Human Dendritic Cell Enrichment and Their Activation of T Cells
Ruth Lubin, Ruth Lubin, Rotem Gvili, Rotem Gvili, Idit Hazan, Idit Hazan, Simon Yona, Simon Yona
Abstract
Dendritic cells (DCs) enable the immune system to mount and modulate precisely targeted responses to various threats across the organism by bridging innate and adaptive immunity. Historically, DCs have been classified as conventional (cDC) and plasmacytoid (pDC). More recently, cDCs were acknowledged as a heterogenous population composed of several subsets. Examining the functional diversity of cDCs in healthy homeostasis and pathology requires a robust experimental pipeline, beginning with an efficient enrichment protocol in preparation for cell sorting. Unfortunately, several commercial DC enrichment kits were developed before the discovery of the more recently described DC populations. Here, we detail two approaches to enrich human blood DCs or certain DC subsets and an in vitro protocol to examine DC stimulation of naïve T cells. The methods employed here overcome many hurdles encountered while enriching human DC subsets. Basic Protocol 1 describes a method that will enrich pDC, Axl Siglec6-DC (AS-DC), cDC1, DC2, DC3, monocytes, and human HLA+ cells by crosslinking unwanted cells to erythrocytes. Basic Protocol 2 describes the enrichment of pDC, AS-DC, cDC1, and DC2 but not DC3 via a highly efficient negative magnetic selection that is valuable in circumstances where DC3 is not required. Finally, Basic Protocol 3 describes a conventional protocol to perform a Mixed leucocyte Reaction (MLR) following the isolation of these DC subsets. These methods detail the advantages and pitfalls when isolating a heterogeneous population of cells. © 2023 The Authors. Current Protocols published by Wiley Periodicals LLC.
Basic Protocol 1 : Human peripheral mononuclear phagocyte enrichment
Basic Protocol 2 : DC enrichment of pDC, cDC1, AS-DC, and DC2 but not DC3
Basic Protocol 3 : Basic mixed lymphocyte reaction protocol with sorted human DC subsets
INTRODUCTION
In his ground-breaking work on graft rejection, Peter Medawar revealed that mammalian organisms could distinguish between self and non-self by accepting autologous skin grafts while rejecting allogeneic grafts (Billingham et al., 1951; Billington, 2003; McDevitt, 2000). This rate of tissue rejection was accelerated following a second graft from the same unrelated donor compared to a second graft from a different individual, revealing the existence of immunological memory. Two decades later, Doherty and Zinkernagel established that T cells recognize viral antigens presented by class I haploidentical recipients, suggesting that T cell receptors required simultaneous self-recognition of the MHC in conjunction with foreign antigens, known as MHC restriction (Zinkernagel & Doherty, 1974). Several studies at the time indicated the existence of an “ accessory cell ” that prompts T-cell proliferation (Bach & Hirschhorn, 1964; Bain et al., 1964). In 1973, Steinman and Cohn identified a rare population of cells with a tree-like morphology, termed dendritic cells (DC: dendron meaning “tree” in Greek). The DC has the unrivaled capability to prime naïve T cells in the mixed leucocyte reaction (Steinman & Cohn, 1973; Steinman & Witmer, 1978).
Later studies revealed DC to be bone marrow-derived members of the mononuclear phagocyte system, found in tissues throughout the body, particularly at barrier sites, lymph nodes, and the spleen (Tussiwand et al., 2023) (Guilliams et al., 2018). DCs act as sentinel antigen-presenting cells that capture and process antigens (foreign or malignant substances) and present them to T cells to initiate an adaptive immune response. A unique population of DCs, plasmacytoid DCs (pDC), are characterized by their ability to produce large volumes of type I interferons upon viral infection. Conventional DCs (cDC) remain unparalleled in stimulating naïve T cells.
With the advent of new technologies, including flow cytometry and single-cell RNA sequencing (scRNA-Seq), it became apparent that the DC family forms a more heterogenous leucocyte population than previously thought (Segura, 2022). Originally, DCs were categorized as cDC and pDC. A study conducted by the Amit group at the Weizmann Institute of Science using scRNA-Seq of mouse splenocytes (Jaitin et al., 2014) identified cDC1 and pDC within the DC population, with a smaller proportion comprising a heterogenous cDC2 population. Interestingly, this cDC2 population comprised at least three additional subsets based on clustering analysis. Human studies in two independent laboratories characterized the diversity of human peripheral blood DC subsets by scRNA-Seq (See et al., 2017; Villani et al., 2017). Adding to the complexity of the DC compartment, circulating human DCs are composed of several populations: cDC1 (CD141+, CLEC9a+), cDC2 subsets (CD1c+), and pDC (CD123+) as previously defined (Guilliams et al., 2014), and a newly identified subset referred to as AS-DC (also known as pre-DC, DC5, or tDC based on the expression of AXL and SIGLEC6), which share several markers with mature pDC, such as the CD123 (Leylek et al., 2019; See et al., 2017; Villani et al., 2017). The CD1c+ population represents a heterogeneous population with broad expression levels of CD5, CD163, and CD14 and includes DC2 (CD5+) and DC3 (CD5-) (Bourdely et al., 2020; Cytlak et al., 2020; Dutertre et al., 2019; See et al., 2017; Villani et al., 2017). An overview of these populations is highlighted in Table 1.
DC name | Membrane markers | Reported phenotype |
---|---|---|
cDC1 | CD141, CLEC9a |
|
DC2 | FcεR1α, CD1c, CD5 |
|
DC3 | FcεR1α, CD1c, CD163 |
|
AS-DC (tDC or preDC) | CD123, CD45RA, AXL, Siglec6 |
|
pDC | CD123, CD45RA, CD303, CD304 |
|
- All DC populations are HLA-DR positive. The distinct attributes of DC2 and DC3 remain an active research concern.
Heterogeneity within the DC compartment has been revealed by scRNA-Seq and high dimensional flow cytometry approaches. To examine the function of these discrete DC populations, immunologists must perform flow-activated cell sorting (FACS) on a fraction of cells that comprise less than 1% of human circulating leucocytes. A critical step when isolating DC subsets in preparation for cell sorting is pan-DC enrichment. Unfortunately, many commercial DC enrichment kits were developed before the discovery of these recently identified DC populations and removed rather than enriched certain novel DC subsets.
Here, we describe what DC subsets can be confidently obtained using two enrichment strategies and the benefits of each approach. A critical consideration when enriching such a complex heterogenous cell population is ensuring no “minor” subset is lost along the way. We first describe a protocol for the enrichment of HLA-expressing “myeloid” cells by crosslinking unwanted leucocytes to erythrocytes in a process termed rosetting, in which red blood cells cluster around a single cell that looks like a rose. This is followed by density centrifugation, in which cross-linked leucocyte-erythrocyte complexes and granulocytes pass through the density layer, resulting in a refined HLA+ myeloid cell population at the interface between the plasma and the density gradient. Next, we detail a protocol developed to enrich pDC, cDC1, AS-DC, and DC2 but not CD14+ DC3 to achieve a significantly more pure population of blood DCs by negative magnetic selection of peripheral blood mononuclear cells (PBMCs). Finally, to assess the ability of these DC populations to activate naïve T cells, we explain the primary allogeneic mixed leucocyte reaction (MLR) using allogenic human naïve T lymphocytes as responders to the isolated cDC subsets.
CAUTION : When working with human material, institutional safety precautions must be followed, including wearing appropriate personal protective equipment (PPE), using a disinfectant, and working at the correct biosafety level. Consult with your institution's biological safety department officer to ensure that all proper precautions are taken.
NOTE : All protocols involving humans and human samples must first be reviewed and approved by an institutional review board (IRB) or must follow local guidelines for the use of human samples. All participants must provide informed consent. Schedule the date and time with the volunteers in advance to ensure the smooth running of the experiment.
