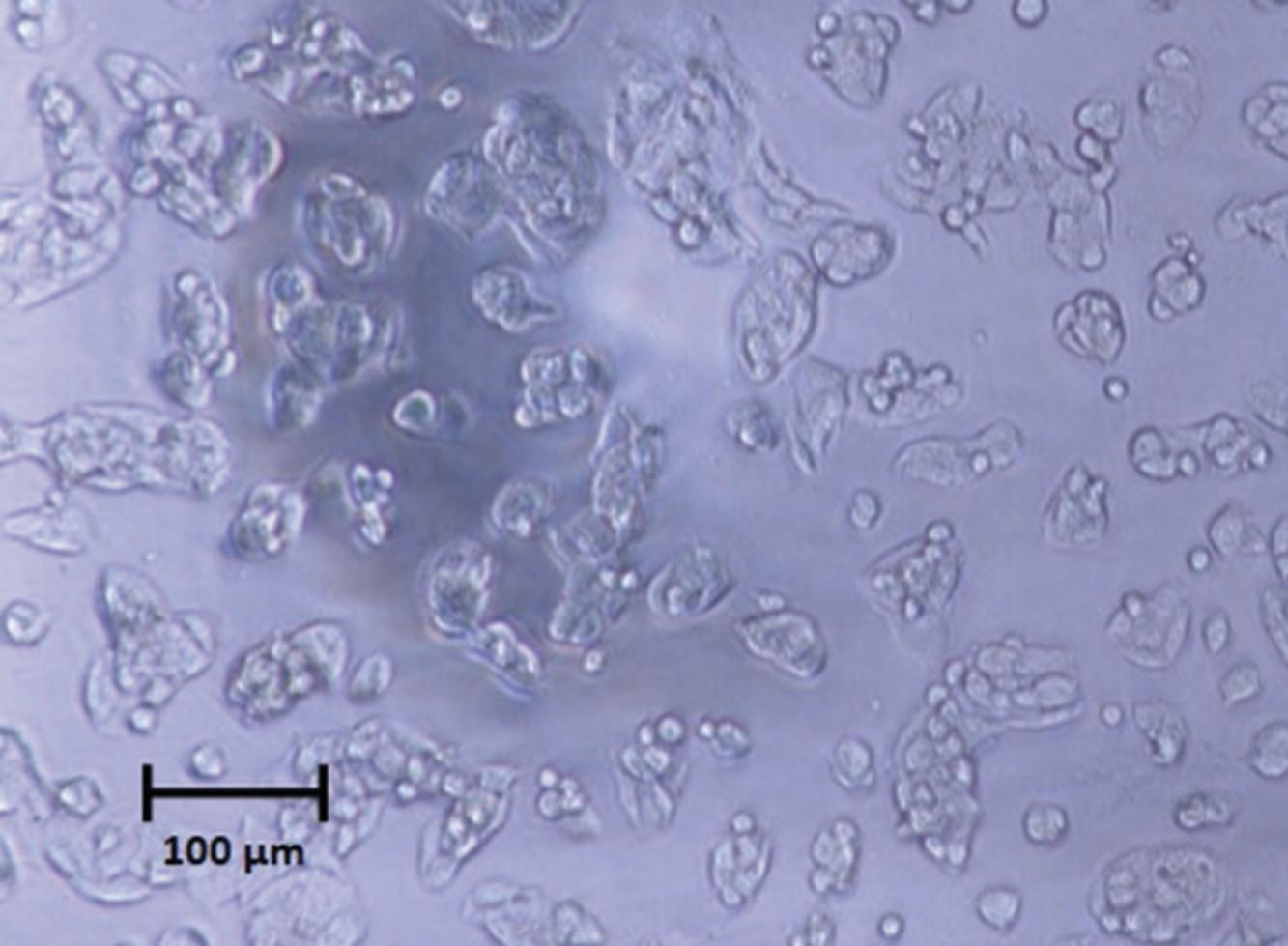
27.Add 4 µl ONE-Glo reagent to each well including cells (first four columns of plates 1 and 2). Incubate at room temperature for 30 min.
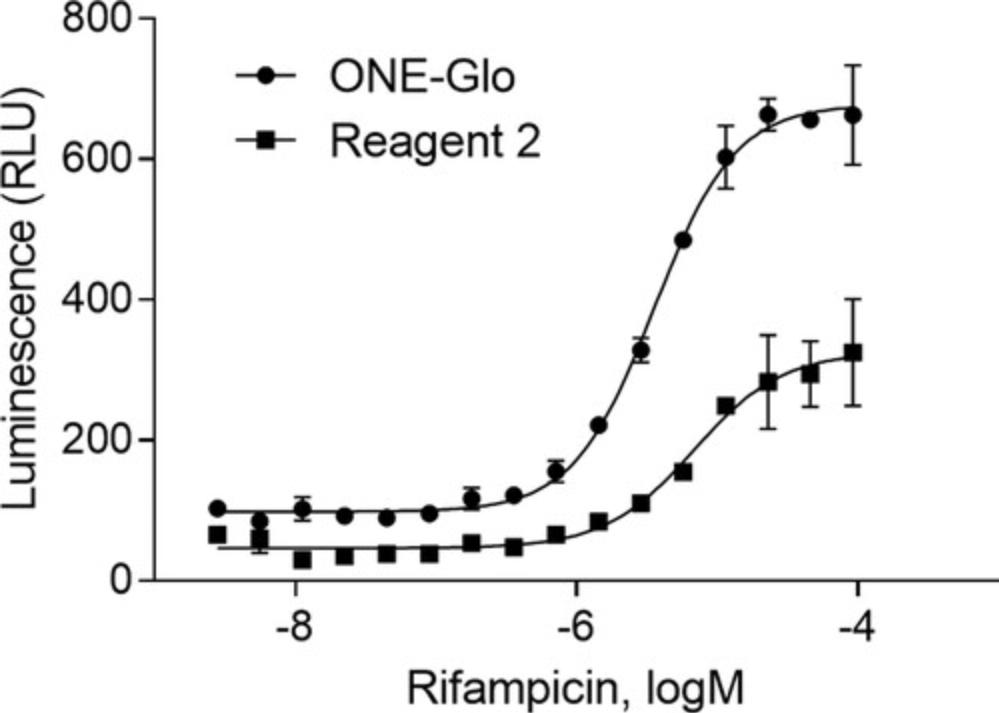
28.Acquire the luminescence signal from the plate using a plate reader.
29.Analyze the data using the equation below, and generate the % activity curve using GraphPad (Fig. 5).
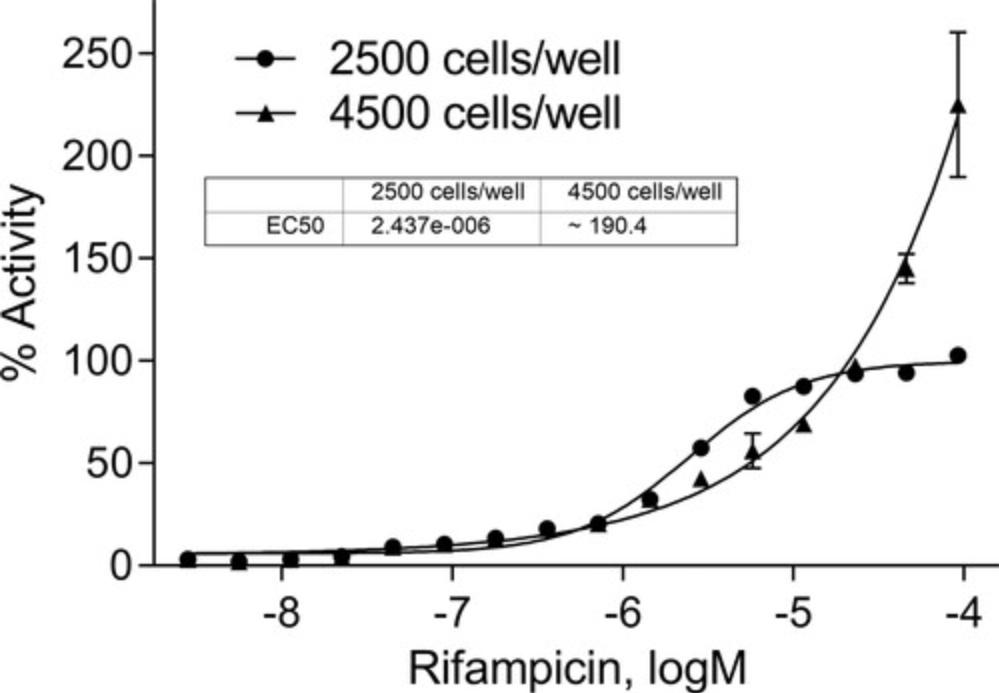
30.Acquire an EC50 for all compounds.
