Isolation of High-Quality Total RNA from Small Animal Articular Cartilage for Next-Generation Sequencing
Roland Takács, Roland Takács, Szilárd Póliska, Szilárd Póliska, Tamás Juhász, Tamás Juhász, Krisztina B. Barna, Krisztina B. Barna, Csaba Matta, Csaba Matta
Abstract
Articular cartilage is characterized by a low density of chondrocytes surrounded by an abundant extracellular matrix (ECM) consisting of a dense mixture of collagens, proteoglycans, and glycosaminoglycans. Due to its low cellularity and high proteoglycan content, it is particularly challenging to extract high-quality total RNA suitable for sensitive high-throughput downstream applications such as RNA sequencing (RNA-Seq). Available protocols for high-quality RNA isolation from articular chondrocytes are inconsistent, resulting in suboptimal yield and compromised quality. This poses a significant difficulty in the application of RNA-Seq to study the cartilage transcriptome. Current protocols rely either on dissociation of cartilage ECM by collagenase digestion or pulverizing cartilage using various methods prior to RNA extraction. However, protocols for cartilage processing vary significantly depending on the species and source of cartilage within the body. Protocols for isolating RNA from human or large mammal (e.g., horse or cattle) cartilage samples are available, but this is not the case for chicken cartilage, despite the species being extensively used in cartilage research. Here, we present two improved RNA isolation protocols based on pulverization of fresh articular cartilage using a cryogenic mill or on enzymatic digestion using 1.2% (w/v) collagenase II. Our protocols optimize the collection and tissue processing steps to minimize RNA degradation and enhance RNA purity. Our results show that RNA purified from chicken articular cartilage using these methods has appropriate quality for RNA-Seq experiments. The procedure is applicable for RNA extraction from cartilage from other species such as dog, cat, sheep, and goat. The workflow for RNA-Seq analysis is also described here. © 2023 The Authors. Current Protocols published by Wiley Periodicals LLC.
Basic Protocol 1 : Extraction of total RNA from pulverized chicken articular cartilage
Alternate Protocol : Extraction of total RNA from collagen-digested articular cartilage
Support Protocol : Dissection of chicken articular cartilage from the knee joint
Basic Protocol 2 : RNA sequencing of total RNA from chicken articular cartilage
INTRODUCTION
Osteoarthritis (OA) is a common musculoskeletal disorder that affects the whole joint, causing structural alterations in articular cartilage, subchondral bone, ligaments, capsule, synovium, and periarticular muscles (Hunter & Bierma-Zeinstra, 2019). OA involves gradual changes in cartilage composition, leading to structural destruction and eventually joint failure. The pathogenesis of OA is complex and involves mechanical, inflammatory, and metabolic factors. Despite its socioeconomic burden, there are no regulatory-agency-approved disease-modifying osteoarthritis drugs (DMOADs) (Oo, Little, Duong, & Hunter, 2021). This necessitates research into the signaling pathways that regulate cartilage homeostasis in health and disease using cutting-edge methodology. However, due to the low cellularity and high proteoglycan content of articular cartilage, conventional RNA extraction protocols fail to provide high-quality total RNA suitable for sensitive downstream applications such as RNA sequencing (RNA-Seq). This is mainly attributable to the dense extracellular matrix (ECM) of articular cartilage, which prevents compounds conventionally used to lyse cells and extract nucleic acids (such as TRIzol) from accessing tissue-resident chondrocytes and cartilage progenitor cells. As a result, cartilage must be pre-processed before RNA extraction using either harsh homogenization conditions (Le Bleu et al., 2017; Ruettger, Neumann, Wiederanders, & Huber, 2010) or enzymatic digestion methods that isolate chondrocytes from articular cartilage tissues (Lau, Peck, Huang, & Wang, 2015; Yan et al., 2021; Yonenaga et al., 2017). However, homogenization protocols compromise RNA integrity (Ruettger et al., 2010), and the lengthy cell isolation procedures during enzymatic digestion of cartilage ECM may introduce changes in the transcriptome of chondrocytes, which decreases the suitability of such protocols for efficient application of high-sensitivity downstream applications when studying normal cartilage homeostasis. Furthermore, protocols available for RNA extraction from human articular cartilage (Le Bleu et al., 2017) have not been validated for chicken articular cartilage, despite the fact that the chicken has long been used as a model organism to study chondrogenesis (Ahrens, Solursh, & Reiter, 1977; Searls, 1973) and is still widely employed in cartilage research (Jerkovic et al., 2017; Vago et al., 2022).
Here, we report two refined high-efficiency protocols to extract high-quality total RNA from chicken articular cartilage that can overcome the limitations of the existing methodology. Here, we present optimized conditions for pulverizing freshly isolated chicken articular cartilage using a cryogenic mill (see Basic Protocol 1) and for digesting articular cartilage using collagenase type II (see Alternate Protocol). We also describe how extract total RNA suitable for RNA-Seq analysis (see Basic Protocol 2). A refined step-by-step guide to dissecting the chicken knee joint and harvesting articular cartilage slices is also included (see Support Protocol).
CAUTION : Prior to harvesting cartilage slices and beginning the RNA extraction procedure, make sure to carry out RNase decontamination by wiping down all designated lab benches, dissection tools, and pipettes with an RNase decontamination solution.
STRATEGIC PLANNING
Articular cartilage harvesting, mincing, and further processing must be performed in a sterile and RNase-free environment. Before harvesting and beginning the RNA extraction procedure, all designated lab benches, dissection tools, and pipettes should be wiped down with an RNase decontamination solution such as RNaseZap or RNase AWAY. All solutions and equipment coming into contact with articular cartilage must also be sterile and RNase-free. After the animal has been sacrificed (according to local ethical standards and requirements), the legs should be washed using ample tap water and disinfected using 3% H2O2 or 70% ethanol. The 3% H2O2 is also suitable for inhibiting RNase activity.
To prevent significant RNA degradation, the entire procedure must be completed in a reasonably short period of time. For Basic Protocol 1, it is important to prepare the cryogenic mill in advance (i.e., add the required amount of liquid nitrogen to cool the device) so that pulverization can start immediately after cartilage slices have been harvested from the joint. For Basic Protocol 1, it is important to snap-freeze cartilage slices in liquid nitrogen for at least 2 min and then store in a liquid nitrogen tank until use.
For the Alternate Protocol, the chondrocyte isolation procedure is performed under standard cell culture conditions. Medium and solutions coming into contact with harvested cartilage slices must be sterile and always contain both antibiotic and antifungal chemicals.
NOTE : All protocols involving live animals must be reviewed and approved by an Institutional Animal Care and Use Committee (IACUC) and must conform to government regulations for the care and use of laboratory animals.
CAUTION : Appropriate personal protective equipment (PPE) consisting of gloves, facemask, and clean lab coat must be worn at all times when handling fresh tissue and materials.
Basic Protocol 1: EXTRACTION OF TOTAL RNA FROM PULVERIZED CHICKEN ARTICULAR CARTILAGE
Pulverizing articular cartilage in a cryogenic mill has been suggested to provide the most adequate homogenization method to minimize RNA degradation, yield a fairly high RNA concentration, and maintain physiological transcriptomic composition of chondrocytes for downstream analyses including real-time qPCR, microarray analysis, and RNA-Seq (Le Bleu et al., 2017). In this protocol, articular cartilage shavings harvested from 5-week-old broiler knee joints (see Support Protocol) are cryo-pulverized and then processed in 0.1-g portions through a modified TRIzol-based protocol to produce high-quality total RNA. The grinding process using an SPEX SamplePrep 6775 Freezer/Mill is illustrated in Figure 1.