NOTE : These protocols assume familiarity with standard tissue culture methods and equipment availability for such procedures, including a biosafety cabinet, centrifuge, water bath, tissue culture incubator, standard laboratory plastic ware, and flow cytometry machines and analysis software.
Basic Protocol 1: HUMAN PERIPHERAL MONONUCLEAR PHAGOCYTE ENRICHMENT
The human DC family currently comprises five principal populations: pDC, cDC1, AS-DC, DC2, and DC3.Many commercial tools developed to isolate DC were designed before the discovery of certain DC subsets and the proprietary cocktail of negative selection antibodies overlaps with the newly identified DC subsets: DC3 expresses CD14 and AS-DC express CD5 or CD2.This protocol provides instructions for the enrichment of all known circulating human DC subsets and other mononuclear phagocytes from peripheral blood using the commercially available RosetteSep HLA Myeloid Cell Enrichment Kit from Stemcell Technologies. Following density centrifugation with Ficoll, erythrocytes, and granulocytes pass through the Ficoll layer due to their higher density and form a large pellet at the bottom of the tube. RosetteSep removes the lymphoid fractions of leucocytes by crosslinking these unwanted cells to erythrocytes, resulting in the formation of a compact rosette leucocyte-erythrocyte complex that also passes through the Ficoll layer. The purified HLA+ myeloid cells are highly enriched at the interface between the plasma and the Ficoll media for downstream analysis.
Materials
-
Fresh blood obtained from healthy volunteers
-
RosetteSep HLA Myeloid Cell Enrichment Kit (STEMCELL Technologies, cat. no. 15272HLA)
-
Dulbecco's Phosphate-Buffered Saline (PBS), Ca2+- and Mg2+-free (Sartorius, cat. no. 02-023-1A)
-
Ficoll Paque Plus density gradient media (Cytiva)
-
FACS buffer (see recipe)
-
0.5% Trypan Blue (Sartorius, cat. no. 03-102-1B)
-
Antibodies for flow cytometry analysis (see Table 2)
-
Human TruStain FcX (BioLegend)
-
Brilliant stain buffer (BD Biosciences, cat. no. BD563794)
-
Tourniquet
-
Alcohol wipes
-
Butterfly needle 21G (BD Biosciences)
-
Heparin or EDTA vacutainers (BD Biosciences)
-
15- and 50-ml conical centrifuge tubes (Greiner bio-one)
-
1.5-ml microcentrifuge tubes (Scientific Specialties)
-
5-ml FACS tubes (Falcon)
-
Tube rotator (Thermo Scientific)
-
Eppendorf centrifuge 5810R (or equivalent)
-
Sterile pipette (Greiner bio-one)
-
Countess II automated cell counter (Invitrogen)
-
Countess Cell counting chamber slides (Invitrogen cat. no. C10228)
-
Cytek Aurora (Cytek Biosciences)
Clone | Fluorophore | Antibody | Company | Dilution in a final volume of 150 µl - up to 10 × 7 cells |
---|---|---|---|---|
HIT3a | APC-Cy7 | CD3 | BioLegend | 1:50 |
HIB19 | APC-Cy7 | CD19 | BioLegend | 1:50 |
2H7 | APC-Cy7 | CD20 | BioLegend | 1:50 |
G10F5 | APC-Cy7 | CD66b | BioLegend | 1:50 |
1D11 | APC-Cy7 | CD314 | BioLegend | 1:50 |
G46-6 | APC-R700 | HLA-DR | BD Biosciences | 1:50 |
S5/1 | PE/DAZZLE | CD88 | BioLegend | 1:50 |
A59 | FITC | CD89 | BioLegend | 1:50 |
M5E2 | BUV563 | CD14 | BD Biosciences | 1:50 |
3G8 | BUV496 | CD16 | BD Biosciences | 1:50 |
HI100 | BV711 | CD45RA | BioLegend | 1:50 |
7G3 | BUV395 | CD123 | BD Biosciences | 1:100 |
UCHT2 | PE | CD5 | BioLegend | 1:50 |
# 108724 | APC | AXL | R & D Systems | 1:10 |
3.9 | BV421 | CD11c | BioLegend | 1:50 |
M80 | PerCP-CY5.5 | CD141 | BioLegend | 1:50 |
L161 | BV650 | CD1c | BioLegend | 1:50 |
AER-37 (CRA-1) | BV510 | FCeR1a | BioLegend | 1:50 |
GHI/61 | BV785 | CD163 | BioLegend | 1:50 |
-
NOTE: please titrate these antibodies for optimal staining.
Phlebotomy
1.In accordance with the Declaration of Helsinki and following institutional research board approval, blood is drawn from the median cubital vein of a human donor using a 21G butterfly needle and aseptic technique. Collect the blood in lithium heparin- or EDTA-treated vacutainers. Invert the tubes five times immediately after obtaining the blood.
2.Transfer the blood to a 15- or 50-ml centrifuge tube, depending on the volume collected.
HLA Myeloid cells enrichment
3.Add 12.5 μl of RosetteSep HLA Myeloid Cell Enrichment solution for every 1 ml of blood collected.
4.Incubate the blood at room temperature (∼20°C) for 20 min on a rotator.
5.Remove the tube from the rotator and dilute the blood 1:1 with sterile PBS.
6.For density centrifugation, prepare a 50-ml tube with 15 ml Ficoll.
7.Carefully add 20 ml of the diluted blood dropwise over the Ficoll layer, avoiding mixing. If using an electronic pipette, ensure the flow is set to gravity. Centrifuge for 20 min at 950 × g and 20°C, with no brake and the lowest acceleration setting.
8.Carefully remove the interphase layer via pipette. This layer contains the enriched HLA+ PBMCs. Transfer the cells to a sterile 50-ml centrifuge tube using a sterile Pasteur pipette. Dilute the cells in a large volume of FACS buffer, three times the original volume, to dilute any residual Ficoll, and invert the tube once before washing the cells.
9.Centrifuge the cells for 5 min at 400 × g and 4°C (now with the brake and acceleration on maximum). Discard the supernatant.
Cells counting
10.Resuspend the enriched HLA+ cell pellet in 10 ml FACS buffer. Ensure the cells are fully resuspended and kept on ice for the remainder of the protocol.
11.In a 1.5-ml centrifuge tube or U-bottom 96-well plate, add 10 μl of the resuspended cells and 10 μl Trypan Blue (0.5% Solution). Ensure the cells are properly mixed by pipetting up and down several times without introducing bubbles.
12.Remove 10 µl of the stained cell solution and place it in the cell counting chamber slide. Insert the slide into the Countess II automated cell counter to enumerate cells per ml sample.
Antibodies staining
13.Wash the leucocyte fraction by centrifugation for 5 min at 400 × g and 4°C, then discard the supernatant.
14.Resuspend the cell pellet in 50 µl FACS buffer plus 5 µl Fc Receptor Blocking Human TruStain FcX per 1 × 107 cells for 10 min.
15.Add the appropriate antibodies. A cocktail of CD3, CD19, CD20 CD66b, and CD314 lineage markers plus HLA-DR helps discriminate mononuclear phagocytes from lineage-positive cells. A cocktail of CD88, CD89, CD14, CD16, CD45RA, CD123, CD5, AXL, CD11c, CD141, CD1c, FceR1a, and CD163 helps define mononuclear phagocytes (Table 2 and Fig. 1). Add 50 µl Brilliant stain buffer to prevent cross-reactivity of Brilliant fluorescent polymer dyes. Mix well and incubate for 20 min at 4°C in the dark.