31.Choose the optimum cell number, based on the % activity curve and EC50.
Basic Protocol 2: QUANTITATIVE HIGH-THROUGHPUT SCREENING OF A COMPOUND LIBRARY TO CLASSIFY hPXR ACTIVATORS
Once the assay has been optimized according to Basic Protocol 1, a high-throughput screen can be conducted. Profiling thousands of compounds at one time will reduce total experiment time and reagent supplies. In essence, Basic Protocol 2 is the same assay as that completed in Basic Protocol 1, just on a larger scale. This protocol describes the steps in performing a high-throughput luciferase reporter gene assay to identify hPXR activators from a large library of compounds. After the screening is complete, the potency and efficacy of each compound tested can be calculated. The potential PXR agonists identified from the screen can be further examined in the confirmation assays (covered in Basic Protocol 3). This protocol will also explain how to identify the most potent and efficacious compounds by calculating the maximum response and EC50 values; however, there is a need to account for the shape of the concentration curve as well, previously explained in a methods chapter (Huang, 2016).
NOTE : This protocol must be completed in a biological safety cabinet, to keep contamination to a minimum.
Materials
- HepG2-CYP3A4-hPXR stable cell line (Dr. Taosheng Chen, Department of Chemical Biology and Therapeutics, St. Jude Children's Research Hospital; Lin et al., 2008)
- PXR assay medium (see recipe)
- Phenol-red-free Dulbecco's Modified Eagle's Medium (DMEM; ThermoFisher Scientific, cat. no. 31053028)
- FBS, charcoal stripped (ThermoFisher Scientific, cat. no. 12676029)
- 100 mM sodium pyruvate (ThermoFisher Scientific, cat. no. 11360070)
- 200 mM l-glutamine (ThermoFisher Scientific, cat. no. 25030081)
- Positive control plate (Basic Protocol 1, step 26)
- Compound library plates: e.g., Tox21 10K compound library plates (Attene-Ramos et al., 2013)
- ONE-Glo Luciferase Assay System (Promega, cat. no. E6130)
- Biological safety cabinet (Labconco, cat. no. 302610101, or equivalent)
- Isotemp water bath (Fisher Scientific, cat. no. FSGPD20) or equivalent
- CO2 incubator (ThermoFisher Scientific, cat. no. 3310, or equivalent)
- 50-ml tubes (Corning Life Sciences, cat. no. 352070, or equivalent)
- Centrifuge (ThermoFisher Scientific, cat. no. 75004521, or equivalent)
- 225-cm2 (T-225) cell culture flasks (Corning Life Sciences, cat. no. 431082), Millicell HY 5-layer T-1000 cell culture flasks (Millipore Sigma, cat. no. PFHYS1008), or 175-cm2 collagen-I-coated flasks (ThermoFisher Scientific, cat. no. 132708)
- 10-ml pipets (Corning Life Sciences, cat. no. 357551, or equivalent)
- Pipettor (Integra Biosciences, cat. no. 155019, or equivalent)
- Tissue culture microscope (Carl Zeiss Microscopy, LLC, cat. no. 491237-0013-000, or equivalent)
- Cell counter (Nexcelom Biosciences, cat. no. Cellometer Auto T4-IQOQ, or equivalent)
- White/solid 1536-well flat-bottom cell-culture-treated plate (Greiner Bio-One, cat. no. 789173-F)
- Multidrop™ Combi Reagent Dispenser (ThermoFisher Scientific, cat. no. 5840300), or equivalent
- Microplate lids (Wako Automation, cat. no. 08-3241-0005)
- Pintool Station (Wako Automation, cat. no. Pintool1144-3100 PLUS VELOCITY), or equivalent
- ViewLux Plate Reader (Perkin Elmer, cat. no. 1430-0010A, or equivalent)
- GraphPad Prism Software (or equivalent)
1.Warm assay medium to 37°C using a water bath. Let trypsin warm up to room temperature.
2.Perform steps 12-17 of Basic Protocol 1, using assay medium instead of culture medium. Count the newly made cell suspension.
3.Generate a 5.0 × 105 cell/ml solution. Plate 5 µl of cell suspension into every well of solid white 1536-well plates using a multidrop liquid dispenser. Place a microplate lid on top of each plate, place the plates in a 37°C, 5% CO2 incubator, and incubate ∼4-5 hr.
4.Transfer 23 nl of the previously generated positive control plate from Basic Protocol 1, step 26, as well as 23 nl from the compound library plates, to the cell plate using a Wako Pintool station (Fig. 6).
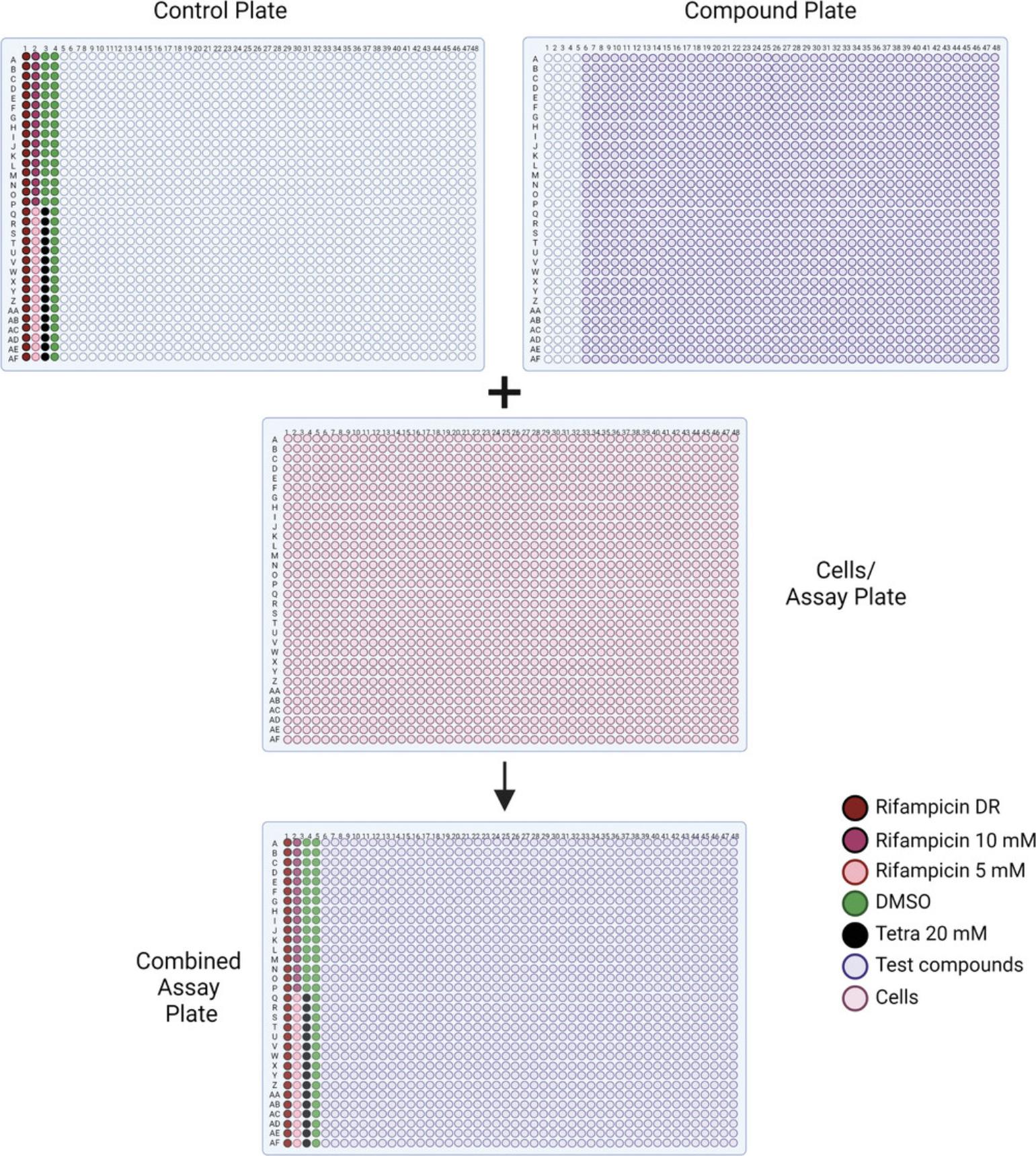
5.Incubate at 37°C, 5% CO2 for 24 hr.