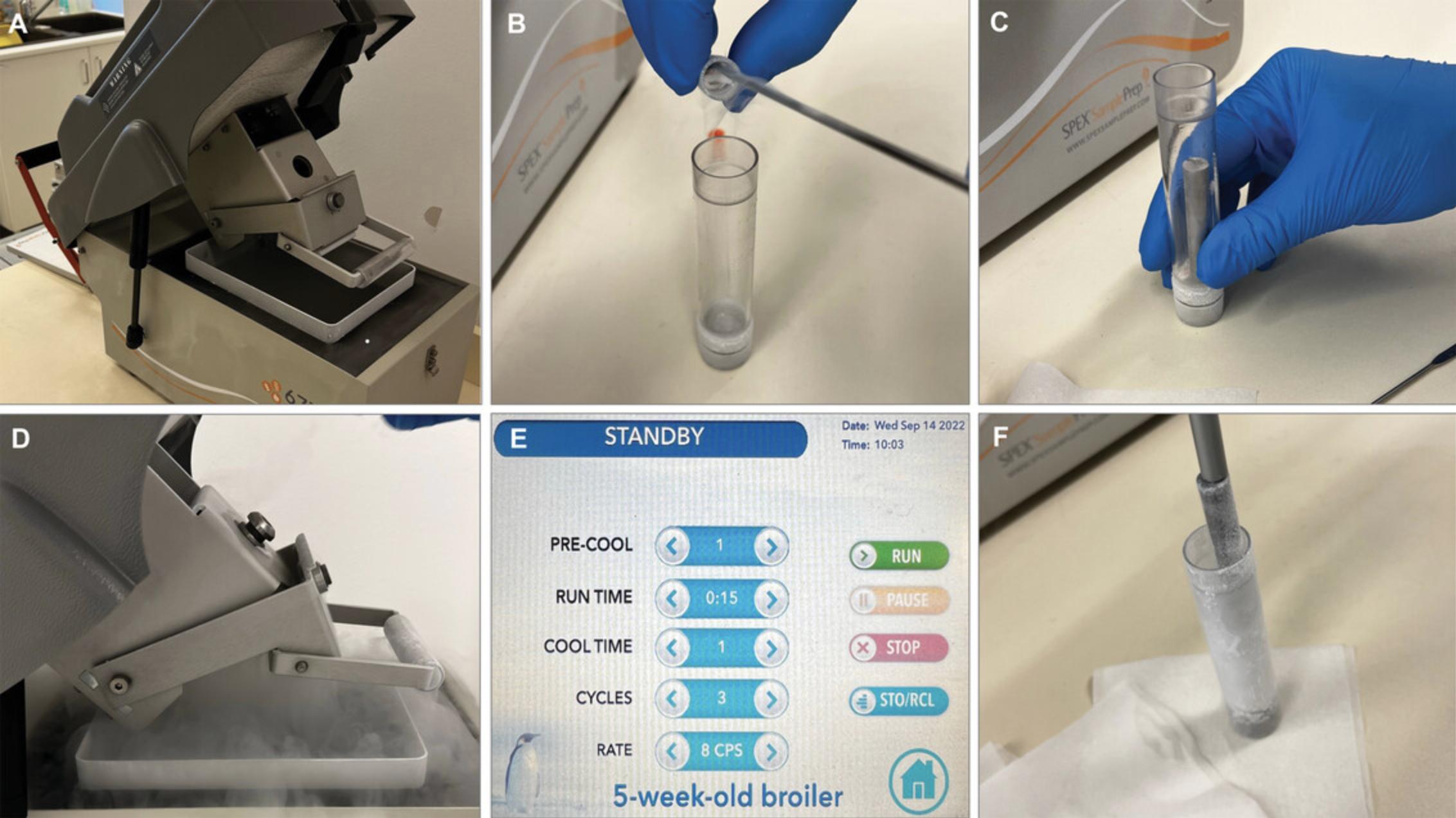
NOTE : All materials, equipment, and solutions coming into contact with cartilage shavings must be sterile and RNase-free. If not sterilized by the manufacturer, materials can be sterilized by autoclaving or in a hot air oven or (in the case of liquids and solutions) by passing through a 0.22-μm filter unit.
Materials
-
Liquid nitrogen
-
Articular cartilage shavings from chicken (see Support Protocol)
-
TRIzol reagent (TRIzolate, UD Genomed, cat. no. URN0103, or equivalent)
-
RNase decontamination solution (RNaseZap or RNase AWAY)
-
Chloroform (Merck, cat. no. 288306, or equivalent)
-
NaCl/NaOAc mixture: 2.4 M sodium chloride and 1.6 M sodium acetate
-
Isopropanol (Merck, cat. no. 278475, or equivalent)
-
Ethanol (Merck, cat. no. 32221-M, or equivalent)
-
Nuclease-free water (Promega, cat. no. P119E, or equivalent)
-
Cryogenic mill (SPEX SamplePrep 6775 Freezer/Mill or equivalent)
-
1.5-ml microcentrifuge tubes (Eppendorf or equivalent)
-
Fume hood
-
Variable-speed mixer (Thermolyne Maxi Mix III 6580 or equivalent)
-
PCR UV cabinet (Biosan UVT-S-AR or equivalent)
Grind tissue
1.Fill the liquid nitrogen container from the cryogenic mill up to the fill line. Set the grinding program to the following parameters (Fig. 1E):
- 1 min pre-cool
- three cycles of 15-s grinding at 8 cycles per second (cps)
- 1 min cool down time
Refer to the manufacturer's instruction manual for specific operational guidelines and details. The manual for the SPEX mill is available at https://static.yanyin.tech/literature/current_protocol/10.1002/cpz1.692/attachments/6775-freezermill-manual.pdf.
The required grinding parameters may vary significantly depending on the species and age (and probably also fitness level) of the animal as well as the specifications of the cryogenic grinder used. This protocol was developed using 5-week-old broilers. The general principle is to allow enough cool down time between cycles and try to keep the frequency to a minimum to ensure optimal brittleness of the samples throughout the protocol.
CAUTION: Liquid nitrogen is an extremely cold material with risks including cold burns, frostbite, permanent eye damage, asphyxiation, and explosion caused by pressure buildup. To minimize risk, always implement prudent safety practices, including handling in well-ventilated areas and handling slowly to minimize splashing. Always wear appropriate PPE, including eye/face and skin protection.
2.Transfer the first batch of articular cartilage shavings (flash-frozen in liquid nitrogen) to the chilled small grinding vial of the mill (the SPEX vial has a sample capacity of 0.1-5.0 g). Run the grinding protocol.
3.Transfer pulverizes tissue in 0.1-g portions (or slightly less, but not more) to 1.5-ml microcentrifuge tubes, place in a fume hood, and proceed immediately to the next step.
Extract RNA
4.Immediately add 1 ml chilled TRIzol to each tube and incubate 15 min at room temperature in a variable-speed mixer.
5.Centrifuge 5 min at 12,000 × g , 4°C, to pellet tissue debris.
6.Transfer tubes to a PCR UV cabinet that has been thoroughly decontaminated with an RNase decontamination solution.
7.Pipette supernatant to new 1.5-ml tubes and add 200 μl chloroform. Shake well for 30 s and leave at room temperature for 3 min.
8.Centrifuge 5 min at 12,000 × g , 4°C, until three phases separate. Transfer the top (aqueous) layer to a new 1.5-ml tube.
9.Use a 1000-μl pipette to estimate the sample volumes and then add 1 volume of NaCl/NaOAc mixture to each tube (final 1.2 M and 0.8 M of each salt, respectively).
10.Add 0.3 volume of 100% isopropanol and incubate 10 min at room temperature to precipitate the RNA.
11.Pellet RNA by centrifuging 10 min at 12,000 × g , 4°C.
12.Wash pellet twice with 1 ml of 75% (v/v) ethanol, centrifuging 10 min at 12,000 × g , 4°C, after each wash.
13.Dry samples for up to 15 min at room temperature.
14.Resuspend each pellet in 50 μl nuclease-free water. To fully resuspend, vortex for 10 s, then centrifuge 10 s at 2500 × g , room temperature. Repeat for a total of three times.
15.Measure RNA concentration and purity using a suitable spectrophotometer (e.g., NanoDrop ND-1000). Store purified RNA up to several weeks at −80°C.
