Role of High-Throughput Electrophysiology in Drug Discovery
Chang Liu, Chang Liu, Tianbo Li, Tianbo Li, Jun Chen, Jun Chen
Abstract
Due to their important physiological functions, ion channels are key therapeutic targets for a variety of disorders. However, electrophysiological assessment of ion channel activity is technically challenging and has been a bottleneck in the discovery of drugs that modulate channel function. To address this issue, automated patch clamp platforms have been developed with improved throughput and broader applications. An overview of the current status of high-throughput electrophysiology and its applications in drug discovery is provided. © 2019 The Authors.
INTRODUCTION
Ion channels are macromolecular complexes that control ion flow across the plasma membrane and membranes of intracellular organelles (Hille, 2001). The opening and closing of the ion-conducting channel pore can be regulated by voltage, temperature, osmolarity, mechanical force, and/or the binding of chemical ligands. The electrical signals mediated by ion channel activity are essential for diverse physiological functions ranging from cognition, sensation, muscle contraction, and volume regulation to cellular signaling. Inherited dysfunction of ion channels can cause a variety of diseases (i.e., channelopathies), such as epilepsy, cardiac arrhythmia, and myotonia (Ptacek, 2015; Ryan & Ptacek, 2010). Many ion channels have been pursued as key therapeutic targets (Wickenden, Priest, & Erdemli, 2012). Indeed, ion channels represent the second largest class of targets, behind G-protein-coupled receptors, for drugs approved by the US Food and Drug Administration (Overington, Al-Lazikani, & Hopkins, 2006). Unintended drug effects on ion channels cause significant toxicity risks that can be life threatening. For example, unintended block of hERG K+ channels can result in QT prolongation, ventricular arrhythmia, and sudden cardiac death. In recent years, high-throughput electrophysiology has emerged to meet the increasing demand to assess ion channel function. In this article, we provide an overview of the current status of high-throughput electrophysiology, practical considerations, and applications.
Manual Patch Clamp
The biophysical properties of ion channel function is routinely studied by manual patch clamp, an electrophysiological procedure developed in the 1970s by Neher and Sakmann (1976) who were later awarded a Nobel prize for this revolutionary invention. A typical manual patch clamp experiment begins with the fabrication of fine-tipped glass microelectrodes as recording pipettes. The pipette tip is placed onto the surface of a cell (Fig. 1A), and when gentle back-suction is applied, a tight electrical seal with a resistance of several gigaohms (GΩ) is achieved between the pipette tip and the cell membrane. This tight seal is critical for lowering background noise recording of bioelectrical currents across the membrane. To gain electrical access to the interior of the cell, the small piece of membrane within the pipette tip is ruptured by brief negative pressure or an electric pulse, or the membrane is perforated by including a pore-forming antibiotic (e.g., nystatin) in the recording pipette. The resulting “whole-cell” configuration allows precise control of transmembrane voltage (i.e., voltage clamp) and the recording of currents across the entire plasma membrane in response to stepped change in transmembrane voltage in accordance with the electrochemical gradient of the permeant ion(s). In addition to the whole-cell configuration of voltage clamp, single-channel currents can be recorded from a membrane patch in inside-out or outside-out configurations. Although patch clamp is considered the gold standard for high-fidelity analysis of ion channel function, it requires considerable training and is notoriously labor intensive. A highly skilled electrophysiologist can usually generate 15 to 30 data points per day, which is a very low-throughput rate considering that a typical chemical library consists of millions of compounds.
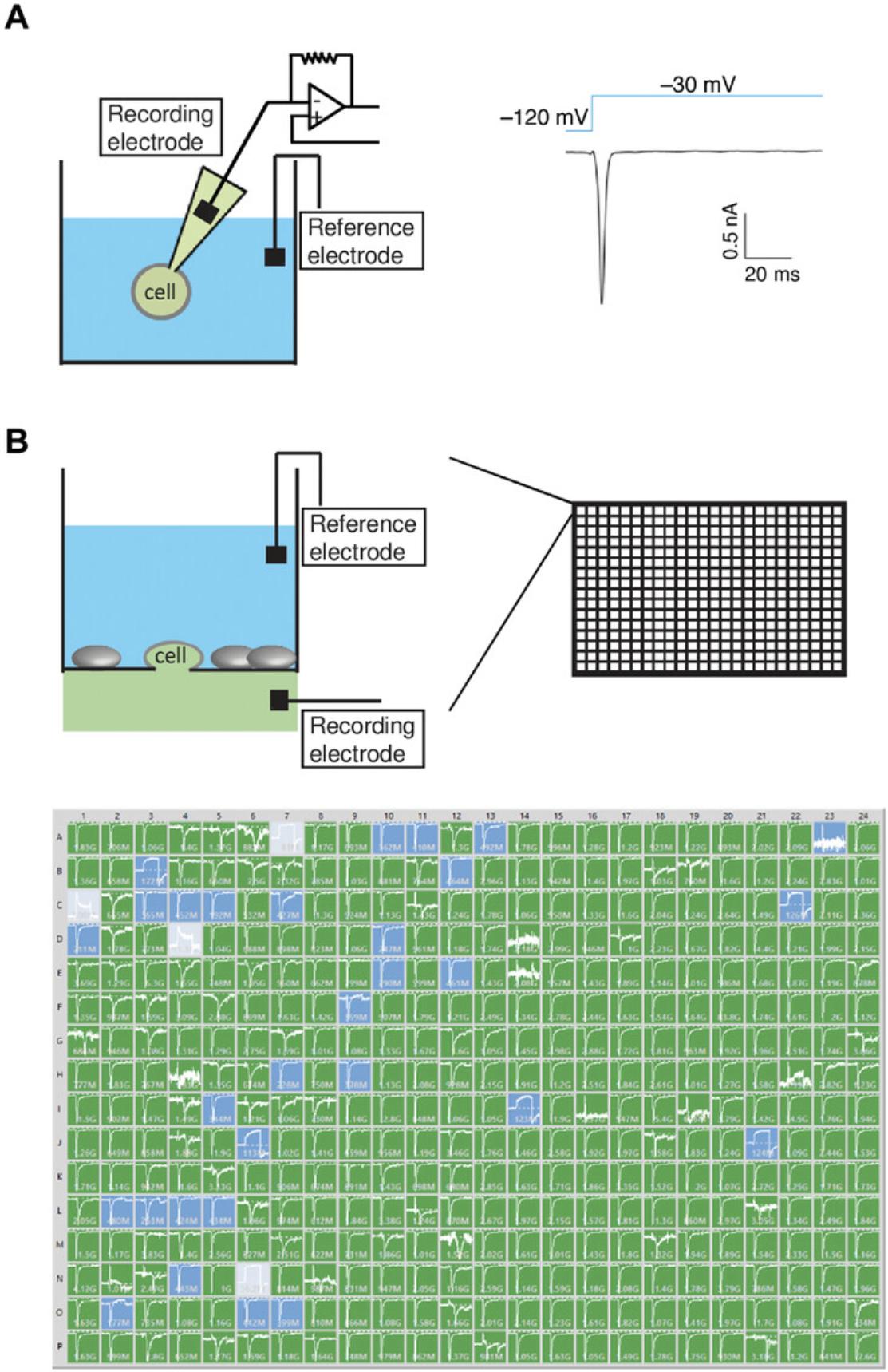
Automated Electrophysiology Platforms