6.Follow steps 28-31 of Basic Protocol 1.
7.Select positive compounds as well as a few negative compounds. Repeat assay with newly formed compound plates comprising those selected compounds.
8.Proceed to Basic Protocol 3 to complete follow-up studies on a small collection of the most promising compounds.
Basic Protocol 3: PERFORMING PHARMACOLOGICAL CHARACTERIZATION AND qPCR ASSAYS TO CONFIRM hPXR ACTIVATORS
Once a manageable group of compounds has been chosen after a primary screening, such as in Basic Protocol 2, additional studies can be conducted to further confirm compound modulation of PXR. Here we first discuss how to perform a pharmacological study, which will display a shift in EC50 values and concentration response curves if the compound's activity is due to PXR activation. If, after the addition of increasing amounts of PXR antagonist (SPA70; Lin et al., 2008), a compound's EC50 value becomes higher in conjunction with a right-shifting concentration response curve, the compound is further confirmed as a PXR activator. The second section demonstrates how to perform an RT-qPCR assay to identify the induction or inhibition of the mRNA expression of a particular gene. In this case, we are looking at the main DME that PXR regulates, CYP3A4.If a compound activates PXR, it should also increase the expression level of CYP3A4. The combination of these assays should corroborate the previous data, from Basic Protocol 2, and a safe conclusion can be made to identify a compound as a true PXR activator.
NOTE : This protocol must be completed in a biological safety cabinet, to keep contamination to a minimum.
Materials
- Candidate compounds (Basic Protocol 2, step 7)
- HepG2-CYP3A4-hPXR stable cell line (Dr. Taosheng Chen, Department of Chemical Biology and Therapeutics, St. Jude Children's Research Hospital; Lin et al., 2008)
- PXR assay medium (see recipe)
- Dimethyl sulfoxide (DMSO; Millipore Sigma, cat. no. 472301, CAS no. 67-68-5)
- SPA70 (Millipore Sigma, cat. no. SML2662, CAS no. 931314-31-7)
- ONE-Glo Luciferase Assay System (Promega, cat. no. E6130)
- William's E Medium, phenol red free (ThermoFisher Scientific, cat. no. A1217601)
- HepaRG™ Thawing and Plating Medium Supplement (Lonza Bioscience, cat. no. MHTAP)
- 10,000 U/ml Penicillin-Streptomycin (Pen-Strep; ThermoFisher Scientific, cat. no. 15140122)
- 70% (v/v) ethanol
- NoSpin™ HepaRG™ cells (Lonza Bioscience, cat. no. NSHPRG)
- PBS, pH 7.4, calcium chloride and magnesium chloride free (ThermoFisher Scientific, cat. no. 10010072)
- RNeasy Midi Kit (Qiagen, cat. no. 75144)
- High-Capacity RNA-to-cDNA™ Kit (ThermoFisher Scientific, cat. no. 4387406)
- TaqMan™ Gene Expression Master Mix (ThermoFisher Scientific, cat. no. 4370074)
- UltraPure™ DNase/RNase-Free Distilled Water (ThermoFisher Scientific, 10977023)
- Human GAPDH Endogenous Control (FAM™/MGB probe, non-primer limited; ThermoFisher Scientific, cat. no. 4352934E, Assay ID: Hs99999905_m1)
- Human CYP3A4 (FAM™/MGB probe; ThermoFisher Scientific, cat. no. 4331182, Assay ID: Hs00604506_m1)
- Clear 1536-well compound plates (Greiner Bio-One, cat. no. 789270-C)
- Biological safety cabinet (Labconco, cat. no. 302610101, or equivalent)
- Tissue culture microscope (Carl Zeiss Microscopy, LLC, cat. no. 491237-0013-000, or equivalent)
- 50-ml tubes (Corning Life Sciences, cat. no. 352070, or equivalent)
- 200-µl micropipettor (Rainin, cat. no. 17008652, or equivalent)
- 200-µl pipet tips (Rainin, cat. no. 30389268)
- White/solid 1536-well flat-bottom cell-culture-treated plate (Greiner Bio-One, cat. no. 789173-F)
- BioRAPTR2 Flying Reagent Liquid Dispenser (Let's Go Robotics, Inc., Model No. BR2), or equivalent
- Microplate lids (Wako Automation, cat. no. 08-3241-0005)
- CO2 incubator (ThermoFisher Scientific, cat. no. 3310, or equivalent)
- Pintool Station (Wako Automation, cat. no. Pintool1144-3100 PLUS VELOCITY) or equivalent
- ViewLux Plate Reader (Perkin Elmer, cat. no. 1430-0010A) or equivalent
- GraphPad Prism Software (or equivalent)
- Isotemp water bath (Fisher Scientific, cat. no. FSGPD20) or equivalent
- 10-ml pipets (Corning Life Sciences, cat. no. 357551, or equivalent)
- Pipettor (Integra Biosciences, cat. no. 155019, or equivalent)
- Centrifuge (ThermoFisher Scientific, cat. no. 75004521, or equivalent)
- BioCoat® Collagen-1-coated 12-well plate (Corning Life Sciences, cat. no. 354500)
- Nanodrop spectrophotometer (DeNovix DS-11+) or equivalent
- MicroAmp® Optical 384-Well Reaction Plate with Barcode (ThermoFisher Scientific, cat. no. 4309849)
- QuantStudio 5 Real-Time PCR System (ThermoFisher Scientific, ref. no. A28135) or equivalent
- MicroAmp® Optical Adhesive Film (ThermoFisher Scientific, cat. no. 4311971)
- Sealing roller
- Cell counter (Nexcelom Biosciences, cat. no. Cellometer Auto T4-IQOQ or equivalent)
- Thermal Cycler Model 2720 (Applied Biosystems, cat. no. 4359659) or equivalent
Executing the pharmacologic study
1.Aliquot the promising compounds chosen, from Basic Protocol 2, step 7, into clear 1536-well compound plates.
2.Generate a HepG2-CYP3A4-hPXR cell suspension, using assay medium from the cells still being passaged from Basic Protocol 1, step 20, with a concentration of 6.25 × 105 cells/ml. Plate 4 µl cell suspension into every well of white/solid 1536-well plates using a liquid dispenser. Place a lid on top of each plate, place the plates in a 37°C, 5% CO2 incubator, and incubate ∼4-5 hr.
3.Transfer 23 nl from each well of the previously generated positive control plate from Basic Protocol 1, step 26, as well as 23 nl from each well of the compound plates produced in step 2, to each cell plate using a Wako Pintool station (Fig. 6).
4.Using assay medium, make 2.5 ml (per plate) of co-treatment (2.5 µM SPA70, 3.75 µM SPA70, and DMSO):
- a.Add 6.25 µl of 1 mM SPA70 stock (in DMSO) to 2.5 ml of assay medium to generate 2.5 µM SPA70.
- b.Add 9.38 µl of 1 mM SPA70 stock (in DMSO) to 2.5 ml of assay medium to generate 3.75 µM SPA70.
- c.
Add 9.38 µl of DMSO to 2.5 ml of assay medium to represent the negative control.
Always use the same amount of DMSO as the highest amount of compound added. SPA70 is a known selective PXR antagonist (Lin et al., 2008). The concentrations of SPA70 chosen here were identified using optimization assays. However, the concentrations may vary depending on the performance of the assay in a different laboratory.
5.In every well of the respective plates, add 1 µl of DMSO co-treatment to plate 1, 2.5 µM SPA70 (final concentration of 0.5 µM) co-treatment to plate 2, and 3.75 µM SPA70 (final concentration of 0.75 µM) co-treatment to plate 3.
6.Follow steps 28-30 of Basic Protocol 1 to perform the luciferase reporter gene experiment on these plates.