Alternate Protocol: EXTRACTION OF TOTAL RNA FROM COLLAGEN-DIGESTED ARTICULAR CARTILAGE
If a cryogenic mill is not available, an alternate method is to mince the cartilage shavings and then digest them to release chondrocytes from the ECM for RNA extraction. Minced shavings are digested with collagenase type II overnight in a CO2 incubator. The chondrocytes are pelleted by centrifugation, resuspended in TRIzol reagent, and then total RNA is extracted. As an alternative, we also describe how to use the collagenase-digested sample to set up primary chondrocyte cultures.
NOTE : All materials, equipment, and solutions coming into contact with cartilage shavings must be sterile and RNase-free. If not sterilized by the manufacturer, materials can be sterilized by autoclaving or in a hot air oven or (in the case of liquids and solutions) by passing through a 0.22-μm filter unit. The entire protocol, including filtration of the collagenase solution, should be performed in a laminar flow hood suitable for cell culture.
Additional Materials (also see Basic Protocol 1)
- Collagenase type II (Merck, cat. no. C2-28, or equivalent)
- Complete DMEM (see recipe)
- 0.22-μm disposable syringe filter unit (Millex-GS, Merck, cat. no. SLGSV255F, or equivalent)
- 15-ml centrifuge tube (Falcon, Corning, or equivalent)
- Sterile disposable stainless steel scalpels (no. 15)
- Sterile forceps
- 37°C incubator and orbital shaker (Stuart S1505, or equivalent)
Mince and digest tissue
1.Dissolve 100 mg collagenase type II in 8.3 ml complete DMEM (final 1.2% w/v) and pass through a 0.22-μm syringe filter into a 15-ml centrifuge tube.
2.Mince cartilage shavings into small chips (i.e., 1-2 mm) using sterile stainless steel disposable scalpels (no. 15) and a pair of sterile forceps. Transfer to a 1.5-ml microcentrifuge tube (Fig. 2).
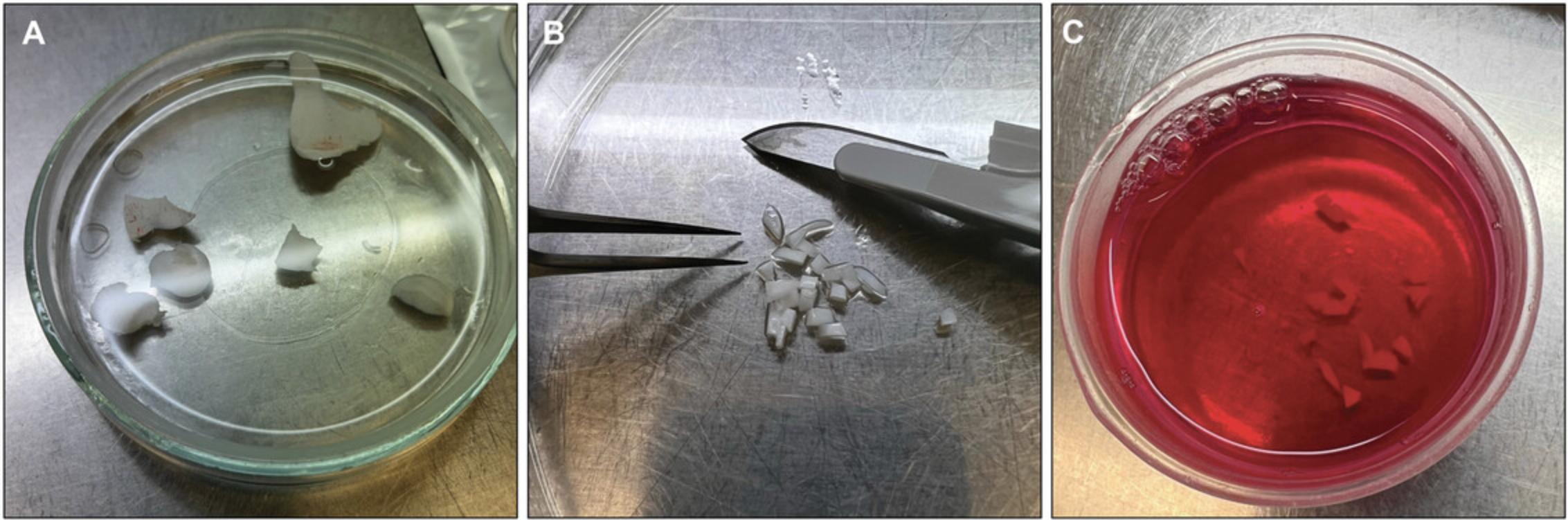
3.Weigh cartilage chips and transfer ∼0.1-g portions into sterile 15-ml centrifuge tubes.
4.Add 3 ml filtered collagenase solution to each centrifuge tube, fasten the caps securely, and incubate overnight (∼16 hr) in a 37°C incubator with an orbital shaker at 150 cycles/min.
Process for RNA extraction
5a. Centrifuge digested tissue for 10 min at 5000 × g , 4°C. Carefully remove the supernatant.
6a. Add 1 ml TRIzol reagent to each pellet and incubate 15 min at room temperature on a rotator.
7a. Proceed with RNA extraction as described (see Basic Protocol 1, step 5).
Process for primary chondrocyte culture
5b. Decant the digested tissue through a 40-μm cell strainer to obtain a single-cell suspension.
6b. Pellet cells by centrifuging 5 min at 300 × g. Carefully remove the supernatant and pool pellets originating from the same joint surface.
7b. Wash pellet twice with high-glucose DMEM without FBS (10 ml).
8b. Resuspend cells in high-glucose DMEM with 10% FBS (∼2 ml per joint).
9b. Add trypan blue and count cells to determine concentration and viability.
Support Protocol: DISSECTION OF CHICKEN ARTICULAR CARTILAGE FROM THE KNEE JOINT
The following steps describe how to obtain articular cartilage shavings from fresh broiler knee joints. With minor modifications, the method can be applied to other medium-sized animals such as rabbit, dog, or cat. The resulting cartilage shavings can be used for total RNA extraction or primary chondrocyte cultures.
Materials
-
Three broilers, 35-38 days old (∼5 weeks) (sourced from local poultry farm)
-
70% (v/v) ethanol
-
3% (v/v) H2O2
-
Liquid nitrogen (∼200 ml) in an uncovered container
-
1× phosphate-buffered saline (PBS; Thermo Fisher Scientific, Gibco, cat. no. 18912014, or equivalent)
-
A few squares of absorbent paper (blue roll paper)
-
Sterile disposable scalpels or scalpel blades (no. 10 or 15)
-
Strong bristled brush
-
Laminar flow hood (suitable for sterile cell culture work)
-
Biological safety cabinet (BSL-1)
-
Sterile metal tray
-
2-ml cryogenic vials (Corning, with orange caps, or equivalent)
-
150-mm glass Petri culture dishes
1.Obtain at least three broilers, ∼5 weeks of age, from a local poultry farm.
2.Sacrifice one animal according to local standard ethical practices.
3.Isolate the lower limbs, starting with incisions in the feathery skin made using a disposable scalpel. Remove the lower limb at the hip joint, and then remove the distal part of the limb at the joint between the tibiotarsus and the tarsometatarsus (the intertarsal joint).
4.Clean the legs by rinsing well with warm tap water.
5.Use a strong bristled brush to remove loosely attached material, then wash the legs with cold tap water to thoroughly remove all remaining traces of dirt from the skin.
6.Disinfect the isolated limbs by spraying liberally with 70% ethanol, then wipe limbs with paper towels moistened with 70% ethanol.
7.Lay limbs in a supine position, i.e., with the anterior aspect of the joint facing upwards. Using a disposable scalpel, make a lengthwise incision starting from the top and dissect away the skin by making a circumferential incision around the area of the limb where the feathers and scales meet. Take care not to pierce the joint capsule of the knee joint.
8.Rinse the skinned limb with water, then with 3% H2O2.
9.Place the skinned limb anterior-side-upward on a clean and sterile metal tray inside a sterile biological safety cabinet (Fig. 3). Flex the limb at the joint.
