RNA Extraction from Plant Tissue with Homemade Acid Guanidinium Thiocyanate Phenol Chloroform (AGPC)
Baltasar Zepeda, Baltasar Zepeda, Julian C. Verdonk, Julian C. Verdonk
AGPC
home-made buffers
molecular biology protocol
multiple plant species and tissues
nucleic acids
RNA isolation
Abstract
Gene expression studies are a powerful technique to study biological processes, and isolating RNA that is pure, intact, and in sufficient amounts for downstream applications is key. Over the years, the field has moved to the use of commercial kits and ready-made extraction buffers for RNA isolation. This became particularly problematic during the COVID-19 crisis when supply chains were affected and when RNA extraction and analysis reagents were suddenly scarce at a time when they were particularly required. Acid guanidinium thiocyanate-phenol-chloroform (AGPC) is one of the oldest RNA extraction solutions, in use since 1987. It is known as a ready-made solution, sold under different brand names, and is typically the most expensive reagent in the RNA extraction process. In this article, we describe how to prepare a low-cost homemade AGPC solution and provide tips on how to use it for obtaining high-quality RNA, as well as describe possible modifications for different conditions. The protocol is based on a phase separation, where RNA is maintained in the aqueous phase and DNA and proteins remain in the interphase and organic phase. After cleaning, precipitation, and resuspension steps, the RNA is ready to be quantified and used for downstream applications. By following this protocol, good yields of high-quality RNA can be obtained from a wide variety of tissues and organisms, and we exemplify the approach here using plant tissues. Some plant tissues contain extra interferents (such as sugars), and for high-quality RNA isolation from those tissues, an alternate protocol is provided. © 2022 The Authors. Current Protocols published by Wiley Periodicals LLC.
Basic Protocol : RNA isolation with homemade acid guanidinium thiocyanate-phenol-chloroform (AGPC)
Alternate Protocol : RNA isolation from high carbohydrate‒containing tissues using an NTES-AGPC combination
INTRODUCTION
Studies of gene expression are common in research laboratories, and differential gene expression analysis is regularly used to study biological processes or compare among genotypes, tissues, and developmental stages (Spies, Renz, Beyer, & Ciaudo, 2019; Verdonk, Haring, Tunen, & Schuurink, 2005; Wang, Gerstein, & Snyder, 2009). In addition, differential gene expression can provide insights into the effect of a particular chemical treatment (Meneses et al., 2020). The most common technique to study the expression of single genes is reverse transcription followed by quantitative real-time polymerase chain reaction (RT-qPCR). Here, gene fragments are amplified from cDNA with gene-specific primers, and amplification is monitored at every cycle. RT-qPCR has largely replaced other methods for gene expression analysis, such as gel-based RT-PCR and RNA gel blot analysis (i.e., northern blot; Bustin et al., 2009). For global studies, and due to advances in technology, the costs of sequencing-based methods such as RNA-seq have dropped drastically, making transcriptome-wide studies accessible to a vast number of laboratories (Kukurba & Montgomery, 2015).
Analysis of gene expression requires the isolation of high-quality RNA. While RNA extraction is one of the most common methods in molecular biology, it is also quite challenging, mainly because of the potential for breakdown of the RNA during extraction by extremely stable RNase enzymes (Arraiano et al., 2010). To inactivate RNases and obtain intact RNA, extraction methods commonly include organic solvents, protein destabilizing agents, and other toxic chemicals. A very popular choice for RNA extraction is the combination of phenol and chloroform (Schneiderbauer, Sandermann, & Ernst, 1991), but cetyltrimethylammonium bromide and 2-mercaptoethanol are also common choices (Gambino, Perrone, & Gribaudo, 2008). More recently, a very affordable method using nontoxic homemade buffers was developed (see Current Protocols article: Oñate-Sánchez & Verdonk, 2021; Oñate-Sánchez & Vicente-Carbajosa, 2008). In addition to obtaining intact RNA, a successful RNA extraction method should yield RNA that is free from contaminants. As such, RNA extraction methods must fulfill two goals: inactivate RNases and separate RNA from other cellular components. Furthermore, for most downstream applications, it is desirable to remove DNA contamination, which usually copurifies with RNA during typical extraction procedures. This can be done through specific precipitation of RNA (Lee, Cheung, & Leung, 2015), DNase treatment (Dotti & Bonin, 2011), or a combination of both (Dotti & Bonin, 2011).
RNA extraction used to be a very bothersome process, mostly due to possible RNase activity. For instance, glassware needed to be baked for hours at high temperatures to inactivate the highly stable RNases. In addition, reagents needed to be treated with RNase-inactivating chemicals such as diethyl pyrocarbonate (DEPC), mostly because the water used in the laboratory could not be trusted to be free of RNase activity. Thankfully, there have been incredible advancements in molecular biology in the last few decades, and RNA extraction is currently much simpler. In order to inactivate potential RNases, common practice is to autoclave pipette tips and tubes. Alternatively, sterile nuclease-free tips and tubes are commercially available. Further, instead of using DEPC-treated water, laboratories that own a Milli-Q water filter machine can safely use that water directly for RNA work because it removes all traces of protein, including RNases. Given these advances in the generation of RNase-free reagents, and the fact that RNases are released from the tissue of interest during processing, it is often more important to focus on the inactivation of RNases within the RNA extraction protocol itself by using solutions that facilitate this process.
One of the oldest techniques to isolate RNA is based on using an acid guanidinium thiocyanate-phenol-chloroform (AGPC) solution (Chomczynski & Sacchi, 1987). AGPC is used to extract RNA from various tissues, and it can result in high yield even when starting from low amounts of starting material. AGPC is often used in commercial kits and ready-made extraction buffers such as TRIzol, which made the AGPC technique also highly reproducible and scalable. However, because of the increasing use of these ready-made methods, the actual composition of the working solution became less known. This also makes it harder to make modifications or devise alternative protocols. Further, the recent COVID-19 crisis demonstrated that reliance on commercial kits and ready-made solutions with proprietary compositions can lead to an inability to perform certain crucial experiments during times of supply shortages. In such cases, do-it-yourself approaches can be incredibly useful (see Current Protocols article: Graham, Dugast-Darzacq, Dailey, Darzacq, & Tjian, 2021).