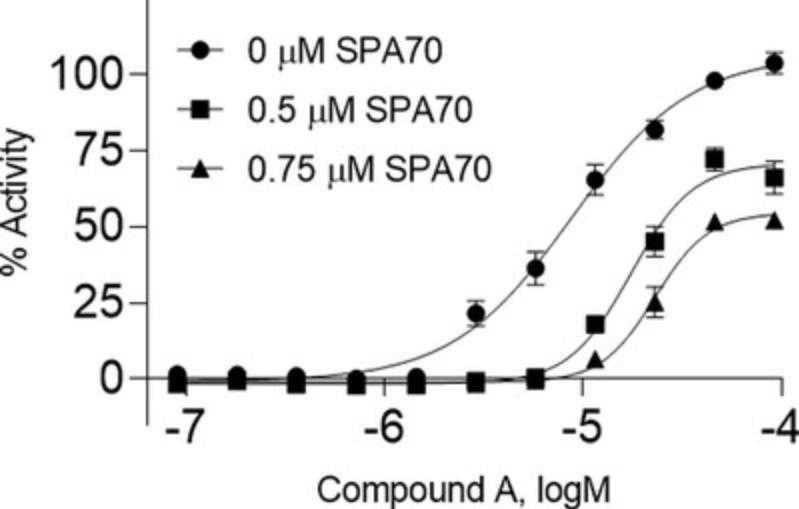
Quantitative real-time polymerase chain reaction
7.Make HepaRG™ culture medium by combining 200 ml of William's E Medium, 23.6 ml of HepaRG™ Thawing and Plating Medium Supplement (MHTAP), and 0.5 ml (25 U/ml) of Pen-Strep.
8.Warm the HepaRG™ culture medium in a 37°C water bath.
9.Thaw a frozen vial of HepaRG™ cells in a 37°C water bath until only a small piece of ice remains (∼90 sec).
10.Add the entire vial of cells into 9 ml of the prewarmed HepaRG™ culture medium.
11.Centrifuge 5 min at 900 rpm (100 × g), room temperature.
12.Remove the supernatant and resuspend the pellet using 10 ml fresh prewarmed HepaRG™ culture medium.
13.Plate HepaRG™ cells in a 12-well collagen-I-coated plate at a concentration of 8 × 105 cells/well (Day 0).
14.Incubate the plates at 37°C, 5% CO2.
15.Acquire the EC70 for each selected compound using GraphPad software.
16.On day 3, after determining that the cells are healthy by assessment under a microscope, exchange the old medium with 1 ml fresh medium per well.
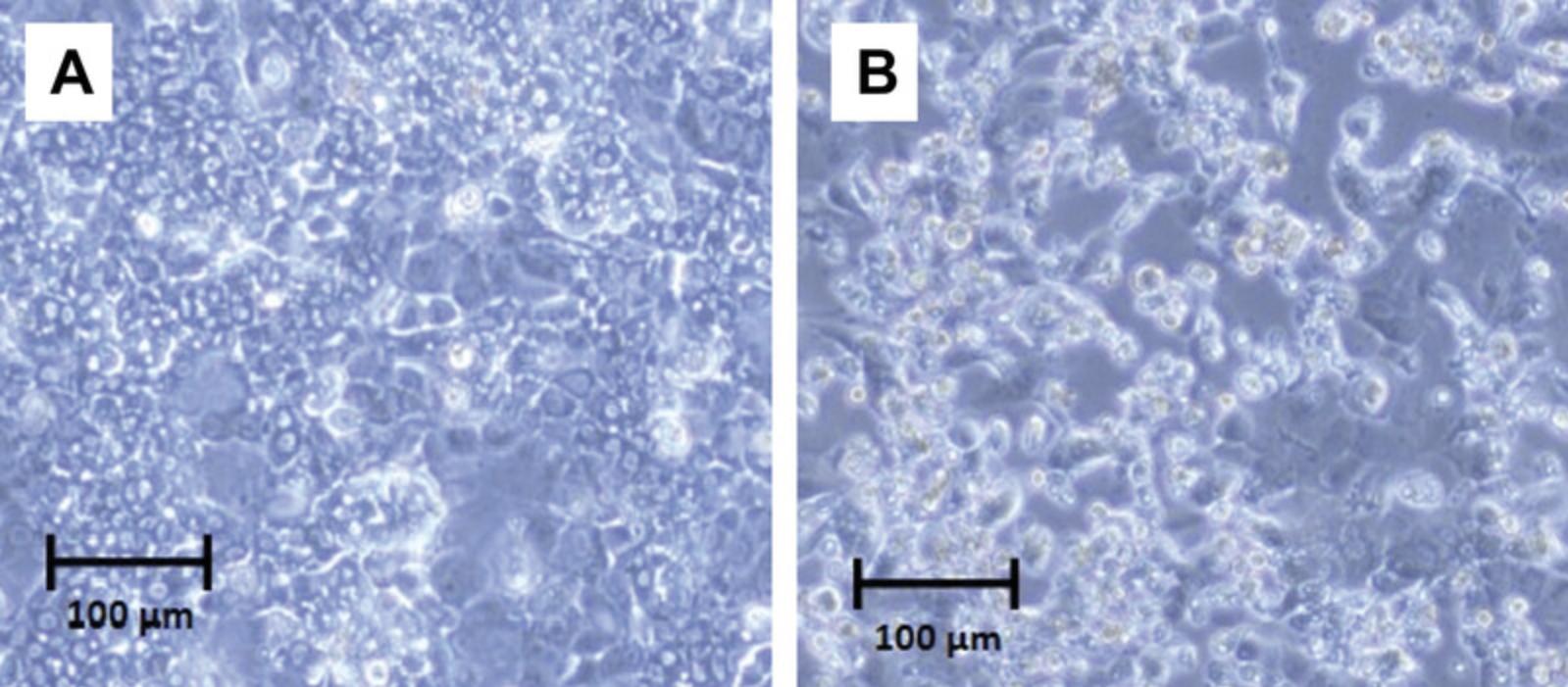
17.Treat the cells with each of the promising compounds (one well per treatment) by adding the correct amount of stock solution to the fresh medium.
18.Gently shake the plate vertically, as well as horizontally, four times. This will ensure that the compound is spread around the well and into the correct homogenous concentration.
19.Replace the treated plate in the 37°C, 5% CO2 incubator and incubate 24 hr.
20.After the 24-hr treatment, examine each well under a microscope.
21.Suck out the medium and wash each well with 1 ml PBS two times.
22.Harvest the cells by adding 350 µl lysis buffer to each well.
23.Perform RNA extraction using the RNeasy kit, following the kit instructions precisely.
24.Determine the RNA concentration of each sample using a spectrophotometer.
25.Using 1 µg cDNA, perform reverse transcriptase using a high-capacity RNA-to-cDNA kit according to the manufacturer's instructions.
26.Add 80 µl DNase/RNase-free distilled water to each sample.
27.Calculate how many wells, in a 384-well PCR assay plate, are needed for each gene. Figure 9 shows an example of a plate setup for five cDNA samples in triplicate.
