Live Cell Monitoring and Enrichment of Stem Cell-Derived β Cells Using Intracellular Zinc Content as a Population Marker
Jeffrey C. Davis, Jeffrey C. Davis, Aharon Helman, Aharon Helman, José Rivera-Feliciano, José Rivera-Feliciano, Christine M. Langston, Christine M. Langston, Elise N. Engquist, Elise N. Engquist, Douglas A. Melton, Douglas A. Melton
Abstract
Our laboratory and others have developed protocols to generate glucose-responsive stem cell–derived β cells in vitro. The cells resulting from these protocols could supplement or replace the use of human cadaveric islets for cell-based therapy for diabetes. The combination of an unlimited supply of pluripotent stem cell–derived β cells and gene-editing approaches will facilitate numerous in vitro studies not possible with cadaveric islets. Here, we describe a protocol for fluorescent labeling and isolation of stem cell–derived β cells. This purification of SC-β cells is based on intracellular zinc content and is a simple method to complement other approaches for generating and assaying these cells. © 2019 The Authors.
Basic Protocol : Fluorescent labeling and isolation of stem cell-derived β cells
Basic Protocol:
Protocols to generate SC-β cells have been successfully performed in a number of laboratories (Nostro et al., 2015; Pagliuca et al., 2014; Rezania et al., 2014; Russ et al., 2015). These cells share a glucose responsiveness profile and similarity in gene expression to human cadaveric islets (Pagliuca et al., 2014; Veres et al., 2019). These protocols yield mixed populations of α, β, δ, and other cells types of the islet, as well as undifferentiated progenitors or smaller populations of undesired cells.
Insulin comprises approximately 10% of the total protein content within the β cell (Weir & Bonner-Weir, 2013). This abundance of insulin requires efficient packing and storage in secretory vesicles, which is achieved in SC-β cells (Pagliuca et al., 2014). Organization of insulin into dense core granules is facilitated by crystallization, seeded by two zinc ions at the center of each hexamer within the secretory vesicle (Dodson & Steiner, 1998). Thus, β cells have high levels of intracellular zinc and high expression of zinc transporters. Appropriate expression of zinc transporters and packaging of insulin have previously been confirmed in the SC-β population (Pagliuca et al., 2014), and high intracellular zinc has been used to image and isolate the islet β cell population (Burdette, Frederickson, Bu, & Lippard, 2003; Latif, Noel, & Alejandro, 1988; Lukowiak et al., 2001; Meeusen, Tomasiewicz, Nowakowski, & Petering, 2011). Here, we describe the use of live-cell zinc dyes for isolation and monitoring of SC-β cells. Recent reports have also suggested that enrichment of the SC-β population for extended culture may improve insulin secretory profiles of SC-β cells using genetic reporters (Nair et al., 2019; Veres et al., 2019). Here, we describe a method to enrich SC-β cells without the need for gene editing, allowing studies of SC-β biology across multiple genetic backgrounds and human islets.
The dye N -(6-methoxy-8-quinolyl)-p -toluenesulfonamide (TSQ) offers a simple, broadly applicable method to analyze the SC-β population across multiple backgrounds, as the TSQ+ population labels a large fraction (>80%) of the insulin+ population. This was measured using a differentiated genetic knock-in reporter line, INSmCherry, for insulin expression, as analyzed by flow cytometry (Fig. 1A,B). Furthermore, reaggregation after sorting allows for homogenous clusters of similar size and intracellular zinc content to human islets (Fig. 1C). These cells can be cultured in 96-well format, allowing large-scale experiments with multiple conditions and a defined number of cells in each cluster.