Here, we describe a simple procedure to prepare a homemade AGPC solution, and we discuss how to execute a clean RNA extraction, providing tips and tricks to optimize the procedure and increase yield. In the Basic Protocol, we describe a standard RNA extraction method from the grinding of samples to the final quantification and analysis of the resulting RNA material. Although we focus on plant tissue in this manuscript, there are many examples in the literature where AGPC is used for RNA extractions from animals, fungi, bacteria, and viruses (Akin, Wu, & Lin, 1998; Béra-Maillet, Ribot, & Forano, 2004; Kang, Denman, Morrison, Yu, & McSweeney, 2009; Sparmann, Jäschke, Loehr, Liebe, & Emmrich, 1997; Wang et al., 2011). We also provide an Alternate Protocol, which can be used to optimize RNA extraction from tissues with high carbohydrate content (such as fruits and seeds) using the homemade AGPC in combination with another home-made buffer (Reyes et al., 2011).
The RNA isolated using the methods described here can be used in all common downstream applications for gene expression analysis, including RT-qPCR, and is even of enough quality for RNA-seq analysis. The fact that the AGPC solution can be prepared at a relatively low cost and with commonly available reagents will be beneficial to many research laboratories.
Basic Protocol: RNA ISOLATION WITH HOMEMADE ACID GUANIDINIUM THIOCYANATE-PHENOL-CHLOROFORM (AGPC)
Here we describe the extraction of RNA using homemade AGPC, which is a versatile solution used to solubilize DNA, RNA, or protein. Proteins, such as RNases, are denatured by guanidinium thiocyanate and phenol. The crucial steps involved in this protocol are phase separation, extraction, precipitation, and resuspension in different solutions. Phase separation is initiated by adding chloroform, which separates DNA, RNA, and proteins from each other. After a centrifugation step, the RNA is maintained in the aqueous (top) phase, while the proteins and DNA remain largely in the interphase (middle) and organic (lower) phase. The aqueous phase is transferred to a new tube, and the RNA is then precipitated with isopropanol. Finally, the pellet is washed with 70% ethanol, dried, and resuspended in RNase-free water.
Materials
-
Frozen tissue sample
-
Liquid nitrogen
-
Homemade AGPC (see recipe)
-
100% chloroform
-
Buffer-saturated phenol, pH 8 (e.g., Millipore Sigma, cat. no. 77607)
-
1:1 phenol:chloroform prepared with buffer-saturated phenol
-
100% isopropanol
-
70% ethanol prepared with RNase-free water
-
RNase-free ultrapure water (e.g., DEPC-treated water, Thermo Fisher Scientific, cat. no. 4387937, or Milli-Q water)
-
Agarose (CAS no. 9012-36-6)
-
TBE or TAE buffer (e.g., Millipore Sigma, cat. nos. T7527-1L and T8280-1L, respectively)
-
Tissue grinder (e.g., mortar and pestle, CryoMill, Eppendorf Autoclavable Safe-Lock Micropestle, or equivalent)
-
1.5-ml microcentrifuge tubes, RNase-free
-
Spatula, sized for tubes
-
Microcentrifuge, 12,000 to 16,000 maximum speed
-
Spectrophotometer (e.g., NanoDrop)
-
Additional reagents and equipment for agarose gel electrophoresis (see Current Protocols article: Armstrong & Schulz, 2015)
CAUTION : Because of the use of organic compounds, all steps must be performed in a suitable fume hood with efficient ventilation. Safety glasses and reagent-impermeable protective gloves should be worn. Avoid contact of the solutions with eyes or skin. Before starting, be aware of the safety protocols of your institution in case of inhalation or contact of the reagent with eyes or skin.
Sample preparation
1.Grind tissue to a fine powder in the presence of liquid nitrogen.
2.Weight 100 to 200 mg resulting powder, and transfer to a cold 1.5-ml microcentrifuge tube. Avoid thawing sample.
Cell lysis and protein precipitation
3.Add 1 ml cold (4°C) AGPC solution and close tube. Mix tissue with the solution by inversion six times.
4.Shake vigorously for 15 s.
5.Incubate sample at room temperature for 5 min.
6.Add 0.25 ml chloroform.
7.Shake vigorously for 15 s.
8.Centrifuge 5 min at maximum speed, room temperature, in a microcentrifuge.
9.Transfer aqueous (upper) phase to a new tube.
10.Optional : Perform 1:1 phenol:chloroform treatment of the (upper) aqueous phase, followed by chloroform treatment:
-
Add 1 volume (i.e., 0.5 ml) phenol:chloroform to the aqueous phase.
-
Shake vigorously for 15 s.
-
Centrifuge 5 min at maximum speed, room temperature, in a microcentrifuge.
-
Transfer aqueous (upper) phase to a new tube.
This step is recommended when the interphase is a thick band and/or debris can still be seen floating in the upper phase.
Wash and solubilization
11.Add 1 volume (i.e., 0.5 ml) isopropanol at room temperature to precipitate.
12.Mix well by inverting.
13.Incubate at room temperature for 10 min to precipitate RNA.
14.Centrifuge 5 min at maximum speed, room temperature, in a microcentrifuge.
15.Discard supernatant.
16.Wash pellet with 1 volume room temperature 70% ethanol, and pour off supernatant.
17.Dry pellet at room temperature.
18.Resuspend pellet in the appropriate volume of RNase-free ultrapure water.
19.Quantify RNA using a spectrophotometer, and inspect quality using a 1% (w/v) agarose gel in TBE or TAE buffer.