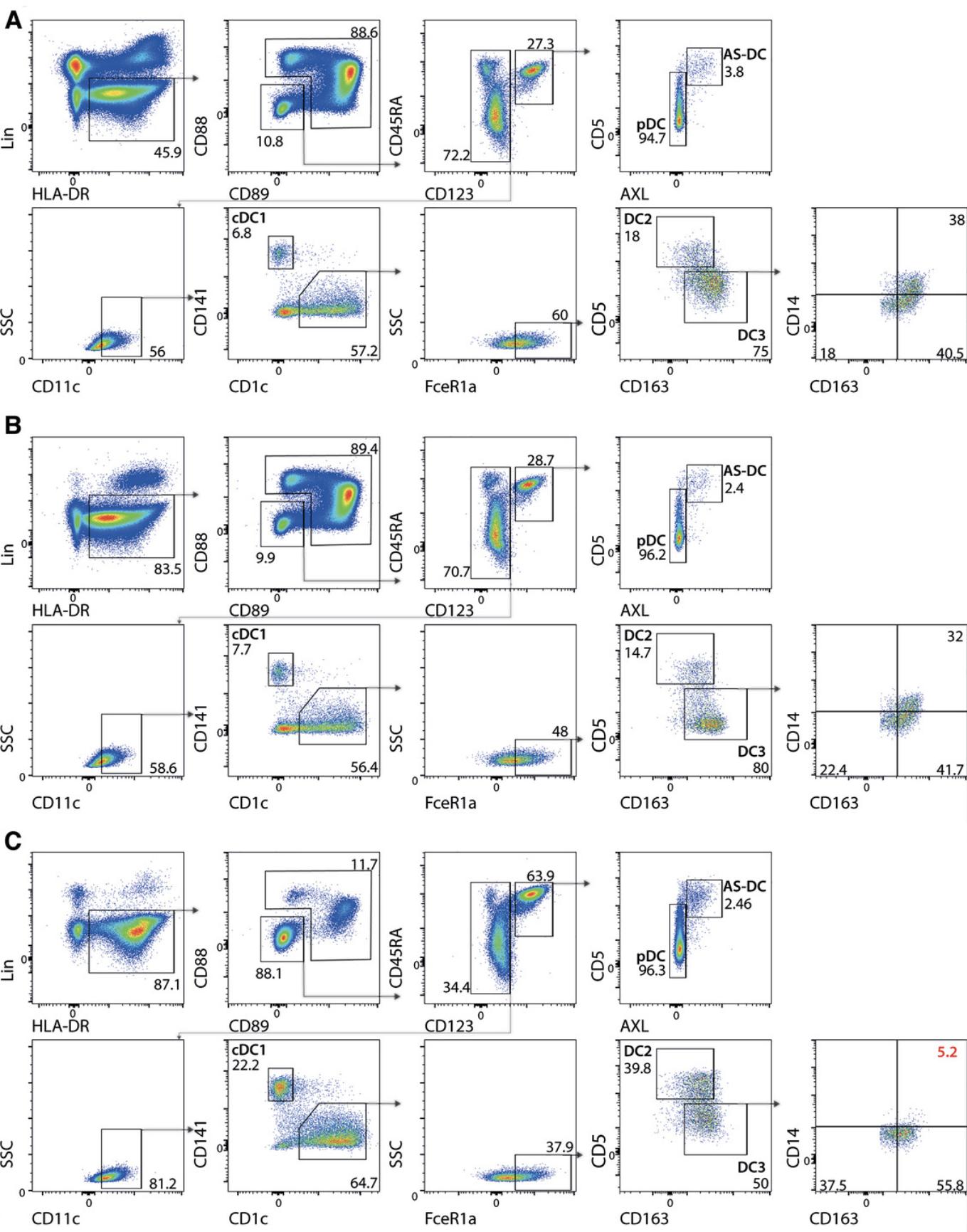
16.Resuspend the cells in 1 ml and wash twice with FACS buffer by centrifuging for 5 min at 400 × g and 4°C. Remove the supernatant.
17.Resuspend the cell pellet in 300 µl FACS buffer and filter the stained cells through the 70-µm mesh into a 5-ml flow cytometry tube to remove clumps before analysis.
18.Determine the purity of the enriched HLA+ fractions and DC subsets by flow cytometry or perform cell sorting if required according to the strategy described in Figure 1.After sorting, verify the purity of each sorted DC subset by running a small sample from each collection tube. Acquire 300-500 events on the FACS machine to assess the quality of the sort.
Basic Protocol 2: DC ENRICHMENT OF: pDC, cDC1, AS-DC, AND DC2 BUT NOT DC3
As discussed above, cDC can be divided into cDC1, DC2, DC3, and an additional population of AS-DC. This protocol achieves a significantly more purified population of blood DC that includes pDC, cDC1, DC2, and AS-DC but not CD14+ DC3 by negative magnetic cell sorting (Fig. 1). Non-target cells are magnetically labeled against specific antigens absent on DC. Magnetically labeled non-target cells are retained in a MACS column (Miltenyi Biotec), while unlabeled DC pass through. The enriched DC fraction can be sorted by FACS downstream for further analysis.
Materials
- Buffy coat, <24-hr old, sourced from the clinical blood bank in accordance with national and institutional guidelines
- PBS, Ca2+- and Mg2+-free (Biological Industries/Sartorius)
- Ficoll Paque Plus density gradient media (Cytiva)
- Pre-cooled FACS buffer (see recipe)
- 0.5% Trypan Blue (Sartorius, cat. no. 03-102-1B)
- Pan-DC Enrichment Kit, human (Miltenyi Biotec, cat. no. 130-100-777)
- Primary antibodies for flow cytometry analysis (see Table 2)
- Brilliant stain buffer (BD Biosciences, cat. no. BD563794)
- Tube rotator (Thermo Scientific)
- 15- and 50-ml conical centrifuge tubes (Greiner bio-one)
- 1.5-ml centrifuge tubes (Scientific Specialties)
- Sterile pipette (Greiner bio-one)
- Eppendorf centrifuge 5810R (or equivalent)
- Countess II automated cell counter (Invitrogen)
- Countess cell counting chamber slides (Invitrogen cat. no. C10228)
- MACS multistand (Miltenyi Biotec, cat. no. 130-042-303)
- MidiMACS separator (Miltenyi Biotec, cat. no. 130-042-302)
- MACS LS columns (Miltenyi Biotec, cat. no. 130-042-401)
- 5-ml round-bottom (FACS) tubes (Falcon)
- 15-ml centrifuge tube stand
- Cytek Aurora (Cytek Biosciences) or flow cytometer capable of analyzing >10 colors
Enrich mononuclear leucocyte fraction by density gradient
1.Transfer blood from the buffy coat to a sterile 50-ml sterile centrifuge tube.
Isolate peripheral blood mononuclear cells (PBMC) from the buffy coat by density gradient centrifugation (see Basic Protocol 1, step 6).
2.Harvest and transfer the interphase layer of the PBMC from the density centrifugation to a sterile centrifuge tube using a sterile Pasteur pipette. Add an equal volume of FACS buffer and slightly invert the tube.
3.Wash the cells by centrifuging for 5 min at 400 × g and 4°C (with brake and acceleration on). Discard the supernatant.
4.Resuspend the pellet in 10 ml PBS and determine the total number of viable cells (see Basic Protocol 1, steps 10-12)
5.Wash the cells by centrifugation for 5 min at 400 × g and 4°C, and remove the supernatant.
6.Resuspend the leucocyte pellet to a concentration of 1 to 1.5 × 108 in 350 µl FACS buffer. Transfer each 350 µl aliquot of resuspended cells to a fresh sterile 15-ml tube.
Magnetic labeling and DC isolation
7.Add 50 µl FcR blocking reagent (from the Pan-DC Enrichment Kit, human) to each tube of cells. Immediately add 100 µl Pan DC biotin-antibody cocktail.
8.Mix well by pipetting up and down carefully to avoid introducing bubbles, and incubate for 5 min at room temperature.