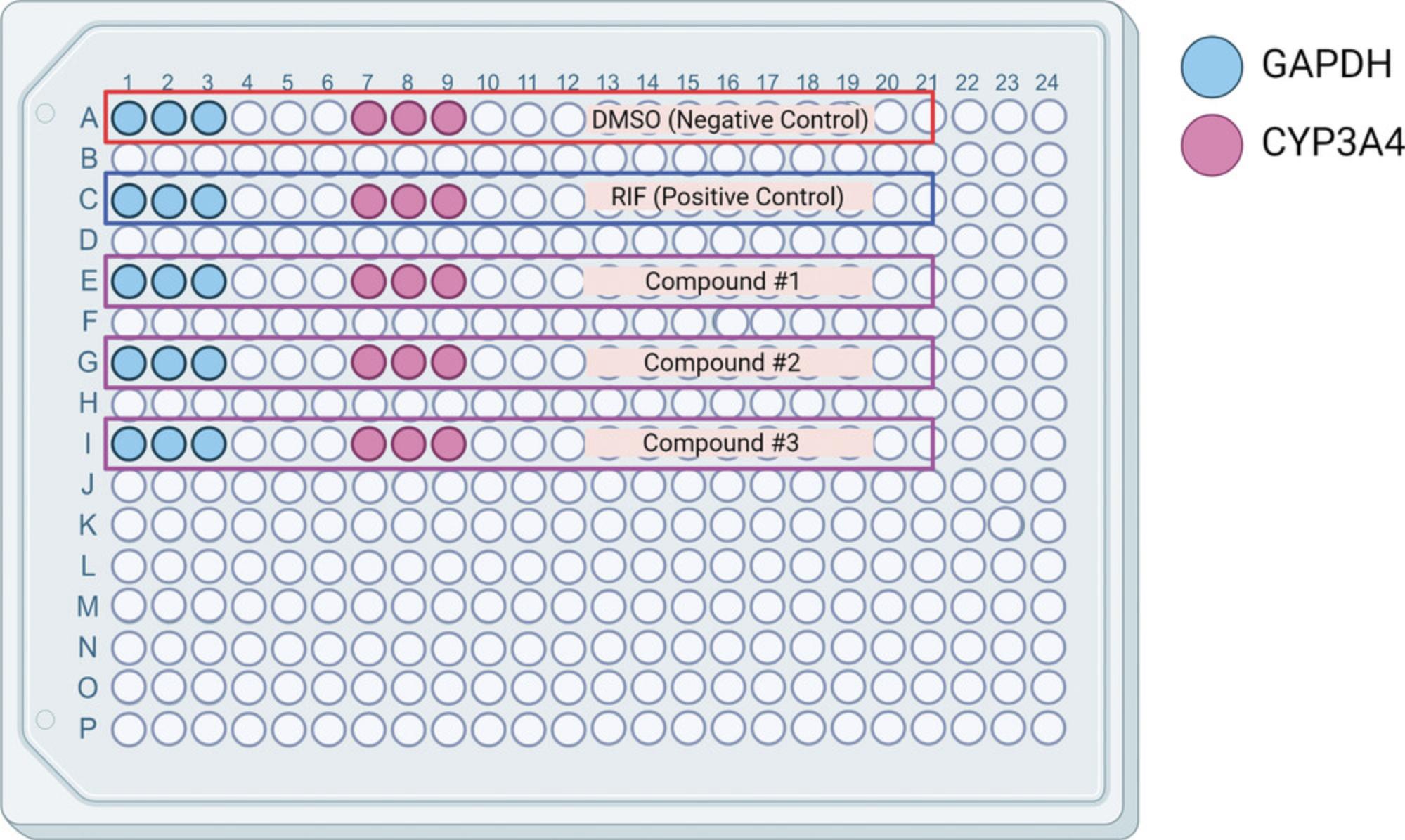
28.Generate gene mixes according to Table 1 using TaqMan™ Master Mix and the appropriate gene probes.
1 well | GAPDH (18 wells) | CYP3A4 (18 wells) | |
---|---|---|---|
2× master mix (µl) | 10 | 180 | 180 |
Probe (µl) | 1 | 18 | 18 |
Water (µl) | 5 | 90 | 90 |
29.Add 16 µl of each mix to the corresponding wells being assayed for that gene.
30.Add 4 µl of each diluted cDNA sample into the correct wells, creating a total of 20 µl per well.
31.Add an adhesive seal firmly onto the plate using a sealing roller, and ensure all sides are correctly stuck.
32.Mix and spin down the plate, using a vortex (for a few seconds at maximum power) and centrifuge (for 1 min at 1000 rpm, or 163 × g), respectively, to ensure there is a homogenous liquid completely spun into the bottom of the well.
33.Place the plate into the corresponding block of a PCR machine (thermal cycler).
34.Perform PCR using a protocol that best fits the primers bought, according to the manufacturer's instructions.
35.Analyze the data according to the fold = 2−ΔΔCt method, where ΔCt represents the differences in cycle threshold numbers between CYP3A4 (or the genes in question) and GAPDH, and ΔΔCt represents the relative change in these differences between negative control and treatment groups (Livak & Schmittgen, 2001).
REAGENTS AND SOLUTIONS
PXR assay medium
- 920 ml phenol-red-free Dulbecco's Modified Eagle's Medium (DMEM; ThermoFisher Scientific, cat. no. 31053028)
- 50 ml charcoal-stripped FBS (ThermoFisher Scientific, cat. no. 12676029; 5% v/v)
- 10 ml 10,000 U/ml Penicillin-Streptomycin (Pen-Strep; ThermoFisher Scientific, cat. no. 15140122; 1% v/v)
- 10 ml of 100 mM sodium pyruvate (ThermoFisher Scientific, cat. no. 11360070; 1 mM)
- 10 ml of 200 mM l-glutamine (ThermoFisher Scientific, cat. no. 25030081; 2 mM)
- Filter under biological safety cabinet conditions
- Store up to 1 month at 4°C.
PXR culture medium
- 880 ml of Eagle's Minimum Essential Medium (EMEM; ATCC, cat. no. 30-2003)
- 100 ml Characterized Fetal Bovine Serum (FBS; Cytiva, cat. no. SH30071.03; 10% v/v)
- 10 ml Pen-Strep (ThermoFisher Scientific, cat. no. 15140122; 1% v/v)
- 10 ml geneticin (ThermoFisher Scientific, cat. no. 10131027; 500 µg/ml
- Filter under biological safety cabinet conditions
- Store up to 1 month at 4°C.
COMMENTARY
Background Information
Identification and characterization of potential novel modulators of nuclear receptors are important steps when identifying toxic drug-drug interactions, determining toxicity, or assessing potential therapeutic benefits. Previously, this process has been low throughput and, therefore, time consuming and slow (Attene-Ramos et al., 2013). The ethics of utilizing in vivo animal models, when other options are available, has also become a global concern (Hsu et al., 2016). However, there have been recent strides in generating a high-throughput method that can be used for different nuclear receptors (Lynch, Mackowiak, et al., 2019; Lynch et al., 2021; Lynch et al., 2017; Lynch, Zhao et al., 2019). When screening in a high-throughput manner, thousands of compounds can be assayed in one experiment, leading to a quick and reliable technique. One of the main disadvantages to these methods is the lack of an efficient metabolic capability. When a drug is ingested, it often undergoes metabolism, and these high-throughput assays do not often account for this natural process. Immortalized cell lines are frequently used in high-throughput screens due to the ease of working with them and their availability, cost, and durability. However, these cells do not usually contain all necessary metabolic components and may not represent human conditions. Therefore, follow-up studies are always an essential part of validating compounds identified from a high-throughput screen.
The detailed protocols described above were finalized after many different optimization steps, including examining various reagents, machines, and equipment settings. However, different machines may require different settings and reagents. For instance, Figure 4 represents the difference between the use of ONE-Glo and Reagent 2, clearly identifying ONE-Glo as the superior reagent in this laboratory for this assay. The cell-type is another aspect that should be addressed when utilizing a luciferase reporter gene assay; transient transfection can be used with low- or medium-throughput assays but will be a disadvantage in a high-throughput assay. When performing a transient transfection, each well must include cells containing both plasmids (i.e., PXR and CYP3A4-luc); the transiently transfected cells often contain heterogenic cell populations, causing a high level of variability in the assay. Therefore, it is crucial to have a stable cell line when performing a high-throughput nuclear receptor luciferase reporter gene assay.
Pharmacological assays employing in vivo animal models are often used to assess a drug's activity (Drug Discovery and Evaluation: Pharmacological Assays, 2016). However, as previously noted, it has become imperative to decrease animal usage in the scientific community. The pharmacological assay described above allows the determination of PXR agonistic activity without the use of animals. Once again, however, a metabolic component is missing from this assay, and further experiments are necessary to verify the activity. In the assay described here, SPA70, a known PXR antagonist, is used as the inhibitor of PXR. Before this compound was identified as a selective and potent PXR antagonist, ketoconazole and sulforaphane were the best options. However, they have shown to be ineffective in vivo, potentially due to toxicity (Lin et al., 2017). Therefore, it is advisable to ensure that the pharmacological assay can be completed using varying concentrations of SPA70.
Quantifying mRNA levels, here of a specific gene being regulated by a nuclear receptor, is a common technique when studying chemical activation. Historically, northern blot analysis, introduced in 1977, was the standard method to detect a change in gene expression (Alwine, Kemp, & Stark, 1977). Utilizing electrophoresis, separated bands of mRNA are transferred from an agarose gel to a membrane. The membrane is then probed and signal is detected. The recently preferred and more precise method, RT-qPCR, is a quicker and more sensitive method that requires a significantly smaller amount of mRNA for detection (Bustin, 2000). However, it also poses some drawbacks, including inappropriate results when the slightest contamination occurs. Therefore, it is imperative to work in as aseptic an area as possible when performing this assay.