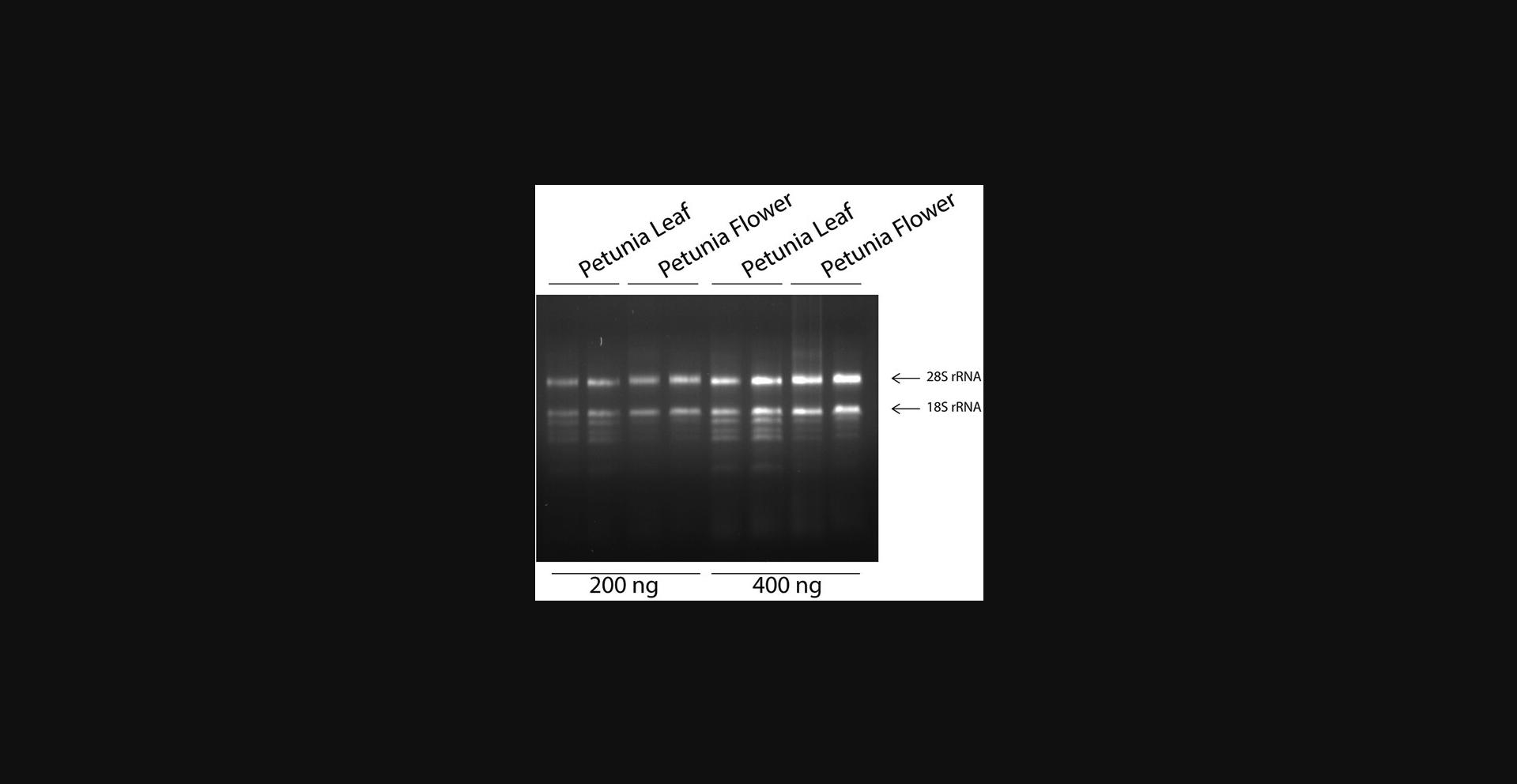
20.Store RNA at ‒20°C or ‒80°C.
Alternate Protocol: RNA ISOLATION FROM HIGH CARBOHYDRATE‒CONTAINING TISSUES USING AN NTES-AGPC COMBINATION
For some extractions, especially from high carbohydrate‒ and/or secondary metabolite‒containing tissues, the Basic Protocol can result in RNA that exhibits significant contamination with these compounds. Such is the case when using, for instance, fruits and some other complex plant tissues. In those cases, we advise following an alternate method, which uses a combination of the NTES buffer and APGC. This method follows the same general procedure as the Basic Protocol but features an initial phenol-chloroform phase separation step that reduces the amount of interfering contaminants. The method was used by Holding et al. (2007) and Reyes et al. (2011) for RNA extraction from maize kernels, which contain high levels of starch, and has been used in our laboratory to isolate good-quality RNA from several high carbohydrate‒containing tissues such as tomato fruit, mango fruit, and marigold petals (J. C. Verdonk, personal communication).
Additional Materials (also see the Basic Protocol)
- NTES buffer (see recipe)
- Refrigerated microcentrifuge
Sample preparation
1.Follow steps 1 and 2 in the Basic Protocol.
Cell lysis and protein precipitation
2.Add 200 µl NTES buffer and 200 µl of 1:1 phenol:chloroform (4°C).
3.Thaw while shaking vigorously.
4.Centrifuge 10 min at maximum speed, 4°C, in a microcentrifuge.
5.Add 200 µl of 1:1 phenol:chloroform (4°C).
6.Shake well to mix, and centrifuge 10 min at maximum speed, 4°C, in a microcentrifuge.
7.Add 200 µl chloroform (4°C) and shake well.
8.Place tubes on ice for 5 min.
9.Centrifuge 10 min at 10,000 × g , 4°C.
10.Take aqueous phase (upper) to a new tube.
11.Add 600 µl AGPC solution.
12.Shake well for 15 s, and incubate for 5 min at room temperature.
13.Add 200 µl chloroform. Shake well and incubate at room temperature for 2 to 3 min.
14.Centrifuge 10 min at 10,000 × g , room temperature.
15.Take upper aqueous phase to a new tube.
Wash and solubilization
16.Add 1 volume isopropanol and mix well. Continue with the Basic Protocol from step 12 onward.