9.Add 400 µl FACS buffer to each tube.
10.Add 100 µl Pan DC microbead-antibody cocktail to each tube. Mix by pipetting up and down and incubate for 5 min at room temperature.
11.Wash the cells by adding 10 ml FACS buffer and centrifuge for 5 min at 400 × g and 4°C. Remove all the supernatant.
12.Resuspend the cell pellet to 1.5 × 10⁸ cells per 500 µl FACS buffer.
13.Attach the MidiMACS Separator to the MACS MultiStand and place the LS column in the separator. Place a 15-ml collection tube on a stable tube stand below the LS column.
14.Prepare each column by washing it with 5 ml FACS buffer.
15.Wait until the column reservoir is empty before proceeding to the next stage.
16.Replace the 15-ml collection tube below the LS column with a new sterile 15-ml collection tube labeled “DC fraction”.
17.Apply the 500 µl cell suspension to the column reservoir. Collect flow-through in the 15-ml tube, which now contains the unlabeled, enriched DC population.
18.Wait until the column reservoir has run dry before proceeding to the next step.
19.Add 9 ml FACS buffer to the column in stages. Collect any residual DC retained in the column in the 15-ml centrifuge tube from step 16.
20.Once all FACS buffer has passed through the column and the reservoir is empty, wash the cells by centrifuging the unlabeled DC fraction for 5 min at 400 × g and 4°C. Remove the supernatant and count the cells as described in basic Protocol 1, steps 10-12.
21.Perform antibody staining (see Basic Protocol 1, steps 12-15) to determine the purity of the DC by flow cytometry or proceed to sort the enriched DC subsets by FACS see Figure 1C to discriminate each DC subset.
Basic Protocol 3: BASIC MIXED LYMPHOCYTE REACTION PROTOCOL WITH SORTED HUMAN DC SUBSETS
This protocol describes how to perform a functional readout of DC. The MLR assay uses allogeneic human T lymphocytes as responder cells to cDC. Stable incorporation of the intracellular fluorescent dye 5-(and-6)-carboxyfluorescein diacetate succinimidyl ester (CFSE) into the T cells can be used as a tool to monitor the proliferation of T cells—each time a cell divides, the fluorescence will be halved, and this change can be measured. This protocol can also examine the response of T cells to DCs stimulated by cytokines or a drug of your choice. The extent of T cell proliferation is then measured by conventional flow cytometry.
Additional Materials (also see Basic Protocol 2)
-
Fetal Bovine Serum (FBS) (Sartorius, cat. no. 04-127-1A)
-
Iscove's Modified Dulbecco's Medium (IMDM) (Sartorius, cat. no. 01-058-1A)
-
Fresh blood obtained from healthy volunteers
-
Naive Pan T Cell Isolation Kit (Miltenyi Biotec, cat. no. 130-097-095)
-
CellTrace CFSE Cell Proliferation Kit (Thermo Fisher, cat. no. C34557)
-
Antibodies for flow cytometry (see Table 3)
-
LPS-EB (LPS from E. coli O111:B4) (InvivoGen)
-
Biological safety hood
-
BD FACSAria III Cell Sorter (BD Biosciences)
-
U-bottom 96-well plate (Falcon)
-
Humidified 37°C, 5% CO2 cell culture incubator (Eppendorf)
Clone | Fluorophore | Antibody | Company | Dilution in a final volume of 150 µl; up to 107 cells |
---|---|---|---|---|
HIT3a | Pacific Blue | CD3 | BioLegend | 1:50 |
SK3 | APC | CD4 | BioLegend | 1:50 |
SK1 | PE-Cy7 | CD8 | BioLegend | 1:50 |
DC culture and stimulation
1.Isolate DCs (see Basic Protocol 1 or 2) followed by DC subset cell sorting, according to the appropriate gating strategy (Fig. 1) using the BD FACSAria III Cell Sorter or a similar machine into FACS tubes containing 300 µl of 1:1 FBS:PBS.
2.Wash the sorted DC subsets (including in any combination of pDC, cDC1, AS-DC, DC2, or DC3) twice in PBS by centrifugation for 5 min at 400 × g and 4°C, then remove the supernatant.
3.Resuspend the cell pellet and count the cells (see Basic Protocol 1 steps 10-12).
4.Adjust the final volume to a concentration of 50,000 cells/ml in IMDM 10% (see recipe).
5.Resuspend the DC thoroughly by pipetting up and down and dispense 100 µl of the adjusted cell suspension into each well of a U-bottom 96-well plate (5000 cells/well). Prepare two sets of triplicate wells for each subset and condition (every stimulus requires equal control and, in certain cases, an FMO).
6.Add 100 µl of 10% IMDM to two additional sets of triplicate wells as the control group.
7.Add 1 µl agonist or vehicle to the appropriate wells, such as LPS, at a final 100 ng/ml concentration.
8.Incubate DC for 1 hr (or for an appropriate period up to 12 hr) in a humidified 37°C, 5% CO2 incubator. Allow the DC to acclimatize while preparing the T cells to be added at the end of the next step.
Magnetic labeling and T cells isolation
9.Draw 20 ml blood from a healthy donor or the volume necessary to perform the assay (see Basic Protocol 1, steps 1-2).
10.Enrich the mononuclear leucocyte fraction by Ficoll density gradient centrifugation (see Basic Protocol 1, steps 5-7).
11.Determine the total number of viable cells by cell counting (see Basic protocol 1, steps 10-12) and wash the cells twice in 20 ml PBS by centrifugation for 5 min at 400 × g and 4°C, removing the supernatant.
12.Adjust PBMC to 1 × 107 in 30 µl sterile FACS buffer. Transfer each 30 µl of resuspended cells to a fresh sterile 15-ml tube.
13.Add 10 µl Naive Pan T Cell Biotin-Antibody Cocktail (from the Naive Pan T Cell Isolation Kit) per 10⁷ cells.
14.Add 10 µl Anti-TCRγ/δ-Biotin per 10⁷ cells.
15.Mix well by pipetting up and down gently and incubate for 5 min in the refrigerator, not on ice (4°C).
16.Add 30 µl FACS buffer per tube and flick the tube several times.
17.Add 20 µl Naive Pan T Cell MicroBead Cocktail per 10⁷ cells.
18.Mix well by pipetting up and down and incubating for 10 min in the refrigerator (4°C).
19.Add 400 µl FACS buffer per tube. Now each tube should contain 500 µl.
20.Prepare the LS column (see Basic Protocol 2, stages 14-16).
21.Apply cell suspension to the column. Collect flow-through in a 15-ml tube containing the enriched naïve unlabeled T cells.
22.Wait until the column reservoir is empty before proceeding to the next step.
23.Wash the column with 9 ml FACS buffer. Collect unlabeled naïve T cells in the same 15-ml centrifuge tube from step 20.
24.Remove the collection tube and wash the cells by centrifugation for 5 min at 400 × g and 4°C, removing the supernatant.
Stain the cells with CFSE
25.Determine the total viable cells obtained in the previous step by cell counting (see Basic Protocol 1, steps 10-11). Typically, this can vary between 2 and 5 × 105 cells per ml of blood.
26.Adjust cell concentration to 1 × 106 cells in 1 ml sterile PBS.
27.Transfer each 1 ml of resuspended cells to a different 15-ml tube.
28.Prepare CFSE stock solution immediately before it is required by adding 18 µl DMSO to one vial of CellTrace CFSE dye reagent and mixing well.
29.Add 1 µl CSFE dye stock solution in DMSO to each tube containing enriched naïve T cells.
30.At room temperature, Incubate the cells for 20 minutes in the dark.
31.Add 5 ml of 10% IMDM and incubate for 5 min in the dark to quench any excess dye.
32.Wash the cells by centrifugation for 5 min at 400 × g and 4°C, removing the supernatant.