Critical Parameters
Basic Protocol 1 needs to be completed within a biological safety cabinet. Many specific steps in an experiment need to be optimized so that the assay will work to its optimum level. Cell type, cell number per assay well, reagents used, treatment time, plate type, and signal output reader are all aspects that must be optimized for this assay. Another vital issue is the positive control plate. As all compounds are compared to the positive and negative controls, it is crucial that these compounds be at their optimal concentration and purity. It is also pivotal to keep the assay plates inside the incubator, at 37°C and 5% CO2, throughout the experiment for as long as possible so that the cells can achieve their healthiest forms.
The main goal of high-throughput screening is to acquire reliable, reproducible data efficiently and quickly. Therefore, the core attribute necessary for Basic Protocol 2 is to generate an assay that is easily duplicated without high levels of human error. Utilizing a robot is the simplest way to produce this type of experiment as it increases screening speed and minimizes mistakes. Because this experiment is a high-throughput version of Basic Protocol 1, many of the same critical parameters apply here as well. However, having access to a double-stable cell line is very important to this assay, as transient transfection may not guarantee assay reproducibility.
One of the most crucial aspects of Basic Protocol 3 is the selection of promising compounds using intuitive parameters (potency, efficacy, curve quality, and reproducibility). For example, a compound may have an efficacy of 100% of the positive control, rifampicin, due to an outlier. In this instance, the concentration curve may be flat with one data point up very high (Fig. 10) generating a false positive; in this case, the compound should not be selected for further, more in-depth review. The pharmacological study has many of the same parameters as Basic Protocol 1, as it is just an addendum to that experiment. However, one thing to be mindful of is selecting a known nuclear receptor inhibitor with good potency and efficacy, such as SPA70 for this specific protocol. When performing experiments requiring the use of mRNA, as when executing RT-qPCR, it is imperative to always use RNase-free water and be mindful of possible contaminants, such as oil from ungloved hands or droplets from talking or coughing near the sample. The cells chosen for the RT-qPCR assay should already have the nuclear receptors and target genes inside the system so that chemical induction can be seen. This experiment will determine how much RNA is being generated, and therefore human primary hepatocytes or HepaRG cells are the optimal cell models for this study. Another point of contention for the RT-qPCR assay is which type of dye to use, e.g., TaqMan versus SYBR Green. This will depend on the primers or probes bought and the specific machine's capability; therefore, it is always necessary to make sure those aspects match up.
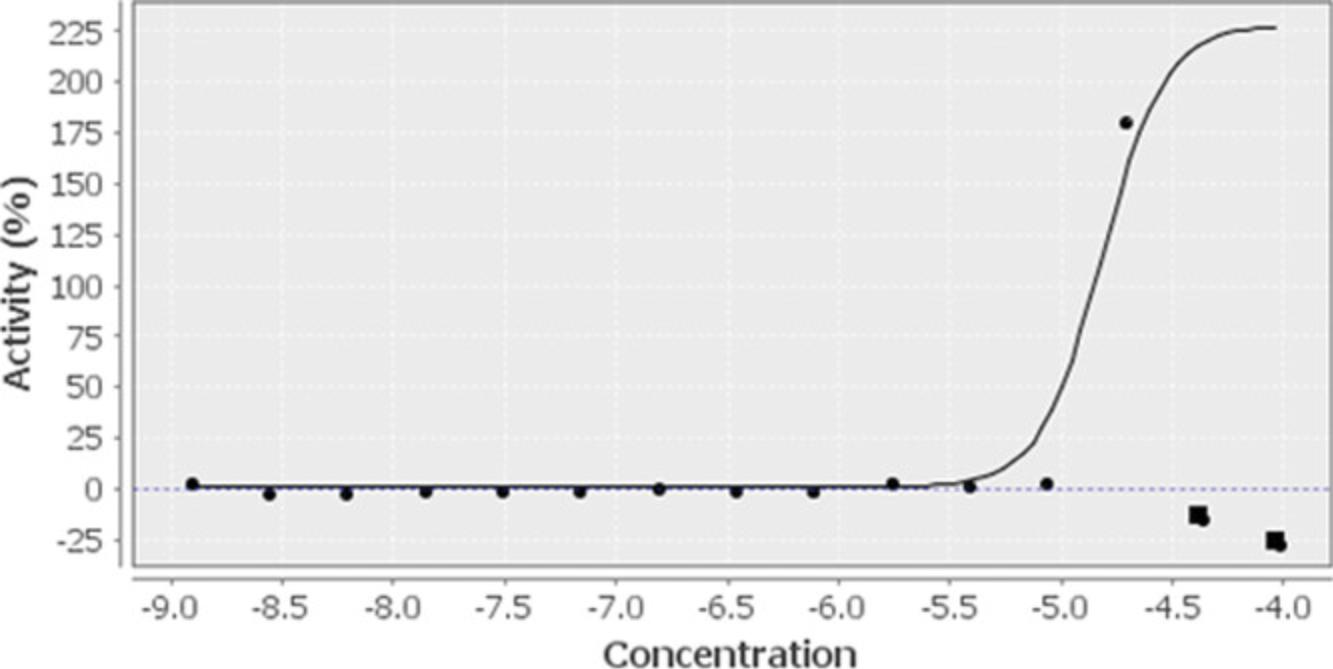
Troubleshooting
Once optimized, the screening protocol should be easy to repeat quickly and efficiently. However, optimization and follow-up steps, such as in these protocols, are nuanced and subject to many potential complications. Table 2 lists some ways in which to avoid these problems by explaining solutions in a clear and precise manner.
Problem | Possible cause | Solution |
---|---|---|
Cells won't detach from flask | Cells not washed with PBS free of CaCl2 and MgCl2 | Suck out trypsin, wash the flask twice with CaCl2- and MgCl2-free PBS, and add fresh trypsin. |
Overexposure when reading luminescence | Overly long exposure | Decrease exposure time. |
Too many cells in each well | Replate cells at a lower density. | |
Low signal when reading luminescence | Insufficiently long exposure | Increase exposure time. |
Not enough cells | Replate cells at a higher density. | |
Positive control not at proper concentration | Redo positive control plate using a higher concentration. | |
Reagent not strong enough | Acquire a different luminescent reagent and try the assay again. | |
Low RNA concentration | Low amount of RNA harvested | Plate more wells and pool samples together. |
Ethanol not added to Buffer RPE (Qiagen RNeasy Midi Kit) | Check that ethanol was added to RPE. If samples have already been extracted without the added ethanol, the experiment will need to be begun again and repeated. | |
Low or no amplification during PCR | Low purity | Re-purify or consult purification kit manual. |
Not enough cDNA | Increase amount of DNA added or number of PCR cycles if suitable. | |
Deficient primers | Check the primer design to ensure they are target specific and complementary. | |
PCR conditions are not ideal | Check the manufacturers’ instructions (for machine, primers, master mix, etc.) and adjust protocol as needed. |
Understanding Results
When performing a luciferase reporter gene assay, the concentration response curve of a compound is the main result that needs to be understood; the potency and efficacy calculated based on this curve using any plotting software (e.g., GraphPad) will characterize whether the compound is a modulator of the nuclear receptor. Comparing a positive control compound to test compounds is an optimal way to analyze the screened data.
Using the results of the equation from Basic Protocol 1, step 30, any compound producing reporter gene activity >100% is more efficacious than the positive control. However, even if a chemical has an efficacy of 100% that of rifampicin, if that potency is seen at a concentration that is not clinically relevant, that compound will not be the best choice to select for further review when assessing the data in humans. Figure 11 displays the concentration response curves from a structural cluster of compounds identified in the PXR activator Tox21 10,000 compound screen. Here, the most potent and efficacious compound, compound A, was chosen for further study (Lynch et al., 2021). Compound C could have also been selected; however, there were other compounds showing similar results and we were interested in identifying as many compounds from different structural clusters as possible. Compound B also had a good curve, but had only a 50% efficacy and a lower potency, ruling it out among other, more qualified compounds. These data represent the expected type of result when performing nuclear receptor luciferase reporter gene assays.
