Expansion Microscopy: Protocols for Imaging Proteins and RNA in Cells and Tissues
Asmamaw T. Wassie, Asmamaw T. Wassie, Fei Chen, Fei Chen, Shoh M. Asano, Shoh M. Asano, Ruixuan Gao, Ruixuan Gao, Paul W. Tillberg, Paul W. Tillberg, Edward S. Boyden, Edward S. Boyden
antibody
confocal microscopy
expansion microscopy
FISH
fluorescence microscopy
hydrogel
imaging
immunohistochemistry
immunocytochemistry
light-sheet microscopy
super-resolution microscopy
tissue clearing
Abstract
Expansion microscopy (ExM) is a recently developed technique that enables nanoscale-resolution imaging of preserved cells and tissues on conventional diffraction-limited microscopes via isotropic physical expansion of the specimens before imaging. In ExM, biomolecules and/or fluorescent labels in the specimen are linked to a dense, expandable polymer matrix synthesized evenly throughout the specimen, which undergoes 3-dimensional expansion by ∼4.5 fold linearly when immersed in water. Since our first report, versions of ExM optimized for visualization of proteins, RNA, and other biomolecules have emerged. Here we describe best-practice, step-by-step ExM protocols for performing analysis of proteins (protein retention ExM, or proExM) as well as RNAs (expansion fluorescence in situ hybridization, or ExFISH), using chemicals and hardware found in a typical biology lab. Furthermore, a detailed protocol for handling and mounting expanded samples and for imaging them with confocal and light-sheet microscopes is provided. © 2020 The Authors.
Introduction
In expansion microscopy (ExM), preserved biological samples are embedded within a swellable hydrogel and physically expanded isotropically, leading to optically transparent samples which allow for nanoscale resolution and aberration-free microscopy imaging on conventional diffraction-limited microscopes (Chen, Tillberg, & Boyden, 2015). Recently, two new ExM versions have been developed, termed protein retention expansion microscopy (proExM; Tillberg et al., 2016) and expansion fluorescence in situ hybridization (ExFISH; Chen et al., 2016), where protein (proExM) and RNA (ExFISH) molecules are chemically anchored to the hydrogel and probed upon expansion. This unit describes the most common scenarios for proExM and ExFISH sample preparation, as well as general guidelines for sample handling and image acquisition.
In proExM, proteins are covalently anchored to a hydrogel matrix with a commercially available small molecule (Acryloyl-X SE, or AcX for short; Tillberg et al., 2016) that binds to primary amine groups on proteins (Fig. 1E, left) and is then incorporated into the hydrogel polymer during the process of polymerization (also known as gelation of a hydrogel; schematized in Fig. 1A to 1C). An enzymatic digestion using proteinase K (ProK) or a high-temperature treatment with detergent mechanically disrupts the embedded sample, allowing for isotropic expansion in water. One can anchor native proteins to the gel using AcX and apply fluorescent antibodies post-expansion (post-expansion staining, Fig. 1C). This version uses a mechanical homogenization method employing high temperature and detergent, without protease. One can alternatively apply AcX to fixed specimens expressing fluorescent proteins and/or labeled already with fluorescent antibodies, incorporating these directly into the gel (pre-expansion staining, Fig. 1A, or pre-expansion fluorescent protein expression, Fig. 1B). Fluorescent proteins and antibodies are sufficiently resistant to proteolysis that they largely survive the ProK application. Utilizing a lens with 300-nm diffraction-limited resolution, the resulting ∼4.5× linear expansion of the embedded samples (∼90× volumetric expansion) allows imaging of the sample with ∼300 nm/4.5∼60- to 70-nm effective resolution.
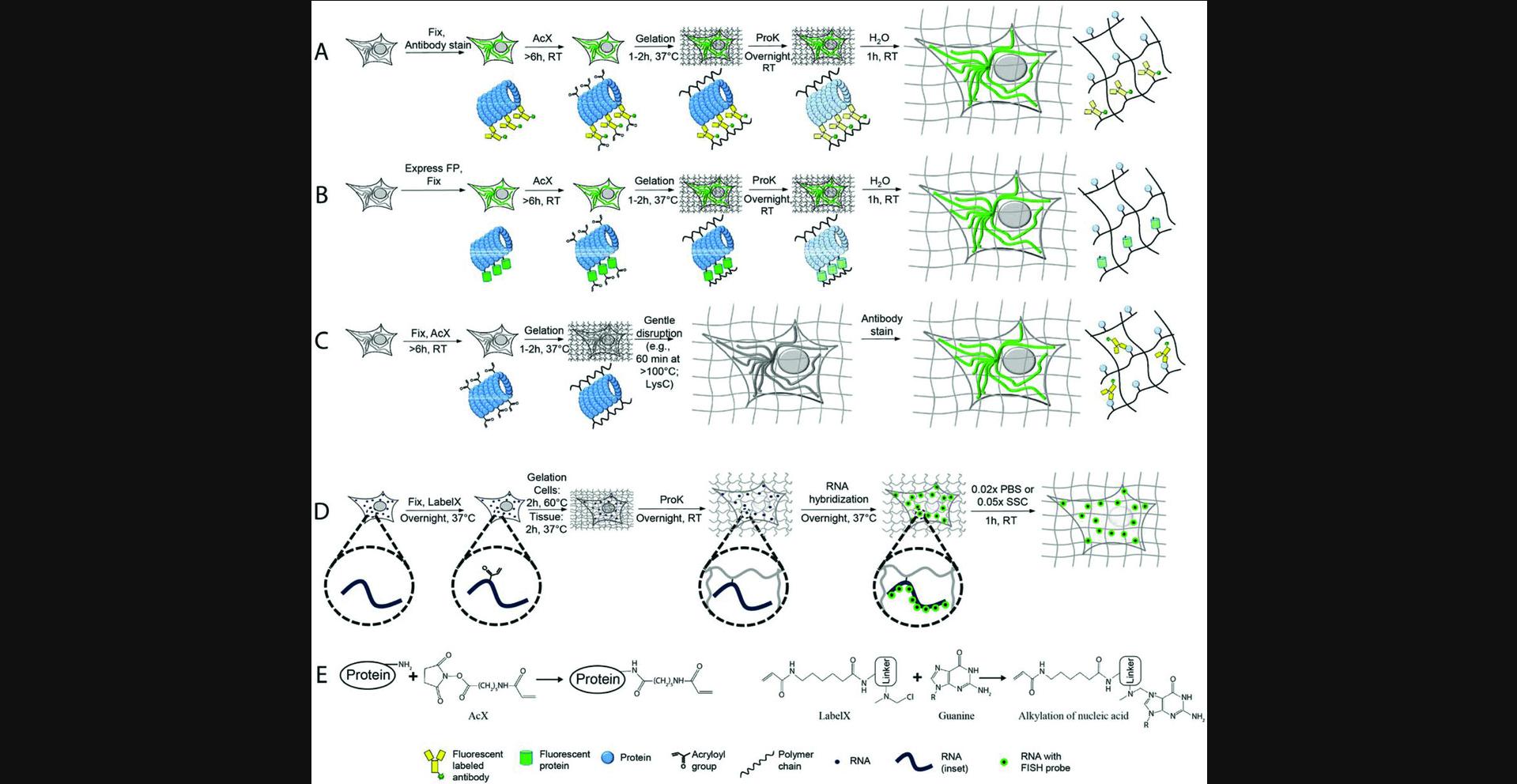
In ExFISH, RNAs are covalently anchored to the hydrogel matrix with a small molecule, which we call LabelX (which can be easily synthesized by reacting two commercially available molecules), that binds to guanine (in RNA and DNA, Fig. 1E, right) and also to the hydrogel polymer (Chen et al., 2016). The ExFISH pipeline is shown in Figure 1D: samples are treated with LabelX, the swellable hydrogel polymer is formed, ProK is applied, and then the specimen is washed with phosphate-buffered saline (PBS). Afterwards, RNA-FISH probes are applied to the sample in a hybridization buffer to label RNA molecules of interest. The sample is then expanded and imaged in a low-salt buffer. This nonzero salt concentration, which allows RNA-FISH probes to remain stably bound, results in a ∼3× linear expansion (∼27× volumetric expansion), or an effective resolution for a 300-nm diffraction-limited lens of ∼300 nm/3∼100 nm.
This unit is structured into the following sections. In Basic Protocols 1 and 2, we describe proExM protocols for cells and intact tissues (of <200 μm pre-expansion thickness) that have been immunostained before expansion (pre-expansion staining proExM), or have fluorescent proteins expressed before expansion, as illustrated in Figure 1A,B. These two basic protocols are written independently, so that readers can start with either of the two, with minimal reference to the other. The next section, Basic Protocol 3, describes steps that help with processing of thick tissues or tissue slices (e.g., 200 to 500 μm thick, or potentially thicker), which have been immunostained or had fluorescent proteins expressed before expansion (as illustrated in Fig. 1A, B). Support Protocol 1 describes the protocol for immunostaining after expansion (post-expansion staining proExM, as illustrated in Fig. 1C). We also briefly discuss key considerations when choosing antibodies and dyes for proExM in Support Protocol 2. In Basic Protocols 4 and 5, we describe ExFISH methodology for the cultured cells and intact tissues, respectively. In the last protocol, Basic Protocol 6, we describe different mounting strategies for preparing expanded ExM samples for imaging on inverted confocal microscopes and the Zeiss Z.1 light-sheet microscope. This section on imaging applies to all ExM-processed samples, including products of the proExM and ExFISH protocols here described.
It may be helpful to note that sample preparation and imaging with proExM and ExFISH only require chemicals and microscopes found in a typical biology lab or imaging facility, with the exception of anchoring reagents and sodium acrylate, which are available commercially at modest cost. Almost all the protocol steps can be carried out at room temperature on a wetlab bench (unless otherwise noted in the protocol). Basic equipment required includes a benchtop vortex mixer, a shaker, a 37°C incubator (and a 60°C incubator for ExFISH of cultured cells), a desiccator, a 4°C refrigerator, and a –20°C freezer. All the materials and supplies used in this unit are commercially available. Unless otherwise noted, in this unit “water” specifically means “double-distilled water.”
Basic Protocol 1: proExM for Cultured Cells
Materials
- 1 M potassium hydroxide (KOH) in water
- 30% (v/v) ethanol in water
- 10 mg/ml sterile fibronectin in Phosphate-buffered saline (PBS; Current Protocols, 2001)
- Cells of interest, e.g., human embryonic kidney (HEK) cells
- Cell culture medium suitable for the cell type of choice (e.g., Dulbecco's Modified Eagle Medium (DMEM) for human embryonic kidney (HEK) cells)
- 4% (w/v) paraformaldehyde (PFA) in PBS or, for stronger fixation, a mixture of 4% (w/v) PFA and 0.1% (w/v) glutaraldehyde in PBS
- 100 mM glycine in PBS
- 0.1% (w/v) Triton X-100 in PBS
- Blocking buffer: 5% (v/v) normal donkey serum in 0.1% (w/v) Triton X-100 in PBS (store up to 1 month or longer at 4°C); the serum should be from the animal host of the secondary antibodies, so other types of sera should be used instead of normal donkey serum if the secondary antibodies are from other animals
- Primary and secondary antibodies for immunostaining
- AcX/DMSO stock solution (see recipe)
- Gelling solution for proExM of cultured cells (see recipe)
- Digestion buffer (see recipe)
- Cell culture substrates (e.g., coverslips of sizes, shapes, and surface modifications suitable for the culture protocol of choice), such as 12-mm round coverslips or a removable-chamber cover glass; e.g., Sigma, cat. no. GBL 112358
- Chamber cover glass removal tool (Sigma, catalog no. GBL 103259)
- 35-mm petri dishes
- Microcentrifuge tubes
- Shaker
- 22 × 22 mm No. 1.5 coverslips (0.15 to 0.19 mm thickness)
- 22 × 22 mm No. 2 coverslips (0.19 to 0.23 mm thickness)
- Microscope slides
- Ice bath or cold block chilled to 4°C
- Parafilm
- Razor blade
- Forceps
- Nunc 4-well rectangular plate (Thermo Fisher, cat. no. 267061)
- Paintbrush
- Diamond scribe for cutting (scribing) thin glass
- Dissection microscope
Cell culture
Cells can be cultured according to protocols of your choice. However, if there is no strong preference, we recommended culturing cells on a substrate separable from the culture well (e.g., on coverslips) or on a removable-chamber cover glass (e.g., Sigma, cat. no. GBL 112358) for easy gelation and sample handling in later steps.
The cell culture protocol described below uses adherent cell lines (e.g., HEK or COS7) and has demonstrated consistent results. If removable-chamber cover glasses are used for cell culture, start with the fibronectin glass surface modification in step 2.
1.Treat 12-mm round coverslips with 1 M KOH in water for 30 min, rinse with water three times, and store in 30% (v/v) ethanol in water.
2.Air-dry the cleaned 12-mm round coverslips in a sterile environment (i.e., biosafety cabinet), place in a 35-mm petri dish, and subsequently treat with 10 mg/ml sterile fibronectin in PBS for 30 min at 37°C. Remove the fibronectin solution immediately before cell plating.
3.Cells are plated and cultured on the surface of either the 12-mm coverslips or the removable-chamber cover glass to a confluency of 50% to 80% in a humidified incubator set at 5% CO2 and 37°C.
Cell fixation
Cells can be fixed using protocols of your choice. For instance, 4% (w/v) paraformaldehyde (PFA) in PBS can be used. For stronger fixation that preserves more ultrastructure, you might try using a mixture of PFA and a small percentage of glutaraldehyde (e.g., 4% (w/v) PFA plus 0.1% (w/v) glutaraldehyde in PBS), although glutaraldehyde-containing fixatives have been seldom used in expansion microscopy studies to date. The 4% (w/v) PFA in PBS is typically prepared freshly prior to the fixation.
The fixation protocol described below has demonstrated consistent results. Note that all the washing steps should be performed quickly to avoid sample dehydration.
4.Replace the cell culture medium with 4% (w/v) PFA in PBS and incubate for 10 min at room temperature.
5.Remove the PFA solution and wash the cells for 5 min with 100 mM glycine in PBS to quench the fixation.
6.Remove the glycine solution and wash the cells twice for 5 min each with PBS.
7.We recommend moving to the next steps of the protocol immediately.
Immunostaining/immunohistochemistry (optional)
For immunostaining after fixation, follow protocols of your choice; you can essentially perform conventional histology. The protocol described below has demonstrated consistent results with fixed cells prepared by the protocols described earlier.
8.Permeabilize the fixed cells by applying 0.1% (w/v) Triton X-100 in PBS at room temperature for 15 min. To reduce nonspecific antibody binding, apply blocking buffer at room temperature for 15 min.
9.Incubate the cells with primary antibodies in the blocking buffer, at the appropriate concentration. The incubation can be done on a shaker at low speed for a period ranging from 1 hr to overnight, at room temperature or at 4°C, depending on the antibody specifications.
10.Remove the antibody solution and wash four times, each time for 5 min with blocking buffer.
11.Incubate the cells with secondary antibodies in blocking buffer on a shaker at low speed for 2 to 4 hr at room temperature or overnight at 4°C. If using antibodies conjugated with dyes, see Support Protocol 2 for a discussion of dye compatibility with pre-expansion staining proExM.
12.Remove the antibody solution and wash four times, each time for 5 min with blocking buffer.
13.We recommend moving to the next steps of the protocol immediately.
Gelation
14.Prepare Acryloyl-X SE (AcX)/DMSO stock solution, monomer solution (Stock X) TEMED stock solution, and ammonium persulfate (APS) stock solution as instructed in Reagents and Solutions.
15.Replace the sample buffer (e.g., the blocking buffer if immunostained following the protocols above) with 0.1 mg/ml Acryloyl-X SE (AcX) in PBS.
16.Leave the cells in the 0.1 mg/ml AcX solution for 2 to 3 hr at room temperature.
17.When cells are cultured on a petri dish, the gelation chamber can be constructed in the meantime, by placing two spacers on a slide, separated from each other by a distance large enough so that the sample cover glass can fit in between (Fig. 2A). Thin and flat objects, such as stacks of coverslips, can serve as the spacers. For example, a stack of two No. 1.5 coverslips has a height of about 0.30 to 0.38 mm; by changing the number or types of the coverslips, the height of the spacers can be adjusted accordingly to match the cell culture substrate (i.e., the sample cover glass) height. The spacer height should be kept close to the cell culture substrate height to avoid leaving excessive gel on top of the sample, but the total thickness of the gel [i.e., (spacer height) – (cell culture substrate height)] should be at least 0.15 mm for easier gel handling in later steps. A droplet of water (e.g., a few µl) can be applied to the spacers to adhere them to the slide (and, if multiple spacers, to each other). If a removable-chamber cover glass is used for the cell culture during steps 2 to 3, the cover glass itself and the gasket can serve as the gelation chamber and the spacer, respectively. In this case, the upper structure of the removable-chamber cover glass can be removed via a removal tool (Sigma, cat. no. GBL 103259). For the gelation chamber lid, a thick and robust coverslip (e.g., a No. 2 coverslip) wrapped in Parafilm can be used, with the Parafilm preventing the gel from sticking to the coverslip when removing the lid in later steps. Make sure the Parafilm surface is flat, clean, and free of folds. We recommend finishing preparation of the gelation chamber and the lid before moving to the next step.
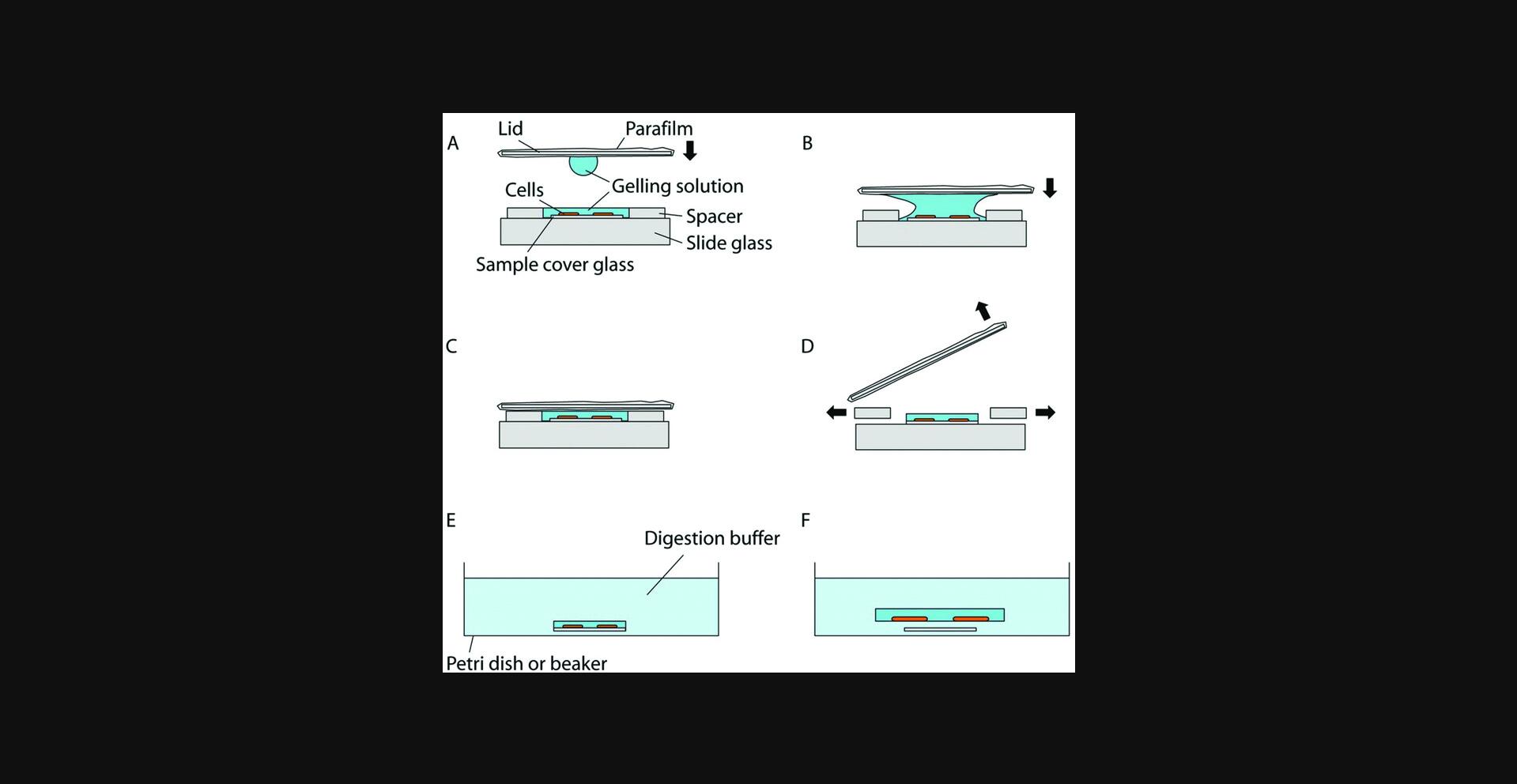
18.After incubating the cells in AcX solution, wash the cells twice, each time for 15 min, with PBS. Begin thawing the components for the gelling solution for proExM—the Stock X, APS, and TEMED stock solutions. Keep the solutions chilled in an ice bath or on a cold block (chilled to 4°C).