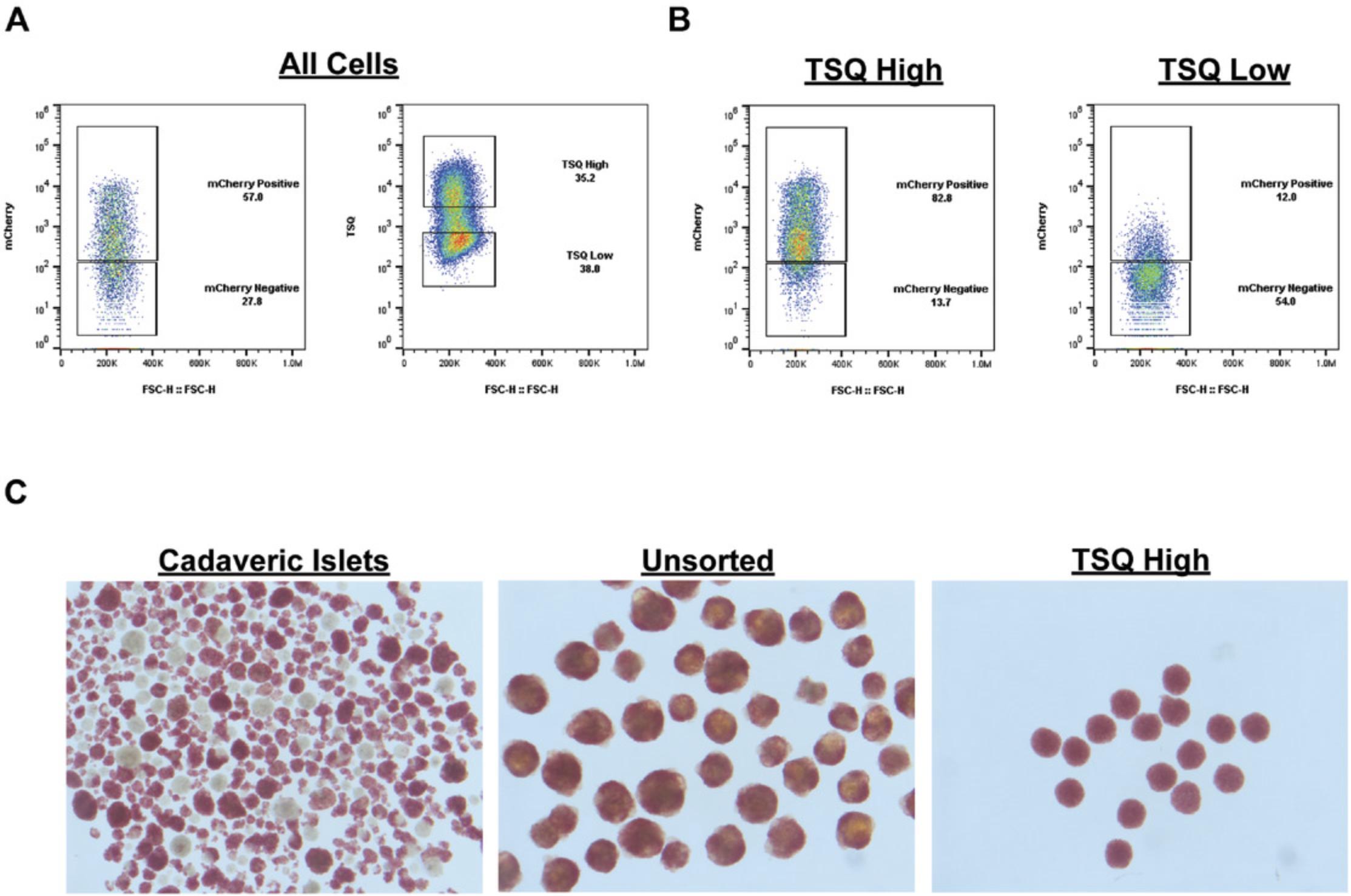
Fluorescent labeling and isolation of stem cell–derived β cells
The following protocol is useful to fluorescently label stem cell–derived β cells, allowing for rapid isolation of differentiated insulin-producing cells without the need for gene-edited reporter lines, and facilitates SC-β cell-specific analyses across many genetic backgrounds. This approach may enable studies with more broadly applicable conclusions by using genetically diverse pluripotent stem cell lines.