33.Resuspend the cell pellet in 1 ml warm 10% IMDM. Wash the cells again for 5 min at 400 × g and 4°C, removing the supernatant. Repeat this step twice more to ensure the removal of any extracellular dye.
T cell culture
34.Determine the total number of viable cells by counting cells (see Basic Protocol 1, steps 10-12).
35.Adjust the CFSE-labeled T cells to 500,000 cells/ml with 10% IMDM.
36.Add 100 µl of the adjusted cell suspension to each well of the U-bottom 96-well plate (50,000 cells per well) from stage 8, containing the DC or control.
37.Incubate undisturbed in a humidified atmosphere at 37°C and 5% CO2 for 5 days.
Antibody staining
38.On day 5, wash the plate by centrifuging for 5 min at 400 × g and 4°C. Quickly invert the plate to discard the supernatant with a flick.
39.Resuspend the cell pellet with 100 µl/well FACS buffer containing the appropriate antibodies (Table 3). Mix well and incubate for 20 min at 4°C in the dark.
40.Wash the cells by centrifuging for 5 min at 400 × g and 4°C, removing the supernatant.
41.Determine T cell fluorescence and division by flow cytometry analysis (Fig. 2). Proliferation of T cells is calculated by the reduction in fluorescence, as the fluorescence is halved with each division and measured by flow cytometry (Fig. 2). If you add an agonist similar to LPS, enhanced T cell proliferation is expected.
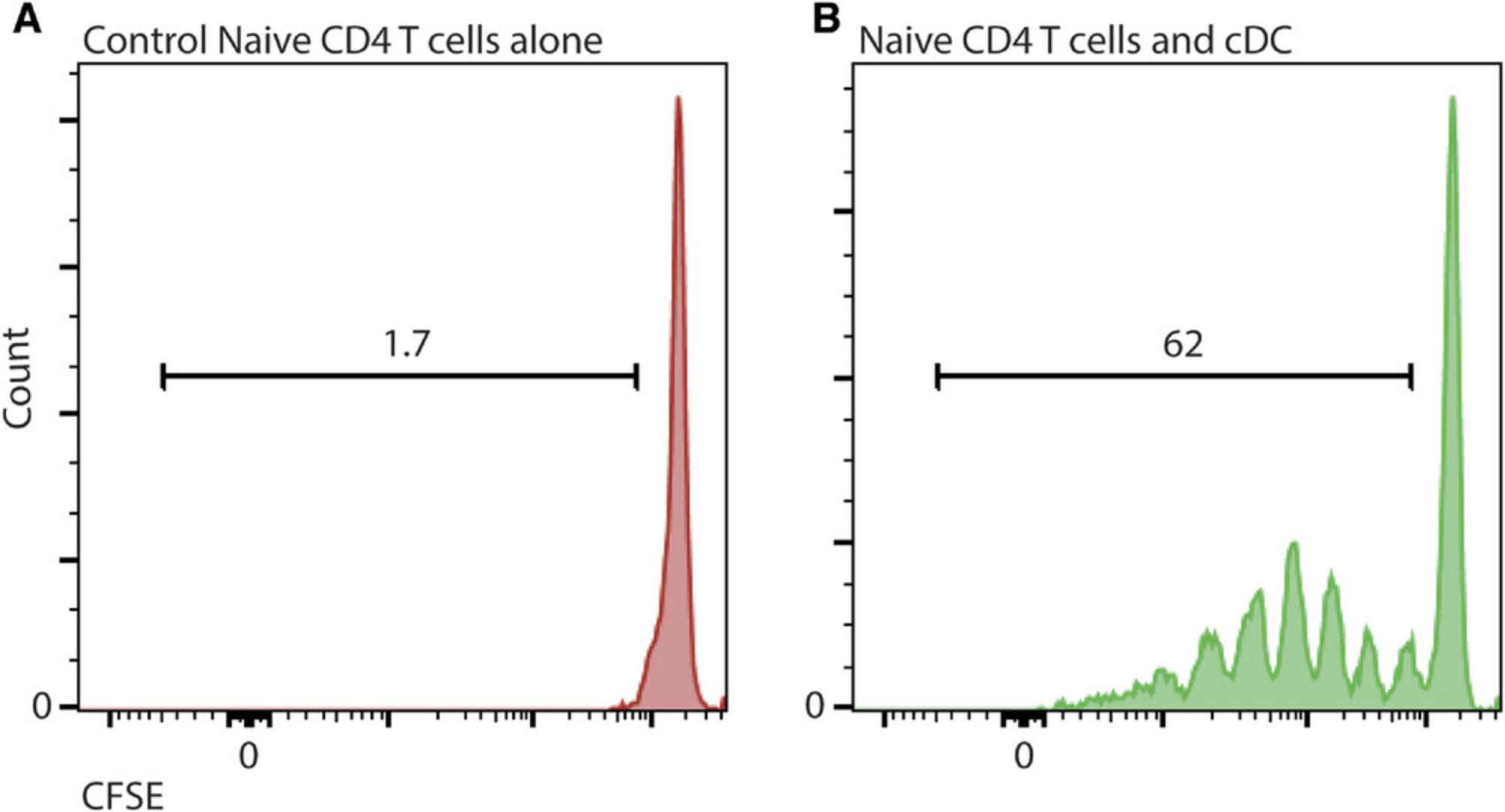
REAGENTS AND SOLUTIONS
All buffers were filtered using Stericup Quick release Millipore Express PLUS 0.22-µm PES, tab 500 ml.
FACS Buffer
- 500 ml Sterile PBS
- 2% (v/v) FBS
- 2 mM EDTA, 0.5 M
- Store for up to 1 month at 4°C.
IMDM 10% in FBS
- 500 ml IMDM
- 5 ml Penicillin-Streptomycin (10,000 U/500 ml)
- 10% (v/v) FBS
- Store for up to 1 month at 4°C.
COMMENTARY
Background Information
Historically, the most common practice when working with DC in vitro involved isolating monocytes via PBMC adherence after peripheral blood density gradient centrifugation and the differentiation of these adherent monocytes into antigen-presenting cells by growing them in a medium supplemented with GM-CSF and IL-4. However, it is now acknowledged that most DCs arise from a dedicated precursor independent of monocytes (Tussiwand et al., 2023), and cultured monocytes generate a heterogenous range of cells (Helft et al., 2015). The methods described here isolate human circulating blood DC.
Rosetting by red blood cells was reported in the 1950s (Jandl, 1955). Protocol 1 takes advantage of the rosetting phenomenon where erythrocytes cluster around a single cell and appear as petals on a flower. By rosetting unwanted leucocyte-erythrocyte complexes followed by density centrifugation, the unwanted leucocyte fraction passes through the density layer to pellet with the granulocytes, leaving an enriched population of the desired cells at the interface between the plasma and the density gradient.
Critical Parameters and Troubleshooting
The most critical factor when isolating DC comprising less than 1% of circulating leucocytes is access to an adequate blood volume. This becomes more critical when isolating specific DC subsets, such as AS-DC, that comprise only a few hundred cells in every milliliter of peripheral blood. It is preferable to work with freshly isolated blood where possible. Buffy coats are ideal for larger volumes, with around 4 to 9 × 108 PBMC following density centrifugation. However, buffy coats can be highly variable. Aging has been reported to influence the immune response and circulating leucocyte numbers. To avoid these extrinsic factors that can confuse the data, blood donors under the age of 45 years are optimal. In addition, blood draws conducted at the same time of day will avoid the possibility of any circadian influences. Finally, ensuring consistency among healthy volunteers is always advisable, avoiding recently vaccinated or sick individuals. The relative proportions of the DC in the immune system vary significantly following infection, including the common cold.