19.Create the gelling solution for proExM now by mixing Stock X, water, TEMED stock solution, and APS stock solution in a 47:1:1:1 ratio (gelling solution; also see recipes in Reagents and Solutions), in this order (i.e., APS last) in a microcentrifuge tube, and vortex for a few seconds.
20.Immediately after the previous step, remove the PBS from the cells using a pipet and add a small amount of the mixed gelling solution to the cells on the cell culture substrate. If a removable-chamber cover glass is used, remove the PBS and add the gelling solution to fill in the chamber, then continue to step 22.
21.Use a pair of forceps to pick up and place the cell culture substrate (i.e., the coverslip on which cells are cultured) with the cultured cells between the spacers in the gelation chamber. Make sure the cells are facing upward. Slightly press the cell culture substrate down, to hold it in place. If the gelling solution flows away, reapply a small amount of gelling solution to the cells.
22.Add a droplet (∼20 µl) of gelling solution to one side of the Parafilm-covered lid, and flip it over so that the droplet hangs from the surface due to surface tension (Fig. 2A). Slowly lower the lid so that the droplet will fuse with the gelling solution on the sample coverslip (Fig. 2B). Once fused, continue lowering the lid so it rests on the spacers. Make sure no air pockets are introduced inside the solution during this process.
23.Using a pipet with a fine tip, keep adding additional gelling solution to both open sides of the chamber until the space between the lid, spacers, and slide is filled with gelling solution (Fig. 2C).
24.Place the gelation chamber in an incubator at 37°C for 1 hr for polymerization. Be careful not to tilt or shake the chamber during the transfer and the gelation.
Digestion
25.Take out the gelation chamber from the incubator.
26.Slowly insert a razor blade from the side between the lid and the spacers and pry the lid off. Once the lid is separated from the gel, use the razor blade to remove the spacers (Fig. 2D). If a removable-chamber cover glass is used, remove the lid, then use forceps to peel off the black gasket without removing the gel from the cover glass.
27.Using a razor blade, trim the gel to a small volume around the sample. This will keep the gel compact and aid in finding cells in later steps. Use the razor blade to remove the cell culture substrate and the gel, together, from the glass slide. If a removable-chamber cover glass is used, trim the gel but do not remove the gel from the cover glass.
28.Prepare digestion buffer as described in Reagents and Solutions (final concentration of ProK: 8 U/ml).
29.Since the gel will expand by about 1.5× during digestion, choose a suitably large container (e.g., petri dish) for the digestion. Also, use a separate container for each sample to avoid sample mix-up. Place the cell culture substrate with the gel in the container and fill it with digestion buffer of volume at least 10-fold excess of that of the gel (Fig. 2E). Immerse the gel in digestion buffer for at least 2 to 3 hr until it naturally peels off from the sample coverslip (Fig. 2F). If a removable-chamber cover glass is used, use a diamond scribe to separate each sample and its corresponding piece of the cover glass, by carefully scribing the cover glass and breaking each piece of glass off bearing its piece of gel. Place each piece of cover glass with the gel in a container and immerse in the digestion buffer as described above. A Nunc 4-well rectangular plate, for example, is convenient to contain several pieces of cover glass for digestion. The detached cell substrate can be removed using forceps, to leave only the cell-containing gel in the digestion buffer.
30.Leave the gel immersed in the digestion buffer overnight at room temperature in the dark.
Storage and expansion
31.If not done previously, remove the now-detached cell substrate (e.g., coverslip) from the digestion container carefully using forceps without disturbing the sample gel. Remove the digestion buffer and add PBS, and store the gel at 4°C in the dark if storage for later imaging is desired.
32.If the gel needs to be transferred to another container (e.g., a larger container for trimming, or the sample holder for imaging), you can use a paintbrush of suitable size to pick up and move the gel. To avoid gel dehydration, add some PBS to the container.
33.Before expanding the sample, we recommend trimming the gel to the minimum size necessary. You can use a dissection microscope or a low-magnification wide-field microscope to locate the region of interest. To minimize sample movement, most of the surrounding liquid can be temporarily removed during trimming. Under the microscope, use a razor blade to cut and remove gel outside of the region of interest. We advise trimming the gel into an asymmetric shape to easily deduce the orientation. Make sure to trim the gel down to a reasonable size for handling and imaging, as the expansion will introduce an additional 2 to 2.5× (to a total of about 4.5×) linear increase in size. Transfer the gel with the regions of interest to a container (e.g., a petri dish of suitable size for expansion, or the sample holder for imaging) that is large enough to contain the expanded gel.
34.Fill the container and immerse the gel in water and wait for 20 min. Exchange the water and wait for another 20 min. Redo the exchange one more time (20 min in fresh water, three times in total).
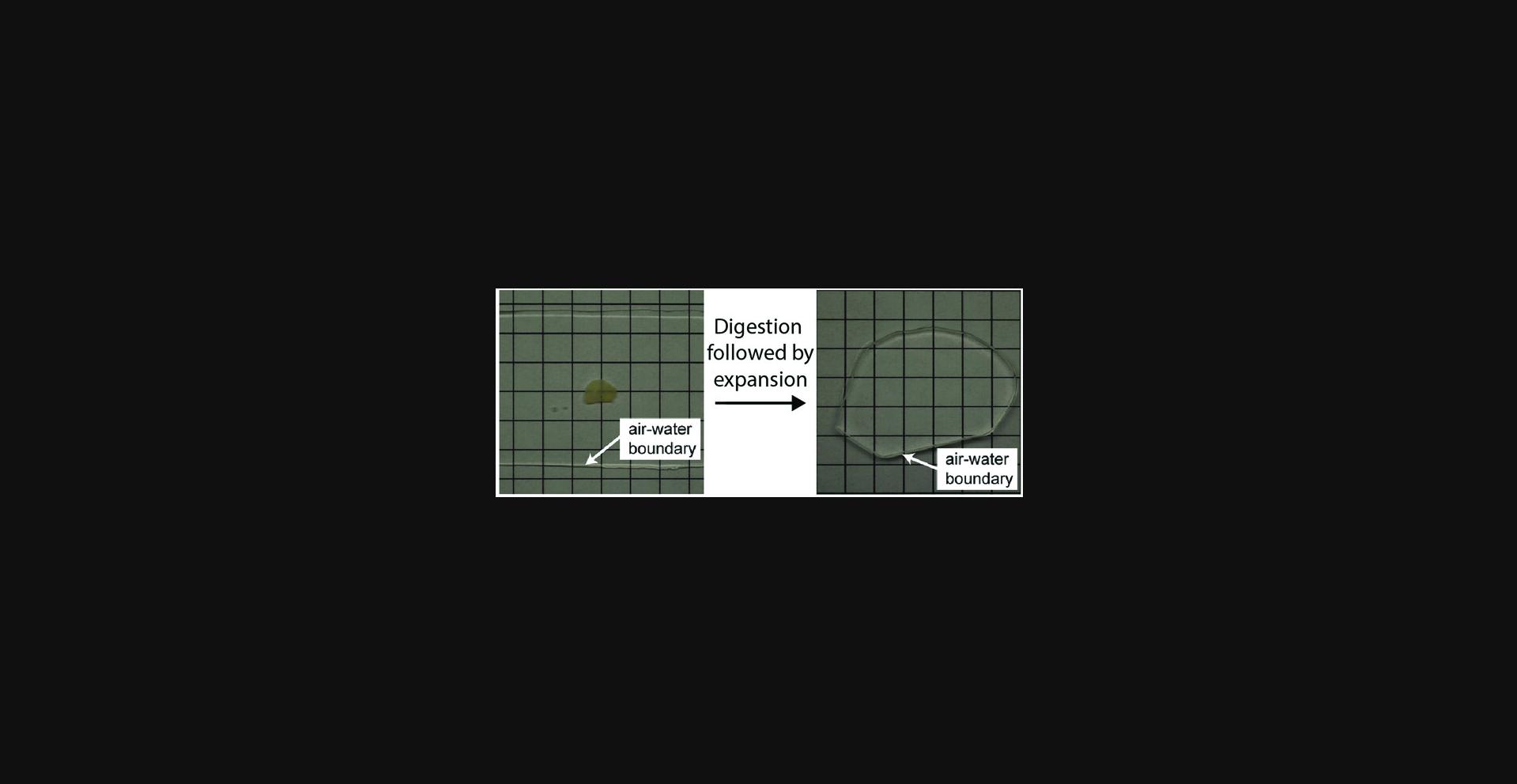
35.If you need to transfer the expanded gel to a new container, it needs to be handled with care, as it is somewhat delicate in the expanded state. First remove most of the surrounding water and then use a brush to move the gel onto a clean glass coverslip placed in the same container. Next, gently lift the coverslip (with the gel on top) out of the container with forceps. The gel can be transferred by slowly brushing it off the coverslip to the new container. This method can be also used to flip the gel by inverting the coverslip bearing the gel, in case the gel is facing in the wrong direction.
Basic Protocol 2: proExM for Intact Tissues
The basic protocol for intact tissues differs slightly from that for cultured cells. Due to the thickness of the samples, intact tissues require a longer time for AcX diffusion. Furthermore, the gelling solution is supplemented with a polymerization inhibitor to allow for longer diffusion of the gelling solution through the samples before the polymerization begins.
Materials
-
Phosphate-buffered saline (PBS; Current Protocols, 2001)
-
4% (w/v) paraformaldehyde (PFA) in PBS or, for stronger fixation, a mixture of 4% (w/v) PFA and 0.1% (w/v) glutaraldehyde in PBS
-
Sinking solution (see recipe)
-
Dry ice
-
2-methylbutane
-
Optimal cutting temperature compound (OCT), M-1 or another embedding matrix
-
0.1% (w/v) Triton X-100 in PBS
-
Blocking buffer: 5% (v/v) normal donkey serum in 0.1% (w/v) Triton X-100 in PBS (store up to 1 month or longer at 4°C): the serum should be from the animal host of the secondary antibodies, so other types of sera should be used instead of normal donkey serum if the secondary antibodies are from other animals
-
Primary and secondary antibodies for immunostaining
-
AcX/DMSO stock solution (see recipe)
-
Gelling solution for expansion of intact tissues (see recipe)
-
Digestion buffer (see recipe)
-
Vibratome or cryostat microtome
-
22 × 22 mm No. 1.5 coverslips (0.15 to 0.19 mm thickness)
-
22 × 22 mm No. 2 coverslips (0.19 to 0.23 mm thickness)
-
Microscope slides
-
Parafilm
-
Microcentrifuge tubes
-
Paintbrush
-
Ice bath or cold block chilled to 4°C
-
Razor blade
-
Forceps
-
Petri dishes
-
Dissection microscope
Tissue preparation
In this basic proExM tissue protocol, the thickness of the tissue or the tissue slice is assumed to be <200 µm in its thinnest dimension. For tissue samples with thickness above 200 μm, see Basic Protocol 3.
Tissues can be fixed using protocols of your choice. For instance, immersion or perfusion fixation with 4% (w/v) paraformaldehyde (PFA) in PBS can be used. For stronger fixation that preserves more ultrastructure, you might try a mixture of PFA and a small percentage of glutaraldehyde (e.g., 4% (w/v) PFA plus 0.1% (w/v) glutaraldehyde in PBS), although glutaraldehyde-containing fixatives have been less used in expansion microscopy protocols to date. The 4% (w/v) PFA in PBS solution is typically prepared freshly prior to the fixation.
1.After fixation, if tissues are thicker than 200 µm, cut them into <200 µm slices using a vibratome. Alternatively, for slicing in a cryostat, the tissue needs first to be cryoprotected by immersing it in sinking solution until the tissue sinks to the bottom. The tissue is then taken out and frozen using dry ice and 2-methylbutane. After embedding it in OCT, M-1 or another embedding matrix, the sample is ready to be sliced using a cryotome.
Immunostaining/immunohistochemistry (optional)
For immunostaining after fixation, follow protocols of your choice. The protocol described below has demonstrated consistent results with fixed brain tissues prepared by the protocols described earlier.
2.Permeabilize the fixed tissue by applying 0.1% (w/v) Triton X-100 in PBS at room temperature for 15 min, then in blocking buffer at room temperature for 6 hr.
3.Incubate the tissues with primary antibodies in the blocking buffer. The incubation can be done on a shaker at low speed overnight at room temperature or at 4°C.
4.Remove the antibody solution and wash four times, each time for 30 min with blocking buffer, to remove unbound primary antibodies.
5.Incubate the tissues with secondary antibodies in the blocking buffer on a shaker at low speed overnight at room temperature or at 4°C. If using antibodies conjugated with dyes, see Support Protocol 2 for a description of dye performance and compatibility with pre-expansion staining proExM.
6.Remove the antibody solution and wash four times, each time for 30 min with blocking buffer to remove unbound secondary antibodies.
7.We recommend moving to the gelation steps immediately.
Gelation
8.Prepare Acryloyl-X SE (AcX)/DMSO stock solution, monomer solution (Stock X), TEMED stock solution, ammonium persulfate (APS) stock, and 4-hydroxy-TEMPO (4HT) solution as instructed in Reagents and Solutions.
9.Replace the sample buffer (e.g., the blocking buffer if immunostained following the protocols above) with 0.1 mg/ml Acryloyl-X SE (AcX) in PBS.
10.Leave the tissues in AcX solution for >6 hr (overnight is fine) at room temperature with no shaking.
11.Meanwhile, to construct the gelation chamber, place two spacers on a slide, separated from each other (Fig. 4A). Thin and flat objects, such as stacks of coverslips, can serve as the spacers. For example, a stack of two No. 1.5 coverslips has a height ranging from 0.30 to 0.38 mm, and by changing the number or types of the coverslips, the height of the spacers can be adjusted to accommodate the tissue thickness. The spacer height should be kept close to the tissue thickness to avoid leaving excessive gel on top of the sample, but the total thickness of the gel (i.e., the spacer height) should be at least 0.15 mm for easier gel handling in later steps. A droplet of water (e.g., a few µl) can be applied to spacers to adhere them to the slide (and, if multiple spacers, to each other). For the gelation chamber lid, a thick and robust coverslip (e.g., a No. 2 coverslip) wrapped in Parafilm can be used, with the Parafilm preventing the gel from sticking to the coverslip when removing the lid in later steps. Make sure the Parafilm surface is flat, clean, and free of folds. We recommend finishing preparing the gelation chamber and the lid before moving to the next step.
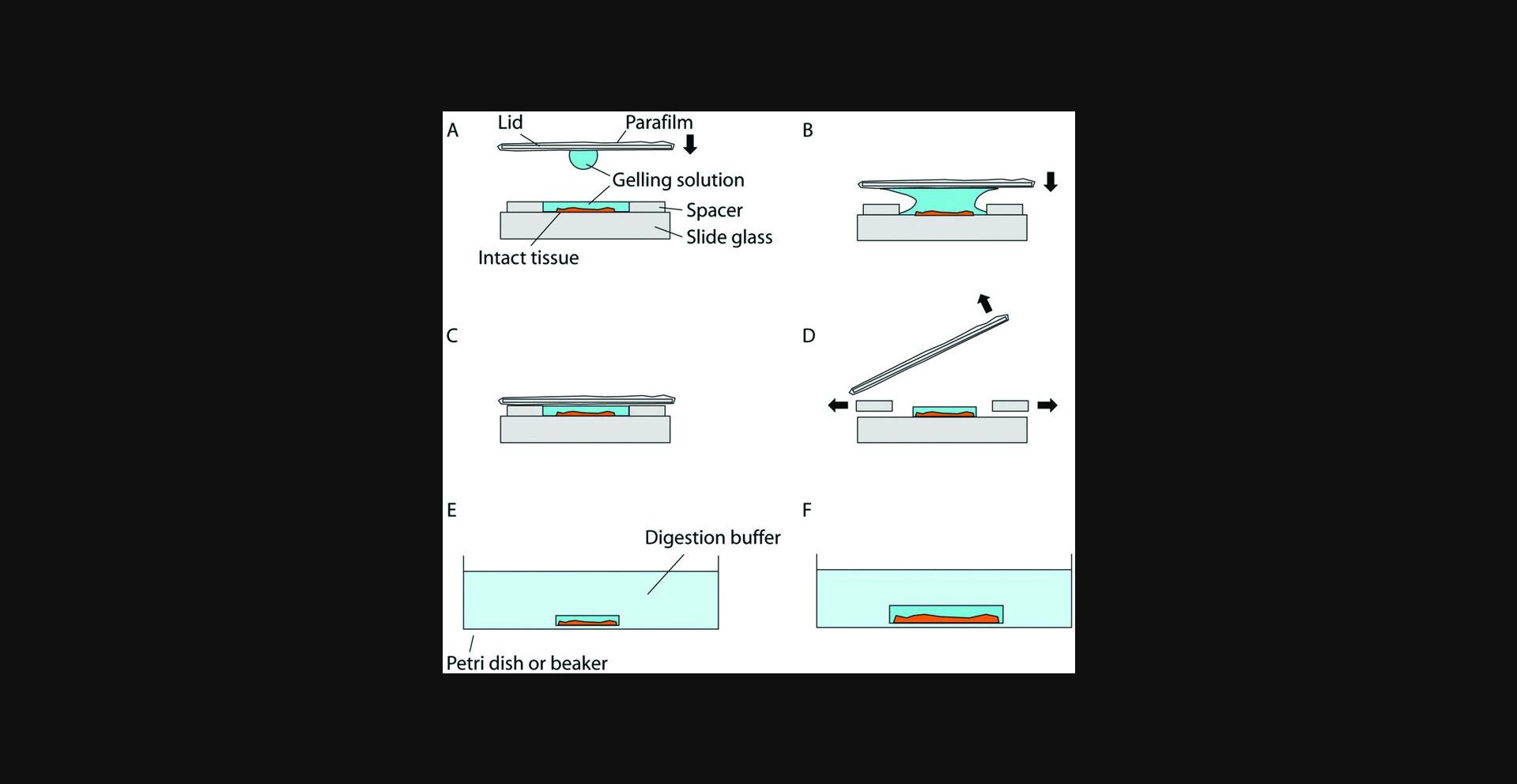
12.After incubating the tissues in the AcX solution, wash the tissues twice, each time for 15 min, with PBS. Begin thawing the components for the gelling solution for proExM: Stock X, 4HT, APS and TEMED stock solutions. Keep the solutions chilled in an ice bath or on a cold block (chilled to 4°C).
13.Add Stock X, 4HT, TEMED and APS in a 47:1:1:1 ratio in this order (i.e., APS last) into a microcentrifuge tube (gelling solution; also see recipe in Reagents and Solutions), and vortex for a few seconds. The amount of gelling solution should be scaled up or down accordingly to the size of the gelation chamber and the size of the tissue or tissue slice and should be at least 100-fold excess in volume. For instance, at least 400 µl of gelling solution is used to incubate a 100-μm single coronal slice of mouse brain, enough to fill a gelation chamber with a 22 × 22 × 0.38 mm void, which can fit a full coronal mouse brain slice. To prevent premature polymerization of the gelling solution, APS should be added to the solution last before vortexing. In addition, the mixed gelling solution should be kept in an ice bath or on a cold block (chilled to 4°C). Avoid excessive warming of the gelling solution by, for example, holding the lid of the microcentrifuge tube rather the body of the tube. Finally, we recommend completing the next steps (until placing the microcentrifuge tube with the gelling solution and the sample at 4°C in step 15) within 5 min to prevent premature gelation.
14.Immediately after the previous step, use a soft paintbrush to transfer and immerse the tissues in the gelling solution in the microcentrifuge tube.
15.Keep the microcentrifuge tube containing the sample and the gelling solution at 4°C in the dark for 30 min. After the 30 min incubation, we recommend completing the next few steps (until placing the gelation chamber in a 37°C incubator in step 19) within 5 min to prevent premature gelation.
16.Use the paintbrush to transfer and place the tissue slice between the spacers in the gelation chamber. Make sure the slice is lying flat without wrinkles or distortions. We recommend first adding a droplet of 20 µl of the gelling solution in between the spacers, transferring the tissue slice into the droplet, and using the paintbrush to slowly flatten the tissue within the liquid environment, gently pressing it onto the glass surface.
17.Add a droplet of 20 µl of the gelling solution to one side of the lid, and flip it over so that the droplet hangs from the surface due to surface tension (Fig. 4A). Slowly lower the lid so that the droplet will fuse with the gelling solution on the sample coverslip (Fig. 4B). Once fused, continue lowering the lid so it rests on the spacers. Make sure no air pockets are introduced inside the solution during this process.
18.Using a pipettor and a fine pipet tip, keep adding additional gelling solution to both open sides of the chamber until the space between the lid, spacers, and the slide is filled (Fig. 4C).
19.Place the gelation chamber in an incubator at 37°C for 2 hr for polymerization. Be careful not to tilt or shake the chamber during the transfer and the gelation.