Given the low throughput and technical challenges of manual patch clamp, there has been considerable effort to automate the technique. Several automated patch clamp platforms have been invented since the 1990s (Dunlop, Bowlby, Peri, Vasilyev, & Arias, 2008). In general, these platforms can utilize either glass pipettes or planar arrays.
Pipette-based automated patch clamp platforms are based on the same physical principles as manual patch clamp but automate the processes of pipette handling, tight seal formation, achievement of whole-cell recording configuration, and compound application. There are major differences in cell catching and GΩ seal formation among pipette-based platforms. For examples, semi-automated Apatchi-1 (Asmild et al., 2003) and fully automated Robopatch (Vasilyev, Merrill, Iwanow, Dunlop, & Bowlby, 2006) employ robots to mimic the conventional patch clamp procedure. Cells are randomly encountered by the recording pipettes and are patched in the solution or air/liquid interface. In contrast, for Flyscreen the cell suspension is flushed to the inside of a glass pipette to achieve GΩ seals with a single cell at the tip of the pipette (Lepple-Wienhues, Ferlinz, Seeger, & Schafer, 2003). These glass pipette–based platforms reduce technical difficulties while maintaining a high quality of ionic current recording. Several platforms enable parallel recordings of multiple cells. For example, the Patchserver system (Multichannel Systems Inc., Germany) is capable of recording up to four cells simultaneously. As these systems only moderately increase throughput compared with manual patch clamp, they are inadequate for conducting large-scale drug screens.
Planar array–based automated patch clamp platforms include multiple commercially available instruments (Dunlop et al., 2008). Instead of recording currents using glass pipettes, planar array–based platforms utilize a planar chip containing multiple micropores that capture cells dispensed onto the chips (Fig. 1B). Suspended cells are drawn towards the micropores by applying negative pressure to facilitate formation of an electrical seal. The pore-delineated membrane is disrupted to form the whole-cell recording configuration by applying further negative pressure or by exposing the membrane to a perforating reagent. Because of the parallel recordings from multiple wells in the same plate/chip, planar array–based automated patch clamp platforms usually allow for much higher throughput. IonWorks HT is the first system (Molecular Devices) allowing data collection from a 384-well plate format (Schroeder, Neagle, Trezise, & Worley, 2003). Later models such as IonWorks Quattro and Barracuda adopted a perforated patch clamp approach that increases recording stability. While all of these platforms increase throughput rates, because of suboptimal seal resistance (<200 MΩ), their data quality is below that obtained using the conventional patch clamp approach.
Several other planar array automated patch clamp formats enable formation of GΩ seals and a corresponding enhancement in recording quality. The throughput rates of these devices range from low (Port-a-Patch, Nanion Technologies) to medium (PatchXpress, Molecular Devices; Patchliner, Nanion Technologies; QPatch, Sophion Bioscience) to high (Syncropatch 384/768PE, Nanion Technologies; Qube, Sophion Bioscience) as shown in Table 1 (Bell & Dallas, 2018; Li & Chen, 2018). The GΩ seal–based recordings of these devices are made possible by using precisely controlled negative pressure and by using fluoride ions in the internal solution. For platforms such as Patchliner and Syncropatch, a “seal enhancer” solution containing a high concentration of Ca2+ (10 to 40 mM) is added to boost GΩ seal formation and is subsequently washed out before commencing current recordings. Port-a-Patch, Patchliner, QPatch, and Qube adopt microfluidic designs that allow for relatively rapid solution switching (e.g., <50 ms). This feature is important for fast-desensitizing ligand-gated ion channels such as the α7 nicotinic acetylcholine receptor (Dunlop et al., 2007; Friis, Mathes, Sunesen, Bowlby, & Dunlop, 2009; see Current Protocols article: Obergrussberger et al., 2014). The microfluidic design also enables accurate control and testing of multiple compound concentrations on a single cell. The Syncropatch platform uses accessory instruments (e.g., Biomek, Beckman Coulter Life Sciences) for liquid handling and pipettes to add and aspirate solutions.