REAGENTS AND SOLUTIONS
S3 medium
- MCDB 131 basal medium (ThermoFisher, cat. no. 10372019)
- 8 mM D-glucose
- 1.23 g/L NaHCO3
- 2% fatty-acid-free bovine serum albumin (Proliant Biologicals, cat. no. 68700)
- 1:200 insulin-transferring-selenium-ethanolamine (ITS-X; ThermoFisher, cat. no. 51500056)
- 2 mM Glutamax (ThermoFisher, cat. no. 35050061)
- 0.25 mM vitamin C
- 1% 100× penicillin/streptomycin (ThermoFisher, cat. no. 15140122)
- Store up to 1 month at 4°C
Sorting buffer
- Phosphate-buffered saline (PBS; ThermoFisher, cat. no. 10010002) containing:
- 2% fetal bovine serum (FBS)
- 0.5% bovine serum albumin (BSA; Proliant Biologicals, cat. no. 68700)
- 1:1000 dilution of 10 mM Rho kinase inhibitor stock
- 125 µM ETDA
- Filter sterilize
COMMENTARY
Background Information
Purification of stem cell–derived β cells for culture or experimental analysis has been reported recently by several groups, including our own (Nair et al., 2019; Veres et al., 2019). In both of these reports, enrichment of the differentiated endocrine population improves glucose-stimulated insulin secretion, making this a desirable process for experiments utilizing SC-β cells from a diverse collection of pluripotent stem cell sources. For this reason, zinc-based enrichment offers a more rapid and broadly applicable method for enriching SC-β cells than genetically encoded reporters (Nair et al., 2019). Alternatively, CD49a enrichment is also useful in a number of different backgrounds, although this requires use of antibodies and needs more time and preparation for enrichment. Rapid analysis utilizing flow cytometry–based readouts of SC-β cells will be easier with TSQ than antibody-based methods, and is a more adaptable technique to combine with flow cytometry following brief or sequential treatments. In all, TSQ-based enrichment and imaging of SC-β cells is a rapid, flow cytometry–based technique for characterization and enrichment of SC-β cells.
Critical Parameters and Troubleshooting
Proper labeling of the SC-β cell population is reproducible using the dye concentrations and methods described here. Unstained controls containing propidium iodide and utilizing empty channels will allow for correct gates to be set during the experiment. Dissociation of SC-β cells can result in some cell death over time, and we recommend short dissociation times and proper quenching of dissociated cells before resuspension in TSQ-containing buffer. Inability to utilize UV-spectrum light to detect the TSQ fluorophore will also reduce the ability to detect and accurately sort SC-β cells by this method.
Anticipated Results
In our hands, the TSQ+ population closely resembles the total insulin+ cell population size resulting from SC-β cell differentiation protocols. TSQ does not overlap with emission spectra from common fluorophores residing in the green to far red channels, facilitating combination of several different dyes or transgenes with TSQ-based analysis. Reaggregation of TSQ-enriched SC-β cells occurs quickly. Immediately after sorting and plating TSQ-sorted cells into 96-well plates, cells form a sheet along the bottom of the well, which quickly forms connections and becomes spherical within 24 to 48 hr. These clusters are durable and easily withstand manipulation with pipetting or repeated wash steps. Clusters of 5000 cells usually generate a single spheroid after reaggregation. Deviation from 5000 cells per cluster can result in multiple clusters in a single well if too many or too few cells are allocated into each well. However, even under these conditions, we do not see significant variability in overall cluster integrity or health of culture. These clusters are stable for several weeks in culture before use.
We generally recover a fraction (<10%) of the starting insulin+ population, largely due to inefficiencies from FACS enrichment. This can be overcome by reducing stringency of the flow sorting settings and gates set during the sort, albeit at the cost of overall purity. Efficiency of the sort can also be improved by diluting samples if the abort rate is too high on the sorting instrument. These decisions should be made on a case-by-case basis to balance need for large cell numbers with desired purity of the resulting clusters.
Time Considerations
While TSQ-based sorting allows for reproducible enrichment of the SC-β population, cell sorting methods can be time consuming. In our experience, robust differentiations resulting in >30% insulin-producing cells can yield up to 5 million cells from a starting population of 50 million unenriched cells after 4 hr of sorting time. While preparation of the dissociated cells for sorting is quite rapid, available flow cytometry sorting time and numbers of differentiated cells are limiting factors for maximal cell enrichment and recovery for downstream experiments.
Acknowledgments
The authors would like to acknowledge the organizers of the 2018 International Society for Stem Cell Research meeting in Melbourne, Australia, as well as Dr. Nidhi Bansal for inviting this report. We would also like to thank the members of the Harvard University Bauer Core Facility flow cytometry core. This work was supported by grants from the National Institutes of Health and the National Institute of Digestive and Diabetic Kidney Disease: UC4 DK104159 and UC4 DK104165.