Digestion
20.Take out the gelation chamber from the incubator.
21.Slowly insert a razor blade from the side between the lid and the spacers and pry the lid open. Once the lid is separated from the gel, continue to use the razor blade to remove the spacers in a similar fashion (Fig. 4D).
22.Using a razor blade, trim off any excess gel.
23.Prepare digestion buffer as described in Reagents and Solutions (final concentration of ProK is 8 U/ml).
24.Use a paintbrush to wet the gel and its sides with the digestion buffer. Wait for 10 to 30 sec, until the digestion buffer soaks the gel border.
25.Peel off the gel by gently probing the space between the gel and the glass surface with a fine paintbrush. Since the gel will expand to about 1.5× during digestion, choose a suitably large container, such as a petri dish, for the digestion step. For small tissue and tissue slice samples, 1.5-ml microcentrifuge tubes can be used as the container. Use separate containers filled with digestion buffer for each sample. Leave gels immersed in digestion buffer for >8 hr (overnight is fine) at room temperature in the dark (Fig. 4E, F).
Storage and expansion
26.Remove the digestion buffer and add PBS, and store the gel at 4°C in the dark if storage for later imaging is desired. Be cautious not to suck up the gel while pipetting.
27.If the gel needs to be transferred to another container (e.g., a larger container for trimming or the sample holder for imaging), you can use a paintbrush of suitable size to pick up and move the gel. To avoid sample dehydration, add some PBS to the new container.
28.Before expanding the sample, we recommend trimming the gel to the minimum size necessary. You can use a dissection microscope or a low-magnification wide-field microscope to locate the region of interest. To minimize sample movement, most of the surrounding liquid can be temporarily removed during trimming. Under the microscope, use a razor blade to cut and remove gel outside of the region of interest. We advise trimming the gel into an asymmetric shape to easily deduce the orientation. Make sure to trim the gel to a reasonable size for handling and imaging, as the expansion will introduce an additional 2× to 2.5× (to a total of about 4.5×) linear increase in size. Transfer the gel with the regions of interest to a container (e.g., a petri dish of suitable size for expansion, or the sample holder for imaging) that is large enough to contain the expanded gel.
29.Fill the container, immerse the gel in water, and wait for 20 min. Replace the water with fresh water and wait for another 20 min. Redo the exchange one more time (20 min in fresh water for three changes in total). The sample should be fully expanded and optically cleared (see Fig. 3 for an expanded mouse brain hemi-slice) and ready for imaging (these times are shorter than in previous publications, but reflect numbers that work for routine use in our lab). An example of pre-expansion and post-expansion images of a brain slice containing Brainbow 3.0-expressing neurons is shown in Figure 5.For long-term imaging, e.g., when imaging a large volume of specimen, sample mounting is necessary after the expansion and before the imaging (see Basic Protocol 6). Sample mounting attaches the sample to the sample holder and thus prevents it from drifting, and can also help keep the specimen within the working distance of the objective lens. For a quick check of the expanded sample under the microscope (<5 min), there is typically no need for sample mounting. On an inverted microscope, for example, you can use a pipet to temporarily (<5 min) remove the water around the gel to prevent it from sliding away.
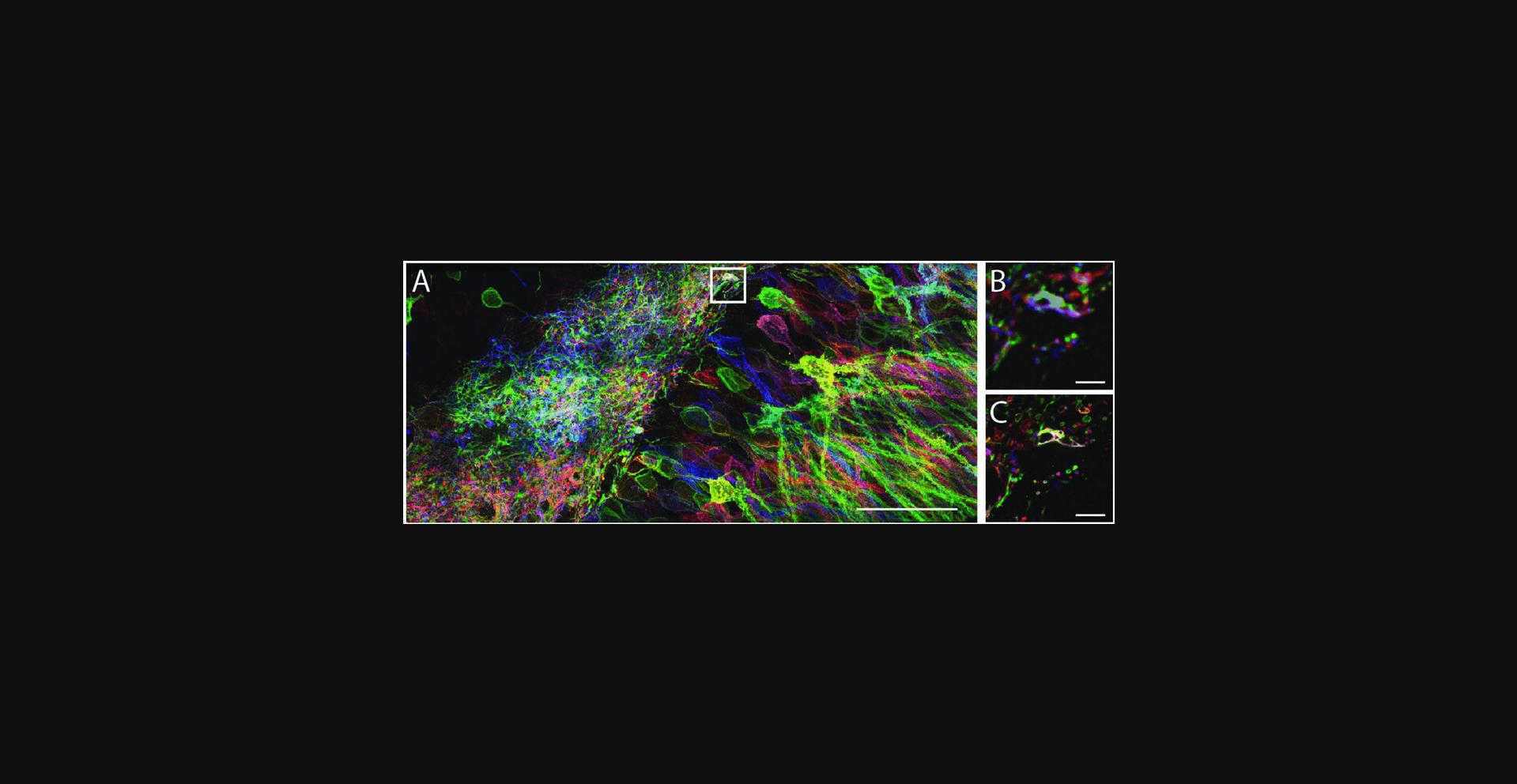
30.If you need to transfer the expanded gel to a new container, it needs to be handled with care, as it is somewhat delicate in the expanded state. First, remove most of the surrounding water and then use a brush to move the gel onto a clean glass coverslip placed in the same container. Next, gently lift the coverslip (with the gel on top) out of the container with forceps. The gel can be transferred by slowly brushing it off the coverslip to the new container. This method can be also used to flip the gel by inverting the coverslip bearing the gel, in case the gel is facing in the wrong direction.
Basic Protocol 3: proExM for Thick Intact Tissue Samples (200 to 500 μm)
For tissues and tissue slices of 200 to 500 μm thickness, the basic tissue protocol (Basic Protocol 2) needs to be modified to allow for deeper penetration of reagents. In addition, the digestion time needs to be prolonged. We have successfully used this protocol to expand samples of up to 500 μm thickness; expanding samples thicker than 500 μm may be possible as well.
Additional Materials (also see Basic Protocol 2)
- 2-(N -morpholino)ethanesulfonic acid (MES)–buffered saline (MBS): 100 mM MES/150 mM NaCl in water, adjusted to pH 6
1.Tissue sample generation is outlined in steps 1 to 7 of Basic Protocol 2, and can be used for thicker tissue samples.
2.For the AcX step (Basic Protocol 2, step 9), a slightly acidic buffer should be used to suppress the reactivity of the NHS ester of the AcX molecule to allow for deeper penetration into the tissue. We typically use MES-buffered saline (MBS) for this slightly acidic buffer. Dilute the 10 mg/ml AcX/DMSO stock solution 1:100 in the MBS. Leave tissue in the AcX in MBS solution for up to 24 hr at room temperature, depending on the thickness of the sample. We typically allow more time for thicker tissues. For instance, we leave a 500-μm brain slice in AcX/MBS solution for 24 hr.
3.For the gelling solution preparation (Basic Protocol 2, step 13), increase the amount of 4HT by 50% (i.e., 47: 1.5 ratio between the Stock X and 4HT) for longer inhibition of polymerization during the incubation. For the incubation (Basic Protocol 2, step 15), submerge the sample in the gelling solution for 45 to 60 min (instead of 30 min) at 4°C before transferring it to the gelation chamber.
4.For the digestion (Basic Protocol 2, step 25), a longer and stronger digestion is necessary. The ProK digestion can be carried out for a longer time and/or at higher temperature (up to 50° to 60°C), depending on the biological composition of the tissue and how strongly it is crossed-linked after fixation. For a 250-μm thick mouse brain slice, for example, a 2-day digestion at room temperature has yielded adequate digestion of the sample. In this case, the gelled brain slice was digested in digestion buffer (with ProK) at room temperature for 1 day, then the old buffer was exchanged with fresh digestion buffer (with ProK) and digested for another day.
Support Protocol 1: Post-Expansion Immunostaining for proExM
Immunostaining can be performed after expansion, as illustrated in Figure 1C. One can perform the mechanical homogenization step using ProK, then add antibodies afterward. However, only some epitopes, such as YFP, can survive the ProK treatment and can be stained post-expansion; most epitopes do not.
To preserve most epitopes, a milder method, such as protein denaturing by autoclaving in an alkaline detergent-rich buffer (or, alternatively, treatment with a mild protease that cuts at only a small number of sites within proteins, like LysC), can be used for mechanical homogenization (Fig. 1C, also see Supplementary Figure 1 of Tillberg et al., 2016). We describe the protocol for autoclave-mediated disruption below. For examples using various antibodies, see Supplementary Figure 1 of Tillberg et al., 2016.
The following protocol refers to Basic Protocol 2, and an example using Thy1-YFP mouse brain slices and post-expansion anti-GFP staining is included (Fig. 6).
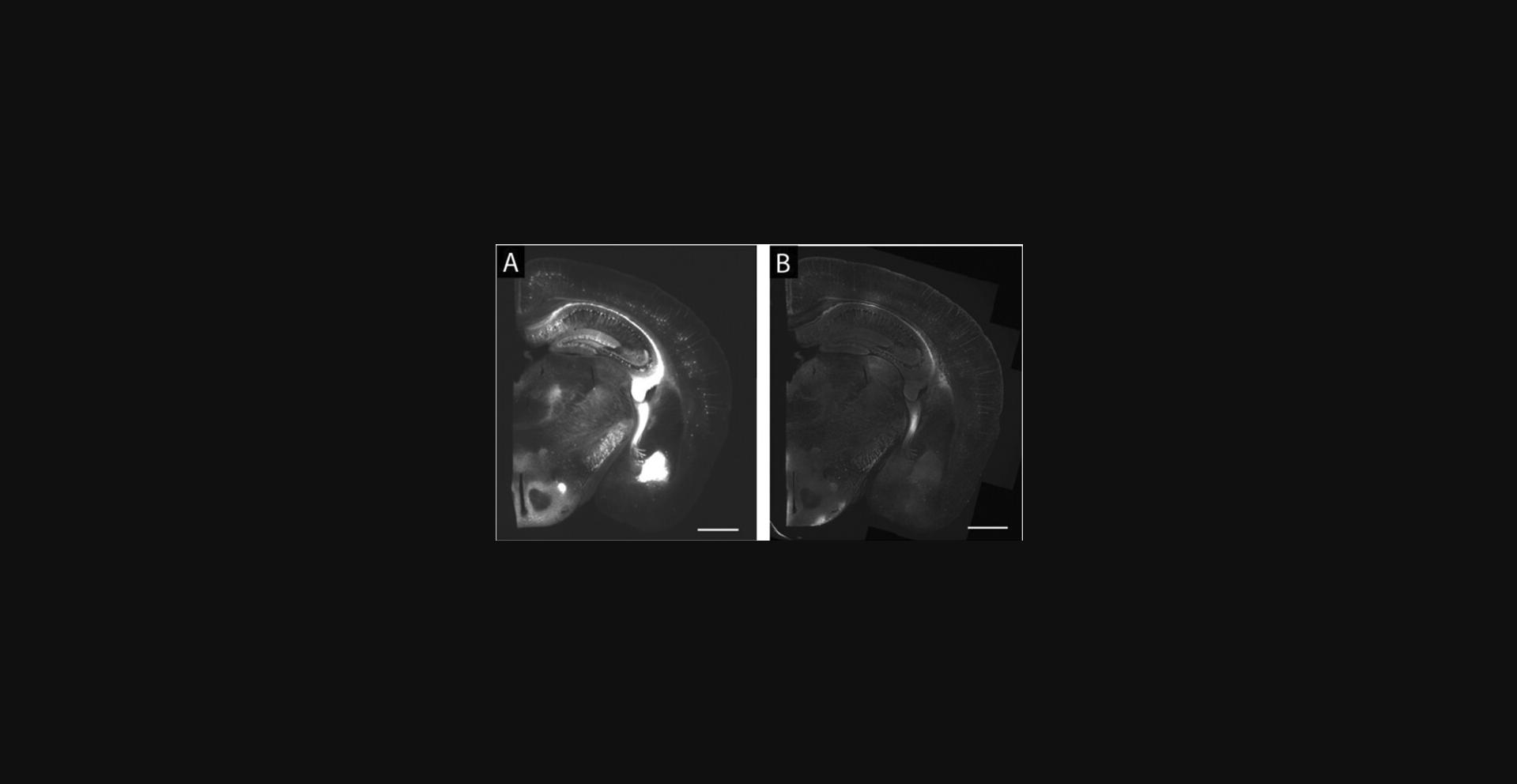
Additional Materials (also see Basic Protocol 2)
- Alkaline buffer: 100 mM Tris base, 5% (w/v) Triton X-100, 1% (v/v) SDS, in water
1.Infuse a mouse brain slice with AcX and embed in a hydrogel as described in Basic Protocol 2.
2.Soak the sample in the alkaline buffer for 15 min, then replace the solution with fresh alkaline buffer of the same composition. Place the sample, immersed in the buffer, in an autoclavable container (e.g., polypropylene bottle), leaving the lid slightly open to allow for pressure relief. Autoclave the samples in the alkaline buffer on liquid sterilization mode, at a temperature of 121°C, for 1 hr. During this and subsequent steps, the sample will expand visibly.
3.Let the sample cool down to room temperature and wash twice, each time for 15 min, with PBS.
4.Incubate the sample with primary antibodies in 0.1% (w/v) Triton X-100 plus 5% (v/v) normal donkey serum in PBS (blocking buffer) on a shaker at low speed overnight at room temperature or 4°C.
5.Remove the primary antibody solution and wash four times, each time for 30 min, with blocking buffer.
6.Incubate the sample with fluorescently labeled secondary antibody of choice in the blocking buffer on a shaker at low speed overnight at room temperature or 4°C.
7.Remove the secondary antibody solution and wash four times, each time for 30 min, with blocking buffer.
8.Replace the buffer with PBS for storage (for up to several weeks), if desired. Expand to the final factor of ∼4.5× with three 20-min washes in water, and then proceed with imaging (see Basic Protocol 6).
Support Protocol 2: Fluorescent Proteins, Antibodies, and Dyes for proexm
For the variation of proExM illustrated in Figure 1C (post-expansion staining proExM), any dye conjugated to the secondary antibody should be compatible with the protocol, as the dye is only administered at the end of the process.
For the variations of proExM illustrated in Figure 1A,B (pre-expansion staining proExM), most fluorescent proteins are compatible with the previously described basic proExM protocols (see Fig. 1b of Tillberg et al., 2016). For example, β-barrel (e.g., GFP-like) fluorescent proteins are protease resistant and can survive ProK digestion quite well (e.g., >50% fluorescence retention for many fluorescent proteins), but non-β-barrel fluorescent proteins (e.g., infrared fluorescent proteins based on bacteriophytochromes) are degraded by the ProK step. For antibodies conjugated with fluorescent dyes, most dyes are compatible with the basic proExM protocols (e.g., >40% fluorescence retention) except for the cyanine-family dyes (Fig. 1c of Tillberg et al., 2016). Cyanine-family dyes, such as Cy3, Cy5, and Alexa Fluor 647, are degraded during the polymerization reactions. For far-red dyes, accordingly, we recommend Atto 647N or CF 633.
Basic Protocol 4: Exfish for Cultured Cells
ExFISH enables expansion microscopy of RNA by using a small-molecule linker, which we call LabelX, to covalently anchor endogenous RNA molecules within specimens to the swellable gel of ExM. LabelX is analogous to AcX in proExM, but enables RNA molecules to be interrogated after expansion using RNA FISH. For ExFISH-processed cultured cells, single-molecule FISH (smFISH) is used to stain RNA molecules post-expansion.
Materials
- Phosphate-buffered saline (PBS; Current Protocols, 2001)
- 10% neutral buffered formalin (NBF; EMS, cat. no. 15742-10)
- 70% (v/v) ethanol in nuclease-free water
- MOPS buffer (see recipe)
- LabelX (see recipe)
- VA-044 stock solution (see recipe)
- Nitrogen gas source
- Digestion buffer (see recipe)
- Nuclease-free water
- Wash buffer for smFISH (WA-10; see recipe)
- smFISH hybridization buffer (see recipe)
- smFISH probes: we primarily use commercially available smFISH probes (e.g., Stellaris ® RNA-FISH probes from LGC Biosearch Technologies). Alternatively, it is possible to design custom smFISH probes conjugated to a fluorophore of one's choice (Raj & Tyagi, 2010).
- Parafilm
- Tupperware container (modified as described in the text below) as an airtight chamber
- 16-well Culturewell removable-chamber cover glasses from Grace Bio Labs (Sigma, cat. no. GBL112358)
- Chamber coverglass removal tool (Sigma, cat. no. GBL 103259)
- Vacuum desiccator and vacuum source
- Glass microscope slides, 1-mm-thick
- Kapton Tape or Scotch Tape
- 60°C incubator
- Razor blades
- Diamond scribe for cutting (scribing) thin glass
- Forceps
- Nunc 4-well rectangular plates (e.g., Thermo Fisher, catalog no. 267061)
- Epoxy glue
Cell culture preparation
Cells can be grown as desired to suit experimental needs. However, for ease of gelation and imaging, as well as handling, we have found 16-well Culturewell removable-chamber cover glasses from Grace Bio Labs (Sigma, cat. no. GBL112358) to be convenient for pre-expansion fluorescence in situ hybridization (ExFISH) imaging, as well as subsequent gelation and digestion. In cases where single-molecule FISH (smFISH) is performed before gelation and expansion, we have found it convenient to plate cells in 8-well Nunc Lab-Tek chambered cover glasses (Thermo Fisher, catalog no.155411).
Fixation and permeabilization of cultured cells
1.Wash cells once with PBS warmed to 37°C to avoid any heat shock.
2.Add 10% neutral buffered formalin and fix cells for 10 min at room temperature (all steps are at room temperature unless otherwise indicated). Wash with PBS twice, each time for 2 min at room temperature.
3.Replace buffer with 70% (v/v) ethanol in nuclease-free water for permeabilization. Fixed cells can be stored in 70% (v/v) ethanol in nuclease-free water at 4°C for up to 2 weeks. For immediate use, fixed cells should be permeabilized with 70% (v/v) ethanol in nuclease-free water for 1 hr at room temperature.
LabelX treatment of cultured cells
4.Rehydrate cells permeabilized with 70% (v/v) ethanol in nuclease-free water by washing twice with PBS for 5 min per wash at room temperature. Pre-incubate fixed cultured cells with 20 mM MOPS buffer for 5 min.
5.Prepare LabelX as described in Reagents and Solutions, and then dilute in 20 mM MOPS buffer to the desired concentration.
6.Remove the preincubation MOPS buffer and add LabelX in MOPS buffer to the fixed cultured cells. For cells grown in the removable-chamber cover glass, use 80 μl of LabelX in MOPS buffer. For cells grown in Nunc Lab-Tek chambered cover glasses, use a volume of 120 μl. Incubate overnight at 37°C. Afterwards, wash twice with PBS at room temperature for 5 min each.