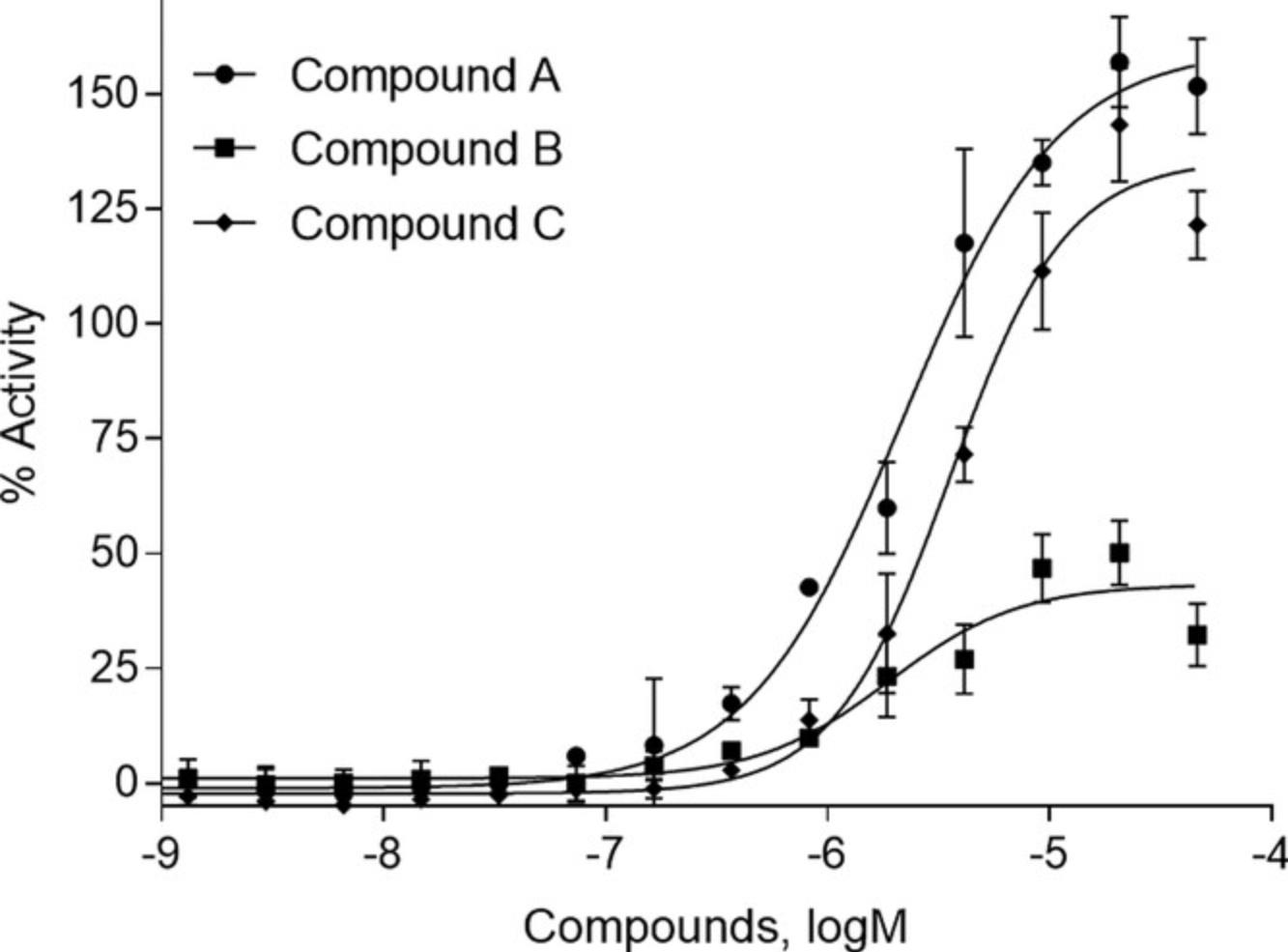
The more in-depth and complicated data to decipher with these methods are those from Basic Protocol 3. The pharmacological assay is similar to the first two basic protocols, in that its endpoint is still concentration response curves. However, when an inhibitor is added to the treatment condition, a positive compound will generate a curve shifted to the right as compared to that without inhibitor addition (Fig. 7). This right-shift means that the EC50 will be higher, equaling a reduced potency. Compound A, the previously chosen compound, shows a right-shifted curve in an inhibitor-concentration-dependent manner.
The RT-qPCR experiment normalizes treatment groups to a negative control, DMSO, to generate a xenobiotic-induced fold induction. The value for the DMSO group should always equal 1. After acquiring the gene fold induction for each treatment, a statistical analysis needs to be performed in order to capture any significant change. Because the data include triplicate values for a control and more than one treatment, also in triplicate, the statistical comparison tool that should be used is one-way analysis of variance (ANOVA) with post-hoc Dunnett's analysis. The easiest way to perform this analysis is to input the data into GraphPad Prism software and utilize the correct functions. Figure 12 gives an example of what these data might look like for a test of six compounds, including compound A. RIF is the positive control of induction for CYP3A4 through PXR modulation, and compounds A, 3, 4, and 5 statistically significantly induce CYP3A4 activity. Once a compound is identified as statistically different from the negative control, it is defined as an actual inducer of the nuclear receptor being tested. In this example, four of the six compounds tested appear to be PXR activators, through the induction of CYP3A4. However, other nuclear receptors also modulate CYP3A4, which suggests that more studies need to be conducted, potentially using PXR knockout cells, to confirm their true activities.
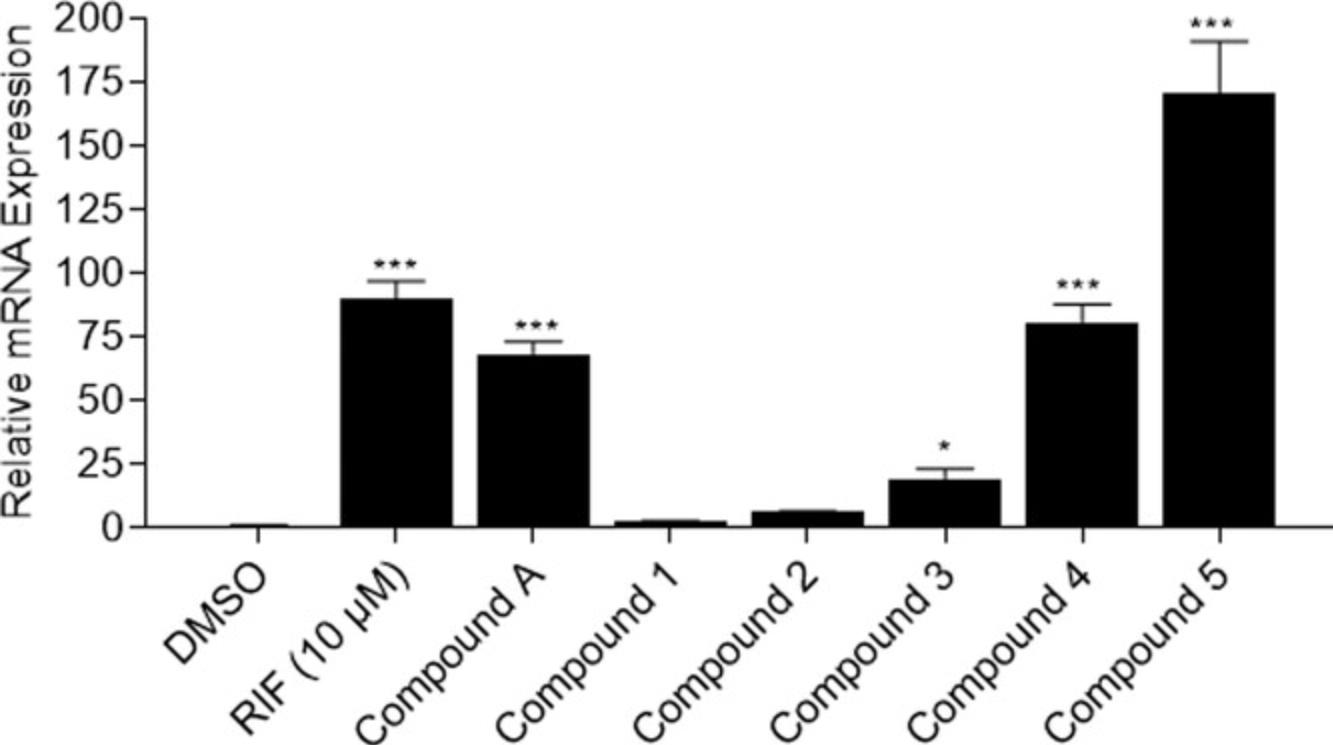
Time Considerations
Depending on the assay conditions in a protocol, it could take anywhere from a few weeks to a few months to optimize this assay. When thawing frozen cells, we suggest allowing at least one cell passage before using them in an assay. For example, thaw cells on Monday, passage cells on Thursday, and the cells will be ready for use on the following Monday. Each time you perform the nuclear receptor luciferase reporter gene assay, it should take about the same amount of time. After the cells are plated and allowed to attach to the bottom of the assay well (3-5 hr), the cells are treated for an optimal amount of time; we suggest between 15 and 24 hr depending on the type of assay. Adding detection reagents, incubating the assay plate, and reading the plates generally takes ∼2 hr. Therefore, altogether, one complete assay will take between 20 and 31 hr. Once the reagents, treatment timing, cells, and machine settings are optimized, a larger screen can take place. The timing for this screen will depend on how many compounds and plates you are screening at once, as well as the machinery available. If no robot is available, we suggest working with ∼10-15 plates at a time due to hands-on restrictions. Using more than 15 plates at a time may get confusing and lead to timing issues as well as an increased chance of human error. For the pharmacological study in Basic Protocol 3, the time expectance is the same (between 20 and 31 hr) as for the initial optimization assay because it is a repeat experiment and adding an inhibitor adds no extra time.