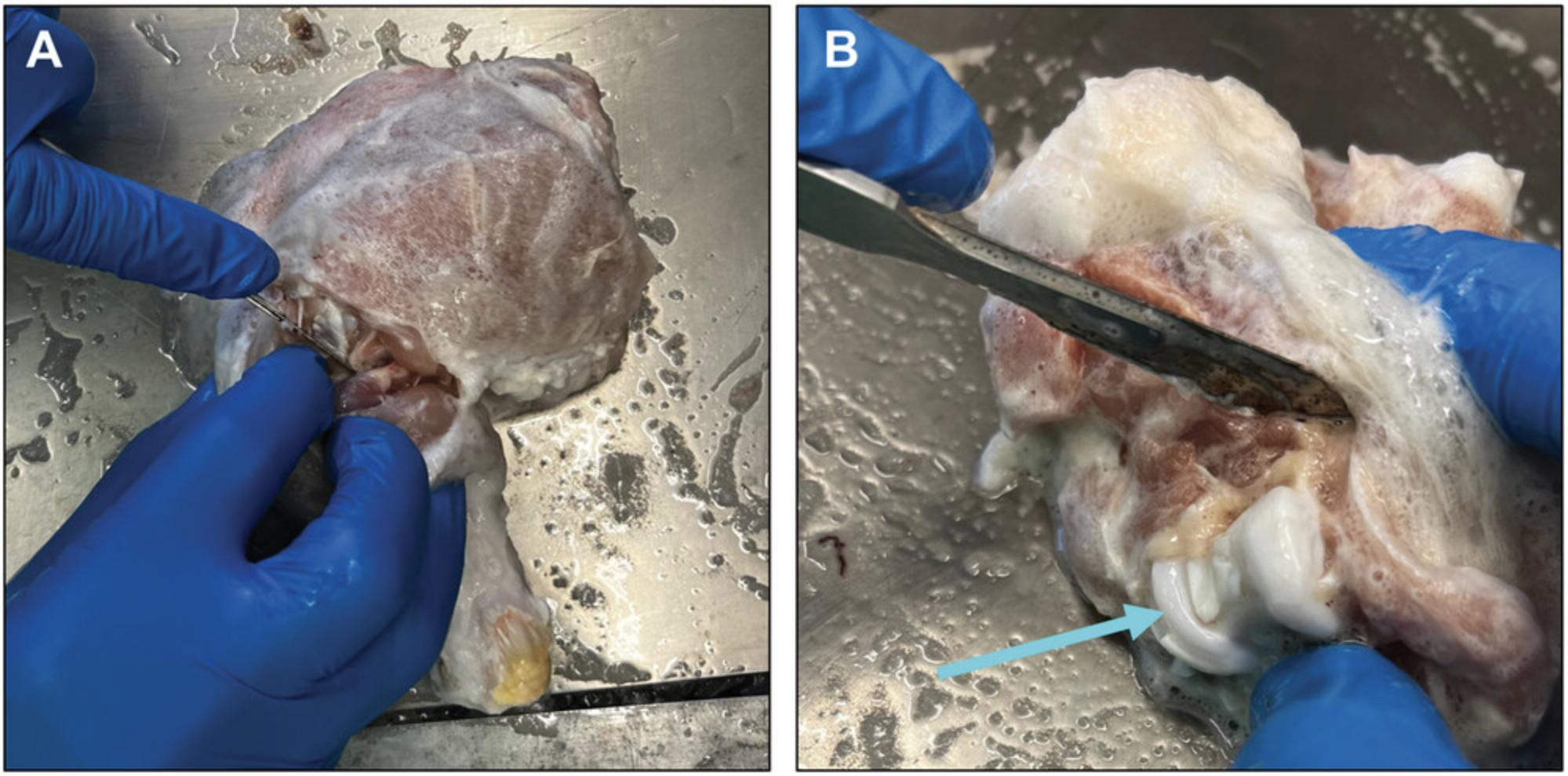
10.Make a longitudinal incision along the anterior or lateral side of the joint capsule, taking care not to cut into the cartilage (Fig. 3). Continue the incision laterally until the joint is exposed on three sides. Carefully open the joint fully by flexing the limb.
11.Obtain thin cartilage shavings from the articular surface of the femur and tibia using a new sterile disposable scalpel. Follow the curved shape of the articular surfaces as closely as possible to obtain shavings of roughly uniform shape and depth (Fig. 2).
12.For pulverization in a cryogenic mill (see Basic Protocol 1), place shavings directly into a cryogenic vial immersed in liquid nitrogen. For enzymatic digestion of cartilage (see Alternate Protocol), transfer shavings to a 150-mm glass Petri dish containing 30 ml 1× PBS.
Basic Protocol 2: RNA SEQUENCING OF TOTAL RNA FROM CHICKEN ARTICULAR CARTILAGE
RNA sequencing (RNA-Seq) is powerful method to obtain global gene expression data of experimental samples using isolated total RNA. RNA-Seq analysis is suitable to determine the number of expressed genes and level of expression and to identify a list of differentially expressed genes between various experimental conditions. This methodology has been widely applied in cartilage research (Hu et al., 2022; Huang et al., 2022; Huynh, Zhang, & Guilak, 2019; Sunkara et al., 2021; Wu et al., 2021). There are three major steps in an RNA-Seq experiment: (1) quality checking of RNA samples, (2) library preparation and sequencing, and (3) analysis and interpretation of sequencing data. Given that detailed protocols are available online as indicated for each kit below, we only very briefly describe the main points for each step, highlighting critical points and giving specific suggestions.
Materials
-
Total RNA samples (see Basic Protocol 1 and Alternate Protocol)
-
RNA 6000 Nano Kit (Agilent Technologies, cat. no. 5067-1511)
-
NEBNext Poly(A) mRNA Magnetic Isolation Module (New England Biolabs, cat. no. E7490)
-
NEBNext Ultra II RNA Library Prep Kit with Sample Purification Beads (New England Biolabs, cat. no. E7775S)
-
DNA 1000 Kit for sizing and quantitation of dsDNA fragments 25-1000 bp (Agilent Technologies, cat. no. 5067 1504)
-
Quant-iT dsDNA Assay Kit, high sensitivity (HS) (Thermo Fisher Scientific, cat. no. Q32854)
-
NextSeq 500/550 High Output Kit v2.5 (75 Cycles) (Illumina, cat. no. 20024906)
-
NEBNext Multiplex Oligos for Illumina (Index Primers, Sets 1-4) (New England Biolabs, cat. nos. E7335S, E7500S, E7710S, and E7730S)
-
2 N NaOH (Merck, cat. no. 72068, or equivalent)
-
200 mM Tris·Cl, pH 7.5 (Trizma hydrochloride, Sigma-Aldrich, cat. no. T5941-500G, dissolved in MilliQ water)
-
2100 Bioanalyzer (Agilent Technologies, cat. no. G2939A, or equivalent)
-
Qubit 4 Fluorometer with wi-fi (Thermo Fisher Scientific, cat. no. Q33238)
-
96-well U-bottom polystyrene microplate (Rahway, cat. no. M2186 Greiner, or equivalent)
-
Magnetic Stand-96 (Thermo Fisher Scientific, cat. no. AM10027)
-
MagnaRack Magnetic Separation Rack (Thermo Fisher Scientific, cat. no. CS15000, or equivalent)
-
200-µl, 8-tube PCR strip tubes (PCR performance tested, transparent, polypropylene, flat cap; Sarstedt, cat. no. 72.991.002, or equivalent)
-
ProFlex 96-well PCR System (Thermo Fisher Scientific, cat. no. 4484075, or equivalent)
-
NextSeq 500 System (Illumina)
QC of RNA samples
Determining the quality/integrity of RNA samples is a critical step because it significantly affects library preparation. RNA integrity correlates with sample degradation and is calculated based on fragment size distribution. Fluorescent labeling of the RNA sample combined with capillary gel electrophoresis is a sensitive method for this purpose. The 2100 Bioanalyzer with the RNA 6000 Nano Kit is a suitable platform that requires only 1 μl input RNA as the sample. The qualitative range of this platform is 5-500 ng/μl and the quantitative range is 25-500 ng/μl. The instrument is controlled by 2100 Expert software. An electropherogram is generated showing the fragments in the RNA sample. Based on the fragment distribution, the software calculates an RNA integrity number (RIN) that ranges from 1 to 10, with the highest value meaning the best quality RNA. The RIN determines the most optimal protocol for library preparation (see step 2 below). The software also calculates a concentration value of the samples using the fluorescent signal intensity levels.