REAGENTS AND SOLUTIONS
AGPC solution
-
0.4 M ammonium thiocyanate
-
0.8 M guanidine thiocyanate
-
0.1 M sodium acetate, pH 5
-
5% (v/v) glycerol
-
38% (v/v) phenol
-
1.Prepare aqueous solution: In ∼500 ml RNase-free water, dissolve 37.45 g ammonium thiocyanate (76.12 g/mol, CAS no. 1762-95-4) and 116.27 g guanidine thiocyanate (118.16 g/mol, CAS no. 593-84-0). Add 41 ml of 3 M sodium acetate, pH 5 (see recipe). Finally, add 61.5 ml of 100% glycerol (CAS no. 56-81-5).
-
Melt 500 g phenol crystals (CAS no. 108-95-2) in an ∼50°C water bath. Carefully add melted phenol to 500 ml aqueous solution prepared in the first part. Mix well.
Add RNase-free water to a final volume of 1.23 L.
CAUTION: Always use personal protective equipment and work in a fume hood. Phenol is extremely slippery and dangerous, and you are working with a large amount. Be careful when melting and adding phenol; it is probably best to not do this alone and to let someone help you.
This solution is comparable to TRIzol (Invitrogen, cat. no. 15596026), but the solution is not pink.
We made the calculation considering 500 g phenol crystals as 38% of the final mixture, which gives a final volume of 1.23 L. All amounts of the components of the mixture are calculated for a final volume of 1.23 L.
It is easiest to buy 500 g phenol crystals in a glass bottle (i.e., Millipore Sigma, cat. no. P1037-500G or comparable). This bottle can be placed in a water bath at ∼50°C without any need to handle the crystals.
With 500 g phenol as 38% (v/v) and the density of phenol (1.07 g/ml), this will be 500 g / 1.07 g/ml = 467.3 ml total volume. Therefore, the total volume of the AGPC solution should be 467.3 ml × (100% / 38%) = 1229.73 ml (∼1.23 L). This is the final volume for the total solution, as stated above.
Although molecular biology‒grade phenol is used here, we have tested lower-purity phenol (e.g., Kosher/Halal food‒grade phenol; e.g., Millipore Sigma, cat. no. W322318-1KG-K), which is significantly cheaper, and we have found it to work well.
The homemade AGPC solution is good for years if it is stored in a dark, cold place at 4°C. It is recommended to make working aliquots of 250 or 500 ml and also to store aliquots at 4°C while not using them. Depending on the type of phenol used, the AGPC solution oxidizes to an orange pinkish color. However, we have found that this does not negatively affect the quality of the solution or the RNA extraction.
NTES buffer
- 100 mM sodium chloride (CAS no. 7647-14-5)
- 20 mM Tris·HCl, pH 8 (e.g., Invitrogen, cat. no. 15568025)
- 10 mM EDTA (CAS no. 60-00-4)
- 20% (w/v) SDS (CAS no. 151-21-3)
- Store 25- to 50-ml aliquots at 20°C for up to 1 month
Use RNase-free water for all solutions.
Sodium acetate, 3 M, pH 5
Add 142.89 g sodium acetate trihydrate (136.08 g/mol, CAS no. 6131-90-4) to 250 ml RNase-free water. Add ∼35 ml glacial acetic acid (60.05 g/mol, 1.049 g/ml) to the solution. When all salt has been dissolved, adjust pH to 5 with additional glacial acetic acid. Add RNase-free water to 500 ml. Autoclave and store at room temperature until needed.
COMMENTARY
Background Information
The method for RNA isolation described in this article was initially described as AGCP extraction (Chomczynski & Sacchi, 1987), and with that protocol extraction of pure RNA at high yield could be achieved. In the original article, the principle was extraction, precipitation, wash, and solubilization and was a solid alternative to the method most commonly used at that time, guanidinium-thiocyanate (Chirgwin, Przybyla, MacDonald, & Rutter, 1979), which while efficient for RNA extraction and separation of RNA and DNA takes more time and requires the use of an ultracentrifuge (Chomczynski & Sacchi, 2006). The Chomczynski and Sacchi method was picked up by many companies and was branded under different commercial names, such as TRIzol, Tri-Reagent, or TRIsure, all with the same principle and advantages. One of the main benefits of using AGPC compared with the original guanidinium-thiocyanate method by Chirgwin and colleagues is the reduced amount of time and sample needed for RNA extraction. Another advantage of the method is that, compared with other phenol-based protocols (see Current Protocols articles: Collart & Oliviero, 1993; Ribaudo, Gilman, Kingston, Choczynski, & Sacchi, 1992), AGPC can be carried at room temperature and without extensive incubation times. In addition, and compared with column-based kits, AGCP yields a higher RNA quantity (Sultan et al., 2014). Another advantage of the AGPC method is the wide range of tissues it can be used for (Akin et al., 1998; Béra-Maillet et al., 2004; Kang et al., 2009; Sparmann et al., 1997; Wang et al., 2011). Despite its many advantages, the protocol uses hazardous components, and caution is needed when following it. Further, as mentioned before, various factors could affect RNA isolation such as higher amounts of RNases or the presence of interferents such as carbohydrates, and in the latter case the AGCP method may not yield the desired results. This can be solved by using the Alternate Protocol (NTES-AGPC combination), which has been successfully used, for instance, on maize aleurones (Holding et al., 2007; Reyes et al., 2011).