Literature Cited
- Burdette, S. C., Frederickson, C. J., Bu, W., & Lippard, S. J. (2003). ZP4, an improved neuronal Zn2+ sensor of the Zinpyr family. Journal of the American Chemical Society , 125(7), 1778–1787. doi: 10.1021/ja0287377.
- Dodson, G., & Steiner, D. (1998). The role of assembly in insulin's biosynthesis. Current Opinion in Structural Biology , 8(2), 189–194.
- Latif, Z. A., Noel, J., & Alejandro, R. (1988). A simple method of staining fresh and cultured islets. Transplantation , 45(4), 827–830.
- Lukowiak, B., Vandewalle, B., Riachy, R., Kerr-Conte, J., Gmyr, V., Belaich, S., … Pattou, F. (2001). Identification and purification of functional human beta-cells by a new specific zinc-fluorescent probe. Journal of Histochemistry and Cytochemistry , 49(4), 519–528. doi: 10.1177/002215540104900412.
- Meeusen, J. W., Tomasiewicz, H., Nowakowski, A., & Petering, D. H. (2011). TSQ (6-methoxy-8-p-toluenesulfonamido-quinoline), a common fluorescent sensor for cellular zinc, images zinc proteins. Inorganic Chemistry , 50(16), 7563–7573. doi: 10.1021/ic200478q.
- Nair, G. G., Liu, J. S., Russ, H. A., Tran, S., Saxton, M. S., Chen, R., … Hebrok, M. (2019). Recapitulating endocrine cell clustering in culture promotes maturation of human stem-cell-derived beta cells. Nature Cell Biology , 21(2), 263–274. doi: 10.1038/s41556-018-0271-4.
- Nostro, M. C., Sarangi, F., Yang, C., Holland, A., Elefanty, A. G., Stanley, E. G., … Keller, G. (2015). Efficient generation of NKX6-1+ pancreatic progenitors from multiple human pluripotent stem cell lines. Stem Cell Reports , 4(4), 591–604. doi: 10.1016/j.stemcr.2015.02.017.
- Pagliuca, F. W., Millman, J. R., Gurtler, M., Segel, M., Van Dervort, A., Ryu, J. H., … Melton, D. A. (2014). Generation of functional human pancreatic beta cells in vitro. Cell , 159(2), 428–439. doi: 10.1016/j.cell.2014.09.040.
- Rezania, A., Bruin, J. E., Arora, P., Rubin, A., Batushansky, I., Asadi, A., … Kieffer, T. J. (2014). Reversal of diabetes with insulin-producing cells derived in vitro from human pluripotent stem cells. Nature Biotechnology , 32(11), 1121–1133. doi: 10.1038/nbt.3033.
- Russ, H. A., Parent, A. V., Ringler, J. J., Hennings, T. G., Nair, G. G., Shveygert, M., … Hebrok, M. (2015). Controlled induction of human pancreatic progenitors produces functional beta-like cells in vitro. EMBO Journal , 34(13), 1759–1772. doi: 10.15252/embj.201591058.
- Veres, A., Faust, A. L., Bushnell, H. L., Engquist, E. N., Kenty, J. H., Harb, G., … Melton, D. A. (2019). Charting cellular identity during human in vitro beta-cell differentiation. Nature , 569(7756), 368–373. doi: 10.1038/s41586-019-1168-5.
- Weir, G. C., & Bonner-Weir, S. (2013). Islet beta cell mass in diabetes and how it relates to function, birth, and death. Annals of the New York Academy of Sciences , 1281, 92–105. doi: 10.1111/nyas.12031.
Citing Literature
Number of times cited according to CrossRef: 13
- Abiramy Jeyagaran, Max Urbanczyk, Shannon L. Layland, Frank Weise, Katja Schenke-Layland, Forward programming of hiPSCs towards beta-like cells using Ngn3, Pdx1, and MafA, Scientific Reports, 10.1038/s41598-024-64346-4, 14 , 1, (2024).