Feature | Port-a-Patch (Nanion) | Patchliner (Nanion) | PatchXpress (Molecular Devices) | QPatch (Sophion) | Qube (Sophion) | Syncropatch 384/768PE (Nanion) |
---|---|---|---|---|---|---|
Throughput/run | 1 | 16 | 16 | 48 | 384 | 384/768 |
Seal resistance (GΩ) | >1 | >1 | >1 | >1 | >1 | >1 |
Recording configuration | Single cell | Single cell | Single cell | Single cell and PPCb | Single cell and PPCb | Single cell and PPCb |
Solution switch time | <150 ms | <50 ms | <50 ms | 80 ms | 50 ms | 50 ms |
Wash-off | Yes | Yes | Incompletec | Yes | Yes | Incompletec |
Multiple compound addition | Yes | Yes | Yes | Yes | Yes | Yes |
Internal perfusion | Yes | Yes | No | No | Yes | Yes |
Temperature control | Yes | Yes | No | Yes | Yes | Yes |
Current clamp | Yes | Yes | No | Yes | Yes | Yes |
Technical reference | (Bruggemann et al., 2003) | (Bruggemann, Stoelzle, George, Behrends, & Fertig, 2006) | (Tao et al., 2004) | (Asmild et al., 2003) | (Chambers, Witton, Adams, Marrington, & Kammonen, 2016) | (Obergrussberger et al., 2016) |
- a Some platforms may not be commercially available; please contact vendors for details.
- b PPC (population patch clamp) is a recording of ensemble current from 2 to 36 cells.
- c Solution exchange is not complete due to lack of flow-through design.
In addition to their higher throughput, the planar array–based automated patch clamp platforms offer several capabilities that are difficult to achieve using manual electrophysiology recording techniques. These include:
- 1.Population patch clamp recording. Under this configuration, a single well contains multiple micropores so that currents from a population of cells can be recorded at once. The number of cells recorded in each well can be customized in some platforms (20 cells for Ionflux; 4 or 8 cells for Syncropatch; and 2, 6, 10, 16, and 36 cells for Qube). Population patch clamp significantly improves the success rate and data consistency across the chip plate (Dale, Townsend, Hollands, & Trezise, 2007; Finkel et al., 2006). However, resolution and recording stability can be compromised using this approach.
- 2.Internal solution exchange. Changing the internal solution is difficult with manual patch clamp as it requires exchange of the internal solution contained within the small volume and access to the restricted space of the recording electrode. In contrast, for planar array–based platforms, the internal solution is more readily perfused and exchanged. For example, the effect of intracellular pH on the TREK-1 channel has been analyzed using Syncropatch (Sauter, Sorensen, Rapedius, Bruggemann, & Novak, 2016).
- 3.Test of rare samples. Automated platforms usually require only a small solution volume (e.g., 50 µl), as opposed to a few milliliters needed when conducting manual electrophysiology experiments. This feature facilitates analysis of samples that are available in only limited quantities, such as peptide toxins.
- 4.Temperature control. Most automated patch clamp platforms offer the option of temperature control, a useful feature for compound screening at physiological temperature and for the study of temperature-sensitive ion channels (Papakosta et al., 2011).
PRACTICAL CONSIDERATIONS
It is no small undertaking to implement automated electrophysiology platforms. Beside the initial investment on instruments, consumable cost can be substantial and should be taken into consideration. To run experiments successfully, hardware settings, voltage protocols, solution protocols, membrane conditions, and target expression levels should be optimized. Often a significant amount of effort is spent on optimizing conditions and troubleshooting. Additionally, results from automated patch clamp experiments should be corroborated by different platforms and conventional electrophysiology. Hence, automated patch clamp does require technical expertise and highly trained personnel. Due to these considerations, many companies opt to rely on contract research organizations for their electrophysiology needs. In the following section, we focus on the technical aspects of automated patch clamp experiments.
Cell Lines and Expression System
Because a typical automated patch clamp experiment requires tens of millions of cells, stable cell lines expressing the ion channel of interest are most commonly used. Factors often taken into consideration are host cell background, existence of auxiliary subunits, basal current contamination (by constitutively expressed ion channels), current density, and homogeneity. Several rounds of clone selection are often needed to develop a high-quality stable cell line, with automated electrophysiology being particularly useful for large-scale testing. For example, by using Syncropatch, a 384-well formatted automated patch clamp system, it is possible to simultaneously test 24 clones, with 16 cells per clone. In addition to stable cell lines, transiently transfected cells have also been used for automated electrophysiology (Vanoye et al., 2018), although this can sometimes suffer from variable levels of channel/receptor expression. Theoretically, a baculoviral expression system may also lend itself for use in automated electrophysiology.
With conventional electrophysiology experiments, cells are examined visually, and the “best” cells are selected for study. In contrast, with automated electrophysiology experiments, cells are caught randomly from a large pool, making the use of uniform high-quality cells essential. In our experience, using a gentle cell dissociation protocol, removing cell debris, maintaining cells at lower temperature, and adjusting cell densities are effective ways to improve the overall condition of the cells (Li et al., 2017).