Gelation
The Grace removable-chamber cover glass has an upper structure that can be removed with a chamber cover glass removal tool (Sigma, cat. no. GBL 103259). After removal of the chamber, a 1-mm silicone spacer remains with the cover glass and can be used to cast the gel (protocol described below, as well as in Basic Protocol 2). If cells are grown on a coverslip, then a chamber can be made using coverslip spacers (see Basic Protocol 2). If cells are grown in Nunc Lab-Tek chambered cover glasses, we have found that the bottom coverslip along with its 1-mm gasket can be removed from the rest of the plate, and used to cast the gel.
The gelation of fixed cultured cells for ExFISH is different from that of other ExM variants because VA-044, instead of APS, is used as a polymerization initiator. We found that the use of APS/TEMED yields a dim auto-fluorescent background that is significant in the context of smFISH, where the signals themselves are extremely dim. VA-044 is preferable because its use results in minimal auto-fluorescence, which is ideal for smFISH imaging. However, VA-044 has a higher decomposition temperature, and leads to a slower polymerization process. As a result, inhibition due to ambient oxygen can affect gel formation. We have found that degassing followed by perfusion with nitrogen displaces oxygen dissolved in the gelling solution and facilitates gel formation. Therefore, gelation is carried out under nitrogen atmosphere in a humidified chamber, which provides a nitrogen-filled environment and minimizes evaporation.
We typically use a Tupperware container, but any container that provides an airtight seal can be used for the humidified chamber (Fig. 7). We create two holes in the lid of the Tupperware using a syringe needle. To make the chamber humidified, we add a small amount of water to the bottom of the Tupperware. We position an empty 24-well plastic bottom plate at the very bottom of the Tupperware to provide a raised platform onto which we place the cover glass with the cells and gelling solution; the water level should be much lower than the height of the 24-well plate so that the sample being gelled is kept well above the water.
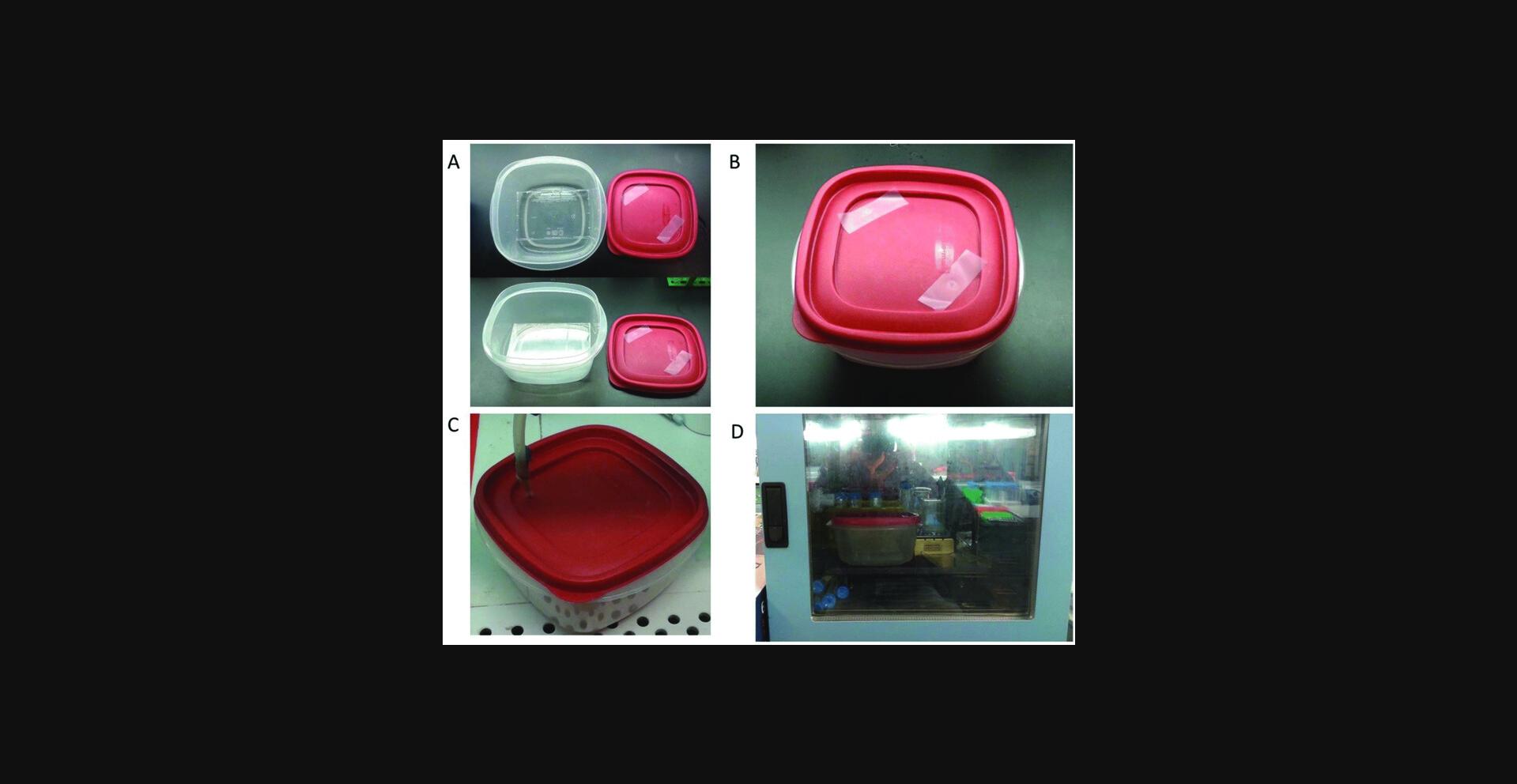
7.For the Grace removable-chamber cover glass : Use the chamber cover glass removal tool to remove the top wells, leaving behind the black silicone gasket. Leave 40 μl of PBS inside the well to keep the cells hydrated. For the Lab-Tek chambered cover glass, first glue the bottom of the cover glass to a 1-mm-thick microscope glass slide for mechanical support, using epoxy glue. After the glue has cured, use a razor blade to pry apart the cover glass from the top wells leaving behind the plastic gasket. Leave 100 μl PBS inside the well to keep the cells hydrated.
8.Prepare gelling solution for ExFISH as follows.
9.In a cold block, or on ice, distribute the gelling solution for ExFISH into 200-μl aliquots in microcentrifuge tubes. Degas for 10 min in a vacuum desiccator.
10.Remove any remaining PBS from the wells on the cover glasses and add the degassed gelling solution for ExFISH. For the Grace removable-chamber cover glass, add 40 μl of gelling solution. Add 200 μl of gelling solution if using the Lab-Tek chambered cover glass. Place the cover glass in a vacuum desiccator and degas for another 10 min. In the meantime, prepare a glass slide covered in Parafilm as the lid (see Fig. 2 and associated text, above). Make sure the Parafilm surface is flat, clean, and free of folds. The Parafilm prevents the gel from sticking to the lid when removing the lid in later steps. Remove the cover glass from the desiccator and immediately place the Parafilm glass slide directly on top of the cover glass. Any excess gelling solution might spill out to the sides. Proceed to the following steps without delay.
11.Place the cover glass onto the plastic platform in the humidified chamber. Seal the chamber (Fig. 7A,B).
12.Remove the tape covering the holes. Connect the inlet of the nitrogen line to a syringe tip and insert the tip through one hole (Fig. 7C). Slowly, turn on the nitrogen flow regulator to flush nitrogen into the chamber. Increase the flow until you can feel the airflow coming out of the other hole. Flush the chamber with nitrogen for 10 min. When done, remove the inlet and immediately seal both holes with tape again (e.g., Kapton Tape or Scotch Tape).
13.Place the chamber in a 60°C incubator for 2 hr to initiate the gelation (Fig. 7D). Be careful not to tilt or shake the chamber during the transfer and the gelation.
Digestion
14.When gelation is finished, take the humidified chamber out of the 60°C incubator and remove the cover glass. Using a razor blade, gently pry open the Parafilm-covered lid from the top of the wells. For the Grace Biolabs removable-chamber cover glass, gently remove the black silicone gasket by peeling it off using your hands or a pair of forceps. For the Lab-Tek chambered cover glass, do not attempt to remove the plastic gasket. At this point, you will see that gels will have formed in the individual wells on the cover glass.
15.For digestion, place the cover glass in a Nunc 4-well rectangular plate. Prepare digestion buffer with proteinase K as described in Reagents and Solutions. Add 6 ml of digestion buffer to the well containing the cover glass (if using any other container, add digestion buffer at at least 10 times the gel volume to completely cover the cover glass and gels). Make sure the gel is entirely immersed for at least 2 to 3 hr until it naturally peels off from the sample cover glass (Fig. 2F). If a removable-chamber cover glass is used, use a diamond scribe to separate each sample and its corresponding piece of the cover glass, by scribing the cover glass and breaking each piece of glass off bearing its piece of gel. Note that the cell culture substrate is placed so that the gel is on top of the substrate and the cultured cells are at the bottom surface of the gel. Optionally, the detached cell substrate can be carefully removed using forceps, to just leave the cell-containing gel in the digestion buffer.
16.Leave the gel immersed in the digestion buffer overnight at room temperature in the dark.
Storage and expansion
17.After digestion, gels can be expanded (temporarily, see below) in a petri dish, or any container large enough to hold the gels. To fully expand, wash gels with excess volume nuclease-free water three times with at least 1 hr per wash. Also see Basic Protocol 1.
smFISH staining of cultured cells after expansion
18.Since the gels formed in either of the above chambered cover glasses have a thickness of ∼1 mm before expansion, the thickness of the expanded gels needs to be cut down to facilitate efficient diffusion of FISH probes through the gel. To shave down fully expanded gels to a thickness of 1 mm, we use 1-mm-thick microscope glass slides as spacers as follows. Prepare a large plastic dish to use as a cutting board. We often use the plastic covers of Nunc 4-well rectangular plates, although any similarly sized flat plastic surface will work. Use a small amount of epoxy to glue two 1-mm-thick glass microscope slides on their flat slide onto the plastic surface. Position the two glass slides such that they are aligned along their longest dimension with a 2.5- to 3-cm space in between. Allow the epoxy to harden.
19.Position a fully expanded gel in between the two glass slides on the plastic cover. The expanded gel should fit in between the two glass slides. If not, trim the gel with a razor blade. Position the gel such that the side of the gel with cultured cells is facing the bottom.
20.Place a razor blade across the glass slides so that it bridges the slides. Carefully slide the razor blade along the glass slides and through the gel to shave off everything except the bottom 1 mm of the gel containing the cells.
21.Carefully collect the shaved gel at the bottom containing the fixed and expanded cells and move it to PBS. If needed, at this point, shaved gels can be stored in PBS at 4°C for up to 1 month.
22.Proceed to smFISH staining, which can be performed in any container, though we often use glass bottom 24-well plates for the convenience of imaging right after staining.
23.Prepare gels by incubating with wash buffer (WA-10) for 30 min at room temperature.
24.Prepare probes by diluting in smFISH hybridization buffer at the concentration indicated by the vendor for smFISH. We typically carry out the hybridization at a total probe concentration of 100 nM. Vortex to mix.
25.Remove the wash buffer from the gels. Add the hybridization buffer with probes onto the gels. Add enough volume to completely cover the gel to be stained (for 24-well plates, we recommend 300 µl). Incubate overnight (or for >6 hr) at 37°C.
26.Wash gels twice with excess volume (e.g., 500 μl for 24-well plates) of WA-10 at 37°C with 30 min per wash.
27.Wash once with excess volume of PBS at 37°C for 30 min.
28.Imaging can be performed in PBS or any other buffer of choice [expansion factor is determined by salt concentration: using regular PBS results in ∼2× expansion; using 0.02× PBS (diluted in water) results in ∼3× expansion while still preserving hybridization].
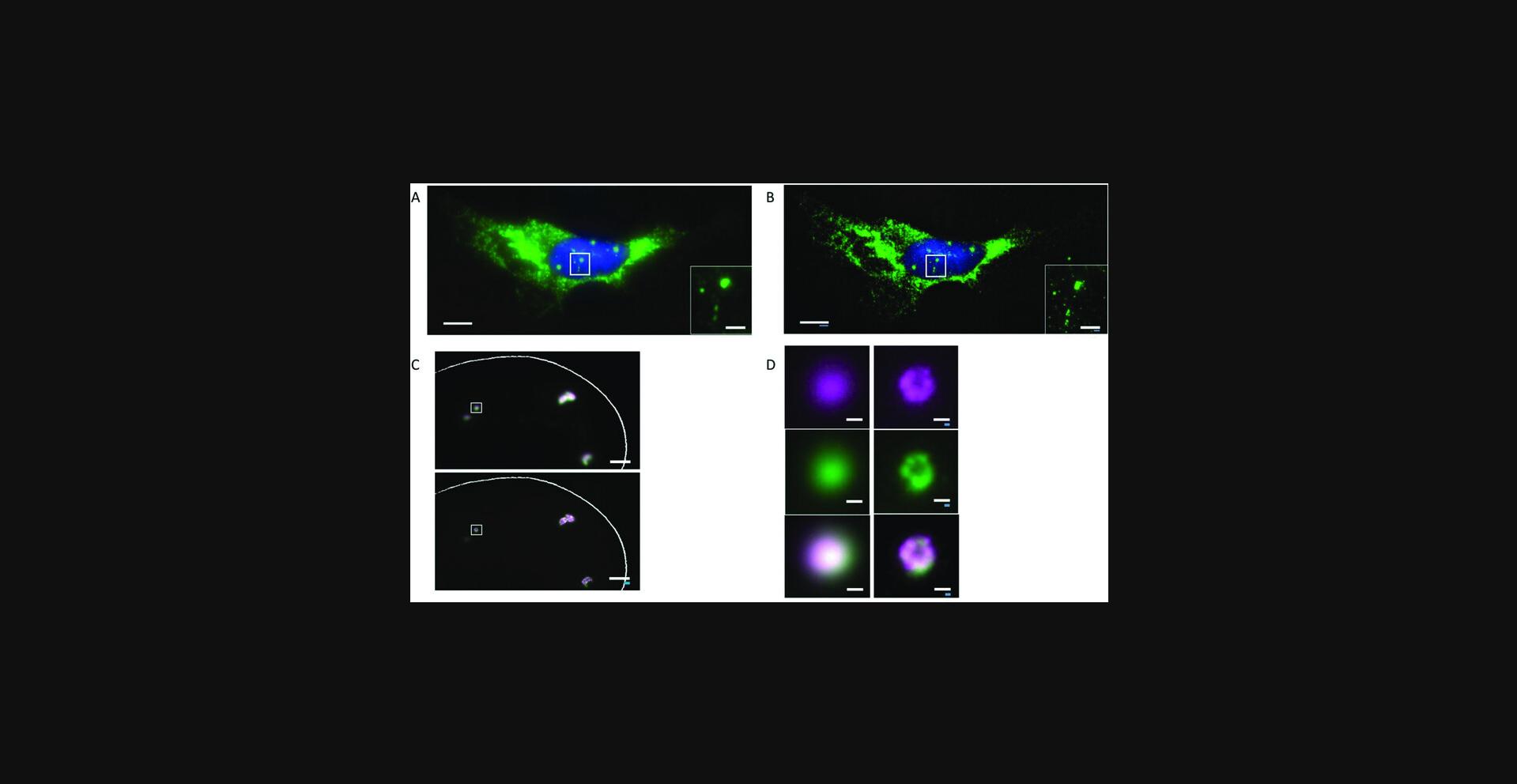
Basic Protocol 5: Exfish for Tissues
The protocol for performing ExFISH in tissues is different from the cultured cell protocol in a few key aspects. First, the tissue ExFISH protocol requires signal amplification after performing FISH, because conventional smFISH is too dim for confocal imaging and similar 3-D imaging techniques needed for tissues. As a result, we use the hybridization chain reaction (HCR) to amplify the signal from FISH probes. Second, the gelling procedure is more similar to that described in the proExM protocol for tissues (Basic Protocol 2) than to the procedure for cultured cells. Third, higher concentrations of LabelX are used, because better RNA retention yields are observed in tissues at higher concentrations. Finally, after Label-X treatment, it is possible to use AcX to retain fluorescent proteins.
Materials
- Nuclease-free water
- Phosphate-buffered saline (PBS; Current Protocols, 2001)
- 4% (w/v) paraformaldehyde (PFA) in PBS
- 70% (v/v) ethanol in nuclease-free water
- MOPS buffer (see recipe)
- LabelX (see recipe)
- Wash buffer for HCR-FISH (WA-20; see recipe)
- HCR-FISH hybridization buffer (see recipe)
- HCR-FISH probe design: probes for HCR-FISH are designed using Stellaris Probe Designer software from LGC Biosearch Technologies. 22-bp binding sequences spaced 2 bp apart are designed using the software. We often aim for at least 20 sequences targeting each transcript of interest. To design HCR-FISH probes, HCR initiator sequences (Choi, Beck, & Pierce, 2014) are appended to each binding sequence via a 2-base spacer (AA). The initiator sequences can be appended to either the 5′ end or 3′ end of each binding sequence depending on the orientation of the initiator sequences; I1 initiators are appended to the 5′ end while I2 initiators are appended to the 3′ end. The HCR-FISH probes generated by this procedure are 60 bp in final length.
- Amplification buffer (see recipe)
- HCR Hairpins (purchased from Molecular Instruments; each hairpin comes at 3 µM concentration in a storage buffer)
- 5× SSCT (see recipe) and 0.05 × SSCT (see recipe)
- Vibratome
- 24-well culture plates
- Additional reagents and equipment for transcardial perfusion (Chen et al., 2015), vibratome cutting (Chen et al., 2015), and proExM for intact tissues (Basic Protocol 2)
Tissue fixation and slicing
Tissues can be fixed using protocols of your choice. Below is a perfusion fixation and slicing protocol for mouse brain slices that has demonstrated consistent results.
1.Transcardially perfuse the mouse brain (Chen et al., 2015) with ice-cold PBS (5 to 10 ml) followed by ice-cold 4% (w/v) paraformaldehyde in PBS (∼30 ml).
2.Post-fix the brain in 4% (w/v) paraformaldehyde in PBS overnight at 4°C.
3.Wash the brain with PBS for 30 min once, before cutting slices.
4.Cut brain slices on a vibratome (Chen et al., 2015) in PBS.
5.Slices can be stored in PBS if they are used immediately (within 1 to 2 days), or stored in 70% (v/v) ethanol in nuclease-free water for longer times at 4°C.
LabelX treatment, gelation, and digestion of tissues and tissue slices
6.If tissues or tissue slices were stored in 70% (v/v) ethanol in nuclease-free water, rehydrate by washing twice with PBS for 15 min per wash at room temperature. Otherwise, proceed with the rest of the protocol.
7.Pre-incubate tissues or tissue slices with 20 mM MOPS buffer for 30 min.
8.Prepare LabelX by diluting in 20 mM MOPS buffer at a final Label-IT amine concentration of 0.1 mg/ml (i.e., a 1:10 dilution from the LabelX stock solution).
9.Remove the pre-incubation MOPS buffer and add LabelX in MOPS buffer to the slices. For convenience, tissues or tissue slices can be processed in 24-well or 48-well plates. Use enough volume to cover the slices: for example, in a 24-well plate, four 50-µm sections can be incubated together in 250 µl of solution.
10.Incubate tissues or tissue slices with LabelX in MOPS overnight at 37°C. Afterwards, wash twice with PBS for 15 min per round.
11.Optional : To retain fluorescent proteins in tissue slices from transgenic specimens, it is possible to do an additional incubation with AcX. Incubate slices with 0.05 mg/ml AcX in PBS at room temperature for at least 6 hr. Wash twice with PBS for 15 min per wash.
12.Proceed to gelation and digestion as described in Basic Protocol 2.
13.After digestion, wash gels with PBS twice, each time for 30 min. Gels can be stored in the dark in PBS at 4°C after this step for up to 2 months.
Staining gelled slices with HCR-FISH
While staining can be performed in any chamber, we often use 24-well plates.
14.Prepare gels by incubating with wash buffer (WA-20) for 30 min at room temperature.
15.Prepare probes by diluting in HCR-FISH hybridization buffer at the desired concentration (∼1 nM per probe). Vortex to mix.
16.Remove the wash buffer from the gels. Add the hybridization buffer with probes onto the gels. Add enough volume to completely cover the gel to be stained (for 24-well plates, 300 µl per well). Incubate overnight at 37°C.
17.Wash gels twice with excess volume (e.g., 500 µl for 24-well plates) of WA-20 at 37°C for 30 min per wash. For slices thicker than 100 µm before gelation, increase wash times to 45 min per wash.
18.Wash once with excess volume PBS at 37°C for 2 hr.
19.Wash once with excess volume of PBS at room temperature for 2 hr. For slices thicker than 100 µm before gelation, increase wash time to 4 hr. If needed, after this step, gels stained with FISH probes can be stored overnight in PBS at 4°C before HCR amplification.
HCR amplification (adapted from Choi et al., 2014)
20.Pre-incubate slices with amplification buffer for 30 min at room temperature.