Regarding the RT-qPCR assay, there are various steps which take varying times depending on experience; there are also multiple points at which the experiment can be paused and put in the −80°C or −20°C freezer. Therefore, the assay can take 1 day from harvest to PCR analysis, or it can take however long is necessary given time constraints. Depending on the cell type used, plating of the cells and the time before treatment vary. In this specific protocol, using HepaRG cells, the time from plating to harvesting is 4 days because there is a 24-hr treatment. Once harvested, the lysates can be kept at −80°C until ready to use. The next step is to isolate the RNA, using a kit or TRIzol™, which will take ∼2-4 hr depending on the method and number of samples generated. After the RNA is extracted, the protocol can once again be paused, if desired, and samples kept at −80°C before proceeding. Measuring the RNA concentration and performing reverse transcriptase takes ∼2 hr total, and once again the samples can be stored in a −20°C before proceeding with the next step. Setting up and performing the qRT-PCR will take at least 2 hr depending on the number of samples and genes being measured, the experience of the scientist, and the type of master mix used. With an experienced scientist, one 384-well plate can be fully filled and completed in ∼2.5 hr if using the regular Taqman master mix. If using the fast Taqman master mix, the PCR setup and assay can be completed in ∼1 hr 45 min. Therefore, to complete a qRT-PCR assay once the samples are harvested will take at least 6 hr if there are only a small number of samples and genes to measure. However, depending on whether the researcher stopped at any of various different steps, the assay can be completed weeks or even months later.
Acknowledgments
This work was supported in part by the Intramural Research Program of the National Center for Advancing Translational Sciences (NCATS), National Institutes of Health. The views expressed in this article are those of the authors and do not necessarily reflect the statements, opinions, views, conclusions, or policies of the NIH. Mention of trade names or commercial products does not constitute endorsement or recommendation for use.
Author Contributions
Caitlin Lynch : Data curation, investigation, methodology, validation, writing—original draft, writing—review and editing**; Srilatha Sakamuru** : Data curation, methodology, writing—review and editing**; Menghang Xia** : Conceptualization, investigation, methodology, supervision, writing—review and editing.
Conflict of Interest
The authors report no conflict of interest. All authors have read and approved the final manuscript.
Open Research
Data Availability Statement
The data, tools, and material (or their source) that support Basic Protocol 1 and 3 are available from the corresponding author upon reasonable request. The data that support Basic Protocol 2 are openly available in the Tox21 Gateway at https://tripod.nih.gov//tox21/pubdata/, reference assay tox21-pxr-p1.
Literature Cited
- Alwine, J. C., Kemp, D. J., & Stark, G. R. (1977). Method for detection of specific RNAs in agarose gels by transfer to diazobenzyloxymethyl-paper and hybridization with DNA probes. Proceedings of the National Academy of Sciences of the United States of America , 74(12), 5350–5354. doi: 10.1073/pnas.74.12.5350
- Attene-Ramos, M. S., Miller, N., Huang, R., Michael, S., Itkin, M., Kavlock, R. J., … Xia, M. (2013). The Tox21 robotic platform for the assessment of environmental chemicals—from vision to reality. Drug Discovery Today , 18(15-16), 716–723. doi: 10.1016/j.drudis.2013.05.015
- Bustin, S. A. (2000). Absolute quantification of mRNA using real-time reverse transcription polymerase chain reaction assays. Journal of Molecular Endocrinology , 25(2), 169–193. doi: 10.1677/jme.0.0250169
- F. J. Hock (Ed.). (2016). Drug Discovery and Evaluation: Pharmacological Assays ( 4 ed.). Cham, Switzerland: Springer.
- Huang, R. (2016). A quantitative high-throughput screening data analysis pipeline for activity profiling. Methods in Molecular Biology , 1473, 111–122. doi: 10.1007/978-1-4939-6346-1_12
- Hsu, C.-W., Huang, R., Attene Ramos, M., Austin, C., Simeonov, A., & Xia, M. (2016). Advances in high-throughput screening technology for toxicology. International Journal of Risk Assessment and Management , 20(1-3), 109–135. doi: 10.1504/IJRAM.2017.082562
- Kliewer, S. A., Moore, J. T., Wade, L., Staudinger, J. L., Watson, M. A., Jones, S. A., … Lehmann, J. M. (1998). An orphan nuclear receptor activated by pregnanes defines a novel steroid signaling pathway. Cell , 92(1), 73–82. doi: 10.1016/s0092-8674(00)80900-9
- Kumar, G. N., & Surapaneni, S. (2001). Role of drug metabolism in drug discovery and development. Medicinal Research Reviews , 21(5), 397–411. doi: 10.1002/med.1016
- Lin, W., Wang, Y. M., Chai, S. C., Lv, L., Zheng, J., Wu, J., … Chen, T. (2017). SPA70 is a potent antagonist of human pregnane X receptor. Nature Communications , 8(1), 741. doi: 10.1038/s41467-017-00780-5
- Lin, W., Wu, J., Dong, H., Bouck, D., Zeng, F. Y., & Chen, T. (2008). Cyclin-dependent kinase 2 negatively regulates human pregnane X receptor-mediated CYP3A4 gene expression in HepG2 liver carcinoma cells. Journal of Biological Chemistry , 283(45), 30650–30657. doi: 10.1074/jbc.M806132200
- Livak, K. J., & Schmittgen, T. D. (2001). Analysis of relative gene expression data using real-time quantitative PCR and the 2(-Delta Delta C(T)) method. Methods (San Diego, Calif.) , 25(4), 402–408. doi: 10.1006/meth.2001.1262
- Lynch, C., Mackowiak, B., Huang, R., Li, L., Heyward, S., Sakamuru, S., … Xia, M. (2019). Identification of modulators that activate the constitutive androstane receptor from the Tox21 10K compound library. Toxicological Sciences , 167(1), 282–292. doi: 10.1093/toxsci/kfy242
- Lynch, C., Sakamuru, S., Huang, R., Niebler, J., Ferguson, S. S., & Xia, M. (2021). Characterization of human pregnane X receptor activators identified from a screening of the Tox21 compound library. Biochemical Pharmacology , 184, 114368. doi: 10.1016/j.bcp.2020.114368
- Lynch, C., Sakamuru, S., Huang, R., Stavreva, D. A., Varticovski, L., Hager, G. L., … Xia, M. (2017). Identifying environmental chemicals as agonists of the androgen receptor by using a quantitative high-throughput screening platform. Toxicology , 385, 48–58. doi: 10.1016/j.tox.2017.05.001
- Lynch, C., Zhao, J., Sakamuru, S., Zhang, L., Huang, R., Witt, K. L., … Xia, M. (2019). Identification of compounds that inhibit estrogen-related receptor alpha signaling using high-throughput screening assays. Molecules (Basel, Switzerland) , 24(5), 841. doi: 10.3390/molecules24050841
- Schulman, I. G. (2010). Nuclear receptors as drug targets for metabolic disease. Advanced Drug Delivery Reviews , 62(13), 1307–1315. doi: 10.1016/j.addr.2010.07.002
- Sueyoshi, T., & Negishi, M. (2001). Phenobarbital response elements of cytochrome P450 genes and nuclear receptors. Annual Review of Pharmacology and Toxicology , 41, 123–143. doi: 10.1146/annurev.pharmtox.41.1.123