The battle with RNases is crucial for the extraction of good-quality RNA (Sambrook, & Russell, 2001). RNases are proteins from a family of ribonucleases with an RNA-degrading catalytic activity (Arraiano et al., 2010). These ubiquitous enzymes are present in all living cells and on the skin and laboratory surfaces, but the main source of concern for RNases is the tissue from which the RNA will be extracted. When the tissue is ground, all proteins, including RNases, will be in contact with the nucleic acids in the extract. The key is to keep the mixture as cold as possible (i.e., with liquid nitrogen) to limit the activity of these enzymes until RNase-inactivating conditions are ensured. The AGPC method inactivates RNases by using guanidine thiocyanate as a chaotropic agent (i.e., a compound that disrupts hydrogen bonds in solution). Another key compound in this method, used for the separation between nucleic acids and proteins, is phenol. Phenol plays an important role in protein denaturation by shifting the proteins from polar to nonpolar. Also, the nonpolar nature of phenol makes it easy to interact with the also nonpolar chloroform. Chloroform facilitates phase separation, allowing for a smooth separation between DNA/RNA and proteins. Finally, the low pH of the AGPC solution helps with the separation between DNA and RNA (Farrag, Abri, & Leipzig, 2020). The low pH makes the phosphate groups from DNA uncharged, which maintains the DNA molecules in the organic phase of the solution, while RNA is maintained in the aqueous phase. This allows for the separation between the closely related RNA and DNA molecules.
Currently, the AGPC method is one of the most widely used methods for RNA extraction, used not only for plants but also for extractions from animals, fungi, bacteria, and viruses (Akin et al., 1998; Béra-Maillet et al., 2004; Kang et al., 2009; Sparmann et al., 1997; Wang et al., 2011). Although the solution is commercially available, it is quite simple to prepare from scratch, which is considerably cheaper. Here, we describe the method and also how to prepare the AGPC solution, which we hope will be useful to researchers, particularly in resource-limited settings or when supply chains are affected.
Critical Parameters
A good-quality RNA extraction starts with good-quality starting material. Independent of the nature of the material (human, animal, plant, fungi, or others), the first critical parameter is proper material grinding. When the starting material still contains unground pieces, they can be a source for RNases and other contaminants because intact cells and larger chunks of tissue will contain compartmentalized RNases that will not be inactivated by the AGPC solution. This may cause them to degrade the RNA in subsequent steps of the RNA isolation process. Making absolutely sure that the tissue is ground to a fine powder is the most essential parameter for a good RNA extraction. Additionally, liquid nitrogen is fundamental in the grinding process. Keeping the sample frozen with liquid nitrogen while grinding is crucial, and storing the samples at ‒80°C is ideal for most cases. Once the material is ground and ready for extraction, and once the AGPC solution is added, thawing of the sample is no longer a problem.
Furthermore, RNA extraction is a constant battle against RNases. Having a pipette set only for RNA extractions is good practice but not mandatory. When a dedicated pipette set for RNA extraction is not available, cleaning the pipettes with 70% ethanol and paper towels before using them helps to get rid of any previous material present in the pipettes (which could be a source of RNases). It is highly recommended to use RNase-free tubes and pipette tips and a clean bench, wiped with abundant 70% ethanol.
For a good RNase-free agarose gel, it is good to dedicate an electrophoresis tank for RNA only. Other applications in the laboratory such as plasmid minipreps use RNases, which can end up in the electrophoresis buffer or tank. Washing the tank, gel tray, and combs with dishwashing soap and rinsing well with deionized water before use should be sufficient to get rid of all proteins, including RNases. We also recommend using freshly prepared TAE or TBE buffer. In our experience, the common lab stock of 50× TAE or 10× TBE can be used without problems, but dedicated stocks for RNA gels could prevent contaminations with RNases. Agarose and flasks do not need any special treatments.
Troubleshooting
Even when taking all necessary precautions, it is still possible that RNA degradation will happen. In that case, it is wise to retrace your steps to find the possible cause. The most common cause is incomplete grinding of the tissue, causing RNases to be released from the intact cells at a later step in the protocol. When grinding, it is also possible that the frozen tissue remains as a chunk in the AGPC solution, which thaws slowly. When the tissue in this frozen chunk is not completely saturated with AGPC solution, RNases in the tissue may degrade the RNA. It is advised to close the tube immediately after adding the AGPC solution and to put it in a hot water bath (floating rack with hot tap water) for 10 s before shaking it thoroughly. This will prevent patches of ice where RNA degradation may occur. Other reasons for RNase-mediated degradation are less likely, but it is important to use nuclease (RNase/DNase)-free tips, tubes, water, and other solutions (isopropanol, ethanol). A cleaned workbench, wiped with 70% ethanol, and clean pipettes will also prevent possible RNase contamination. Finally, stay clear of pipettes and laboratory benches used for plasmid minipreps and genomic DNA isolations. These methods typically use high quantities of RNases.
When the RNA is of good quality but the yield is low, there are several things to consider. The starting tissue could have been very small. It is advised to use 100 to 200 mg, and if less is used you may need to scale down all volumes and amounts in the method. Another reason for low yield, which may appear counterintuitive, is that too much tissue was used. Although the method is presented as 1:2 ratio of tissue weight to AGPC solution volume, the originally described ratio was much higher at a 1:10 ratio. Because the method has been scaled down for microcentrifuge tubes, other ratios were tested, and a 1:2 ratio was found to still work very well. Because not all tissues have been thoroughly tested, it is possible that a larger amount of AGPC solution would result in better yields, and this could be evaluated. Make sure to also scale up all other solutions. Similarly, centrifuge times may need to be increased because tabletop centrifuges for larger volume tubes have a lower maximum speed.
A nonoptimal tissue-to-buffer ratio can also lead to genomic DNA contamination. When the ratio is not optimal, it is possible that the pH of the mixture becomes too high, and the buffering capacity of the sodium acetate may not be sufficient anymore. Ideally, a pH of 5 is needed to keep the RNA in the aqueous phase and the genomic DNA phosphorylated and in the organic phase. Therefore, a high degree DNA contamination could be prevented by reducing the amount of tissue or increasing the buffer and other solutions. Just make sure to adjust all volumes accordingly.
DNA contamination can also be caused by shearing of genomic DNA by excessive handling of the samples. Vortexing will increase such contamination because smaller pieces of genomic DNA apparently “mimic” RNA better and tend to separate into the aqueous phase during the extraction.