1.Set up the assay equipment and the 2100 Bioanalyzer, decontaminate the electrodes, and prepare the gel and gel-dye mix. Load the gel-dye mix, RNA 6000 Nano Marker, ladder (1 μl), and samples (1 μl) the onto the chip.
2.Insert the chip into the bioanalyzer and start the chip run from the 2100 Expert software screen.
3.Immediately after the run is finished, remove the chip from the bioanalyzer and dispose of it according to good laboratory practices.
4.Analyze the sample well results.
Alternative options for QC
If only a small amount of input RNA is available, the more sensitive RNA 6000 Pico Kit can be used (qualitative range 50-5000 pg/μl). Other types of instruments that can be used to determine fragment size distribution include TapeStation Instruments and the Agilent Fragment Analyzer System.
Library preparation
There are several protocols for library preparation based on input RNA sample quality and the aim of the study. The first crucial step is to remove rRNA. rRNA comprises ∼80%-90% of total RNA and its expression levels are not usually of interest. If the input RNA is of good quality (RIN > 6), a poly(A) RNA selection protocol should be used. This employs oligo(dT)-conjugated magnetic beads that bind the poly(A) tail of mature mRNA, effectively removing other RNA species (rRNA, pre-mRNA, and non-coding RNA) from the total RNA sample. The NEBNext Poly(A) mRNA Magnetic Isolation Module should be used to enrich the full-length mature mRNA population. Library preparation is then performed using the NEBNext Ultra II RNA Library Prep Kit with Sample Purification Beads. In general, the manufacturer's protocol should be followed (https://international.neb.com/protocols/2017/02/05/protocol-for-use-with-nebnext-poly-a-mrna-magnetic-isolation-module-e7490-and-nebnext-ultra-ii-di). We provide some specifications and modifications below.
1.Start the mRNA isolation, fragmentation, and priming from total RNA.
2.During poly(A) RNA selection, transfer the mixture of RNA sample and oligo(dT)-conjugated magnetic beads to a 96-well U-bottom plate and place on a Magnetic Stand-96 to capture the beads.
3.During library preparation for magnetic separation, use NEBNext Sample Purification Beads (part of the kit) and perform separation in a 96-well U-bottom plate and a Magnetic Stand-96.
4.Before adaptor ligation, make a 25-fold dilution of NEBNext Adaptor in Adaptor Dilution Buffer (both parts of the kit).
5.Use 10 PCR cycles in the step for PCR enrichment of adaptor-ligated DNA.
6.Run 1 μl library on the 2100 Bioanalyzer using a DNA 1000 Kit to determine fragment size distribution, average fragment size, and concentration of the library.
7.Measure concentration on a Qubit fluorometer using the Quant-iT dsDNA Assay Kit HS kit.
Alternative options for library preparation
Poly(A) selection cannot be used for partially or highly degraded RNA samples, as the degradation causes fragmentation of mRNA, which will disconnect some fragments from their poly(A) tail and lead to overrepresentation of the 3′ ends of transcripts in the sequencing results. For such samples, an rRNA depletion protocol is one option. In the first step, use the NEBNext rRNA Depletion Kit v2 (Human/Mouse/Rat, cat. no. E7405) to remove rRNA species. Using this method, pre-mRNAs and non-coding RNAs can be examined in addition to mature poly(A) mRNAs. The efficiency of rRNA depletion is not always 100%, however, so some rRNA contamination can still be detected during sequencing. Furthermore, intronic sequence reads can be present because of the pre-mRNA content. 3′ end sequencing is also an option for generating a library from highly degraded RNA samples. In this case, only the 3′ ends will be represented in the sequencing and not the full-length transcripts.
Sequencing
Sequencing is performed on an Illumina NextSeq 500 instrument using single read with a 75-bp read length. The preparation steps for sequencing are as follows:
Library normalization and pooling
1.Use the following equation to calculate the nanomolar concentration of the libraries based on the average fragment size (determined by the bioanalyzer) and the concentration in ng/μl (measured by Qubit fluorometer):
2.Dilute each library to 4 nM and pool equal volumes of the normalized libraries together in a microcentrifuge tube.
Library denaturation and loading
3.Follow the Illumina NextSeq 500 denaturation protocol. Briefly, denature 5 μl of 4 nM library pool with 5 μl freshly prepared 0.2 N NaOH for 5 min at room temperature. Stop denaturation by adding 5 μl of 200 mM Tris·Cl, pH 7, and mixing thoroughly. Add 985 μl of HT1 buffer (resulting in 1 ml of 20 pM denatured library). For loading, add 1180 μl of HT1 buffer to 117 μl of 20 pM denatured library (yielding 1.3 ml of 1.8 pM library) and pipette this solution into the sequencing cartridge.
4.Define sequencing parameters with sample name and indexed barcode information at BaseSpace (https://basespace.illumina.com/dashboard), the cloud-based bioinformatics solution of the Illumina platform. Connect the instrument to BaseSpace and start the sequence run.
Analysis and interpretation of sequencing data
Primary data analysis
Download fastq files from BaseSpace and transfer to the local computer cluster. Employ FastQC for quality control of raw sequencing data (https://www.bioinformatics.babraham.ac.uk/projects/fastqc/).
Generate aligned data BAM files using the HISAT2 algorithm combined with SAMtools (Kim, Paggi, Park, Bennett, & Salzberg, 2019; Li et al., 2009) (see script below). Align raw sequencing data to the Gallus gallus reference genome, version GRCg6a (https://www.ncbi.nlm.nih.gov/assembly/GCF_000002315.6).
mkBAM_hisat2 script:
- echo $INDEX
- for file in ${DIR}/*fastq.gz
- do
- NAME=
basename $file fastq.gz
- hisat2 -k1 -p
{INDEX} -U {NAME}.sam - samtools view -Sb -@
{NAME}.sam > ${NAME}.bam - samtools sort -@
{NAME}_sort.bam ${NAME}.bam - samtools index ${NAME}_sort.bam
- rm -f
{NAME}.bam - done
Downstream analysis
For detailed analysis, use StrandNGS software (https://www.strand-ngs.com/). Normalize BAM files by the Deseq algorithm of the software, then determine differentially expressed genes (DEGs) by ANOVA test with Tukey post hoc test and Benjamini-Hochberg false discovery rate (FDR) for multiple testing correction.
REAGENTS AND SOLUTIONS
Complete DMEM
- 88 ml high-glucose DMEM (Thermo Fisher Scientific, Gibco, cat. no. 10564011)
- 10 ml fetal bovine serum (Thermo Fisher Scientific, Gibco cat. no. 26140)
- 1 ml 100× penicillin/streptomycin solution (Biowest, cat. no. L0022-100)
- 80 μg/ml gentamicin (40 mg/ml gentamicin sulfate solution for injection, Danhson)
- 1.25 μg/ml amphotericin B (Fungizone, Bristol-Myers Squibb)
- 1 ml 100× (200 mM) l-glutamine (Biowest, cat. no. X0550-100)
- Prepare in a sterile laminar flow hood
- Store up to 1 month at 4°C
COMMENTARY
Background Information
Articular hyaline cartilage that covers the epiphyses of bones in synovial joints is a tough, resilient, yet flexible connective tissue that enables the transmission of forces within joints. The biomechanical properties of articular cartilage are attributable to the unique composition of its ECM, which is composed of collagens (primarily type II) and proteoglycans (PGs) with glycosaminoglycan (GAG) chains that function to resist tensile and compressive loads, respectively. Cartilage has a very low cellularity, with chondrocytes (which originate from chondroblasts derived from mesenchymal cells) constituting ∼2% of the total volume of articular cartilage (Fox, Bedi, & Rodeo, 2009).