Quality Control
Quality control of automated patch clamp experiments should be implemented at multiple levels. First, a number of electrophysiology parameters should be optimized, including initial cell capture resistance (>10 MΩ), seal resistance (>500 MΩ), current amplitude (>200 pA), and recording stability by monitoring changes in cell capacitance (<2 fold) and series resistance (<1.7 fold; Li et al., 2017). This optimization is accomplished during the assay development stage by exploring parameters in hardware settings, vacuum pressure, voltage protocols, solution composition, and washing conditions. Another important quality control issue is current stability. Current runup (amplitude increase) or rundown (amplitude decrease) without compound modulation is a common problem that should be monitored. For example, 20% or more rundown can occur with voltage-gated sodium channels during a 10-min recording. While the causes of rundown can include seal deterioration, accumulation of inactivation, and channel turnover, such data must be accounted for during data analysis. Proper positive and negative controls and effects of compound vehicle should be included in the assay. Results should be reassessed if the potency of reference compounds deviates significantly from historical values.
Compound Concentration
Many automated patch clamp platforms use microfluidic designs to ensure a rapid solution switch. For example, Ionflux Mercury switches between pressurized solution streams to reduce solution exchange time. Patchliner, QPatch, and Qube allow rapid, low-volume replacement of solution in the recording compartments. Other planar array-based systems, such as Syncropatch 384/768PE and PatchXpress, directly add and remove compounds in the recording wells. The effects of different methods of solution application have not been investigated systematically. Additionally, the material for chips can potentially influence effective compound concentrations. For example, lipophilic compounds are known to adhere to the microfluidic surfaces and are reported to be absorbed by excess cells in the recording wells (Bridgland-Taylor et al., 2006). Compounds are more likely to adhere to chips made of polydimethylsiloxane (via lipophilic partitioning) than to glass-coated chips. For these and other reasons, compound potencies derived from automated electrophysiology experiments can be underestimated. Therefore, key findings should be corroborated by conventional electrophysiology experiments.
EXAMPLES OF AUTOMATED PATCH CLAMP APPLICATIONS
Ligand Finding
A major challenge in ion channel drug discovery is the identification of tractable lead compounds. This is often achieved through high-throughput screening and lead optimization. At the high-throughput screening stage, hundreds of thousands to millions of compounds are screened against the target of interest. For ion channels, high-throughput screening is usually conducted using radioligand binding or fluorescence-based assays. However, automated electrophysiology is playing an increasingly important role in validating the results from high-throughput screening assays, hits confirmation, lead optimization, and mechanistic studies (Fig. 2). In a recent study aimed at identifying Nav1.7-selective blockers, electrophysiology experiments using Syncropatch revealed that a conventional fluorescence-based membrane potential assay is biased to detect pore blockers only. Given this limitation, we designed an assay using a mutant Nav1.7 channel that retained sensitivity to voltage sensor domain 4 (VSD4) blockers while losing sensitivity to pore blockers (Chernov-Rogan et al., 2018). By combining the use of this mutant channel with its activator 1KαPMTX, a derivative of wasp venom toxin, we designed a membrane potential assay favoring the identification of compounds with non-pore-blocking mechanisms. Following high-throughput screening, we utilized high-throughput electrophysiology to test several thousands of the identified hits on various Nav isoforms to determine potency and subtype selectivity. Also, by testing compounds on channels containing targeted mutations in the pore, VSD4, and other domains, we gained information on drug binding sites and mechanisms of action.
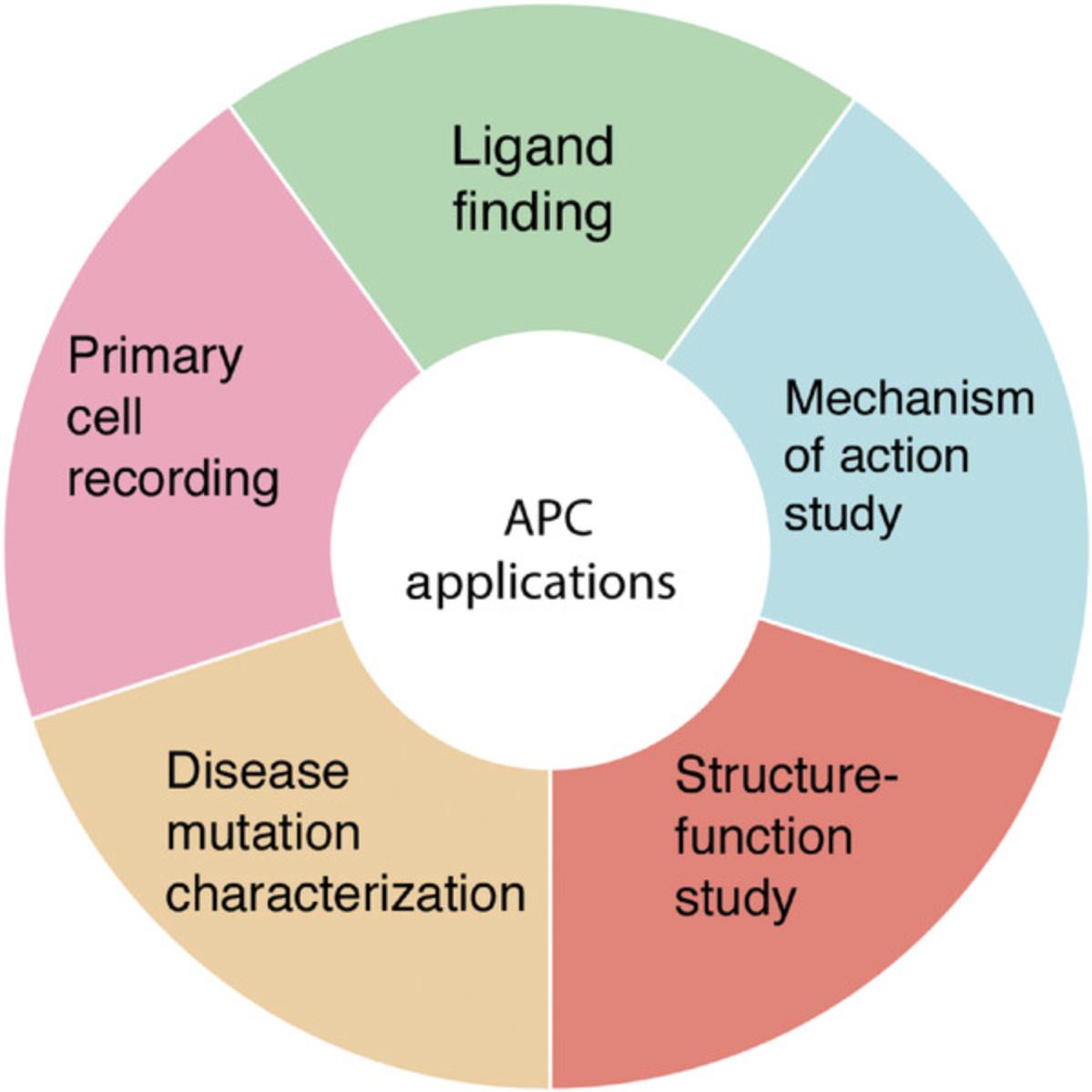
Mechanism of Action Study