Genomic DNA contamination can be illustrated by gel electrophoresis. Supplementary Figure 1 shows a comparison with samples that have genomic DNA contamination. When genomic DNA contamination cannot be completely avoided, it is also possible to assess the impact of contamination by performing a reverse transcription reaction in parallel with a reverse transcription‒free control. It is also possible to use exon-exon spanning primers when available. The best control is a primer that overlaps the intron-exon junction. These last two options, however, require a well-annotated genome sequence to be able to design the primers.
Other contaminants can also be present in the isolated RNA sample. In some cases, after the final step when water has been added to the pellet, the solution becomes turbid, and even vortexing and pipetting up and down does not help. The solution is viscous, and this makes it hard to handle. In such cases, the contamination is most likely caused by carbohydrates, possibly pectins. It is best to try the Alternate Protocol for this tissue, in which the excess of carbohydrates will be removed during the first steps. Alternatively, when the sample is too valuable and cannot be discarded, it is possible to remove the contaminant by centrifugation. Sometimes, freezing the samples at ‒20°C will help with this because the low temperatures will often make some of the contaminants precipitate. A 30-min centrifugation (cold or room temperature) will often then yield a white pellet of the contaminant. The clear aqueous solution can then be moved to a new RNase-free tube.
See Table 1 for a list of common problems with the protocols, their likely causes, and potential solutions.
Problem | Possible cause | Solution |
---|---|---|
RNA degradation | RNase activity | Establish RNase-free conditions and solutions |
Incomplete grinding of tissue; improper homogenization during initial addition of AGPC solution | Grind samples in the presence of liquid nitrogen until a fine powder is obtained; make sure tissue is well mixed with AGPC solution and thawed quickly (in hot water bath) to ensure proper homogenization | |
Degradation during gel electrophoresis | If RNA appears as a smear on the gel, the most likely reason is that the RNA was already degraded; however, it is also possible the gel running environment is not RNase-free; see Critical Parameters for running an RNase-free agarose gel | |
Low yield | Excess of initial material influencing extraction efficiency | AGPC solution is best used at a 1:10 tissue-to-buffer ratio (100 mg sample per 1 ml AGPC solution), although 1:5 and 1:2 ratios work fine; try to use 100 mg tissue and 1 ml AGPC solution |
Presence of contaminants | High level of contaminants such as carbohydrates and other secondary metabolites in tissue | Use Alternate Protocol |
Low OD260/280 ratio | Protein contaminants in sample | Carry out phenol:chloroform step to avoid contamination of both proteins and guanidine salts |
Low OD260/230 ratio | Guanidine salt contaminants in sample | Be careful at the moment of taking the aqueous phase; do not carry part of the interphase or organic phase |
DNA contamination | Ratio of tissue to AGPC closer to 1:1 causing higher pH than optimal; DNA transfer to the aqueous phase | Adapt tissue-to-AGPC ratio to somewhere within 1:5 to 1:10 |
Shearing of genomic DNA during extraction | Do not vortex but shake vigorously instead |
- AGPC, acid guanidinium thiocyanate-phenol-chloroform; OD, optical density.
Understanding Results
Even the most experienced users will occasionally observe sample RNA degradation during an extraction procedure. Degradation of RNA is very common because of the difficulty to inactivate RNases. If the steps in this protocol are followed, RNases should not have had a chance to significantly degrade the RNA. Using gel electrophoresis and spectrophotometry, it will be possible to determine if the extraction was successful.
Figure 1 shows a representative image of an RNA extraction from petunia leaves and flowers using the AGPC method. Two independent samples were used for both tissues, and two concentrations (200 and 400 ng) were loaded on a standard TBE agarose gel. Although RNA separation with formamide or glyoxal is needed for an RNA gel blot (Sambrook & Russell, 2001), that is not needed here to evaluate the integrity of the RNA preparation. The gel in Figure 1 shows the ribosomal RNA bands for each sample. What is also shown is the absence of any genomic DNA trace (compare to Supplementary Fig. 1). To determine if the RNA is of good quality, there are some things to consider: First, there should be no smear below the bands, which indicates smaller pieces and possible breakdown. Second, the highest band should be brighter than the lower one; it is a larger fragment and therefore has more intercalated ethidium bromide. Therefore, if the lower band seems brighter than the top one, you might have degradation.
In parallel with gel inspection, the spectrophotometric results should be used to determine the concentration and purity of the extracted RNA. The concentration of the RNA can be calculated using the OD260, which corresponds to 40 ug/ml RNA per OD unit. If values higher than 1.0 are obtained, the sample should be diluted because the absorption of nucleotides is not linear above that value. In practice, it is advised to use a 10-fold dilution of the extracted RNA for spectrophotometric analysis. The OD260/OD230 and OD260/OD280 ratios can provide information about the purity of the RNA. An OD260/OD280 ratio close to 2.0 is generally accepted as good quality RNA. The OD260/OD230 ratio should be between 2.0 and 2.2. If the ratio is lower, possible contaminants that absorb at 230 nm (such as phenol and/or guanidine) may still be present. These can interfere with downstream applications. These ratios should not, however, in our opinion, be used to discard samples; samples with OD260/OD280 and OD260/OD230 ratios of 1.5 have been used in our laboratory for perfect RT-qPCR experiments. They should, instead, be used as an indication of the quality, and if one or two samples stand out, that could be an indication that something odd occurred during that specific extraction.
It is advised to not rely on the spectrophotometric measurements alone because the nucleotides from degraded RNA will absorb at the same wavelength as RNA and DNA and may give you the idea that you have intact RNA when in fact you may not. Spectrophotometric measurements, together with electrophoretic analysis, will tell you if you have good quality RNA and if you have genomic DNA contamination.
Time Considerations
Grinding and organizing samples can take a lot of time. For fruit and other materials, grinding may take up most of your time. We propose to plan and practice this well before moving to the Basic and/or Alternate Protocols. It is possible that grinding and organizing takes so much time that it is better to keep all samples at ‒80°C and to continue the next day. The preparation of the AGPC solution takes 1 to 2 hr. For the Basic Protocol, extraction times may vary depending on the experience of the operator. It is recommended to start with a small batch to practice and later scale up to a full microcentrifuge (i.e., 24 samples). For 24 samples, the process usually takes about 1 to 2 hr. The Alternate Protocol takes about the same time. The times for drying the RNA pellets may vary.