RNA extraction from this abundant ECM containing a high proportion of aggregating PGs poses a significant problem because both nucleic acids and the sulfated GAG components of PGs are extremely large and highly negatively charged, so that these structural ECM components co-purify with the RNA. Historically, methodology for RNA extraction from cartilage has focused on separation of nucleic acids from contaminant PGs. These older methodologies rely on the use of concentrated denaturing solutions containing guanidinium isothiocyanate followed by ultracentrifugation in cesium trifluoroacetate (Adams, Huang, Yao, & Sandell, 1992; Smale & Sasse, 1992). Another approach is pre-treating cartilage with proteinase K in guanidinium isothiocyanate prior to cesium trifluoroacetate ultracentrifugation to increase the yield and purity of RNA extracted from cartilage matrix (Mallein-Gerin & Gouttenoire, 2004). However, such approaches are rarely used with the availability of more user-friendly mini spin column kits. A more efficient way of extracting high-quality total RNA is to first pulverize cartilage chips using a cryogenic mill (Le Bleu et al., 2017) or a Mikro-Dismembrator (Zheng & McAlinden, 2021). The yield and quality of extracted RNA can further be improved by adding 1.2 M NaCl and 0.8 M NaOAc to the aqueous layer prior to RNA precipitation in the conventional TRIzol protocol (Le Bleu et al., 2017). This method generates RNA samples with impressive RIN values of ∼8 as determined by bioanalysis. Alternatively, combining the TRIzol protocol with spin column chromatography (Norgen Total RNA Purification Kit) has generated total RNA extracts with an average RIN value of ∼7 (Zheng & McAlinden, 2021).
Another approach for obtaining high-quality RNA from chondrocytes derived from articular hyaline cartilage is to remove the ECM PGs prior to RNA extraction using enzymatic digestion. Such methods generally yield better quality RNA, but it should be noted that chondrocytes remain alive during the usually lengthy (overnight) digestion necessary to break down the collagenous ECM, so the procedure likely induces cellular responses that alter transcriptional patterns. Nearly every protocol developed for enzymatic digestion of articular cartilage (including the one described here) employs collagenase to liberate chondrocytes. Collagenases are members of the matrix metalloproteinase family. Type II collagenase (used here) is an enzyme mixture isolated from Clostridium histolyticum (Chen & James, 2001). Other chondrocyte isolation methods rely on pronase or trypsin to partially degrade the ECM before collagenase digestion (Hayman, Blumberg, Scott, & Athanasiou, 2006). Indeed, alterations in mRNA expression patterns have been reported in total RNA isolated from cartilage versus isolated chondrocytes (Hayman et al., 2006). However, since a high number of in vitro studies have been performed on isolated chondrocytes (Diaz-Romero et al., 2005; Loeser, Pacione, & Chubinskaya, 2003), this should be the protocol of choice when comparing RNA expression profiles of chondrocytes cultured in vitro to chondrocytes in “intact” cartilage.
To conclude, two reliable and reproducible protocols, developed by modifying existing procedures, for isolation of high-quality total RNA from chicken articular cartilage are described here. The purified total RNA is suitable for gene expression studies by RNA-Seq analysis.
Critical Parameters and Troubleshooting
In our experience, two external factors have the strongest influence on RNA quality: RNase contamination and time. To minimize contamination with RNases, make sure to carefully decontaminate the chicken legs. Since broiler feet are usually covered in mud and farm detritus, they must first undergo a general rinse in warm tap water. The legs are then washed with cold tap water to thoroughly remove all remaining traces of dirt from the skin. The isolated limbs are disinfected by liberally spraying with 70% ethanol and finally wiped with paper towels moistened with 70% ethanol.
Prior to harvesting cartilage slices and beginning the RNA extraction, all designated lab benches, dissection tools, and pipettes should be wiped down with an RNase decontamination solution such as RNaseZap or RNase AWAY). A 3% H2O2 solution may also be suitable to inhibit RNase activity. All solutions and equipment coming into contact with articular cartilage must be sterile and RNase-free. Appropriate PPE consisting of gloves, facemask, and clean lab coat must be worn at all times when handling tissue and materials. Articular cartilage harvesting, mincing, and further processing must be performed in an RNase-free environment.
Table 1 contains useful tips for troubleshooting the RNA extraction.
Problem | Possible cause | Solution |
---|---|---|
Low yield | Cartilage shavings too small. In general, immature, developing cartilage samples have a higher cell content than fully developed cartilage tissues. Therefore, one should expect an overall lower RNA yield from more mature hypocellular articular cartilage. | Harvest more shavings from the articular surfaces, being careful not to cut too deep (check for signs of oozing blood or exposed subchondral vasculature) |
Degraded RNA (low RIN) | RNase contamination | More thorough RNase decontamination using RNaseZap, RNase AWAY, or 3% H2O2 |
Too much time processing tissue | Speed up processing time | |
Overly harsh pulverization | Adjust pulverization parameters (depending on the equipment used) | |
Contamination (low A260/A230) | High A230 values relative to A260 commonly indicate contamination of TRIzol or lysis buffer. For cartilage samples, low A260/A230 values may also be due to proteoglycan contamination. | To eliminate proteoglycans, try adding 1.2 M NaCl/0.8 M NaOAc to the aqueous layer prior to RNA precipitation |
If the low A260/A230 is not due to proteoglycan contamination, try washing the precipitated RNA pellet once more with ethanol and/or letting it dry more thoroughly before resuspending it in nuclease-free water |
Understanding Results
The quality (RIN) of total RNA isolated from broiler knee articular cartilage using Basic Protocol 1 was ∼6.8 (average RIN = 6.5, n = 3, Table 2, Fig. 4), which is in the same realm of a recently published protocol (Zheng & McAlinden, 2021) employing a Mikro-Dismembrator to pulverize cartilage prior to RNA extraction using TRIzol and a Norgen kit. We were unable to replicate the very impressive RIN values of 7.9 and 8.4 reported by Le Bleu et al. (2017). In that study, the TRIzol method was used on pulverized cartilage, but was modified from the conventional protocol by the addition of high salt to the aqueous layer prior to RNA precipitation, yielding samples with an average A 260/A 280 ratio of 1.8 ± 0.11 and an average A 260/A 230 ratio of 1.9 ± 0.23. Our RNA samples were inferior in terms of A 260/A 230 (average 0.81 ± 0.37 from pulverized cartilage; 1.46 ± 0.13 collagenase-digested cartilage), but superior in terms of A 260/A 280 (average 2.05 ± 0.02 for pulverized cartilage; 2.05 ± 0.07 for collagenase-digested cartilage). Our data are more comparable with the results of Zheng & McAlinden (2021), who also observed lower A 260/A 230 ratios (with very high variability between parallel samples). Nevertheless, total RNA samples with RIN values of ∼6 (or higher) are generally considered to be suitable for RNA-Seq. Indeed, samples prepared from according to Basic Protocol 1 resulted in an average of ∼87% NGS coverage depth when processed according to Basic Protocol 2 for RNA sequencing (Table 2). RNA samples generated using the Alternate Protocol were even better in terms of RIN values (less degradation), with the best sample having a RIN of 7.7 and an average of 7.6 (n = 3, Table 2, Fig. 4). These total RNA samples resulted in a superior NGS coverage depth of over 90% (Table 2).
Method | Sample | Concentration (ng/µl) | A260/A230 | A260/A280 | RIN | Mapped reads (%) |
---|---|---|---|---|---|---|
Pulverized cartilage | 1 | 459.19 | 0.93 | 2.03 | 6.80 | 87.0% |
2 | 444.05 | 1.11 | 2.05 | 6.00 | 86.9% | |
3 | 138.36 | 0.39 | 2.07 | 6.80 | 86.8% | |
Digested cartilage | 1 | 272.30 | 1.59 | 2.05 | 7.60 | 89.1% |
2 | 516.18 | 1.34 | 2.12 | 7.40 | 90.1% | |
3 | 1589.23 | 1.45 | 1.99 | 7.70 | 90.9% |
- a Concentration and absorbance ratios determined using a NanoDrop ND-1000. RIN determined using an Agilent 2100 Bioanalyzer. Mapped reads determined using the HISAT2 algorithm combined with SAMtools.