21.Prepare fluorescently labeled hairpins by snap cooling each hairpin (heat to 95°C for 90 sec, then cool to room temperature in a drawer for 30 min). For each 500 µl of total amplification buffer to be used (in the next step, we use 150 µl amplification buffer per well of a 48-well plate or 250 µl per well of a 24 well plate), 10 µl of each hairpin is needed.
22.Prepare hairpin solution by combining all snap-cooled hairpins in amplification buffer at room temperature, using the right volume of amplification buffer to get to the desired final volume. For example, to prepare 500 µl of total amplification buffer with two hairpins, add 10 µl of each hairpin to 480 µl of amplification buffer. Vortex to mix.
23.Remove the pre-amplification solution, add the hairpin solution to the gels, and incubate 3 to 6 hr at room temperature.
24.Stop amplification with four 30-min washes with 5× SSCT.
Expansion and imaging
25.At this point the samples can be imaged. To expand the samples, wash three times, each time for 10 min with 0.05× SSCT. The expansion factor can be tuned by altering the salt concentration; imaging can also be performed in regular PBS for ∼2× expansion. An example mouse brain slice is shown in Figure 9.Refer to Basic Protocol 6 for how to image expanded gels in the most common microscope setups.
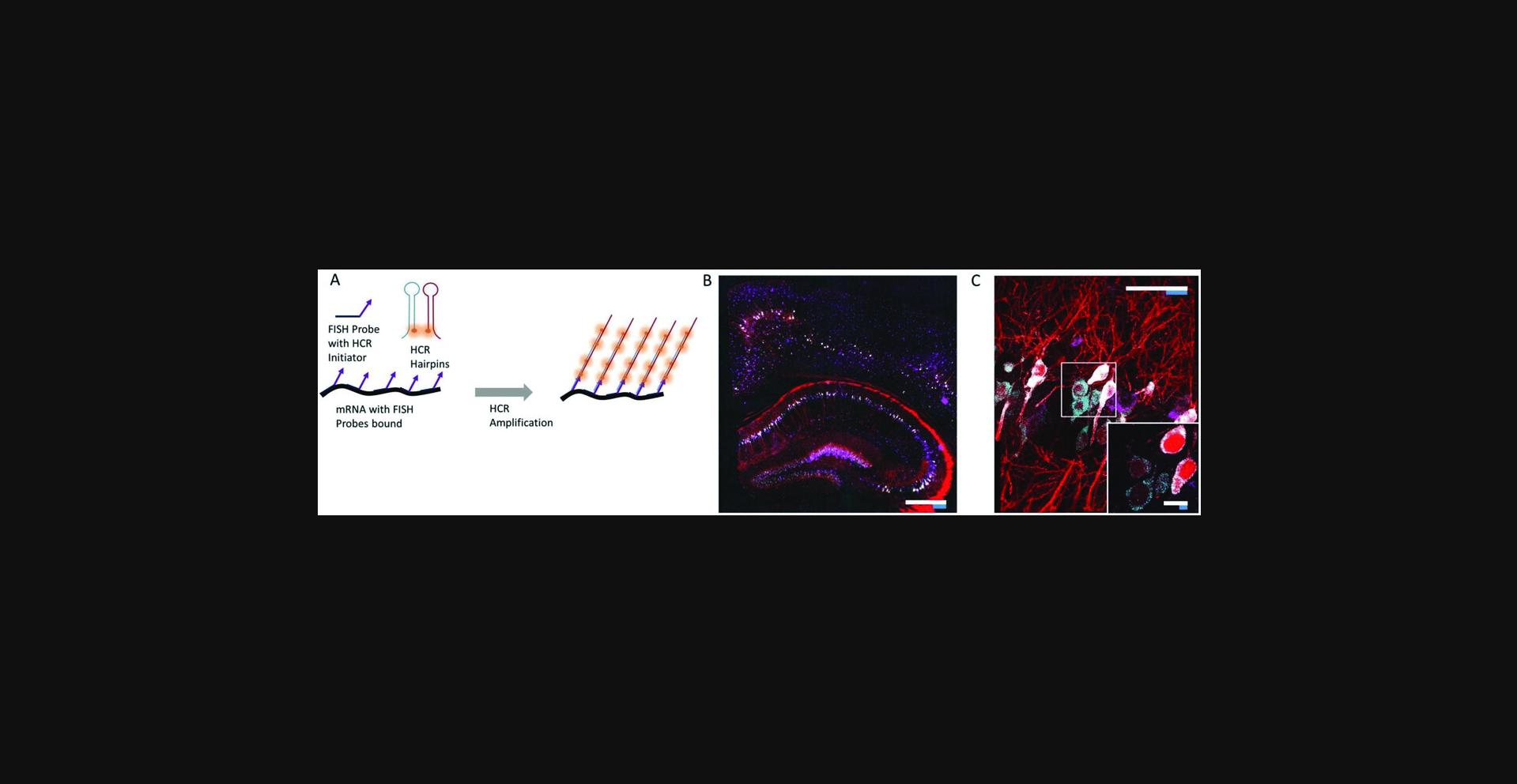
Basic Protocol 6: Imaging Expanded Samples
Materials
- Expanded sample (see protocols above)
- Ethanol
- FISH probes
- 0.1% (w/v) poly-L-lysine in water
- Nitrogen source
- Low-melting-point agarose
- Superglue
- Dissecting or wide-field microscope
- Razor blade
- Coverslips (e.g., 24 × 60 mm No. 1.5 (thickness: 0.15 to 0.19 mm))
- Glass-bottom dishes or multi-well plates (e.g., Mattek, catalog no. P35G-0.170-14-C)
- Paintbrush
- Paper wipes (e.g., Kimwipes)
- Inverted, upright, or light-sheet microscope
Sample expansion for imaging
1.Place the gel-embedded, digested sample in PBS (e.g., step 30 in Basic Protocol 1, end of step 25 in Basic Protocol 2, step 17 in Basic Protocol 4, or step 13 in Basic Protocol 5) in a petri dish.
2.Use a dissection microscope or a wide-field microscope with a low-magnification (e.g., 4× or 10×) objective to find the region of interest.
3.Under the microscope, use a razor blade to remove excess gel (e.g., away from the region of interest).
4.Fully expand the proExM samples with water. ExFISH samples should be stained with FISH probes and expanded in diluted PBS for cultured cells, or diluted SSC buffer for tissues, following the protocols described earlier (i.e., steps 22 to 28 in Basic Protocol 4, or steps 14 to 25 in Basic Protocol 5).
Sample mounting
Mounting an expanded ExM sample on a stable surface can help prevent the sample from drifting during imaging, which is important for obtaining high-quality images. The best mounting method will depend on the geometries of your microscope and the sample holder (coverslip, Petri dish, or well of a multiwell plate, for example), as well as your imaging requirements such as imaging time and the objective type and magnification.
For a quick inspection (<5 min) of the expanded samples using an inverted or upright microscope with a dry objective, there is typically no need for sample mounting. You can use a pipet to temporarily (<5 min) remove the liquid around the gel to prevent it from floating or sliding away.
For long-term (>5 min) imaging or imaging that requires minimal sample drift (e.g., obtaining a z -stack), you might want to mount the sample by physically attaching the expanded samples to the sample holder. In the following sections, we describe three common mounting methods: agarose, poly-L-lysine, and superglue. For inverted microscopes, you can use poly-L-lysine to mount the gel to a stable surface. For upright microscopes, you can use agarose, poly-L-lysine, or superglue. For light-sheet microscopes, you can use superglue or poly-L-lysine.
The advantage of the poly-L-lysine method is that it provides a transparent interface between the gel and the surface of the sample holder. Therefore, it is suitable for microscope configurations that require imaging through the sample holder, such as an inverted microscope. In comparison, agarose can introduce optical scattering and aberration, and superglue is not transparent after curing. The advantage of the agarose method is that you can retrieve your sample after imaging, as the sample mounting is reversible. The sample can be shrunk (and then separated from the agarose) by placing in high-salt buffer after imaging. The advantage of the superglue method is its strong adhesion compared with the other methods. It is suitable for long-term imaging on an upright microscope or a light-sheet microscope, even for days.
Sample mounting with poly-L-lysine
5a. Coverslips, glass-bottom dishes, and glass-bottom multi-well plates can be used as sample holders. Slides or plastic multi-well plates can also be used, but the thickness of the sample holder may limit the choice of objectives and/or cause aberrations on inverted microscopes.
6a. Clean the surface of the sample holder by rinsing with water and then ethanol. After rinsing, let the holder dry in air.
7a. Soak the glass surface with 0.1% (w/v) poly-L-lysine in water for 20 min.
8a. Remove the 0.1% (w/v) poly-L-lysine solution and rinse the coated glass surface with water three times.
9a. Air-dry the glass surface for 1 hr in a clean environment or use nitrogen gas to blow-dry the surface.
10a. Use a coverslip and forceps to pick up and transfer the expanded gel to the poly-L-lysine-modified glass surface, as follows.
1.First, remove most of the liquid around the gel. Then place a clean coverslip next to the gel and use a paintbrush to slide the gel onto the coverslip. 2.Next, lift the coverslip (with the gel on top) out of its container with a pair of forceps. 3.Before transferring the gel to the poly-L-lysine-modified surface, the gel surface that is to be attached needs to be dried for good adhesion. You can use a small piece of a Kimwipe to wick away excess liquid. Start absorbing excess liquid from the sides of the gel and then carefully use, for example, the tip of the paper wipe to absorb the liquid in between the bottom of the gel and the coverslip. 4.Use the paintbrush to slide the gel off of the coverslip onto the poly-L-lysine-modified surface. Be aware of the orientation of the gel in the context of your later microscopy work: for example, the side facing toward the sample holder will be more easily reached by the objective (e.g., within the objective working distance) on an inverted setup. In contrast, on an upright microscopy setup, the side facing away from the sample holder will be more accessible to the objective. Therefore, if the region of interest is closer to one particular side of the gel, place that side closer to the objective when mounting the sample. This practice becomes important for expanded samples, as the sample thickness and the gel thickness are scaled up by the expansion factor.
11a. After the mounting, immediately add a small amount of water (or diluted PBS for ExFISH samples) to keep the gel hydrated and avoid gel shrinkage.
Sample mounting with agarose
5b. Coverslips, glass-bottom dishes, and glass-bottom multi-well plates can be used as sample holders. Slides or plastic multi-well plates can also be used, but the thickness of the sample holder will limit the choice of objectives and/or cause aberrations on inverted microscopes.
6b. Prepare 0.5% (w/v) low-melting agarose solution in the correct imaging medium. For example, for proExM samples, prepare 0.5% (w/v) agarose in water. For ExFISH samples, prepare 0.5% (w/v) agarose in diluted PBS or other buffers of your choice. Keep the agarose solution warm in a 40°C water bath to avoid premature hardening. If hardened, use a short microwave cycle (10 to 20 sec) to melt the agarose solution. Alternatively, heating it to ∼60°C will re-melt it as well.
7b. Transfer the expanded gel to the sample holder of choice (e.g., coverslips and glass-bottom dishes). During the transfer, remove and wick away liquid from the expanded gel (see step 10a, above).
8b. Using a pipet, carefully apply the melted agarose solution onto the edges of the gel. If necessary, you can also embed the entire gel in agarose, using the melted agarose solution.
9b. Wait for the agarose to harden at room temperature or at 4°C, and then add a small amount of water (or diluted PBS for ExFISH samples) to prevent dehydration.
10b. After imaging is complete, you can place the mounted sample in high-salt buffer such as PBS. This will shrink the gel but not the agarose. Using paintbrushes or forceps, you can separate the gel from the agarose. The gel can be then stored in PBS for later use.
Sample mounting with superglue
5c. Coverslips, glass-bottom dishes and glass-bottom multi-well plates can be used as sample holders. Slides or plastic multi-well plates can also be used. However, since the superglue turns opaque upon curing, this mounting method is not compatible with inverted microscopes that require imaging through the sample holder.
6c. Clean the surface of the sample holder by rinsing with water and then ethanol. After rinsing, let the holder dry in air.
7c. Apply superglue to the surface of the sample holder. Only apply the glue to the area where the gel should be attached. Also make sure excessive superglue is removed by paper wipes, leaving only a thin layer.
8c. Transfer the expanded gel to the sample holder of choice. Before the transfer, remove and wick away liquid from the expanded gel (see step 10a).
9c. Once the gel is placed on the glue, wait for 20 to 30 sec for the superglue to cure before adding water (or diluted PBS for ExFISH samples) to hydrate the gel.
Imaging ExM samples with inverted microscopes (e.g., inverted spinning disk confocal microscopes)
A typical inverted microscope setup is shown in Figure 10. The poly-L-lysine mounting method is recommended for inverted microscopes, since the transparent interface between the gel and the poly-L-lysine modified surface will allow for imaging through the sample holder.
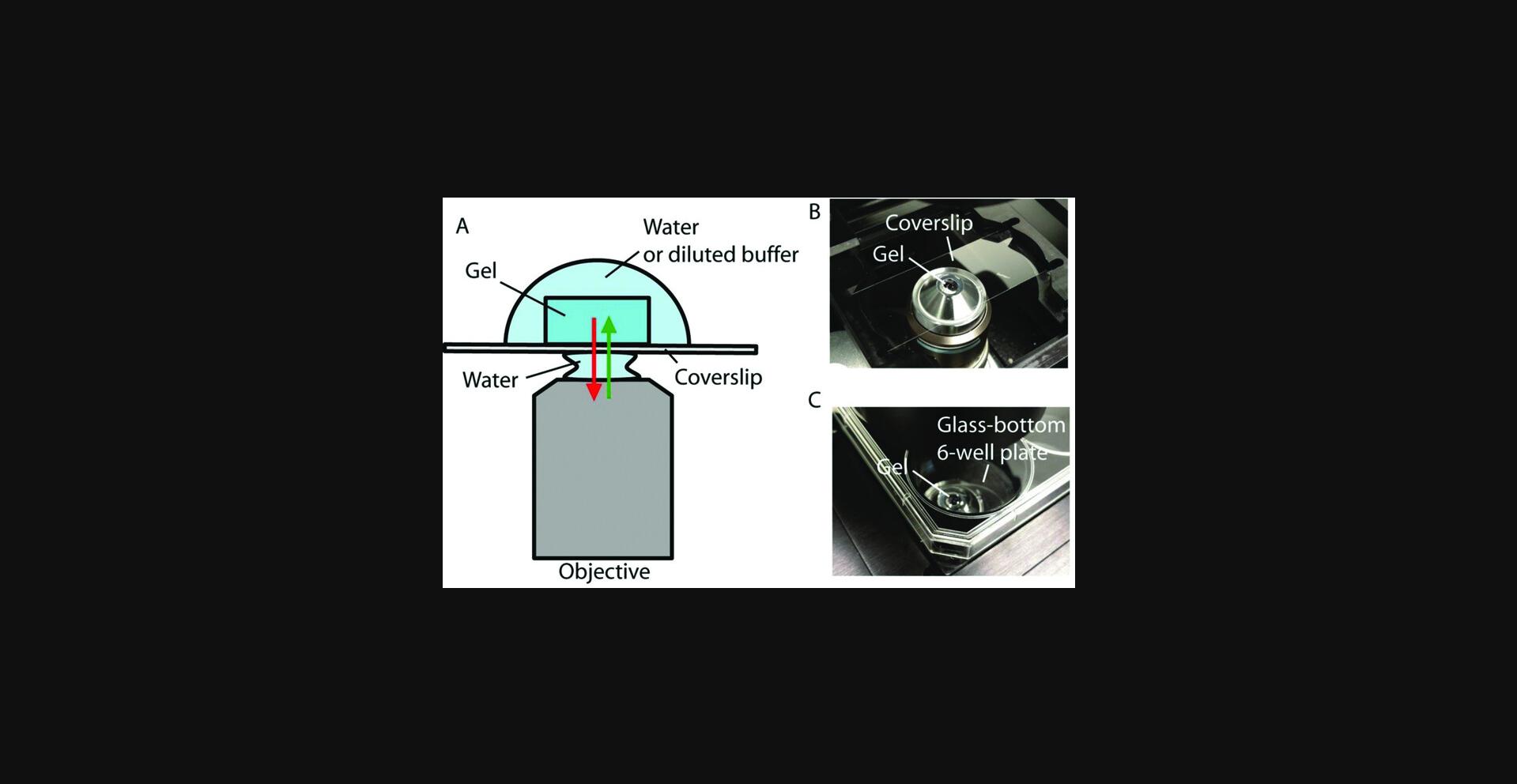
For imaging on an inverted microscope, we recommend starting with low-magnification (4× or 10×) objectives to find the region of interest first, and then switching to high-magnification objectives. Air objectives are typically low-magnification objectives (e.g., 4× or 10×) and can be used at the beginning to find the samples and the regions of interest. For high-magnification imaging (>20×), we recommend using water-immersion objectives to avoid aberrations caused by refractive index mismatch between the aqueous mounting medium and the coverslip or oil. Due to the generally short working distances, oil-immersion objectives can be considered for use mainly when the expanded gel is mounted close (within ∼10 μm) to the coverslip and when the specimen or object of interest is close to the objective (such as in cultured cells or thin tissues). The working distance of the objective is typically not an issue for thin samples such as cultured cells, if the gel is properly mounted on the sample holder (i.e., the sample side of the gel is mounted facing the objective side using the poly-L-lysine method). For some tissue samples, however, the working distance becomes an issue when imaging deep into the gel (e.g., >300 µm). In this case, long-working distance water-immersion objectives are recommended. As with every microscopy experiment, make sure the correction collar on the objective (if applicable) is adjusted to match the thickness of the coverslips.
Many of the wide-field and confocal microscopes used in biology labs and imaging facilities are inverted microscopes. We have found that spinning-disk confocal microscopes offer a good combination of resolution and speed, and are highly suitable for fast nanoscale imaging of expanded tissues. Laser-scanning confocal microscopes can be used most effectively for high signal-to-background imaging of thin and small samples such as cultured cells.
Imaging with upright microscopes
For upright microscopes, any of the three mounting methods, poly-L-lysine, agarose, or superglue, can be used. If you plan to retrieve your sample after imaging, we recommend the agarose mounting method. In the other two methods, the gel is physically attached to the sample holder, but can potentially be cut free using a razor blade if the sample needs to be retrieved (note that this process can damage the sample, if you are not careful). For long-term imaging (e.g., over a few days), we recommend using the superglue mounting method, as it offers the strongest adhesion to the sample holder. The poly-L-lysine method gives a transparent interface between the gel and the sample holder and thus can be imaged on an inverted microscope, too. For imaging on an upright microscope, we recommend starting with low-magnification (4× or 10×) objectives to find the region of interest first, and then switching to high-magnification objectives, which is similar to the procedure on an inverted microscope. For high-magnification imaging (>20×), we recommend using water-dipping or water-immersion objectives, as the gel is immersed in aqueous mounting medium.
Imaging with microscopes with the sample hanging from above (e.g., light-sheet microscopes)
Light-sheet microscopes are suitable for imaging of expanded tissue samples at high speeds, using long-working-distance objectives. To accommodate the objectives, some light-sheet microscopes hang the samples from above. For example, a typical imaging setup of a light-sheet microscope (Zeiss Z.1 light-sheet microscope) is shown in Figure 11. The Z.1 light-sheet microscope uses a rod to hang samples from above the objectives, perpendicular to the microscope's light path. The sample is then enclosed in an imaging chamber filled with aqueous mounting medium. For expanded ExM samples, a thin coverslip can be used as the backing to physically support the gel, and then the coverslip backing can be attached to the rod through a small 3-D-printed adapter, which is available for download at our Web resource http://expansionmicroscopy.org. To attach the gel to the coverslip backing, we recommend the superglue mounting method, as it most strongly attaches the gel to the coverslip. Poly-L-lysine mounting can be also used for short-term imaging, but we have observed detachment of the gel if the mounting is not properly performed (e.g., due to expired poly-L-lysine, fast stage movements, or inadequate water removal from the gel before placement). After mounting the gel to the coverslip backing, the coverslip backing can be attached to the adapter with superglue. The adapter is designed to match the dents at the end of the rod so it can be mechanically fixed to the rod.