Acknowledgments
This work was funded by the Chilean National Agency for Research and Development (ANID) and Chilean National Scholarship Program for Graduate Studies, DOCTORADO BECAS CHILE/2019 – 72200074. The authors thank Dr. Francisca Reyes Márquez and Roel Heutink for their help with the preparation of the RNA gels.
Author Contributions
Baltasar Zepeda and Julian C. Verdonk : conceptualization, funding acquisition, investigation, methodology, validation, writing of original draft, reviewing, and editing.
Conflict of Interest
The authors declare no conflict of interest.
Open Research
Data Availability Statement
Data sharing not applicable to this article as no datasets were generated or analyzed during the current study.
Supporting Information
Filename | Description |
---|---|
cpz1351-sup-0001-FigureS1.docx69.4 KB | Supporting Information |
Please note: The publisher is not responsible for the content or functionality of any supporting information supplied by the authors. Any queries (other than missing content) should be directed to the corresponding author for the article.
Literature Cited
- Akin, A., Wu, C. C., & Lin, T. L. (1998). A comparison of two RNA isolation methods for double-stranded RNA of infectious bursal disease virus. Journal of Virological Methods , 74, 179–184. doi: 10.1016/S0166-0934(98)00082-2.
- Armstrong, J. A., & Schulz, J. R. (2015). Agarose gel electrophoresis. Current Protocols Essential Laboratory Techniques , 10, 7.2.1‒7.2.22. doi: 10.1002/9780470089941.et0702s10.
- Arraiano, C. M., Andrade, J. M., Domingues, S., Guinote, I. B., Malecki, M., Matos, R. G., … Viegas, S. C. (2010). The critical role of RNA processing and degradation in the control of gene expression. FEMS Microbiology Review , 34, 883–923. doi: 10.1111/j.1574-6976.2010.00242.x.
- Béra-Maillet, C., Ribot, Y., & Forano, E. (2004). Fiber-degrading systems of different strains of the genus fibrobacter. Applied and Environmental Microbiology , 70, 2172–2179. doi: 10.1128/AEM.70.4.2172-2179.2004.
- Bustin, S. A., Benes, V., Garson, J. A., Hellemans, J., Huggett, J., Kubista, M., … Wittwer, C. T. (2009). The MIQE guidelines: Minimum information for publication of quantitative real-time PCR experiments. Clinical Chemistry , 55, 611–622. doi: 10.1373/clinchem.2008.112797.
- Chirgwin, J. M., Przybyla, A. E., MacDonald, R. J., & Rutter, W. J. (1979). Isolation of biologically active ribonucleic acid from sources enriched in ribonuclease. Biochemistry , 18, 5294–5299. doi: 10.1021/bi00591a005.
- Chomczynski, P., & Sacchi, N. (1987). Single-step method of RNA isolation by acid guanidinium thiocyanate-phenol-chloroform extraction. Analytical Biochemistry , 162, 156–159. doi: 10.1016/0003-2697(87)90021-2.
- Chomczynski, P., & Sacchi, N. (2006). The single-step method of RNA isolation by acid guanidinium thiocyanate-phenol-chloroform extraction: Twenty-something years on. Nature Protocols , 1, 581–585. doi: 10.1038/nprot.2006.83.
- Collart, M. A., & Oliviero, S. (1993). Preparation of yeast RNA. Current Protocols in Molecular Biology , 23, 13.12.1–13.12.5. doi: 10.1002/0471142727.mb1312s23.
- Dotti, I., & Bonin, S. (2011). DNase treatment of RNA. In G. Stanta (Ed.) Guidelines for molecular analysis in archive tissues (pp. 87–90). Springer-Verlag. doi: 10.1007/978-3-642-17890-0_18.
- Farrag, M., Abri, S., & Leipzig, N. D. (2020). pH-dependent RNA isolation from cells encapsulated in chitosan-based biomaterials. International Journal of Biological Macromolecules , 146, 422–430. doi: 10.1016/j.ijbiomac.2019.12.263.
- Gambino, G., Perrone, I., & Gribaudo, I. (2008). A rapid and effective method for RNA extraction from different tissues of grapevine and other woody plants. Phytochemical Analysis , 19, 520–525. doi: 10.1002/pca.1078.
- Graham, T. G. W., Dugast-Darzacq, C., Dailey, G. M., Darzacq, X., & Tjian, R. (2021). Simple, inexpensive RNA isolation and one-step RT-qPCR methods for SARS-CoV-2 detection and general use. Current Protocols , 1, e130. doi: 10.1002/cpz1.130.
- Holding, D. R., Otegui, M. S., Li, B., Meeley, R. B., Dam, T., Hunter, B. G., … Larkins, B. A. (2007). The maize Floury1 gene encodes a novel endoplasmic reticulum protein involved in zein protein body formation. Plant Cell , 19, 2569–2582. doi: 10.1105/tpc.107.053538.
- Kang, S., Denman, S. E., Morrison, M., Yu, Z., & McSweeney, C. S. (2009). An efficient RNA extraction method for estimating gut microbial diversity by polymerase chain reaction. Current Microbiology , 58, 464–471. doi: 10.1007/s00284-008-9345-z.
- Kukurba, K. R., & Montgomery, S. B. (2015). RNA sequencing and analysis. Cold Spring Harbor Protocols , 2015, 951–969. doi: 10.1101/pdb.top084970.
- Lee, J. T. Y., Cheung, K. M. C., & Leung, V. Y. L. (2015). Extraction of RNA from tough tissues with high proteoglycan content by cryosection, second phase separation and high salt precipitation. Journal of Biological Methods , 2, e20. doi: 10.14440/jbm.2015.40.