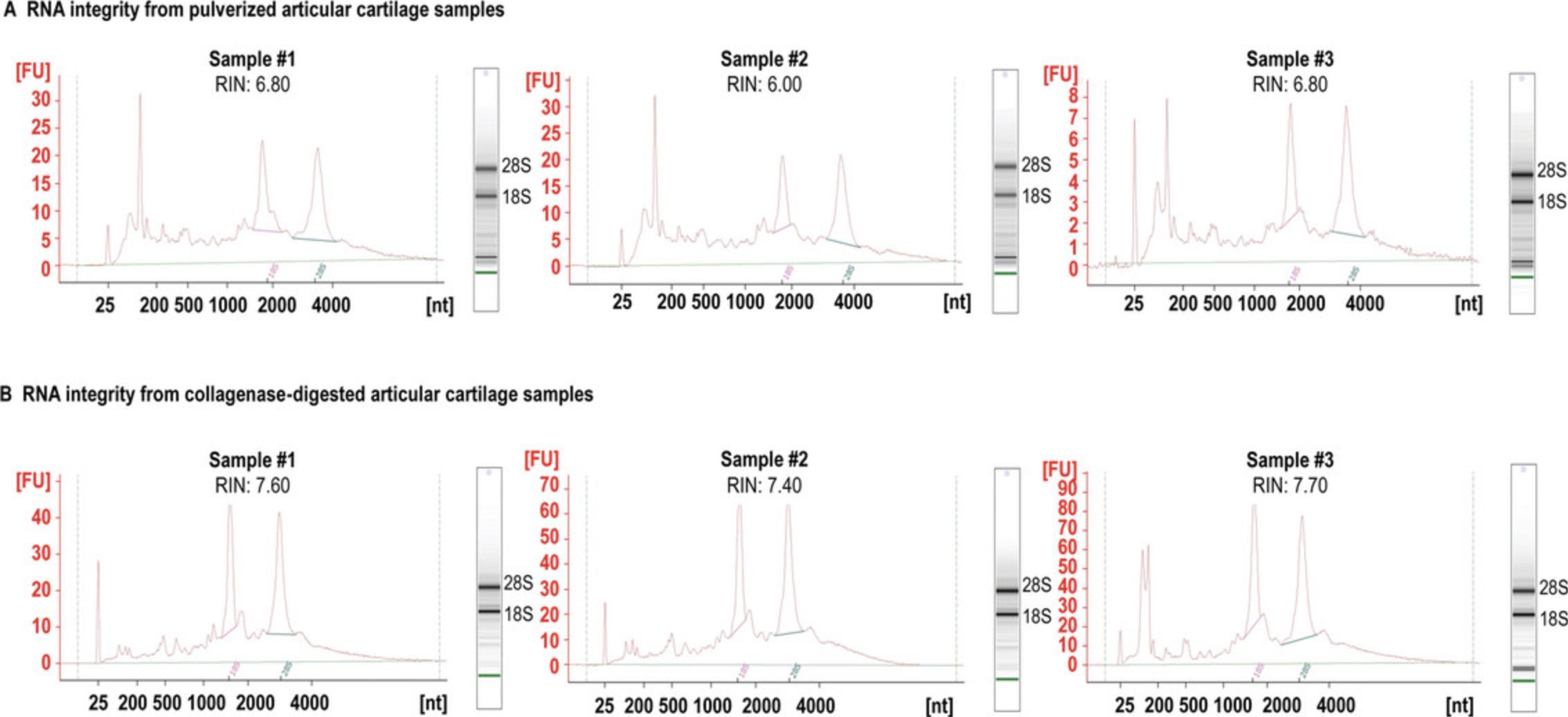
Time Considerations
To prevent RNA degradation, the entire procedure must be completed in a reasonably short time period. The preparation time (preparation of solutions, disinfecting laboratory surfaces, etc.) is ∼2 hr. Dissection of articular cartilage shavings from knee joints takes ∼2 hr, including sacrificing the animals). Generating pulverized cartilage requires ∼1 hr (including preparation time, i.e., pre-cooling the cryogenic grinder with liquid nitrogen), at which point samples can be frozen and stored in liquid nitrogen. After that, an additional ∼3 hr are needed for RNA extraction. Preparations for the collagenase type II digest take ∼1 hr on the first day, an overnight incubation (∼16 hr), and an additional ∼3 hr on the second day for RNA extraction. RNA-Seq and data analysis take ∼2 days, with multiple points at which the procedure can be suspended.
Acknowledgments
C.M. was supported by the Young Researcher Excellence Programme (grant number FK-134304) of the National Research, Development, and Innovation Office, Hungary. Project no. TKP2020-NKA-04 was implemented with support from the National Research, Development and Innovation Fund of Hungary, financed under the 2020-4.1.1-TKP2020 funding scheme. S.P. was supported by the Thematic Excellence Program (grant number TKP2021-NKTA-34) of the University of Debrecen. We are exceptionally grateful to Ms. Éva Szabó, Mrs. Ilona Huszár-Újvári Istvánné, and especially Mrs. Katalin Váriné Debrecen (Katika Néni) for their kind assistance in handling and sacrificing the animals. We also thank Mr. Ferenc Henrik from Kelet-Grain Kft. poultry farm in Hajdúböszörmény, Hungary for providing us with broilers for this work. Ethics License Number: HB/15-ÉLB/03743-2/2022.
Author Contributions
Roland Takács : Conceptualization, investigation, methodology, writing (original draft, review and editing); Szilárd Póliska : data curation, formal analysis, funding acquisition, writing (original draft, review and editing); Tamás Juhász : investigation, writing (review and editing); Krisztina B. Barna : investigation, methodology; Csaba Matta : conceptualization, funding acquisition, methodology, supervision, writing (original draft, review and editing).
Conflict of Interest
The authors declare no conflicts of interest. This paper was written by the authors within the scope of their academic and research positions. None of the authors have any relationships that could be construed as biased or inappropriate. The funding bodies were not involved in the study design, data collection, analysis, or interpretation. The decision to submit the paper for publication was not influenced by any funding bodies.
Open Research
Data Availability Statement
Data sharing is not applicable to this article as no datasets were generated or analyzed during the current study.
Literature Cited
- Adams, M. E., Huang, D. Q., Yao, L. Y., & Sandell, L. J. (1992). Extraction and isolation of mRNA from adult articular cartilage. Analytical Biochemistry , 202(1), 89–95. doi: 10.1016/0003-2697(92)90211-o
- Ahrens, P. B., Solursh, M., & Reiter, R. S. (1977). Stage-related capacity for limb chondrogenesis in cell culture. Developmental Biology , 60(1), 69–82. doi: 10.1016/0012-1606(77)90110-5
- Chen, R. L., & James, R. F. (2001). Characterization of an important enzymatic component in collagenase that is essential for the effective digestion of the human and porcine pancreas. Cell Transplantation , 10(8), 709–716.