Automated electrophysiology has proven useful for mechanism of action studies. For example, detailed kinetics properties of the N -methyl-D-aspartate receptor, a target for neurological diseases, were characterized in response to over 100 GluN2A-subtype-selective positive allosteric modulators (Volgraf et al., 2016). These positive allosteric modulators are thiazolopyrimidinone-containing compounds with prolonged deactivation kinetics as the primary means of positive modulation. Using IonFlux HT (Fluxion Biosciences), correlation between GluN2A potentiation and deactivation kinetics was examined. The study led to the classification of compounds based on different aromatic right-hand groups and the rational design of compounds.
Structure–Function Study
High-throughput electrophysiology has been applied to structure–function studies. We studied Nav1.7 inhibition by Protoxin-II (ProTx2), a 30-residue cysteine-knot peptide toxin isolated from the Peruvian green velvet tarantula (Xu et al., 2019). Structural studies revealed that ProTx2 forms a complex with VSD2 in both activated and deactivated states of the channel. To interrogate the ProTx2 receptor site, we tested over 50 mutant channels and 18 toxin variants using Syncropatch. The results revealed that ProTx2 regulates Nav1.7 via an electrostatic interaction and that this mechanism is conserved for other related toxins, thereby providing a template and rationale for the design of a new class of Nav1.7-selective compounds.
Disease Mutation Characterization
Mutations in ion channels underline many human disorders (Ptacek, 2015). Advances in genetic testing have led to an increase in the number of identified ion channel gene variants. Determining the potential pathogenicity of these variants is now possible with the advent of high-throughput electrophysiology. Syncropatch was used to characterize 30 known KCNQ1 variants and demonstrated strong concordance between Syncropatch and historical data (Vanoye et al., 2018). Next, 48 new variants were characterized over a period of 12 weeks, with ∼70% of the variants classified on the basis of functional data. All variants were transiently expressed by electroporation. Hence the combination of transient transfection and high-throughput electrophysiology enables high-throughput characterization of disease-associated variants.
Recordings from Primary Cells
Due to their fragile surface membrane and irregular shapes, manual electrophysiology recordings from primary cells can be challenging. Nevertheless, progress has been made using automated patch clamp platforms for primary cell studies. Patchliner was applied successfully to record primary cells derived from blood vessels, arthritic joints, and the immune and nervous systems (Milligan et al., 2009). Patchliner has also been used to evaluate human cardiomyocytes derived from pluripotent stem cells (Becker et al., 2013; Rajamohan et al., 2016). In an effort to record rat primary T lymphocytes, we tested various parameters on Syncropatch and optimized the conditions to produce the best results (two 1000-ms suction pulses of 200 mBar and 10-MΩ high-resistance chip). Under these conditions, it was possible to obtain successful recordings on 10% of the primary T cells (Li et al., 2017).
OUTLOOK
The past 15 years have witnessed tremendous progress and evolution in automated patch clamp platforms. These technologies have been successfully integrated into ion channel drug discovery programs, from ligand discovery to lead optimization and mechanistic studies. It is estimated that most ion channel clinical candidates discovered in recent years have been characterized by automated patch clamp. In addition, automated patch clamp has been extensively applied to drug safety testing against hERG, Nav1.5, and other ion channels, resulting in safer drugs. Despite this progress, the current automated patch clamp platforms do not offer adequate throughput to be used as primary tools for high-throughput screening, and cost of instruments and consumables remains high. Compared to manual patch clamp, automated patch clamp has minimized technical challenges but still requires highly trained personnel. Quality control and the analysis of high volumes of data remain challenging. Hence, continued improvement in these areas is expected. Advances in automated patch clamp technologies, along with new technologies such as optical electrophysiology, will facilitate future efforts aimed at the discovery of ion channel modulators.