Cell death may occur when staining cells with vital dyes. Therefore, it is advisable to have surplus T cells and recount the cells after staining. When constructing the fluorochrome cocktails to identify your cells by flow cytometry, consider the color of the vital dye and how it will influence the need to compensate.
Understanding Results
During homeostasis, DC comprises less than 1% of all circulating leucocytes. Flow cytometry helps identify DC, which are differentiated from other leucocytes using a lineage (LIN) panel of monoclonal antibodies (mAb), including CD3, CD19, CD20, CD88, CD89, CD314, and CD66b that eliminate T cells, B cells, monocytes, NK cells, and neutrophils, respectively. DC expresses HLA-DR, which is used to help identify the DC family of cells. CD123 and CD45RA are helpful markers for separating pDC and AS-DC from cDC1, DC2, and DC3. Segregation between pDC and AS-DC is accomplished by utilizing both AXL and CD5 antibodies, although Siglec6 is also an option. pDC is defined as AXL-negative CD5int, while AS-DC is positive for AXL and CD5. Conventional DC express CD11c. cDC1 have high expression of CD141 and low expression of CD1c, while both unactivated DC2 and DC3 have low expression of CD141 and high expression of CD1c and FceR1a. DC2 is distinguished from DC3 by its high expression of CD5 and lower expression of CD163. Lastly, DC3 can be divided into 3 subsets, one expressing high levels of CD14 and CD163, one that expresses CD163 but not CD14, and a double-negative population. Figure 1 describes a gating strategy of PBMC following staining with mAb for identifying the DC subsets described thus far.
The recent identification of DC3 and AS-DC, eliminated in conventional DC enrichment kits, was the trigger to investigate other enrichment strategies. One way to enrich DC subsets is the commercially available human Pan-DC Enrichment Kit. Although this kit is effective in enriching the majority of the DC population, it eradicates the subpopulation of DC3 expressing CD14 (Fig. 1C). On the other hand, Basic Protocol 1 describes an efficient way to enrich all DC subsets and monocytes using a kit designed to enrich HLA 'myeloid' cells but can be used for DC enrichment (Fig. 1B). We attempted in protocol 1 to remove the monocyte fraction following density centrifugation by negative magnetic selection of the CD88/CD89 cells. Unfortunately, the reagents were not optimal to significantly remove the residual monocytes. Nevertheless, Basic Protocol 1 efficiently enriches all DC populations from whole blood in preparation for FACS. In the process of examining several protocols to enrich the circulating DC pool, certain monocyte isolation kits also appeared to enrich a DC fraction. Alas, upon closer inspection, these kits depleted the AS-DC subset, most likely due to the addition of CD5 or CD2 in their proprietary antibody cocktail. We suggest using the kits suggested in Basic Protocol 1 or 2, according to your experimental requirements.
The proportion of circulating DC subsets remains relatively consistent between individuals, with pDC accounting for ∼42%, AS-DC ∼2%, cDC1 ∼4%, DC2 ∼20%, and DC3∼32%. It is worth verifying that the proportions of DC subsets retrieved are relatively consistent in case a donor is fighting an infection before the appearance of symptoms that may affect your results.
Finally, after activating naïve T cells by co-culturing with allogenic DC in the MLR Protocol 3 (Fig. 2) for 5 days, cells were stained with CD3, CD4, and CD8 to identify T cell populations and analyzed by flow cytometry. T cells express CD3; cytotoxic T cells and helper T cells can be discriminated by their expression of CD8 and CD4, respectively. The levels of proliferation for each T cell subset can be determined by their expression of CFSE compared with the levels of unstimulated T cells. When T cells proliferate, fluorescence decreases with each division.
Time Considerations
The enrichment Basic Protocols 1 and 2 require 2-3 hr, including preparation time. It is critical to bear in mind that enrichment will involve a full morning's work before a further 1-2 hr to sort the desired populations. It is advisable to start first thing in the morning. When performing density centrifugation, ensure you factor in the time needed to cool and warm the centrifuge and the time needed for the sample to slow down without the brake. When working with volunteers and phlebotomists, make sure everyone arrives on time. The isolation of T cells and CFSE staining in Basic Protocol 3 requires several wash steps that should be considered. Finally, when turning flow cytometers and FACS machines on and off, allow plenty of time to follow daily QC procedures.
Acknowledgments
SY is supported by an Israel Science Foundation Grant (316/20). RL is supported by Ariane de Rothschild Doctoral Studentship.
Author Contributions
Ruth Lubin : Data curation, Formal analysis, Methodology, Original draft. Rotem Gvili : Formal analysis, Draft review, and editing. Idit Hazan : Formal analysis, Project administration, Draft review, and editing. Simon Yona : Conceptualization, Funding acquisition, Project administration, Original draft
Conflict of Interest
The authors declare no conflict of interest.
Open Research
Data Availability Statement
The data that support the findings of this study are available from the corresponding author upon reasonable request.
Literature Cited
- Alcantara-Hernandez, M., Leylek, R., Wagar, L. E., Engleman, E. G., Keler, T., Marinkovich, M. P., Davis, M. M., Nolan, G. P., & Idoyaga, J. (2017). High-dimensional phenotypic mapping of human dendritic cells reveals interindividual variation and tissue specialization. Immunity , 47(6), 1037–1050 e1036. https://doi.org/10.1016/j.immuni.2017.11.001
- Bach, F., & Hirschhorn, K. (1964). Lymphocyte Interaction: A Potential Histocompatibility Test in Vitro. Science , 143(3608), 813–814. https://doi.org/10.1126/science.143.3608.813
- Bachem, A., Guttler, S., Hartung, E., Ebstein, F., Schaefer, M., Tannert, A., Salama, A., Movassaghi, K., Opitz, C., Mages, H. W., Henn, V., Kloetzel, P. M., Gurka, S., & Kroczek, R. A. (2010). Superior antigen cross-presentation and XCR1 expression define human CD11c+CD141+ cells as homologues of mouse CD8+ dendritic cells. Journal of Experimental Medicine , 207(6), 1273–1281. https://doi.org/10.1084/jem.20100348
- Bain, B., Vas, M. R., & Lowenstein, L. (1964). The development of large immature mononuclear cells in mixed leukocyte cultures. Blood , 23, 108–116. https://doi.org/10.1182/blood.V23.1.108.108