- Diaz-Romero, J., Gaillard, J. P., Grogan, S. P., Nesic, D., Trub, T., & Mainil-Varlet, P. (2005). Immunophenotypic analysis of human articular chondrocytes: Changes in surface markers associated with cell expansion in monolayer culture. Journal of Cellular Physiology , 202(3), 731–742. doi: 10.1002/jcp.20164
- Fox, A. J. S., Bedi, A., & Rodeo, S. A. (2009). The basic science of articular cartilage: Structure, composition, and function. Sports Health , 1(6), 461–468. doi: 10.1177/1941738109350438
- Hayman, D. M., Blumberg, T. J., Scott, C. C., & Athanasiou, K. A. (2006). The effects of isolation on chondrocyte gene expression. Tissue Engineering , 12(9), 2573–2581. doi: 10.1089/ten.2006.12.2573
- Hu, X., Li, Z., Ji, M., Lin, Y., Chen, Y., & Lu, J. (2022). Identification of cellular heterogeneity and immunogenicity of chondrocytes via single-cell RNA sequencing technique in human osteoarthritis. Frontiers in Pharmacology , 13, 1004766. doi: 10.3389/fphar.2022.1004766
- Huang, Z. Y., Luo, Z. Y., Cai, Y. R., Chou, C. H., Yao, M. L., Pei, F. X., … Zhou, Z. K. (2022). Single cell transcriptomics in human osteoarthritis synovium and in silico deconvoluted bulk RNA sequencing. Osteoarthritis and Cartilage , 30(3), 475–480. doi: 10.1016/j.joca.2021.12.007
- Hunter, D. J., & Bierma-Zeinstra, S. (2019). Osteoarthritis. Lancet , 393(10182), 1745–1759. doi: 10.1016/S0140-6736(19)30417-9
- Huynh, N. P. T., Zhang, B., & Guilak, F. (2019). High-depth transcriptomic profiling reveals the temporal gene signature of human mesenchymal stem cells during chondrogenesis. FASEB Journal , 33(1), 358–372. doi: 10.1096/fj.201800534R
- Jerkovic, I., Ibrahim, D. M., Andrey, G., Haas, S., Hansen, P., Janetzki, C., … Mundlos, S. (2017). Genome-wide binding of posterior HOXA/D transcription factors reveals subgrouping and association with CTCF. PLoS Genetics , 13(1), e1006567. doi: 10.1371/journal.pgen.1006567
- Kim, D., Paggi, J. M., Park, C., Bennett, C., & Salzberg, S. L. (2019). Graph-based genome alignment and genotyping with HISAT2 and HISAT-genotype. Nature Biotechnology , 37(8), 907–915. doi: 10.1038/s41587-019-0201-4
- Lau, T. T., Peck, Y., Huang, W., & Wang, D. A. (2015). Optimization of chondrocyte isolation and phenotype characterization for cartilage tissue engineering. Tissue Engineering Part C: Methods , 21(2), 105–111. doi: 10.1089/ten.TEC.2014.0159
- Le Bleu, H. K., Kamal, F. A., Kelly, M., Ketz, J. P., Zuscik, M. J., & Elbarbary, R. A. (2017). Extraction of high-quality RNA from human articular cartilage. Analytical Biochemistry , 518, 134–138. doi: 10.1016/j.ab.2016.11.018
- Li, H., Handsaker, B., Wysoker, A., Fennell, T., Ruan, J., Homer, N., … 1000 Genome Project Data Processing Subgroup. (2009). The sequence alignment/map format and SAMtools. Bioinformatics , 25(16), 2078–2079. doi: 10.1093/bioinformatics/btp352
- Liebman, J., & Goldberg, R. L. (2001). Chondrocyte culture and assay. Current Protocols in Pharmacology , 12, 12.2.1–12.2.18. doi: 10.1002/0471141755.ph1202s12
- Loeser, R. F., Pacione, C. A., & Chubinskaya, S. (2003). The combination of insulin-like growth factor 1 and osteogenic protein 1 promotes increased survival of and matrix synthesis by normal and osteoarthritic human articular chondrocytes. Arthritis and Rheumatism , 48(8), 2188–2196. doi: 10.1002/art.11209
- Mallein-Gerin, F., & Gouttenoire, J. (2004). RNA extraction from cartilage. Methods in Molecular Medicine , 100, 101–104. doi: 10.1385/1-59259-810-2:101
- Oo, W. M., Little, C., Duong, V., & Hunter, D. J. (2021). The development of disease-modifying therapies for osteoarthritis (DMOADs): The evidence to date. Drug Design , Development and Therapy , 15, 2921–2945. doi: 10.2147/DDDT.S295224
- Ruettger, A., Neumann, S., Wiederanders, B., & Huber, R. (2010). Comparison of different methods for preparation and characterization of total RNA from cartilage samples to uncover osteoarthritis in vivo. BMC Research Notes , 3, 7. doi: 10.1186/1756-0500-3-7
- Searls, R. L. (1973). Newer knowledge of chondrogenesis. Clinical Orthopaedics and Related Research , 96, 327–344. doi: 10.1097/00003086-197310000-00043
- Smale, G., & Sasse, J. (1992). RNA isolation from cartilage using density gradient centrifugation in cesium trifluoroacetate: An RNA preparation technique effective in the presence of high proteoglycan content. Analytical Biochemistry , 203(2), 352–356. doi: 10.1016/0003-2697(92)90324-z
- Sunkara, V., Heinz, G. A., Heinrich, F. F., Durek, P., Mobasheri, A., Mashreghi, M. F., & Lang, A. (2021). Combining segmental bulk- and single-cell RNA-sequencing to define the chondrocyte gene expression signature in the murine knee joint. Osteoarthritis and Cartilage , 29(6), 905–914. doi: 10.1016/j.joca.2021.03.007
- Vago, J., Katona, E., Takacs, R., Docs, K., Hajdu, T., Kovacs, P., … Matta, C. (2022). Cyclic uniaxial mechanical load enhances chondrogenesis through entraining the molecular circadian clock. Journal of Pineal Research , 73(4), e12827. doi: 10.1111/jpi.12827
- Wu, C. L., Dicks, A., Steward, N., Tang, R., Katz, D. B., Choi, Y. R., & Guilak, F. (2021). Single cell transcriptomic analysis of human pluripotent stem cell chondrogenesis. Nature Communications , 12(1), 362. doi: 10.1038/s41467-020-20598-y
- Yan, Y., Fu, R., Liu, C., Yang, J., Li, Q., & Huang, R. L. (2021). Sequential enzymatic digestion of different cartilage tissues: A rapid and high-efficiency protocol for chondrocyte isolation, and its application in cartilage tissue engineering. Cartilage , 13(2_suppl), 1064S–1076S. doi: 10.1177/19476035211057242
- Yonenaga, K., Nishizawa, S., Nakagawa, T., Fujihara, Y., Asawa, Y., Hikita, A., … Hoshi, K. (2017). Optimal conditions of collagenase treatment for isolation of articular chondrocytes from aged human tissues. Regenerative Therapy , 6, 9–14. doi: 10.1016/j.reth.2016.08.001
- Zheng, H., & McAlinden, A. (2021). RNA isolation from articular cartilage tissue. Methods in Molecular Biology , 2245, 121–133. doi: 10.1007/978-1-0716-1119-7_9
Key References
- Le Bleu et al. (2017). See above.
Provides key details regarding optimization of high-quality RNA extraction from human articular cartilage following pulverization of cartilage shavings using a cryogenic mill.
- Zheng, & McAlinden (2021). See above.
Provides an updated protocol for extraction of high-quality RNA from human articular cartilage using TRIzol and a Norgen kit following pulverization in a Mikro-Dismembrator.
Citing Literature
Number of times cited according to CrossRef: 3
- Takashi Taguchi, Mandi Lopez, Catherine Takawira, Viable tendon neotissue from adult adipose-derived multipotent stromal cells, Frontiers in Bioengineering and Biotechnology, 10.3389/fbioe.2023.1290693, 11 , (2024).
- Xuanze Ling, Qifan Wang, Pengfei Wu, Kaizhi Zhou, Jin Zhang, Genxi Zhang, Exploration of Potential Target Genes of miR-24-3p in Chicken Myoblasts by Transcriptome Sequencing Analysis, Genes, 10.3390/genes14091764, 14 , 9, (1764), (2023).
- Roland Takács, Judit Vágó, Szilárd Póliska, Peter Natesan Pushparaj, László Ducza, Patrik Kovács, Eun-Jung Jin, Richard Barrett-Jolley, Róza Zákány, Csaba Matta, The temporal transcriptomic signature of cartilage formation, Nucleic Acids Research, 10.1093/nar/gkad210, 51 , 8, (3590-3617), (2023).