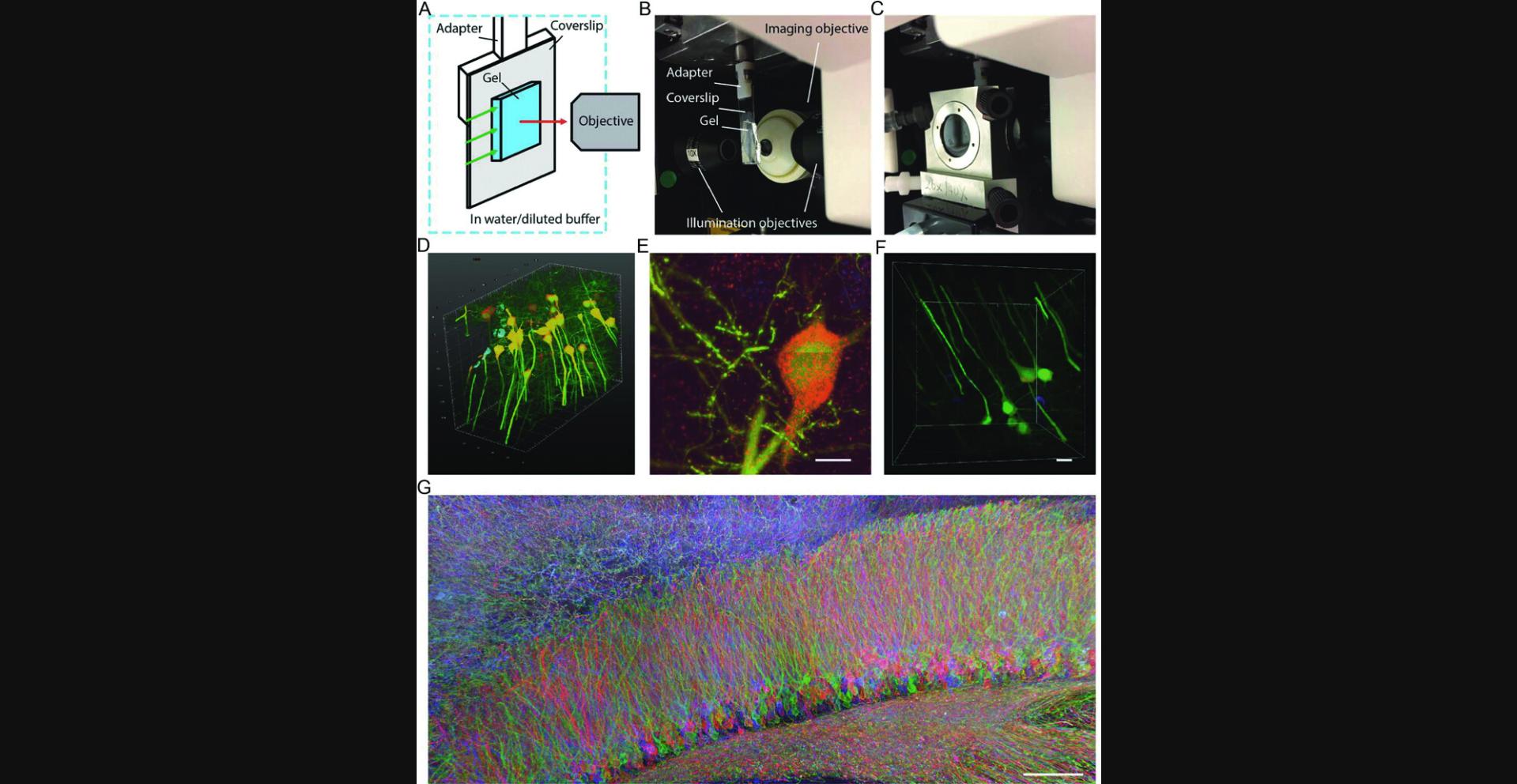
On the Zeiss Z.1 light-sheet microscope, we typically use a 1.0 NA, 20× long-working-distance water-immersion objective for high-resolution imaging. If the imaging volume is large and requires a long imaging time, we can use lower-magnification objectives (5×) to obtain a low-resolution, quick overview of the sample. The Z.1 light-sheet microscope is suitable for imaging large, multi-color samples with its two-camera setup, fast image acquisition, and capability of capturing two color channels simultaneously. In addition, the long working distance of the objective can be used to image large transparent samples. These specifications of the Z.1 light-sheet microscope are particularly advantageous for imaging ExM samples.
We first demonstrated these advantages by imaging a ∼575 × 575 × 160–µm volume (∼6 × 1010 voxels in three colors) of a HCR-ExFISH sample in about ∼3 hr, with a resolution capable of resolving single RNA puncta within the expanded tissue (Fig. 11D-F; Chen et al., 2016). Even larger samples in the millimeter range have been successfully imaged with this system. One such example is shown in Figure 11G, in which a subregion of a mouse hippocampus infected with a Brainbow 3.0 AAV virus (Fig. 5) was expanded with a proExM protocol described earlier. The imaging volume was ∼1.5 × 0.8 × 0.1 mm in pre-expansion units, and the dataset required stitching of 180 individual 3-D image stacks, resulting in a 5 Terabyte, 0.7 Teravoxel dataset with an effective lateral pixel size of about 60 nm.
Reagents and Solutions
Acryloyl-X SE (AcX)/anhydrous DMSO stock solution
- Dissolve 5 mg Acryloyl-X SE (AcX; Invitrogen, cat. no. A20770) in 500 μl anhydrous dimethylsulfoxide (DMSO).
- The AcX/anhydrous DMSO stock solution can be divided into 10- to 20-μl aliquots and stored at −20°C for at least a month. The aliquots should be stored in a sealed container with drying agents (e.g., Drierite) or in a desiccator to avoid hydration.
Amplification buffer
Amplification buffer | Amount |
---|---|
Dextran sulfate | 5 g |
Tween-20 | 0.05 ml |
20× SSC | 12.5 ml |
Nuclease-free water | Add until total volume 50 ml |
This solution contains 5× SSC, 10% (w/v) dextran sulfate, and 0.1% (v/v) Tween-20, and can be divided into 1-ml aliquots and stored at 4°C for up to 2 months.
Digestion buffer
Digestion buffer | Amount | Final concentration (/100 ml solution) |
---|---|---|
Triton X-100 | 2.50 g | 0.50 g |
EDTA, disodium (0.5 M, pH 8) | 1 ml | 0.2 ml |
Tris⋅Cl (1 M) aqueous solution, pH 8 (Current Protocols, 2001) | 25 ml | 5 ml |
NaCl | 23.38 g | 4.67 g |
Water | Add up to a total volume of 500 ml | |
Proteinase K (800 U/ml) | 1:100 dilution | 800 U (= 8 U/ml) |
Total | 500 ml |
Tris base can be used, instead of Tris (1 M) aqueous solution at pH 8. If Tris base is used, the final concentration of Tris needs to be 50 mM, and the pH of the buffer needs to be adjusted to pH 8 (e.g., with hydrochloric acid). The digestion buffer can be stored (minus the proteinase K) as aliquots at −20°C for a few months. Proteinase K can be stored at −20°C per manufacturer guidelines. Add Proteinase K immediately before the digestion step. Total amount 500 ml; amount can be scaled down if desired.
Gelling solution for expansion of intact tissues
Gelling solution | Stock solution concentration (g/100 ml solution) | Amount (µl) | Final concentration (g/100 ml solution) |
---|---|---|---|
Stock X (see recipe) | NA | 188 | NA |
4HT (see recipe) | 0.5 | 4 | 0.01 |
TEMED (see recipe) | 10 | 4 | 0.2 |
APS (see recipe) | 10 | 4 | 0.2 |
Total | 200 |
The gelling solution (total amount 200 µl; scale up or down proportionally to the appropriate amount for the sample) should be prepared at 4°C, and used immediately. Right before vortexing and usage, APS should be mixed into the solution, to prevent premature polymerization of the gel.
Gelling solution for proExM of cultured cells
Gelling solution | Stock solution concentration (g/100 ml solution) | Amount (µl) | Final concentration (g/100 ml solution) |
---|---|---|---|
Stock X (see recipe) | NA | 188 | NA |
TEMED (see recipe) | 10 | 4 | 0.2 |
APS (see recipe) | 10 | 4 | 0.2 |
Water | 4 | ||
Total | 200 |
The gelling solution (total amount 200 µl; scale up or down proportionally to the appropriate amount for the sample) should be prepared at 4°C, and used immediately. Right before vortexing and usage, APS should be mixed into the solution, to prevent premature polymerization of the gel.
Hybridization buffer for hybridization chain reaction FISH (HCR-FISH)
Hybridization buffer without formamide | Amount |
---|---|
Dextran sulfate | 10 g |
20× SSC (Current Protocols, 2001) | 10 ml |
Nuclease-free water | Add until total volume 80 ml |
First, prepare the hybridization buffer without formamide, which can be stored as 10-ml aliquots at 4°C for up to 2 months. Add formamide (20% v/v) right before performing HCR-FISH:
Hybridization buffer for HCR-FISH | Amount |
---|---|
Formamide | 2 ml |
Hybridization buffer without formamide (see above) | 8 ml |
The resultant solution contains 10% (w/v) dextran sulfate, 20% (v/v) formamide and 2× SSC.
Hybridization buffer for single molecule FISH (smFISH)
Hybridization buffer without formamide | Amount |
---|---|
Dextran sulfate | 10 g |
20× SSC | 10 ml |
Nuclease-free water | Add until total volume 90 ml |
First, prepare the hybridization buffer without formamide, which can be stored in 10 ml aliquots at 4°C for up to 2 months. Add formamide (10% v/v) right before performing FISH.
Hybridization buffer for smFISH | Amount |
---|---|
Formamide | 1 ml |
Hybridization buffer without formamide (see above) | 9 ml |
The resultant solution contains 10% (w/v) dextran sulfate, 10% (v/v) formamide, and 2× SSC.
4-Hydroxy-TEMPO (4HT) stock solution
4HT stock solution | Stock solution concentration (g/100 ml solution) |
---|---|
4HT | 0.5 |
The 4HT stock solution is prepared in water, and can be prepared in aliquots of 1 ml and stored at −20°C for at least a month.
LabelX solution
Resuspend Label-IT amine (Mirus Bio, cat. no. MIR3900) at 1 mg/ml in the vendor-provided resuspension buffer. Vortex to mix. React the Label-IT/resuspension buffer solution with AcX/DMSO stock solution (see recipe) at equal mass ratio. For example, add 10 µl of AcX/DMSO stock (at 10 mg/ml) solution to 100 µl of Label-IT solution (both are resuspended in DMSO, so the reaction is carried out in DMSO). React overnight at room temperature on a benchtop shaker. The AcX is now conjugated to Label-IT, resulting in what we call “LabelX.” Store the LabelX solution at −20°C, in a desiccator, for up to 2 months.
Monomer solution (colloquially known as Stock X)
Monomer solution (“Stock X”) | Stock solution concentration (g/100 ml solution) | Amount (ml) | Final concentration (g/100 ml solution) |
---|---|---|---|
Sodium acrylate | 38 (33 wt% due to higher density) | 2.25 | 8.6 |
Acrylamide | 50 | 0.5 | 2.5 |
N,N′-Methylenebisacrylamide | 2 | 0.75 | 0.15 |
Sodium chloride | 29.2 (5M) | 4 | 11.7 |
PBS, 10× stock | 10× | 1 | 1× |
Water | 0.9 | ||
Total | 9.4 |
The Stock X solution (total amount 9.4 ml) can be divided into aliquots of 1 ml each and stored at −20°C for at least 1 month. Low-purity sodium acrylate may appear slightly yellow when dissolved in water. In this case, discard the solution and switch to a new batch or a new bottle of sodium acrylate.
MOPS stock solution
MOPS stock solution (200 mM) | Stock solution concentration (g/100 ml solution) |
---|---|
MOPS | 4.18 |
First, prepare the MOPS stock solution (200 mM, 10 times the final concentration) in nuclease-free water and adjust the pH to 7.7. The MOPS stock solution can be divided into 1-ml aliquots and stored at −20°C for up to 12 months. When preparing MOPS buffer, dilute the MOPS stock solution 1:10 in nuclease-free water to bring the final concentration to 20 mM MOPS.
Sinking solution
Sinking solution | Amount |
---|---|
10× PBS (Current Protocols, 2001) | 50 ml |
Sucrose | 150 g |
Glycine | 3.75 g |
Water | Add until total volume = 500 ml |
Sinking solution [30% (w/v) sucrose and 100 mM glycine in PBS] should be stored at 4°C and can be used for at least 1 month. The sinking solution is used for cryoslicing on a cryostat.
SSCT, 5×
5× SSCT | Amount |
---|---|
Tween-20 | 0.1 ml |
20X SSC | 25 ml |
Nuclease-free water | Add until total volume 100 ml |
This solution contains 5× SSC and 0.1% (v/v) Tween-20, and can be stored at room temperature for up to 1 year.
SSCT, 0.05×
0.05× SSCT | Amount |
---|---|
Tween-20 | 0.1 ml |
20× SSC | 0.25 ml |
Nuclease-free water | Add until total volume 100 ml |
This solution contains 0.05× SSC and 0.1% (v/v) Tween-20, and can be stored at room temperature for up to 1 year.
Tetramethylethylenediamine (TEMED) and ammonium persulfate (APS) stock solutions
TEMED and APS stock solutions | Stock solution concentration (g/100 ml solution) |
---|---|
TEMED | 10 |
APS | 10 |
The TEMED and APS stock solutions are prepared separately in water (i.e., these are two different stock solutions), and can be prepared into aliquots of 1 ml each and stored at −20°C for 2 weeks or more.
VA-044 stock solution
VA-044 stock solution | Stock solution concentration (g/100 ml solution) |
---|---|
VA-044 (Wako Chemicals, cat. no. 27776-21-2) | 25 g |
The VA-044 stock solution is used to aid gelation. The solution should be prepared 1 ml at a time and used immediately. Keep this stock solution on ice while preparing the gelling solution.
Wash buffer for HCR-FISH (WA-20)
WA-20 | Amount |
---|---|
Formamide | 20 ml |
20× SSC | 10 ml |
Nuclease-free water | Add until total volume 100 ml |
This solution contains 20% (v/v) formamide and 2× SSC, and can be prepared as 10-ml aliquots and stored at room temperature for up to 1 week.
Wash buffer for smFISH (WA-10)
WA-10 | Amount |
---|---|
Formamide | 10 ml |
20× SSC (Current Protocols, 2001) | 10 ml |
Nuclease free water | Add until total volume 100 ml |
This solution contains 10% (v/v) formamide and 2× SSC, and can be prepared as 10-ml aliquots and stored at room temperature for up to 1 week.
Commentary
Background Information
We recently discovered that preserved biological samples could be embedded in a polymer hydrogel and physically expanded in an isotropic fashion, thus enabling nanoscale-resolution imaging on conventional diffraction-limited microscopes, a process that we call expansion microscopy (ExM; Chen et al., 2015). Although preserved biological specimens have been embedded in polymer hydrogels (such as polyacrylamide) since the early 1980s for the purpose of improving microscopy of biological specimens (Hausen & Dreyer, 1981; Germroth, Gourdie, & Thompson, 1995), expansion microscopy utilizes a charged polyelectrolyte gel (i.e., sodium polyacrylate) that is capable of 100× volumetric swelling upon exposure to water (Tanaka et al., 1980), and also explicitly links biomolecules and/or labels to the swellable gel while decoupling them mechanically from each other, to ensure even separation. In addition, the hydrogel is synthesized in a densely cross-linked topology that allows it to expand evenly, preserving the relative organization of the biomolecules/labels.
Our original ExM protocols (Chen et al., 2015, 2016; Tillberg et al., 2016) allowed for an isotropic expansion factor of ∼4.5× in each direction (∼100× volumetric expansion), enabling a 300-nm diffraction-limit objective lens to have an effective resolution of ∼300/4.5 ∼ 60 to 70 nm. In these forms of ExM, key biomolecules and labels in a cell or tissue are first covalently equipped with anchoring molecules that enable them to be tethered to a polyacrylate network that is synthesized evenly [and densely, with a spacing of perhaps a few nanometers (Cohen, Ramon, Kopelman, & Mizrahi, 1992)] throughout the specimen. The sample's mechanical structure is then dissolved and disrupted (e.g., by proteolysis or protein denaturation) followed by uniform expansion by dialysis in water. The expansion results in physical separation of the biomolecules or labels, with their relative positions preserved, so that biomolecules previously spaced within the diffraction limit are moved far enough apart so that they can be resolved with a diffraction-limited microscope. In this unit, we describe step-by-step protocols for two versions of ExM, protein retention ExM (proExM; Tillberg et al., 2016) and expansion fluorescence in situ hybridization (ExFISH; Chen et al., 2016), in which proteins and RNAs, respectively, are anchored to the hydrogel. One can also combine the two protocols to examine both proteins and RNA in the same sample [e.g., see Fig. 3 of ref. (Chen et al., 2016)]. Recently, we showed that a sample could be expanded twice, by essentially repeating the ExM procedure, resulting in a ∼4.5 × 4.5 ∼ 20× linear expansion (∼8000× volumetric expansion), and a corresponding resolution of ∼25 nm (Chang et al., 2017). The protocol for this procedure, which we call iterative expansion microscopy (iExM), was not included here since it is evolving, but the current version will continuously be posted at our Web resource http://expansionmicroscopy.org. The protocols in this unit are based on the published protocols associated with the aforementioned protocols (and currently posted at http://expansionmicroscopy.org).
Critical Parameters
For the basic proExM protocols (Basic Protocols 1 and 2), the timing of each step needs to be followed strictly to prevent premature gelation, which could result in distortion, tearing, or lack of expansion. For example, steps 20 to 24 in Basic Protocol 1 and steps 14 to 15 in Basic Protocol 2 should be carried out as quickly as possible, ideally within 5 min. Similarly, steps 9 to 13 in Basic Protocol 4 should be carried out with minimal delay. Specific troubleshooting details are listed in the next section.
Troubleshooting
Problems, potential causes and resolutions are listed in Table 1.
Step | Observed problem | Potential causes and solutions |
---|---|---|
|
Sample prematurely forms a gel before the lid can be placed |
|
|
Air bubble(s) are trapped under the lid |
|
|
Expansion factor is smaller than anticipated |
|
|
Cannot locate expanded samples in water (or other diluted buffers used for expansion) |
|
|
Expanded sample gel breaks during handling | Handling of sample gels becomes increasingly difficult once expanded as the gel becomes more fragile. We recommend using, for example, plastic spatulas and brushes for handling, as described in the sample mounting subsections of Basic Protocol 6 (sample mounting with poly-L-lysine, sample mounting with agarose, sample mounting with superglue). |
|
Expanded sample moves too much during imaging |
|
|
||
Use low melting agarose to fix the gel for upright microscopes (refer to Sample mounting with agarose). | ||
Use superglue to attach the gel for upright or light-sheet microscopes (refer to Sample mounting with superglue) | ||
|
Imaging takes too long | ProExM introduces a linear 4.5× expansion, which translates into a ∼90× volumetric increase. Therefore, if volumetric imaging is performed, it requires about 90× the time compared to the pre-expansion sample volume. We therefore recommend using faster imaging methods, such as light-sheet, or spinning disc confocal microscopy (such as described in Imaging ExM samples with inverted microscopes and Imaging with microscopes with the sample hanging from above). |
|
Low or no fluorescence after expansion | Switch to compatible fluorescent dyes for immunostaining and fluorescent proteins that are proExM-optimized (refer to Support Protocol 2). Furthermore, due the large volumetric expansion, expanded samples are expected to be much dimmer compared to their pre-expansion states. Make sure to use longer exposure time and/or higher laser intensity for imaging. |
After imaging ExM samples | How to perform image processing and data analyses | Traditional image processing programs, such as MATLAB or ImageJ, are ideal for ExM data as well. However, imaging expanded samples sometimes generate large datasets not suitable for these programs. There are a few commercial software packages available for large data visualization and image analyses, such as Arivis Vision4D and Bitplane Imaris. Alternatively, the data can be either cropped or divided up and processed traditionally in smaller data chunks. |
After imaging ExM samples | Samples lose their fluorescence after few weeks | Fluorescent proteins denature in water over time and other fluorescent probes can degrade or rehybridize in non-buffered environments. Therefore, we do not recommend storing ExM samples in their expanded states. For long-term storage, we recommend storing the samples in a buffered environment, such as PBS. In addition, we keep the samples at 4°C in the dark to prevent bleaching of fluorescence. |
|
ExFISH: No visible spots after smFISH or FISH-HCR | To avoid RNA degradation, make sure to use nuclease-free reagents for the sample and reagent preparation. If working with slices, do not store them in PBS at 4°C for more than 3-4 days−either store them in 70% (v/v) ethanol in nuclease-free water at 4°C, or store them frozen with a cryoprotectant. |
|
ExFISH: smFISH/FISH-HCR staining too dim after expansion |
|
|
ExFISH: probe wash out is too slow; high background after staining |
|
Understanding Results
ProExM samples from Basic Protocols 1 to 3 will appear increasingly transparent after mechanical homogenization and expansion. After full expansion to ∼4.5× of the initial size, the proExM samples will appear virtually transparent, matching the refractive index of water (Fig. 3). The expanded samples can be fragile to handle and difficult to locate in water (see Basic Protocol 6 for gel handling and immobilization tips).
Similarly, the digested samples from Basic Protocol 4 and Basic Protocol 5 will appear transparent. During the RNA-FISH staining steps for both protocols, the gel will shrink and expand because of the different salt contents of the buffers involved. Since the expansion step at the end of the staining protocol is carried out in a low-salt buffer instead of water, the final observed expansion is approximately ∼3.3× for both ExFISH protocols.
Fluorescence microscope setups, such as wide-field microscopes, spinning disk and laser scanning confocal microscopes, and light-sheet microscopes, can be used to image the expanded proExM and ExFISH samples (see Basic Protocol 6, as well as Figures 5, 6, 8, 9, and 11 for representative images of the various protocols described here). Expanded samples typically have lower fluorescence intensities compared with their pre-expansion states due to the physical expansion, and therefore require longer exposure time and/or higher laser intensity (refer to the Troubleshooting section if no or low fluorescence) to acquire images of similar brightness.