- Bakdash, G., Buschow, S. I., Gorris, M. A., Halilovic, A., Hato, S. V., Skold, A. E., Schreibelt, G., Sittig, S. P., Torensma, R., Duiveman-de Boer, T., Schroder, C., Smits, E. L., Figdor, C. G., & de Vries, I. J. (2016). Expansion of a BDCA1+CD14+ myeloid cell population in melanoma patients may attenuate the efficacy of dendritic cell vaccines. Cancer Research , 76(15), 4332–4346. https://doi.org/10.1158/0008-5472.CAN-15-1695
- Billingham, R. E., Krohn, P. L., & Medawar, P. B. (1951). Effect of locally applied cortisone acetate on survival of skin homografts in rabbits. British Medical Journal , 2(4739), 1049–1053. https://doi.org/10.1136/bmj.2.4739.1049
- Billington, W. D. (2003). The immunological problem of pregnancy: 50 years with the hope of progress. A tribute to Peter Medawar. Journal of Reproductive Immunology , 60(1), 1–11. https://doi.org/10.1016/S0165-0378(03)00083-4
- Bourdely, P., Anselmi, G., Vaivode, K., Ramos, R. N., Missolo-Koussou, Y., Hidalgo, S., Tosselo, J., Nunez, N., Richer, W., Vincent-Salomon, A., Saxena, A., Wood, K., Lladser, A., Piaggio, E., Helft, J., & Guermonprez, P. (2020). Transcriptional and functional analysis of CD1c(+) Human dendritic cells identifies a CD163(+) subset priming CD8(+)CD103(+) T cells. Immunity , 53(2), 335–352 e338. https://doi.org/10.1016/j.immuni.2020.06.002
- Cella, M., Jarrossay, D., Facchetti, F., Alebardi, O., Nakajima, H., Lanzavecchia, A., & Colonna, M. (1999). Plasmacytoid monocytes migrate to inflamed lymph nodes and produce large amounts of type I interferon. Nature Medicine , 5(8), 919–923. https://doi.org/10.1038/11360
- Chiang, M. C., Tullett, K. M., Lee, Y. S., Idris, A., Ding, Y., McDonald, K. J., Kassianos, A., Leal Rojas, I. M., Jeet, V., Lahoud, M. H., & Radford, K. J. (2016). Differential uptake and cross-presentation of soluble and necrotic cell antigen by human DC subsets. European Journal of Immunology , 46(2), 329–339. https://doi.org/10.1002/eji.201546023
- Cohn, L., Chatterjee, B., Esselborn, F., Smed-Sorensen, A., Nakamura, N., Chalouni, C., Lee, B. C., Vandlen, R., Keler, T., Lauer, P., Brockstedt, D., Mellman, I., & Delamarre, L. (2013). Antigen delivery to early endosomes eliminates the superiority of human blood BDCA3+ dendritic cells at cross presentation. Journal of Experimental Medicine , 210(5), 1049–1063. https://doi.org/10.1084/jem.20121251
- Crozat, K., Guiton, R., Guilliams, M., Henri, S., Baranek, T., Schwartz-Cornil, I., Malissen, B., & Dalod, M. (2010). Comparative genomics as a tool to reveal functional equivalences between human and mouse dendritic cell subsets. Immunological Reviews , 234(1), 177–198. https://doi.org/10.1111/j.0105-2896.2009.00868.x
- Cytlak, U., Resteu, A., Pagan, S., Green, K., Milne, P., Maisuria, S., McDonald, D., Hulme, G., Filby, A., Carpenter, B., Queen, R., Hambleton, S., Hague, R., Lango Allen, H., Thaventhiran, J. E. D., Doody, G., Collin, M., & Bigley, V. (2020). Differential IRF8 transcription factor requirement defines two pathways of dendritic cell development in humans. Immunity , 53(2), 353–358. https://doi.org/10.1016/j.immuni.2020.07.003
- Durand, M., Walter, T., Pirnay, T., Naessens, T., Gueguen, P., Goudot, C., Lameiras, S., Chang, Q., Talaei, N., Ornatsky, O., Vassilevskaia, T., Baulande, S., Amigorena, S., & Segura, E. (2019). Human lymphoid organ cDC2 and macrophages play complementary roles in T follicular helper responses. Journal of Experimental Medicine , 216(7), 1561–1581. https://doi.org/10.1084/jem.20181994
- Dutertre, C. A., Becht, E., Irac, S. E., Khalilnezhad, A., Narang, V., Khalilnezhad, S., Ng, P. Y., van den Hoogen, L. L., Leong, J. Y., Lee, B., Chevrier, M., Zhang, X. M., Yong, P. J. A., Koh, G., Lum, J., Howland, S. W., Mok, E., Chen, J., Larbi, A., … Ginhoux, F. (2019). Single-cell analysis of human mononuclear phagocytes reveals subset-defining markers and identifies circulating inflammatory dendritic cells. Immunity , 51(3), 573–589 e578. https://doi.org/10.1016/j.immuni.2019.08.008
- Fenton, T. M., Kelly, A., Shuttleworth, E. E., Smedley, C., Atakilit, A., Powrie, F., Campbell, S., Nishimura, S. L., Sheppard, D., Levison, S., Worthington, J. J., Lehtinen, M. J., & Travis, M. A. (2017). Inflammatory cues enhance TGFbeta activation by distinct subsets of human intestinal dendritic cells via integrin alphavbeta8. Mucosal Immunology , 10(3), 624–634. https://doi.org/10.1038/mi.2016.94
- Fitzgerald-Bocarsly, P. (1993). Human natural interferon-alpha producing cells. Pharmacology & Therapeutics, 60(1), 39–62. https://doi.org/10.1016/0163-7258(93)90021-5
- Girard, M., Law, J. C., Edilova, M. I., & Watts, T. H. (2020). Type I interferons drive the maturation of human DC3s with a distinct costimulatory profile characterized by high GITRL. Science Immunology , 5(53), eabe0347. https://doi.org/10.1126/sciimmunol.abe0347
- Guilliams, M., Ginhoux, F., Jakubzick, C., Naik, S. H., Onai, N., Schraml, B. U., Segura, E., Tussiwand, R., & Yona, S. (2014). Dendritic cells, monocytes and macrophages: A unified nomenclature based on ontogeny. Nature Reviews Immunology , 14(8), 571–578. https://doi.org/10.1038/nri3712
- Guilliams, M., Mildner, A., & Yona, S. (2018). Developmental and functional heterogeneity of monocytes. Immunity , 49(4), 595–613. https://doi.org/10.1016/j.immuni.2018.10.005
- Haniffa, M., Shin, A., Bigley, V., McGovern, N., Teo, P., See, P., Wasan, P. S., Wang, X. N., Malinarich, F., Malleret, B., Larbi, A., Tan, P., Zhao, H., Poidinger, M., Pagan, S., Cookson, S., Dickinson, R., Dimmick, I., Jarrett, R. F., … Ginhoux, F. (2012). Human tissues contain CD141(hi) cross-presenting dendritic cells with functional homology to mouse CD103(+) Nonlymphoid dendritic cells. Immunity , 37(1), 60–73. https://doi.org/10.1016/j.immuni.2012.04.012
- Helft, J., Bottcher, J., Chakravarty, P., Zelenay, S., Huotari, J., Schraml, B. U., Goubau, D., & Reis e Sousa, C. (2015). GM-CSF mouse bone marrow cultures comprise a heterogeneous population of CD11c(+)MHCII(+) macrophages and dendritic cells. Immunity , 42(6), 1197–1211. https://doi.org/10.1016/j.immuni.2015.05.018
- Jaitin, D. A., Kenigsberg, E., Keren-Shaul, H., Elefant, N., Paul, F., Zaretsky, I., Mildner, A., Cohen, N., Jung, S., Tanay, A., & Amit, I. (2014). Massively parallel single-cell RNA-seq for marker-free decomposition of tissues into cell types. Science , 343(6172), 776–779. https://doi.org/10.1126/science.1247651
- Jandl, J. H. (1955). Sequestration by the spleen of red cells sensitized with incomplete antibody and with metallo-protein complexes.