- Meneses, M., García-Rojas, M., Muñoz-Espinoza, C., Carrasco-Valenzuela, T., Defilippi, B., González-Agüero, M., … Hinrichsen, P. (2020). Transcriptomic study of pedicels from GA3-treated table grape genotypes with different susceptibility to berry drop reveals responses elicited in cell wall yield, primary growth and phenylpropanoids synthesis. BMC Plant Biology , 20, 66. doi: 10.1186/s12870-020-2260-6.
- Oñate-Sánchez, L., & Verdonk, J. C. (2021). Citrate-citric acid RNA isolation (CiAR) for fast, low-cost, and reliable RNA extraction from multiple plant species and tissues. Current Protocols , 1, e298. doi: 10.1002/cpz1.298.
- Oñate-Sánchez, L., & Vicente-Carbajosa, J. (2008). DNA-free RNA isolation protocols for Arabidopsis thaliana, including seeds and siliques. BMC Research Notes , 1, 93. doi: 10.1186/1756-0500-1-93.
- Reyes, F. C., Chung, T., Holding, D., Jung, R., Vierstra, R., & Otegui, M. S. (2011). Delivery of prolamins to the protein storage vacuole in maize aleurone cells. Plant Cell , 23, 769–784. doi: 10.1105/tpc.110.082156.
- Ribaudo, R., Gilman, M., Kingston, R. E., Choczynski, P., & Sacchi, N. (1992). Preparation of RNA from tissues and cells. Current Protocols in Immunology , 3, A.1I.1–A.1I.14. doi: 10.1002/0471142735.im1011s04.
- Sambrook, J., & Russell, D. W. (2001). Molecular cloning: A laboratory manual ( 3rd ed.). Cold Spring Harbor Laboratory Press.
- Schneiderbauer, A., Sandermann, H., & Ernst, D. (1991). Isolation of functional RNA from plant tissues rich in phenolic compounds. Analytical Biochemistry , 197, 91–95. doi: 10.1016/0003-2697(91)90360-6.
- Silva-Sanzana, C., Balic, I., Sepúlveda, P., Olmedo, P., León, G., Defilippi, B. G., … Campos-Vargas, R. (2016). Effect of modified atmosphere packaging (MAP) on rachis quality of “Red Globe” table grape variety. Postharvest Biology and Technology , 119, 33–40. doi: 10.1016/j.postharvbio.2016.04.021.
- Sparmann, G., Jäschke, A., Loehr, M., Liebe, S., & Emmrich, J. (1997). Tissue homogenization as a key step in extracting RNA from human and rat pancreatic tissue. Biotechniques , 22, 408–412. doi: 10.2144/97223bm07.
- Spies, D., Renz, P. F., Beyer, T. A., & Ciaudo, C. (2019). Comparative analysis of differential gene expression tools for RNA sequencing time course data. Briefings in Bioinformatics , 20, 288–298. doi: 10.1093/bib/bbx115.
- Sultan, M., Amstislavskiy, V., Risch, T., Schuette, M., Dökel, S., Ralser, M., … Yaspo, M. L. (2014). Influence of RNA extraction methods and library selection schemes on RNA-seq data. BMC Genomics , 15, 1–13. doi: 10.1186/1471-2164-15-675.
- Verdonk, J. C., Haring, M. A., Tunen, A. J. Van, & Schuurink, R. C. (2005). ODORANT1 regulates fragrance biosynthesis in petunia flowers. Plant Cell , 17, 1–13. doi: 10.1105/tpc.104.028837.1.
- Wang, P., Qi, M., Barboza, P., Leigh, M. B., Ungerfeld, E., Selinger, L. B., … Forster, R. J. (2011). Isolation of high-quality total RNA from rumen anaerobic bacteria and fungi, and subsequent detection of glycoside hydrolases. Canadian Journal of Microbiology , 57, 590–598. doi: 10.1139/w11-048.
- Wang, Z., Gerstein, M., & Snyder, M. (2009). RNA-seq: A revolutionary tool for transcriptomics. Nature Reviews Genetics , 10, 57–63. doi: 10.1038/nrg2484.
Citing Literature
Number of times cited according to CrossRef: 3
- Samuel Asamoah Sakyi, Alfred Effah, Emmanuel Naturinda, Ebenezer Senu, Stephen Opoku, Benjamin Amoani, Samuel Kekeli Agordzo, Oscar Simon Olympio Mensah, James Grant, Elizabeth Abban, Tonnies Abeku Buckman, Alexander Kwarteng, Richard K. Dadzie Ephraim, Kwabena Owusu Danquah, Comparison of Modified Manual Acid‐Phenol Chloroform Method and Commercial RNA Extraction Kits for Resource Limited Laboratories, International Journal of Clinical Practice, 10.1155/2023/9593796, 2023 , 1, (2023).
- Junsoo Park, Minju Bae, Hyeonah Seong, Jin hwa Hong, Su Jin Kang, Kyung hwa Park, Sehyun Shin, An innovative charge‐based extracellular vesicle isolation method for highly efficient extraction of EV‐miRNAs from liquid samples: miRQuick, Journal of Extracellular Biology, 10.1002/jex2.126, 2 , 12, (2023).
- Tyler Dang, Sohrab Bodaghi, Fatima Osman, Jinbo Wang, Tavia Rucker, Shih-Hua Tan, Amy Huang, Deborah Pagliaccia, Stacey Comstock, Irene Lavagi-Craddock, Kiran R. Gadhave, Paulina Quijia-Lamina, Arunabha Mitra, Brandon Ramirez, Gerardo Uribe, Alexandra Syed, Sarah Hammado, Iman Mimou, Roya Campos, Silva Abdulnour, Michael Voeltz, Jinhwan Bae, Emily Dang, Brittany Nguyen, Xingyu Chen, Noora Siddiqui, Yi Tien Hsieh, Shurooq Abu-Hajar, Joshua Kress, Kristina Weber, Georgios Vidalakis, A comparative analysis of RNA isolation methods optimized for high-throughput detection of viral pathogens in California’s regulatory and disease management program for citrus propagative materials, Frontiers in Agronomy, 10.3389/fagro.2022.911627, 4 , (2022).