Time Considerations
Each procedure's duration depends on the properties of the sample, such as thickness and biological composition, as well as the protocol used, but typically falls in the range of 1 day to a few days, with only a few short periods (1 to 2 hr each) of manual work required at each defined timepoint in the protocol (the remainder of the time is either allocated for incubation or washing). For example, with Basic Protocol 2, a tissue slice of 200 μm thickness takes ∼2 days to go from the original fixed and sliced tissue to the final mechanically homogenized state embedded in the hydrogel, ready for expansion. With Basic Protocol 5, the ExFISH protocol similarly takes ∼2 days. In both proExM and ExFISH, digested samples can be stored in PBS at 4°C in the dark for weeks before further processing, expansion, and imaging. Staining the gel-embedded ExFISH samples with RNA FISH probes takes 1 day for cultured cells and 2 days for tissues, after which the stained samples can be readily expanded and imaged. Fluorescence imaging of the expanded samples can take anywhere from minutes to hours, depending on the imaging volume and image acquisition speed.
Acknowledgements
We would like to acknowledge Yosuke Bando and Kazuhiro Hiwada for the image rendering in Figure 11G, Grace H. Huynh and Linyi Gao for help with sample preparation, Eric Betzig, Jennifer Lippincott-Schwartz and Kathy Schaefer at HHMI Janelia Research Campus for insightful discussions, and Wendy C. Salmon at the W. M. Keck Microscopy Facility at the Whitehead Institute for help with imaging. E.S.B. acknowledges, for funding, the Ludwig Foundation and MIT Aging Brain Initiative, NIH 1R01NS102727, John Doerr, NIH 1R01EB024261, the HHMI-Simons Faculty Scholars Program, NIH 1R41MH112318, the Open Philanthropy Project, NIH 1R01MH110932, IARPA D16PC00008, and the U.S. Army Research Laboratory and U.S. Army Research Office under contract/grant number W911NF1510548, NIH 1RM1HG008525, the Chan Zuckerberg Initiative, and NIH Director's Pioneer Award 1DP1NS087724. A.T.W. acknowledges the Hertz Foundation Fellowship.
Conflicts of Interest
E.S.B. is co-founder of a company seeking to commercialize clinical applications of ExM. R.G., A.T.W., P.W.T., F.C., and E.S.B. are inventors on one or more granted or pending patents related to ExM.
Literature Cited
- Chang, J.-B., Chen, F., Yoon, Y.-G., Jung, E. E., Babcock, H., Kang, J. S., … Boyden, E. S. (2017). Iterative expansion microscopy. Nature Methods , 14(6), 593–599. doi: 10.1038/nmeth.4261.
- Chen, F., Tillberg, P. W., & Boyden, E. S. (2015). Expansion microscopy. Science , 347, 543–548. doi: 10.1126/science.1260088.
- Chen, F., Wassie, A. T., Cote, A. J., Sinha, A., Alon, S., Asano, S., … Boyden, E. S. (2016). Nanoscale imaging of RNA with expansion microscopy. Nature Methods , 13, 679–684. doi: 10.1038/nmeth.3899.
- Choi, H. M. T., Beck, V. A., & Pierce, N. A. (2014). Next-generation in situ hybridization chain reaction: Higher gain, lower cost, greater durability. ACS Nano , 8, 4284–4294. doi: 10.1021/nn405717p.
- Cohen, Y., Ramon, O., Kopelman, I. J., & Mizrahi, S. (1992). Characterization of inhomogeneous polyacrylamide hydrogels. Journal of Polymer Science Part B: Polymer Physics , 30, 1055–1067. doi: 10.1002/polb.1992.090300913.
- Current Protocols. (2001). Laboratory stock solutions and equipment. Current Protocols in Cell Biology , 00, A.2A.1–A.2A.10. doi: 10.1002/0471143030.cba02as00.
- Gao, R., Asano, S. M., & Boyden, E. S. (2017). Q&A: Expansion microscopy. BMC Biology , 15, 50. doi: 10.1186/s12915-017-0393-3.
- Germroth, P. G., Gourdie, R. G., & Thompson, R. P. (1995). Confocal microscopy of thick sections from acrylamide gel embedded embryos. Microscopy Research and Technique , 30, 513–520. doi: 10.1002/jemt.1070300608.
- Hausen, P., & Dreyer, C. (1981). The use of polyacrylamide as an embedding medium for immunohistochemical studies of embryonic tissues. Stain Technology , 56, 287–293. doi: 10.3109/10520298109067329.
- Raj, A., & Tyagi, S. (2010). Detection of individual endogenous RNA transcripts in situ using multiple singly labeled probes. doi: 10.1016/S0076-6879(10)72004-8.
- Tanaka, T., Fillmore, D., Sun, S. T., Nishio, I., Swislow, G., & Shah, A. (1980). Phase Transitions in Ionic Gels. Physical Review Letters , 45, 1636–1639. doi: 10.1103/PhysRevLett.45.1636.
- Tillberg, P. W., Chen, F., Piatkevich, K. D., Zhao, Y., Yu, C.-C. J., English, B. P., … Boyden, E. S. (2016). Protein-retention expansion microscopy of cells and tissues labeled using standard fluorescent proteins and antibodies. Nature Biotechnology , 34, 987–992. doi: 10.1038/nbt.3625.
Internet Resources
proExM for tissues: Gelation demonstration.
Expansion microscopy resources.
Citing Literature
Number of times cited according to CrossRef: 114
- Sandra P Cárdenas-García, Sundas Ijaz, Alberto E Pereda, The components of an electrical synapse as revealed by expansion microscopy of a single synaptic contact, eLife, 10.7554/eLife.91931, 13 , (2024).
- Nanako Dei, Kazuhiko Ishihara, Akikazu Matsumoto, Chie Kojima, Preparation and Characterization of Acrylic and Methacrylic Phospholipid-Mimetic Polymer Hydrogels and Their Applications in Optical Tissue Clearing, Polymers, 10.3390/polym16020241, 16 , 2, (241), (2024).
- Nadja Hümpfer, Ria Thielhorn, Helge Ewers, Expanding boundaries – a cell biologist's guide to expansion microscopy, Journal of Cell Science, 10.1242/jcs.260765, 137 , 7, (2024).
- Jinghan Liu, Yuping Yolanda Tan, Wen Zheng, Yao Wang, Lining Arnold Ju, Qian Peter Su, Nanoscale insights into hematology: super-resolved imaging on blood cell structure, function, and pathology, Journal of Nanobiotechnology, 10.1186/s12951-024-02605-2, 22 , 1, (2024).
- Kseniia Bondarenko, Floriane Limoge, Kayvon Pedram, Mathieu Gissot, Joanna C. Young, Enzymatically enhanced ultrastructure expansion microscopy unlocks expansion of in vitro Toxoplasma gondii cysts , mSphere, 10.1128/msphere.00322-24, 9 , 9, (2024).
- Egemen Agi, Eric T. Reifenstein, Charlotte Wit, Teresa Schneider, Monika Kauer, Melinda Kehribar, Abhishek Kulkarni, Max von Kleist, P. Robin Hiesinger, Axonal self-sorting without target guidance in Drosophila visual map formation , Science, 10.1126/science.adk3043, 383 , 6687, (1084-1092), (2024).
- Jonathan Loi, Dhaval Ghone, Xiaofei Qu, Aussie Suzuki, 3D-Aligner: advanced computational tool for correcting image distortion in expansion microscopy, Communications Biology, 10.1038/s42003-024-07035-2, 7 , 1, (2024).
- Shiwei Wang, Tay Won Shin, Harley B. Yoder, Ryan B. McMillan, Hanquan Su, Yixi Liu, Chi Zhang, Kylie S. Leung, Peng Yin, Laura L. Kiessling, Edward S. Boyden, Single-shot 20-fold expansion microscopy, Nature Methods, 10.1038/s41592-024-02454-9, (2024).
- Sumin Kim, Sébastien Phan, Hung Tri Tran, Thomas R. Shaw, Sarah H. Shahmoradian, Mark H. Ellisman, Sarah L. Veatch, Sami J. Barmada, Samuel S. Pappas, William T. Dauer, TorsinA is essential for neuronal nuclear pore complex localization and maturation, Nature Cell Biology, 10.1038/s41556-024-01480-1, 26 , 9, (1482-1495), (2024).
- Yat Ho Chan, Koralege C. Pathmasiri, Dominick Pierre-Jacques, Maddison C. Hibbard, Nannan Tao, Joshua L. Fischer, Ethan Yang, Stephanie M. Cologna, Ruixuan Gao, Gel-assisted mass spectrometry imaging enables sub-micrometer spatial lipidomics, Nature Communications, 10.1038/s41467-024-49384-w, 15 , 1, (2024).
- Ekaterina Borisova, Andrew G. Newman, Marta Couce Iglesias, Rike Dannenberg, Theres Schaub, Bo Qin, Alexandra Rusanova, Marisa Brockmann, Janina Koch, Marieatou Daniels, Paul Turko, Olaf Jahn, David R. Kaplan, Marta Rosário, Takao Iwawaki, Christian M. T. Spahn, Christian Rosenmund, David Meierhofer, Matthew L. Kraushar, Victor Tarabykin, Mateusz C. Ambrozkiewicz, Protein translation rate determines neocortical neuron fate, Nature Communications, 10.1038/s41467-024-49198-w, 15 , 1, (2024).
- Emilie Murigneux, Laurent Softic, Corentin Aubé, Carmen Grandi, Delphine Judith, Johanna Bruce, Morgane Le Gall, François Guillonneau, Alain Schmitt, Vincent Parissi, Clarisse Berlioz-Torrent, Laurent Meertens, Maike M. K. Hansen, Sarah Gallois-Montbrun, Proteomic analysis of SARS-CoV-2 particles unveils a key role of G3BP proteins in viral assembly, Nature Communications, 10.1038/s41467-024-44958-0, 15 , 1, (2024).
- Jan Philipp Delling, Helen Friedericke Bauer, Susanne Gerlach-Arbeiter, Michael Schön, Christian Jacob, Jan Wagner, Maria Teresa Pedro, Bernd Knöll, Tobias M. Boeckers, Combined expansion and STED microscopy reveals altered fingerprints of postsynaptic nanostructure across brain regions in ASD-related SHANK3-deficiency, Molecular Psychiatry, 10.1038/s41380-024-02559-9, 29 , 10, (2997-3009), (2024).
- Piero Sanfilippo, Alexander J. Kim, Anuradha Bhukel, Juyoun Yoo, Pegah S. Mirshahidi, Vijaya Pandey, Harry Bevir, Ashley Yuen, Parmis S. Mirshahidi, Peiyi Guo, Hong-Sheng Li, James A. Wohlschlegel, Yoshinori Aso, S. Lawrence Zipursky, Mapping of multiple neurotransmitter receptor subtypes and distinct protein complexes to the connectome, Neuron, 10.1016/j.neuron.2023.12.014, 112 , 6, (942-958.e13), (2024).
- Akio Masuda, Takaaki Okamoto, Toshihiko Kawachi, Jun-ichi Takeda, Tomonari Hamaguchi, Kinji Ohno, Blending and separating dynamics of RNA-binding proteins develop architectural splicing networks spreading throughout the nucleus, Molecular Cell, 10.1016/j.molcel.2024.07.001, 84 , 15, (2949-2965.e10), (2024).
- Dante Castagnini, Karina Palma, Jorge Jara-Wilde, Nicolás Navarro, María José González, Jorge Toledo, Nicole Canales-Huerta, Paola Scavone, Steffen Härtel, Proteus mirabilis biofilm expansion microscopy yields over 4-fold magnification for super-resolution of biofilm structure and subcellular DNA organization, Journal of Microbiological Methods, 10.1016/j.mimet.2024.106927, 220 , (106927), (2024).
- Natalia A. Rumyantseva, Daria M. Golofeeva, Alexey D. Vedyaykin, SulA does not sequester FtsZ in Escherichia coli cells during the SOS response, Biochemical and Biophysical Research Communications, 10.1016/j.bbrc.2023.149313, 691 , (149313), (2024).
- Andrey Andreev, Evgenia V. Azarova, Jeremy Delahanty, Fluorescence microscopy: backbone of modern biomedical research, Biophotonics and Biosensing, 10.1016/B978-0-44-318840-4.00011-5, (51-92), (2024).
- Luca Pesce, Pietro Ricci, Giancarlo Sportelli, Nicola Belcari, Giuseppe Sancataldo, Expansion and Light‐Sheet Microscopy for Nanoscale 3D Imaging, Small Methods, 10.1002/smtd.202301715, 8 , 10, (2024).
- Brittany M. White‐Mathieu, Jeremy M. Baskin, Super‐Resolution Imaging of Clickable Lipids With Lipid Expansion Microscopy (LExM), Current Protocols, 10.1002/cpz1.1051, 4 , 5, (2024).
- Lucy S. Britto, Deepali Balasubramani, Sona Desai, Phunterion Phillips, Neev Trehan, Ethel Cesarman, Jean L. Koff, Ankur Singh, T Cells Spatially Regulate B Cell Receptor Signaling in Lymphomas through H3K9me3 Modifications, Advanced Healthcare Materials, 10.1002/adhm.202401192, (2024).
- Linda Patt, Dario Tascio, Catia Domingos, Aline Timmermann, Ronald Jabs, Christian Henneberger, Christian Steinhäuser, Gerald Seifert, Impact of Developmental Changes of GABAA Receptors on Interneuron-NG2 Glia Transmission in the Hippocampus, International Journal of Molecular Sciences, 10.3390/ijms241713490, 24 , 17, (13490), (2023).
- Björn Trebels, Stefan Dippel, Janet Anders, Clara Ernst, Brigitte Goetz, Tim Keyser, Karl Heinz Rexer, Ernst A. Wimmer, Joachim Schachtner, Anatomic and neurochemical analysis of the palpal olfactory system in the red flour beetle Tribolium castaneum, HERBST, Frontiers in Cellular Neuroscience, 10.3389/fncel.2023.1097462, 17 , (2023).
- N. A. Rumyantseva, D. M. Golofeeva, I. E. Vishnyakov, A. D. Vedyaykin, Visualization of Escherichia coli Single Cells in the State of SOS Response Using Expansion Microscopy, Цитология, 10.31857/S0041377123030070, 65 , 3, (303-310), (2023).
- Annika Haak, Heiko M. Lesslich, Irmgard D. Dietzel, Visualization of the membrane surface and cytoskeleton of oligodendrocyte progenitor cell growth cones using a combination of scanning ion conductance and four times expansion microscopy, Biological Chemistry, 10.1515/hsz-2023-0217, 405 , 1, (31-41), (2023).
- Yi Cui, Gaojie Yang, Daniel R. Goodwin, Ciara H. O’Flanagan, Anubhav Sinha, Chi Zhang, Kristina E. Kitko, Tay Won Shin, Demian Park, Samuel Aparicio, Edward S. Boyden, Expansion microscopy using a single anchor molecule for high-yield multiplexed imaging of proteins and RNAs, PLOS ONE, 10.1371/journal.pone.0291506, 18 , 9, (e0291506), (2023).
- Zeliha Kilic, Max Schweiger, Camille Moyer, Steve Pressé, Monte Carlo samplers for efficient network inference, PLOS Computational Biology, 10.1371/journal.pcbi.1011256, 19 , 7, (e1011256), (2023).
- N. A. Rumyantseva, D. M. Golofeeva, I. E. Vishnyakov, A. D. Vedyaykin, Visualization of Single Escherichia coli Cells in the State of SOS Response using Expansion Microscopy, Cell and Tissue Biology, 10.1134/S1990519X2306010X, 17 , 6, (692-698), (2023).
- Chao Sun, Kristina Desch, Belquis Nassim-Assir, Stefano L. Giandomenico, Paulina Nemcova, Julian D. Langer, Erin M. Schuman, An abundance of free regulatory (19 S ) proteasome particles regulates neuronal synapses , Science, 10.1126/science.adf2018, 380 , 6647, (2023).
- Mark E. Pownall, Liyun Miao, Charles E. Vejnar, Ons M’Saad, Alice Sherrard, Megan A. Frederick, Maria D. J. Benitez, Curtis W. Boswell, Kenneth S. Zaret, Joerg Bewersdorf, Antonio J. Giraldez, Chromatin expansion microscopy reveals nanoscale organization of transcription and chromatin, Science, 10.1126/science.ade5308, 381 , 6653, (92-100), (2023).
- Ueh-Ting Tim Wang, Xuejiao Tian, Yae-Huei Liou, Sue-Ping Lee, Hsiao-Tang Hu, Chieh-Han Lu, Po-Ting Lin, Ya-Jen Cheng, Peilin Chen, Bi-Chang Chen, Protein and lipid expansion microscopy with trypsin and tyramide signal amplification for 3D imaging, Scientific Reports, 10.1038/s41598-023-48959-9, 13 , 1, (2023).
- Kemal Arda Günay, Tze-Ling Chang, Nathaniel P. Skillin, Varsha V. Rao, Laura J. Macdougall, Alicia A. Cutler, Jason S. Silver, Tobin E. Brown, Chi Zhang, Chih-Chieh Yu, Bradley B. Olwin, Edward S. Boyden, Kristi S. Anseth, Photo-expansion microscopy enables super-resolution imaging of cells embedded in 3D hydrogels, Nature Materials, 10.1038/s41563-023-01558-5, 22 , 6, (777-785), (2023).
- Pascale Baden, Maria Jose Perez, Hariam Raji, Federico Bertoli, Stefanie Kalb, María Illescas, Fokion Spanos, Claudio Giuliano, Alessandra Maria Calogero, Marvin Oldrati, Hannah Hebestreit, Graziella Cappelletti, Kathrin Brockmann, Thomas Gasser, Anthony H. V. Schapira, Cristina Ugalde, Michela Deleidi, Glucocerebrosidase is imported into mitochondria and preserves complex I integrity and energy metabolism, Nature Communications, 10.1038/s41467-023-37454-4, 14 , 1, (2023).
- Hye Yun Kim, Jieun Lee, Hyun-Jin Kim, Byeong Eun Lee, Jaewook Jeong, Eun Jeong Cho, Hyun-Jun Jang, Kyeong Jin Shin, Min Ji Kim, Young Chan Chae, Seung Eun Lee, Kyungjae Myung, Ja-Hyun Baik, Pann-Ghill Suh, Jae-Ick Kim, PLCγ1 in dopamine neurons critically regulates striatal dopamine release via VMAT2 and synapsin III, Experimental & Molecular Medicine, 10.1038/s12276-023-01104-y, 55 , 11, (2357-2375), (2023).
- Vinit Sheth, Xuxin Chen, Evan M. Mettenbrink, Wen Yang, Meredith A. Jones, Ons M’Saad, Abigail G. Thomas, Rylee S. Newport, Emmy Francek, Lin Wang, Alex N. Frickenstein, Nathan D. Donahue, Alyssa Holden, Nathan F. Mjema, Dixy E. Green, Paul L. DeAngelis, Joerg Bewersdorf, Stefan Wilhelm, Quantifying Intracellular Nanoparticle Distributions with Three-Dimensional Super-Resolution Microscopy, ACS Nano, 10.1021/acsnano.2c12808, 17 , 9, (8376-8392), (2023).
- Deena Al Mahbuba, Sayaka Masuko, Shiwei Wang, Deepsing Syangtan, Jeong Seuk Kang, Yuefan Song, Tay Won Shin, Ke Xia, Fuming Zhang, Robert J. Linhardt, Edward S. Boyden, Laura L. Kiessling, Dynamic Changes in Heparan Sulfate Nanostructure in Human Pluripotent Stem Cell Differentiation, ACS Nano, 10.1021/acsnano.2c10072, 17 , 8, (7207-7218), (2023).
- Ha H. Park, Alexander A. Choi, Ke Xu, Size-Dependent Suppression of Molecular Diffusivity in Expandable Hydrogels: A Single-Molecule Study, The Journal of Physical Chemistry B, 10.1021/acs.jpcb.3c00761, 127 , 14, (3333-3339), (2023).
- Gang Wen, Volker Leen, Taoufik Rohand, Markus Sauer, Johan Hofkens, Current Progress in Expansion Microscopy: Chemical Strategies and Applications, Chemical Reviews, 10.1021/acs.chemrev.2c00711, 123 , 6, (3299-3323), (2023).
- Axelle Wilmerding, Paula Espana-Bonilla, Nikolaos-Nikiforos Giakoumakis, Murielle Saade, Expansion microscopy of the chick embryo neural tube to overcome molecular crowding at the centrosomes-cilia, STAR Protocols, 10.1016/j.xpro.2022.101997, 4 , 1, (101997), (2023).