LITERATURE CITED
- Asmild, M., Oswald, N., Krzywkowski, K. M., Friis, S., Jacobsen, R. B., Reuter, D., … Willumsen, N. J. (2003). Upscaling and automation of electrophysiology: Toward high throughput screening in ion channel drug discovery. Receptors & Channels, 9(1), 49–58. doi: 10.3109/10606820308258.
- Becker, N., Stoelzle, S., Gopel, S., Guinot, D., Mumm, P., Haarmann, C., … Bruggemann, A. (2013). Minimized cell usage for stem cell-derived and primary cells on an automated patch clamp system. Journal of Pharmacological and Toxicological Methods , 68(1), 82–87. doi: 10.1016/j.vascn.2013.03.009.
- Bell, D. C., & Dallas, M. L. (2018). Using automated patch clamp electrophysiology platforms in pain-related ion channel research: Insights from industry and academia. British Journal of Pharmacology , 175(12), 2312–2321. doi: 10.1111/bph.13916.
- Bridgland-Taylor, M. H., Hargreaves, A. C., Easter, A., Orme, A., Henthorn, D. C., Ding, M., … Pollard, C. E. (2006). Optimisation and validation of a medium-throughput electrophysiology-based hERG assay using IonWorks HT. Journal of Pharmacological and Toxicological Methods , 54(2), 189–199. doi: 10.1016/j.vascn.2006.02.003.
- Bruggemann, A., George, M., Klau, M., Beckler, M., Steindl, J., Behrends, J. C., & Fertig, N. (2003). High quality ion channel analysis on a chip with the NPC technology. Assay and Drug Development Technologies , 1(5), 665–673. doi: 10.1089/154065803770381020.
- Bruggemann, A., Stoelzle, S., George, M., Behrends, J. C., & Fertig, N. (2006). Microchip technology for automated and parallel patch-clamp recording. Small , 2(7), 840–846. doi: 10.1002/smll.200600083.
- Chambers, C., Witton, I., Adams, C., Marrington, L., & Kammonen, J. (2016). High-throughput screening of Nav1.7 modulators using a giga-seal automated patch clamp instrument. Assay and Drug Development Technologies , 14(2), 93–108. doi: 10.1089/adt.2016.700.
- Chernov-Rogan, T., Li, T., Lu, G., Verschoof, H., Khakh, K., Jones, S. W., … Chen, J. (2018). Mechanism-specific assay design facilitates the discovery of Nav1.7-selective inhibitors. Proceedings of the National Academy of Sciences of the United States of America , 115(4), E792–E801. doi: 10.1073/pnas.1713701115.
- Dale, T. J., Townsend, C., Hollands, E. C., & Trezise, D. J. (2007). Population patch clamp electrophysiology: A breakthrough technology for ion channel screening. Molecular BioSystems , 3(10), 714–722. doi: 10.1039/b706152h.
- Dunlop, J., Bowlby, M., Peri, R., Vasilyev, D., & Arias, R. (2008). High-throughput electrophysiology: An emerging paradigm for ion-channel screening and physiology. Nature Reviews Drug Discovery , 7(4), 358–368. doi: 10.1038/nrd2552.
- Dunlop, J., Roncarati, R., Jow, B., Bothmann, H., Lock, T., Kowal, D., … Terstappen, G. C. (2007). In vitro screening strategies for nicotinic receptor ligands. Biochemical Pharmacology , 74(8), 1172–1181. doi: 10.1016/j.bcp.2007.07.006.
- Finkel, A., Wittel, A., Yang, N., Handran, S., Hughes, J., & Costantin, J. (2006). Population patch clamp improves data consistency and success rates in the measurement of ionic currents. Journal of Biomolecular Screening , 11(5), 488–496. doi: 10.1177/1087057106288050.
- Friis, S., Mathes, C., Sunesen, M., Bowlby, M. R., & Dunlop, J. (2009). Characterization of compounds on nicotinic acetylcholine receptor alpha7 channels using higher throughput electrophysiology. Journal of Neuroscience Methods , 177(1), 142–148. doi: 10.1016/j.jneumeth.2008.10.007.
- Hille, B. (2001). Ion channels of excitable membranes ( 3rd ed.). Sunderland, MA: Sinauer Associates.
- Lepple-Wienhues, A., Ferlinz, K., Seeger, A., & Schafer, A. (2003). Flip the tip: An automated, high quality, cost-effective patch clamp screen. Receptors & Channels, 9(1), 13–17. doi: 10.3109/10606820308257.
- Li, T., & Chen, J. (2018). Voltage-gated sodium channel drug discovery technologies and challenges. In K. Fatima Shad (Ed.). Ion channels in health and sickness. London, UK: IntechOpen. doi: 10.5772/intechopen.80370.
- Li, T., Lu, G., Chiang, E. Y., Chernov-Rogan, T., Grogan, J. L., & Chen, J. (2017). High-throughput electrophysiological assays for voltage gated ion channels using SyncroPatch 768PE. PLoS One , 12(7), e0180154. doi: 10.1371/journal.pone.0180154.
- Milligan, C. J., Li, J., Sukumar, P., Majeed, Y., Dallas, M. L., English, A., … Beech, D. J. (2009). Robotic multiwell planar patch-clamp for native and primary mammalian cells. Nature Protocols , 4(2), 244–255. doi: 10.1038/nprot.2008.230.
- Neher, E., & Sakmann, B. (1976). Single-channel currents recorded from membrane of denervated frog muscle fibres. Nature , 260(5554), 799–802. doi: 10.1038/260799a0.