- Samuel D. Pollock, Israeli M. Galicia-Silva, Mai Liu, Zoe L. Gruskin, Juan R. Alvarez-Dominguez, Scalable Generation of 3D Pancreatic Islet Organoids from Human Pluripotent Stem Cells in Suspension Bioreactors, Tissue Morphogenesis, 10.1007/978-1-0716-3854-5_4, (51-87), (2024).
- Samuel D. Pollock, Israeli M. Galicia-Silva, Mai Liu, Zoe L. Gruskin, Juan R. Alvarez-Dominguez, Scalable generation of 3D pancreatic islet organoids from human pluripotent stem cells in suspension bioreactors, STAR Protocols, 10.1016/j.xpro.2023.102580, 4 , 4, (102580), (2023).
- Audrey V. Parent, Sudipta Ashe, Gopika G. Nair, Mei-Lan Li, Jessica Chavez, Jennifer S. Liu, Yongping Zhong, Philip R. Streeter, Matthias Hebrok, Development of a scalable method to isolate subsets of stem cell-derived pancreatic islet cells, Stem Cell Reports, 10.1016/j.stemcr.2022.02.001, 17 , 4, (979-992), (2022).
- Karla F. Leavens, Juan R. Alvarez-Dominguez, Linda T. Vo, Holger A. Russ, Audrey V. Parent, Stem cell-based multi-tissue platforms to model human autoimmune diabetes, Molecular Metabolism, 10.1016/j.molmet.2022.101610, 66 , (101610), (2022).
- Abiramy Jeyagaran, Chuan-en Lu, Aline Zbinden, Andreas L. Birkenfeld, Sara Y. Brucker, Shannon L. Layland, Type 1 diabetes and engineering enhanced islet transplantation, Advanced Drug Delivery Reviews, 10.1016/j.addr.2022.114481, 189 , (114481), (2022).
- Sevim Kahraman, Danielle Diegisser, Ercument Dirice, Stem cell therapies in diabetes, Regenerative Nephrology, 10.1016/B978-0-12-823318-4.00013-5, (201-210), (2022).
- Sevim Kahraman, Debasish Manna, Ercument Dirice, Basudeb Maji, Jonnell Small, Bridget K Wagner, Amit Choudhary, Rohit N Kulkarni, Harnessing reaction-based probes to preferentially target pancreatic β-cells and β-like cells, Life Science Alliance, 10.26508/lsa.202000840, 4 , 4, (e202000840), (2021).
- Jacqueline V. Schiesser, Thomas Loudovaris, Helen E. Thomas, Andrew G. Elefanty, Edouard G. Stanley, Integrin αvβ5 heterodimer is a specific marker of human pancreatic beta cells, Scientific Reports, 10.1038/s41598-021-87805-8, 11 , 1, (2021).
- Aharon Helman, Andrew L. Cangelosi, Jeffrey C. Davis, Quan Pham, Arielle Rothman, Aubrey L. Faust, Juerg R. Straubhaar, David M. Sabatini, Douglas A. Melton, A Nutrient-Sensing Transition at Birth Triggers Glucose-Responsive Insulin Secretion, Cell Metabolism, 10.1016/j.cmet.2020.04.004, 31 , 5, (1004-1016.e5), (2020).
- Edwin A. Rosado-Olivieri, Idil I. Aigha, Jennifer H. Kenty, Douglas A. Melton, Identification of a LIF-Responsive, Replication-Competent Subpopulation of Human β Cells, Cell Metabolism, 10.1016/j.cmet.2019.12.009, 31 , 2, (327-338.e6), (2020).
- Jeffrey C. Davis, Tiago C. Alves, Aharon Helman, Jonathan C. Chen, Jennifer H. Kenty, Rebecca L. Cardone, David R. Liu, Richard G. Kibbey, Douglas A. Melton, Glucose Response by Stem Cell-Derived β Cells In Vitro Is Inhibited by a Bottleneck in Glycolysis, Cell Reports, 10.1016/j.celrep.2020.107623, 31 , 6, (107623), (2020).
- Niloofar Rasouli, Douglas A. Melton, Juan R. Alvarez‐Dominguez, Purification of Live Stem‐Cell‐Derived Islet Lineage Intermediates, Current Protocols in Stem Cell Biology, 10.1002/cpsc.111, 53 , 1, (2020).