- Hyun-Jin Kim, Byungjae Hwang, Maria Reva, Jieun Lee, Byeong Eun Lee, Youngeun Lee, Eun Jeong Cho, Minseok Jeong, Seung Eun Lee, Kyungjae Myung, Ja-Hyun Baik, Jung-Hoon Park, Jae-Ick Kim, GABAergic-like dopamine synapses in the brain, Cell Reports, 10.1016/j.celrep.2023.113239, 42 , 10, (113239), (2023).
- Ida M.A. Jentoft, Felix J.B. Bäuerlein, Luisa M. Welp, Benjamin H. Cooper, Arsen Petrovic, Chun So, Sarah Mae Penir, Antonio Z. Politi, Yehor Horokhovskyi, Iina Takala, Heike Eckel, Rüdiger Moltrecht, Peter Lénárt, Tommaso Cavazza, Juliane Liepe, Nils Brose, Henning Urlaub, Rubén Fernández-Busnadiego, Melina Schuh, Mammalian oocytes store proteins for the early embryo on cytoplasmic lattices, Cell, 10.1016/j.cell.2023.10.003, 186 , 24, (5308-5327.e25), (2023).
- Jason Scott Holsapple, Lena Schnitzler, Louisa Rusch, Tobias Horst Baldeweg, Elsa Neubert, Sebastian Kruss, Luise Erpenbeck, Expansion microscopy of neutrophil nuclear structure and extracellular traps, Biophysical Reports, 10.1016/j.bpr.2022.100091, 3 , 1, (100091), (2023).
- Nadine Kraft, Thomas S. Muenz, Sebastian Reinhard, Christian Werner, Markus Sauer, Claudia Groh, Wolfgang Rössler, Expansion microscopy in honeybee brains for high-resolution neuroanatomical analyses in social insects, Cell and Tissue Research, 10.1007/s00441-023-03803-4, 393 , 3, (489-506), (2023).
- Katy E. Pannoni, Daniela Gil, Mikel L. Cawley, Mayd M. Alsalman, Logan A. Campbell, Shannon Farris, Layer‐specific mitochondrial diversity across hippocampal CA2 dendrites, Hippocampus, 10.1002/hipo.23512, 33 , 3, (182-196), (2023).
- Aline Timmermann, Dario Tascio, Ronald Jabs, Anne Boehlen, Catia Domingos, Magdalena Skubal, Wenhui Huang, Frank Kirchhoff, Christian Henneberger, Andras Bilkei‐Gorzo, Gerald Seifert, Christian Steinhäuser, Dysfunction of NG2 glial cells affects neuronal plasticity and behavior, Glia, 10.1002/glia.24352, 71 , 6, (1481-1501), (2023).
- Anastasiya Rakovskaya, Margarita Chigriai, Ilya Bezprozvanny, Ekaterina Pchitskaya, Expansion Microscopy Application for Calcium Protein Clustering Imaging in Cells and Brain Tissues, Current Protocols, 10.1002/cpz1.789, 3 , 6, (2023).
- Zhangyu Cheng, Caroline Stefani, Thomas Skillman, Aleksandra Klimas, Aramchan Lee, Emma F. DiBernardo, Karina Mueller Brown, Tatyana Milman, Yuhong Wang, Brendan R. Gallagher, Katherine Lagree, Bhanu P. Jena, Jose S. Pulido, Scott G. Filler, Aaron P. Mitchell, N. Luisa Hiller, Adam Lacy‐Hulbert, Yongxin Zhao, MicroMagnify: A Multiplexed Expansion Microscopy Method for Pathogens and Infected Tissues, Advanced Science, 10.1002/advs.202302249, 10 , 30, (2023).
- Valeria Veneruso, Emilia Petillo, Fabio Pizzetti, Alessandro Orro, Davide Comolli, Massimiliano De Paola, Antonietta Verrillo, Arianna Baggiolini, Simona Votano, Franca Castiglione, Mattia Sponchioni, Gianluigi Forloni, Filippo Rossi, Pietro Veglianese, Synergistic Pharmacological Therapy to Modulate Glial Cells in Spinal Cord Injury, Advanced Materials, 10.1002/adma.202307747, 36 , 3, (2023).
- Rajiv Kumar, Neelam Chhillar, Mimicry at the Material–Cell Interface, Biomimicry Materials and Applications, 10.1002/9781394167043.ch2, (35-81), (2023).
- Polina Kosillo, Kamran M Ahmed, Erin E Aisenberg, Vasiliki Karalis, Bradley M Roberts, Stephanie J Cragg, Helen S Bateup, Dopamine neuron morphology and output are differentially controlled by mTORC1 and mTORC2, eLife, 10.7554/eLife.75398, 11 , (2022).
- Amy Richardson, Victoria Ciampani, Mihai Stancu, Kseniia Bondarenko, Sherylanne Newton, Joern R Steinert, Nadia Pilati, Bruce P Graham, Conny Kopp-Scheinpflug, Ian D Forsythe, Kv3.3 subunits control presynaptic action potential waveform and neurotransmitter release at a central excitatory synapse, eLife, 10.7554/eLife.75219, 11 , (2022).
- Luis Vigetti, Tatiana Labouré, Chloé Roumégous, Dominique Cannella, Bastien Touquet, Claudine Mayer, Yohann Couté, Karine Frénal, Isabelle Tardieux, Patricia Renesto, The BCC7 Protein Contributes to the Toxoplasma Basal Pole by Interfacing between the MyoC Motor and the IMC Membrane Network, International Journal of Molecular Sciences, 10.3390/ijms23115995, 23 , 11, (5995), (2022).
- Cindy Brunskine, Stefan Passlick, Christian Henneberger, Structural Heterogeneity of the GABAergic Tripartite Synapse, Cells, 10.3390/cells11193150, 11 , 19, (3150), (2022).
- Nikhila Krishnan, Maxx Swoger, Lindsay I Rathbun, Peter J Fioramonti, Judy Freshour, Michael Bates, Alison E Patteson, Heidi Hehnly, Rab11 endosomes and Pericentrin coordinate centrosome movement during pre-abscission in vivo, Life Science Alliance, 10.26508/lsa.202201362, 5 , 7, (e202201362), (2022).
- Wei Wang, Yat Ho Chan, SoYoung Kwon, Jamuna Tandukar, Ruixuan Gao, Nanoscale fluorescence imaging of biological ultrastructure via molecular anchoring and physical expansion, Nano Convergence, 10.1186/s40580-022-00318-6, 9 , 1, (2022).
- Limei Zhang, Teresa Padilla‐Flores, Vito S. Hernández, Mario A. Zetter, Elba Campos‐Lira, Laura I. Escobar, Robert P. Millar, Lee E. Eiden, Vasopressin acts as a synapse organizer in limbic regions by boosting PSD95 and GluA1 expression, Journal of Neuroendocrinology, 10.1111/jne.13164, 34 , 9, (2022).
- Claudia Capitini, Luca Pesce, Giulia Fani, Giacomo Mazzamuto, Massimo Genovese, Alessandra Franceschini, Paolo Paoli, Giuseppe Pieraccini, Michael Zasloff, Fabrizio Chiti, Francesco S. Pavone, Martino Calamai, Studying the trafficking of labeled trodusquemine and its application as nerve marker for light‐sheet and expansion microscopy, The FASEB Journal, 10.1096/fj.202201276R, 36 , 12, (2022).
- Abrar Aljiboury, Amra Mujcic, Erin Curtis, Thomas Cammerino, Denise Magny, Yiling Lan, Michael Bates, Judy Freshour, Yasir H. Ahmed-Braimeh, Heidi Hehnly, Pericentriolar matrix (PCM) integrity relies on cenexin and polo-like kinase (PLK)1, Molecular Biology of the Cell, 10.1091/mbc.E22-01-0015, 33 , 9, (2022).
- Xiaofan Zhang, John Martin Sabandal, George Tsaprailis, Ronald L. Davis, Active forgetting requires Sickie function in a dedicated dopamine circuit in Drosophila , Proceedings of the National Academy of Sciences, 10.1073/pnas.2204229119, 119 , 38, (2022).
- Anna Verschueren, Leyna Boucherit, Ulisse Ferrari, Stéphane Fouquet, Céline Nouvel-Jaillard, Michel Paques, Serge Picaud, José-Alain Sahel, Planar polarity in primate cone photoreceptors: a potential role in Stiles Crawford effect phototropism, Communications Biology, 10.1038/s42003-021-02998-y, 5 , 1, (2022).
- Ryohei Kufukihara, Nobuyuki Tanaka, Kimiharu Takamatsu, Naoya Niwa, Keishiro Fukumoto, Yota Yasumizu, Toshikazu Takeda, Kazuhiro Matsumoto, Shinya Morita, Takeo Kosaka, Eriko Aimono, Hiroshi Nishihara, Ryuichi Mizuno, Mototsugu Oya, Hybridisation chain reaction-based visualisation and screening for lncRNA profiles in clear-cell renal-cell carcinoma, British Journal of Cancer, 10.1038/s41416-022-01895-3, 127 , 6, (1133-1141), (2022).
- Brittany M. White, Pratik Kumar, Amanda N. Conwell, Kane Wu, Jeremy M. Baskin, Lipid Expansion Microscopy, Journal of the American Chemical Society, 10.1021/jacs.2c03743, 144 , 40, (18212-18217), (2022).
- Jakub Pospíšil, Miloš Hrabovský, Dáša Bohačiaková, Zuzana Hovádková, Miroslav Jurásek, Jarmila Mlčoušková, Kamil Paruch, Šárka Nevolová, Jiri Damborsky, Aleš Hampl, Josef Jaros, Geometric Control of Cell Behavior by Biomolecule Nanodistribution, ACS Biomaterials Science & Engineering, 10.1021/acsbiomaterials.2c00650, 8 , 11, (4789-4806), (2022).
- Diane Bissen, Maximilian Ken Kracht, Franziska Foss, Amparo Acker-Palmer, Expansion microscopy of mouse brain organotypic slice cultures to study protein distribution, STAR Protocols, 10.1016/j.xpro.2022.101507, 3 , 3, (101507), (2022).
- David M. Sherry, Megan A. Stiles, Improved fluorescent signal in expansion microscopy using fluorescent Fab fragment secondary antibodies, MethodsX, 10.1016/j.mex.2022.101796, 9 , (101796), (2022).
- Gerd Keiser, Gerd Keiser, Microscopy, Biophotonics, 10.1007/978-981-19-3482-7_8, (261-292), (2022).
- Ory Perelsman, Shoh Asano, Limor Freifeld, Expansion Microscopy of Larval Zebrafish Brains and Zebrafish Embryos, Fluorescent Microscopy, 10.1007/978-1-0716-2051-9_13, (211-222), (2022).
- Chi‐Hung Lin, Tzu‐Yang Lin, Shao‐Chun Hsu, Hwei‐Jan Hsu, Expansion Microscopy‐based imaging for visualization of mitochondria in Drosophila ovarian germline stem cells, FEBS Open Bio, 10.1002/2211-5463.13506, 12 , 12, (2102-2110), (2022).
- Lindsay E. Stoyka, Casey L. Mahoney, Drake R. Thrasher, Drèson L. Russell, Anna K. Cook, Anner T. Harris, Ashwin Narayanan, Tiara P. Janado, David G. Standaert, Erik D. Roberson, Laura A. Volpicelli-Daley, Templated α-Synuclein Inclusion Formation Is Independent of Endogenous Tau, eneuro, 10.1523/ENEURO.0458-20.2021, 8 , 3, (ENEURO.0458-20.2021), (2021).
- Ruixi Chen, Xiaofeng Cheng, Yongsheng Zhang, Xiong Yang, Yu Wang, Xiuli Liu, Shaoqun Zeng, Expansion tomography for large volume tissue imaging with nanoscale resolution, Biomedical Optics Express, 10.1364/BOE.431696, 12 , 9, (5614), (2021).
- K. Z. Derevtsova, E. I. Pchitskaya, A. V. Rakovskaya, I. B. Bezprozvanny, Applying the Expansion Microscopy Method in Neurobiology, Journal of Evolutionary Biochemistry and Physiology, 10.1134/S0022093021030157, 57 , 3, (681-693), (2021).
- Kevin L Cox Jr, Sai Guna Ranjan Gurazada, Keith E Duncan, Kirk J Czymmek, Christopher N Topp, Blake C Meyers, Organizing your space: The potential for integrating spatial transcriptomics and 3D imaging data in plants, Plant Physiology, 10.1093/plphys/kiab508, 188 , 2, (703-712), (2021).
- Ruixuan Gao, Chih-Chieh Yu, Linyi Gao, Kiryl D. Piatkevich, Rachael L. Neve, James B. Munro, Srigokul Upadhyayula, Edward S. Boyden, A highly homogeneous polymer composed of tetrahedron-like monomers for high-isotropy expansion microscopy, Nature Nanotechnology, 10.1038/s41565-021-00875-7, 16 , 6, (698-707), (2021).
- Mingyuan Chen, Tengqian Sun, Yanghao Zhong, Xin Zhou, Jin Zhang, A Highly Sensitive Fluorescent Akt Biosensor Reveals Lysosome-Selective Regulation of Lipid Second Messengers and Kinase Activity, ACS Central Science, 10.1021/acscentsci.1c00919, 7 , 12, (2009-2020), (2021).
- Lauranne Drelich, Soulaimane Aboulouard, Julien Franck, Michel Salzet, Isabelle Fournier, Maxence Wisztorski, Toward High Spatially Resolved Proteomics Using Expansion Microscopy, Analytical Chemistry, 10.1021/acs.analchem.0c05372, 93 , 36, (12195-12203), (2021).
- Antoine Gaudreau-Lapierre, Kirk Mulatz, Jean-Claude Béïque, Laura Trinkle-Mulcahy, Expansion microscopy-based imaging of nuclear structures in cultured cells, STAR Protocols, 10.1016/j.xpro.2021.100630, 2 , 3, (100630), (2021).
- Logan A. Campbell, Katy E. Pannoni, Niesha A. Savory, Dinesh Lal, Shannon Farris, Protein-retention expansion microscopy for visualizing subcellular organelles in fixed brain tissue, Journal of Neuroscience Methods, 10.1016/j.jneumeth.2021.109285, 361 , (109285), (2021).
- Diane Bissen, Maximilian Ken Kracht, Franziska Foss, Jan Hofmann, Amparo Acker-Palmer, EphrinB2 and GRIP1 stabilize mushroom spines during denervation-induced homeostatic plasticity, Cell Reports, 10.1016/j.celrep.2021.108923, 34 , 13, (108923), (2021).
- Courtney A. Burger, Nicholas E. Albrecht, Danye Jiang, Justine H. Liang, Ross A. Poché, Melanie A. Samuel, LKB1 and AMPK instruct cone nuclear position to modify visual function, Cell Reports, 10.1016/j.celrep.2021.108698, 34 , 5, (108698), (2021).
- Davide Gambarotto, Virginie Hamel, Paul Guichard, Ultrastructure expansion microscopy (U-ExM), Expansion Microscopy for Cell Biology, 10.1016/bs.mcb.2020.05.006, (57-81), (2021).
- Fabian U. Zwettler, Sebastian Reinhard, Markus Sauer, Ex-dSTORM and automated quantitative image analysis of expanded filamentous structures, Expansion Microscopy for Cell Biology, 10.1016/bs.mcb.2020.05.004, (317-340), (2021).
- Thomas M.D. Sheard, Izzy Jayasinghe, Enhanced expansion microscopy to measure nanoscale structural and biochemical remodeling in single cells, Expansion Microscopy for Cell Biology, 10.1016/bs.mcb.2020.04.019, (147-180), (2021).
- Sven Truckenbrodt, Silvio O. Rizzoli, Simple multi-color super-resolution by X10 microscopy, Expansion Microscopy for Cell Biology, 10.1016/bs.mcb.2020.04.016, (33-56), (2021).
- Ivana Ponjavić, Kruno Vukušić, Iva M. Tolić, Expansion microscopy of the mitotic spindle, Expansion Microscopy for Cell Biology, 10.1016/bs.mcb.2020.04.014, (247-274), (2021).
- Jae-Byum Chang, Ruixuan Gao, Fei Chen, Light-Sheet Fluorescence Microscopy for Multiscale Biological Imaging, Molecular Imaging, 10.1016/B978-0-12-816386-3.00026-0, (373-382), (2021).
- Dong Kong, Jadranka Loncarek, Analyzing Centrioles and Cilia by Expansion Microscopy, Cell Cycle Oscillators, 10.1007/978-1-0716-1538-6_18, (249-263), (2021).
- W. Matt Reilly, Christopher J. Obara, Advances in Confocal Microscopy and Selected Applications, Confocal Microscopy, 10.1007/978-1-0716-1402-0_1, (1-35), (2021).
- Courtney A Burger, Jonathan Alevy, Anna K Casasent, Danye Jiang, Nicholas E Albrecht, Justine H Liang, Arlene A Hirano, Nicholas C Brecha, Melanie A Samuel, LKB1 coordinates neurite remodeling to drive synapse layer emergence in the outer retina, eLife, 10.7554/eLife.56931, 9 , (2020).
- Weng Man Chong, Won-Jing Wang, Chien-Hui Lo, Tzu-Yuan Chiu, Ting-Jui Chang, You-Pi Liu, Barbara Tanos, Gregory Mazo, Meng-Fu Bryan Tsou, Wann-Neng Jane, T Tony Yang, Jung-Chi Liao, Super-resolution microscopy reveals coupling between mammalian centriole subdistal appendages and distal appendages, eLife, 10.7554/eLife.53580, 9 , (2020).
- Arnaldo Parra-Damas, Carlos A. Saura, Tissue Clearing and Expansion Methods for Imaging Brain Pathology in Neurodegeneration: From Circuits to Synapses and Beyond, Frontiers in Neuroscience, 10.3389/fnins.2020.00914, 14 , (2020).
- Steven J. Edwards, Valentina Carannante, Kyra Kuhnigk, Henrik Ring, Tatsiana Tararuk, Finn Hallböök, Hans Blom, Björn Önfelt, Hjalmar Brismar, High-Resolution Imaging of Tumor Spheroids and Organoids Enabled by Expansion Microscopy, Frontiers in Molecular Biosciences, 10.3389/fmolb.2020.00208, 7 , (2020).
- Guillaume Jacquemet, Alexandre F. Carisey, Hellyeh Hamidi, Ricardo Henriques, Christophe Leterrier, The cell biologist's guide to super-resolution microscopy, Journal of Cell Science, 10.1242/jcs.240713, 133 , 11, (2020).
- Sara Rombouts, Marcelo Nollmann, RNA imaging in bacteria, FEMS Microbiology Reviews, 10.1093/femsre/fuaa051, 45 , 2, (2020).
- Yohei Katoh, Shuhei Chiba, Kazuhisa Nakayama, Practical method for superresolution imaging of primary cilia and centrioles by expansion microscopy using an amplibody for fluorescence signal amplification, Molecular Biology of the Cell, 10.1091/mbc.E20-04-0250, 31 , 20, (2195-2206), (2020).
- N A Rumyantseva, A D Vedyaykin, I E Vishnyakov, M A Khodorkovskii, Visualization of the intracellular structures of bacteria using expansion microscopy, Journal of Physics: Conference Series, 10.1088/1742-6596/1697/1/012048, 1697 , 1, (012048), (2020).
- Benjamin C. Campbell, Elisa M. Nabel, Mitchell H. Murdock, Cristina Lao-Peregrin, Pantelis Tsoulfas, Murray G. Blackmore, Francis S. Lee, Conor Liston, Hirofumi Morishita, Gregory A. Petsko, mGreenLantern: a bright monomeric fluorescent protein with rapid expression and cell filling properties for neuronal imaging, Proceedings of the National Academy of Sciences, 10.1073/pnas.2000942117, 117 , 48, (30710-30721), (2020).
- Yehlin Cho, Junyoung Seo, Yeonbo Sim, Jinkyoung Chung, Chan E. Park, Chun Gwon Park, Doory Kim, Jae-Byum Chang, FRACTAL: Signal amplification of immunofluorescence via cyclic staining of target molecules , Nanoscale, 10.1039/D0NR05800A, 12 , 46, (23506-23513), (2020).
- Octavian Bucur, Feifei Fu, Mike Calderon, Geetha H. Mylvaganam, Ngoc L. Ly, Jimmy Day, Simon Watkin, Bruce D. Walker, Edward S. Boyden, Yongxin Zhao, Nanoscale imaging of clinical specimens using conventional and rapid-expansion pathology, Nature Protocols, 10.1038/s41596-020-0300-1, 15 , 5, (1649-1672), (2020).
- Ons M’Saad, Joerg Bewersdorf, Light microscopy of proteins in their ultrastructural context, Nature Communications, 10.1038/s41467-020-17523-8, 11 , 1, (2020).
- Gang Wen, Marisa Vanheusden, Aline Acke, Donato Valli, Robert K. Neely, Volker Leen, Johan Hofkens, Evaluation of Direct Grafting Strategies via Trivalent Anchoring for Enabling Lipid Membrane and Cytoskeleton Staining in Expansion Microscopy , ACS Nano, 10.1021/acsnano.9b09259, 14 , 7, (7860-7867), (2020).
- See more