- Obergrussberger, A., Bruggemann, A., Goetze, T. A., Rapedius, M., Haarmann, C., Rinke, I., … Fertig, N. (2016). Automated patch clamp meets high-throughput screening: 384 cells recorded in parallel on a planar patch clamp module. Journal of Laboratory Automation , 21(6), 779–793. doi: 10.1177/2211068215623209.
- Obergrussberger, A., Haarmann, C., Rinke, I., Becker, N., Guinot, D., Brueggemann, A., … Fertig, N. (2014). Automated patch clamp analysis of nAChα7 and Nav1.7 channels. Current Protocols in Pharmacology , 65, 11.13.11–48. doi: 10.1002/0471141755.ph1113s65.
- Overington, J. P., Al-Lazikani, B., & Hopkins, A. L. (2006). How many drug targets are there? Nature Reviews Drug Discovery , 5(12), 993–996. doi: 10.1038/nrd2199.
- Papakosta, M., Dalle, C., Haythornthwaite, A., Cao, L., Stevens, E. B., Burgess, G., … Grimm, C. (2011). The chimeric approach reveals that differences in the TRPV1 pore domain determine species-specific sensitivity to block of heat activation. Journal of Biological Chemistry , 286(45), 39663–39672. doi: 10.1074/jbc.M111.273581.
- Ptacek, L. J. (2015). Episodic disorders: Channelopathies and beyond. Annual Review of Physiology , 77, 475–479. doi: 10.1146/annurev-physiol-021014-071922.
- Rajamohan, D., Kalra, S., Duc Hoang, M., George, V., Staniforth, A., Russell, H., … Denning, C. (2016). Automated electrophysiological and pharmacological evaluation of human pluripotent stem cell-derived cardiomyocytes. Stem Cells and Development , 25(6), 439–452. doi: 10.1089/scd.2015.0253.
- Ryan, D. P., & Ptacek, L. J. (2010). Episodic neurological channelopathies. Neuron , 68(2), 282–292. doi: 10.1016/j.neuron.2010.10.008.
- Sauter, D. R., Sorensen, C. E., Rapedius, M., Bruggemann, A., & Novak, I. (2016). pH-sensitive K+ channel TREK-1 is a novel target in pancreatic cancer. Biochimica et Biophysica Acta , 1862(10), 1994–2003. doi: 10.1016/j.bbadis.2016.07.009.
- Schroeder, K., Neagle, B., Trezise, D. J., & Worley, J. (2003). Ionworks HT: A new high-throughput electrophysiology measurement platform. Journal of Biomolecular Screening , 8(1), 50–64. doi: 10.1177/1087057102239667.
- Tao, H., Santa Ana, D., Guia, A., Huang, M., Ligutti, J., Walker, G., … Ghetti, A. (2004). Automated tight seal electrophysiology for assessing the potential hERG liability of pharmaceutical compounds. Assay and Drug Development Technologies , 2(5), 497–506. doi: 10.1089/adt.2004.2.497.
- Vanoye, C. G., Desai, R. R., Fabre, K. L., Gallagher, S. L., Potet, F., DeKeyser, J. M., … George, A. L., Jr. (2018). High-throughput functional evaluation of KCNQ1 decrypts variants of unknown significance. Circulation: Genomic and Precision Medicine , 11(11), e002345. doi: 10.1161/CIRCGEN.118.002345.
- Vasilyev, D., Merrill, T., Iwanow, A., Dunlop, J., & Bowlby, M. (2006). A novel method for patch-clamp automation. Pflugers Archiv: European Journal of Physiology , 452(2), 240–247. doi: 10.1007/s00424-005-0029-2.
- Volgraf, M., Sellers, B. D., Jiang, Y., Wu, G., Ly, C. Q., Villemure, E., … Schwarz, J. B. (2016). Discovery of GluN2A-selective NMDA receptor positive allosteric modulators (PAMs): Tuning deactivation kinetics via structure-based design. Journal of Medicinal Chemistry , 59(6), 2760–2779. doi: 10.1021/acs.jmedchem.5b02010.
- Wickenden, A., Priest, B., & Erdemli, G. (2012). Ion channel drug discovery: Challenges and future directions. Future Medicinal Chemistry , 4(5), 661–679. doi: 10.4155/fmc.12.4.
- Xu, H., Li, T., Rohou, A., Arthur, C. P., Tzakoniati, F., Wong, E., … Payandeh, J. (2019). Structural basis of Nav1.7 inhibition by a gating-modifier spider toxin. Cell , 176(5), 1238–1239. doi: 10.1016/j.cell.2019.01.047.
Citing Literature
Number of times cited according to CrossRef: 21
- Shuo Wang, Stephan Nussberger, Tracking the Activity and Position of Mitochondrial β-Barrel Proteins, Transmembrane β-Barrel Proteins, 10.1007/978-1-0716-3734-0_14, (221-236), (2024).
- Joanne G. Ma, Jamie I. Vandenberg, Chai-Ann Ng, Development of automated patch clamp assays to overcome the burden of variants of uncertain significance in inheritable arrhythmia syndromes, Frontiers in Physiology, 10.3389/fphys.2023.1294741, 14 , (2023).
- Mark L Dallas, Damian Bell, Advances in ion channel high throughput screening: where are we in 2023?, Expert Opinion on Drug Discovery, 10.1080/17460441.2023.2294948, 19 , 3, (331-337), (2023).