- Jongbloed, S. L., Kassianos, A. J., McDonald, K. J., Clark, G. J., Ju, X., Angel, C. E., Chen, C. J., Dunbar, P. R., Wadley, R. B., Jeet, V., Vulink, A. J., Hart, D. N., & Radford, K. J. (2010). Human CD141+ (BDCA-3)+ dendritic cells (DCs) represent a unique myeloid DC subset that cross-presents necrotic cell antigens. Journal of Experimental Medicine , 207(6), 1247–1260. https://doi.org/10.1084/jem.20092140
- Klechevsky, E., Morita, R., Liu, M., Cao, Y., Coquery, S., Thompson-Snipes, L., Briere, F., Chaussabel, D., Zurawski, G., Palucka, A. K., Reiter, Y., Banchereau, J., & Ueno, H. (2008). Functional specializations of human epidermal Langerhans cells and CD14+ dermal dendritic cells. Immunity , 29(3), 497–510. https://doi.org/10.1016/j.immuni.2008.07.013
- Kvedaraite, E., Hertwig, L., Sinha, I., Ponzetta, A., Hed Myrberg, I., Lourda, M., Dzidic, M., Akber, M., Klingstrom, J., Folkesson, E., Muvva, J. R., Chen, P., Gredmark-Russ, S., Brighenti, S., Norrby-Teglund, A., Eriksson, L. I., Rooyackers, O., Aleman, S., Stralin, K., … Karolinska, K. I. K. C.-S. G. (2021). Major alterations in the mononuclear phagocyte landscape associated with COVID-19 severity. PNAS , 118(6), e2018587118. https://doi.org/10.1073/pnas.2018587118
- Leal Rojas, I. M., Mok, W. H., Pearson, F. E., Minoda, Y., Kenna, T. J., Barnard, R. T., & Radford, K. J. (2017). Human blood CD1c(+) dendritic cells promote Th1 and Th17 effector function in memory CD4(+) T cells. Frontiers in Immunology , 8, 971. https://doi.org/10.3389/fimmu.2017.00971
- Leylek, R., Alcantara-Hernandez, M., Lanzar, Z., Ludtke, A., Perez, O. A., Reizis, B., & Idoyaga, J. (2019). Integrated cross-species analysis identifies a conserved transitional dendritic cell population. Cell Reports , 29(11), 3736–3750 e3738. https://doi.org/10.1016/j.celrep.2019.11.042
- Maier, B., Leader, A. M., Chen, S. T., Tung, N., Chang, C., LeBerichel, J., Chudnovskiy, A., Maskey, S., Walker, L., Finnigan, J. P., Kirkling, M. E., Reizis, B., Ghosh, S., D'Amore, N. R., Bhardwaj, N., Rothlin, C. V., Wolf, A., Flores, R., Marron, T., … Merad, M. (2020). A conserved dendritic-cell regulatory program limits antitumour immunity. Nature , 580(7802), 257–262. https://doi.org/10.1038/s41586-020-2134-y
- McDevitt, H. O. (2000). Discovering the role of the major histocompatibility complex in the immune response. Annual Review of Immunology , 18, 1–17. https://doi.org/10.1146/annurev.immunol.18.1.1
- Mittag, D., Proietto, A. I., Loudovaris, T., Mannering, S. I., Vremec, D., Shortman, K., Wu, L., & Harrison, L. C. (2011). Human dendritic cell subsets from spleen and blood are similar in phenotype and function but modified by donor health status. Journal of Immunology , 186(11), 6207–6217. https://doi.org/10.4049/jimmunol.1002632
- Poulin, L. F., Salio, M., Griessinger, E., Anjos-Afonso, F., Craciun, L., Chen, J. L., Keller, A. M., Joffre, O., Zelenay, S., Nye, E., le Moine, A., Faure, F., Donckier, V., Sancho, D., Cerundolo, V., Bonnet, D., & Reis e Sousa, C. (2010). Characterization of human DNGR-1+ BDCA3+ leukocytes as putative equivalents of mouse CD8alpha+ dendritic cells. Journal of Experimental Medicine , 207(6), 1261–1271. https://doi.org/10.1084/jem.20092618
- Schlitzer, A., McGovern, N., Teo, P., Zelante, T., Atarashi, K., Low, D., Ho, A. W., See, P., Shin, A., Wasan, P. S., Hoeffel, G., Malleret, B., Heiseke, A., Chew, S., Jardine, L., Purvis, H. A., Hilkens, C. M., Tam, J., Poidinger, M., … Ginhoux, F. (2013). IRF4 transcription factor-dependent CD11b+ dendritic cells in human and mouse control mucosal IL-17 cytokine responses [Research Support, N.I.H., Extramural Research Support, Non-U.S. Gov't]. Immunity , 38(5), 970–983. https://doi.org/10.1016/j.immuni.2013.04.011
- Schlitzer, A., Sivakamasundari, V., Chen, J., Sumatoh, H. R., Schreuder, J., Lum, J., Malleret, B., Zhang, S., Larbi, A., Zolezzi, F., Renia, L., Poidinger, M., Naik, S., Newell, E. W., Robson, P., & Ginhoux, F. (2015). Identification of cDC1- and cDC2-committed DC progenitors reveals early lineage priming at the common DC progenitor stage in the bone marrow. Nature Immunology , 16(7), 718–728. https://doi.org/10.1038/ni.3200
- See, P., Dutertre, C. A., Chen, J., Gunther, P., McGovern, N., Irac, S. E., Gunawan, M., Beyer, M., Handler, K., Duan, K., Sumatoh, H. R. B., Ruffin, N., Jouve, M., Gea-Mallorqui, E., Hennekam, R. C. M., Lim, T., Yip, C. C., Wen, M., Malleret, B., … Ginhoux, F. (2017). Mapping the human DC lineage through the integration of high-dimensional techniques. Science , 356(6342), eaag3009. https://doi.org/10.1126/science.aag3009
- Segura, E. (2022). Human dendritic cell subsets: An updated view of their ontogeny and functional specialization. European Journal of Immunology , 52(11), 1759–1767. https://doi.org/10.1002/eji.202149632
- Segura, E., Durand, M., & Amigorena, S. (2013). Similar antigen cross-presentation capacity and phagocytic functions in all freshly isolated h0. Journal of Experimental Medicine , 210(5), 1035–1047. https://doi.org/10.1084/jem.20121103
- Segura, E., Valladeau-Guilemond, J., Donnadieu, M. H., Sastre-Garau, X., Soumelis, V., & Amigorena, S. (2012). Characterization of resident and migratory dendritic cells in human lymph nodes [Research Support, Non-U.S. Gov't]. Journal of Experimental Medicine , 209(4), 653–660. https://doi.org/10.1084/jem.20111457
- Steinman, R. M., & Cohn, Z. A. (1973). Identification of a novel cell type in peripheral lymphoid organs of mice. I. Morphology, quantitation, tissue distribution. Journal of Experimental Medicine , 137(5), 1142–1162. https://doi.org/10.1084/jem.137.5.1142
- Steinman, R. M., & Witmer, M. D. (1978). Lymphoid dendritic cells are potent stimulators of the primary mixed leukocyte reaction in mice. Proceedings National Academy of Science USA , 75(10), 5132–5136. https://doi.org/10.1073/pnas.75.10.5132
- Tussiwand, R., Guermonprez, P., & Yona, S. (2023). Dendritic cells. In M. F. Flajnik, N. J. Singh, & S. M. Holland (Eds.), Paul's Fundamental Immunology ( 8 ed.). Lippincott Willaims and Wilkins.
- Villani, A. C., Satija, R., Reynolds, G., Sarkizova, S., Shekhar, K., Fletcher, J., Griesbeck, M., Butler, A., Zheng, S., Lazo, S., Jardine, L., Dixon, D., Stephenson, E., Nilsson, E., Grundberg, I., McDonald, D., Filby, A., Li, W., de Jager, P. L., … Hacohen, N. (2017). Single-cell RNA-seq reveals new types of human blood dendritic cells, monocytes, and progenitors. Science , 356(6335), eaah4573. https://doi.org/10.1126/science.aah4573
- Winheim, E., Rinke, L., Lutz, K., Reischer, A., Leutbecher, A., Wolfram, L., Rausch, L., Kranich, J., Wratil, P. R., Huber, J. E., Baumjohann, D., Rothenfusser, S., Schubert, B., Hilgendorff, A., Hellmuth, J. C., Scherer, C., Muenchhoff, M., von Bergwelt-Baildon, M., Stark, K., … Krug, A. B. (2021). Impaired function and delayed regeneration of dendritic cells in COVID-19. Plos Pathogens , 17(10), e1009742. https://doi.org/10.1371/journal.ppat.1009742
- Zinkernagel, R. M., & Doherty, P. C. (1974). Restriction of in vitro T cell-mediated cytotoxicity in lymphocytic choriomeningitis within a syngeneic or semiallogeneic system. Nature , 248(5450), 701–702. https://doi.org/10.1038/248701a0