- Sandra Raposo-Garcia, Celia Costas, M. Carmen Louzao, Mercedes R. Vieytes, Carmen Vale, Luis M. Botana, Synergistic Effect of Brevetoxin BTX-3 and Ciguatoxin CTX3C in Human Voltage-Gated Na v 1.6 Sodium Channels , Chemical Research in Toxicology, 10.1021/acs.chemrestox.3c00267, 36 , 12, (1990-2000), (2023).
- Riley E. Perszyk, Mighten C. Yip, Andrew Jenkins, Stephen F. Traynelis, Craig R. Forest, Robotic cell electrophysiological characterization for drug discovery, Robotics for Cell Manipulation and Characterization, 10.1016/B978-0-323-95213-2.00013-2, (203-221), (2023).
- Igal Sterin, Ana C. Santos, Sungjin Park, Neuronal Activity Reporters as Drug Screening Platforms, Micromachines, 10.3390/mi13091500, 13 , 9, (1500), (2022).
- Monique J. Windley, Jessica Farr, Clifford TeBay, Jamie I. Vandenberg, Adam P. Hill, High throughput measurement of hERG drug block kinetics using the CiPA dynamic protocol, Journal of Pharmacological and Toxicological Methods, 10.1016/j.vascn.2022.107192, 117 , (107192), (2022).
- Tianbo Li, Martin Ginkel, Ada X. Yee, Leigh Foster, Jun Chen, Stephan Heyse, Stephan Steigele, An efficient and scalable data analysis solution for automated electrophysiology platforms, SLAS Discovery, 10.1016/j.slasd.2021.11.001, 27 , 4, (278-285), (2022).
- Shivani Choudhary, Sudhanva S. Kashyap, Richard J. Martin, Alan P. Robertson, Advances in our understanding of nematode ion channels as potential anthelmintic targets, International Journal for Parasitology: Drugs and Drug Resistance, 10.1016/j.ijpddr.2021.12.001, 18 , (52-86), (2022).
- Donald Wlodkowic, Marcus Jansen, High-throughput screening paradigms in ecotoxicity testing: Emerging prospects and ongoing challenges, Chemosphere, 10.1016/j.chemosphere.2022.135929, 307 , (135929), (2022).
- Damian C. Bell, Mark L. Dallas, Advancing Ion Channel Research with Automated Patch Clamp (APC) Electrophysiology Platforms, Ion Channels in Biophysics and Physiology, 10.1007/978-981-16-4254-8_2, (21-32), (2022).
- David W. Herr, The Future of Neurotoxicology: A Neuroelectrophysiological Viewpoint, Frontiers in Toxicology, 10.3389/ftox.2021.729788, 3 , (2021).
- Marcin Szczot, Alec R. Nickolls, Ruby M. Lam, Alexander T. Chesler, The Form and Function of PIEZO2, Annual Review of Biochemistry, 10.1146/annurev-biochem-081720-023244, 90 , 1, (507-534), (2021).
- Riley E. Perszyk, Mighten C. Yip, Ona L. McConnell, Eric T. Wang, Andrew Jenkins, Stephen F. Traynelis, Craig R. Forest, Automated Intracellular Pharmacological Electrophysiology for Ligand-Gated Ionotropic Receptor and Pharmacology Screening, Molecular Pharmacology, 10.1124/molpharm.120.000195, 100 , 1, (73-82), (2021).
- Alison Obergrussberger, Ilka Rinke‐Weiß, Tom A. Goetze, Markus Rapedius, Nina Brinkwirth, Nadine Becker, Maria Giustina Rotordam, Laura Hutchison, Paola Madau, Davide Pau, David Dalrymple, Nina Braun, Søren Friis, Stephan A. Pless, Niels Fertig, The suitability of high throughput automated patch clamp for physiological applications, The Journal of Physiology, 10.1113/JP282107, 600 , 2, (277-297), (2021).
- Matteo Borgini, Pravat Mondal, Ruiting Liu, Peter Wipf, Chemical modulation of Kv7 potassium channels, RSC Medicinal Chemistry, 10.1039/D0MD00328J, 12 , 4, (483-537), (2021).
- Damian C. Bell, Bernard Fermini, Use of automated patch clamp in cardiac safety assessment: Past, present & future perspectives, Journal of Pharmacological and Toxicological Methods, 10.1016/j.vascn.2021.107114, 111 , (107114), (2021).
- Damian C. Bell, Bernard Fermini, Use of automated patch clamp in cardiac safety assessment: past, present and future perspectives, Journal of Pharmacological and Toxicological Methods, 10.1016/j.vascn.2021.107072, 110 , (107072), (2021).
- Carlos G. Vanoye, Christopher H. Thompson, Reshma R. Desai, Jean-Marc DeKeyser, Liqi Chen, Laura J. Rasmussen-Torvik, Leah J. Welty, Alfred L. George, Functional evaluation of human ion channel variants using automated electrophysiology, Ion Channels: Channel Chemical Biology, Engineering, and Physiological Function, 10.1016/bs.mie.2021.02.011, (383-405), (2021).
- Anke Sambeth, Electrophysiology: From Molecule to Cognition, from Animal to Human, Modern CNS Drug Discovery, 10.1007/978-3-030-62351-7_9, (131-147), (2021).
- Janna Bednenko, Paul Colussi, Sunyia Hussain, Yihui Zhang, Theodore Clark, Therapeutic Antibodies Targeting Potassium Ion Channels, Pharmacology of Potassium Channels, 10.1007/164_2021_464, (507-545), (2021).