Differentiation and Subculturing of Renal Proximal Tubular-like Cells Derived from Human iPSC
Tamara Meijer, Tamara Meijer, Elisabeth Naderlinger, Elisabeth Naderlinger, Paul Jennings, Paul Jennings, Anja Wilmes, Anja Wilmes
Abstract
Recently, we have developed a protocol to differentiate human induced pluripotent stem cells (iPSC) into proximal tubular-like cells (PTL) (Chandrasekaran et al., 2021). These cells express proximal tubular-specific markers, including megalin, and form a polarized monolayer expressing tight junction proteins, including ZO-3 and occludin. Furthermore, PTL display functional properties, including megalin-facilitated endocytosis, P-glycoprotein (ABCB1) efflux, and respond to parathyroid hormone. Here, we report step-by-step protocols to culture iPSC prior to differentiation (Basic Protocol 1), to differentiate PTL from iPSC (Basic Protocol 2), and to passage and freeze-thaw PTL (Basic Protocol 3). Additionally, we provide a protocol (Basic Protocol 4) to culture PTL on microporous growth supports (transwells). Immunofluorescence stainings for characteristic markers, including megalin, are shown for unpassaged (Basic Protocol 2) and passaged (Basic Protocol 3) PTL. © 2023 The Authors. Current Protocols published by Wiley Periodicals LLC.
Basic Protocol 1 : iPSC culture
Basic Protocol 2 : iPSC-derived PTL differentiation
Basic Protocol 3 : PTL passaging, culturing, and freezing
Basic Protocol 4 : PTL culturing on transwells
Support Protocol 1 : Preparation of Geltrex-coated cell culture plates
Support Protocol 2 : Preparation of RPTEC/TERT1 or fHDF/TERT166-ECM-coated cell culture plates
Support Protocol 3 : Preparation of human collagen IV-coated cell culture plates
Support Protocol 4 : Immunofluorescence staining
INTRODUCTION
The proximal tubule is a part of the nephron that is particularly susceptible to toxicity due to the presence of numerous transporters and xenobiotic-metabolizing enzymes that may lead to increased intracellular exposure levels to toxic compounds (Jennings et al., 2014). Drug-induced nephrotoxicity can exacerbate chronic kidney disease, which is recognized as a global health problem as the number of patients is on the rise (Awdishu & Mehta, 2017; El Nahas & Bello, 2005). Therefore, it is essential to develop human in vitro renal models that recapitulate human kidney characteristics and in vivo processes to study drug-induced nephrotoxicity. Several renal in vitro models have emerged over the last years to study proximal tubule injury (Jennings et al., 2014), including renal cell lines such as the hTERT-immortalized renal proximal tubular epithelial cell line RPTEC/TERT1 (Limonciel et al., 2012; Wieser et al., 2008; Wilmes et al., 2015). However, one of the limitations of employing cell lines in toxicity studies is that they are derived from a single donor and thus only reflect one genetic background (Jennings, 2015; Rauch et al., 2014). The discovery of induced pluripotent stem cells (iPSC) (Takahashi & Yamanaka, 2006) has opened up new opportunities in the fields of toxicity testing and regenerative medicine (Rauch et al., 2014). The possibility of differentiating human iPSC from healthy donors, as well as from donors carrying genetic diseases, into various target cell types allows the investigation of drug-induced toxicity in human in vitro cell models with different genetic backgrounds (Jennings, 2015; Rauch et al., 2014).
Human iPSC can be directly differentiated into proximal tubular-like cells (PTL) within 14 days by the addition of small molecules and growth factors (Chandrasekaran et al., 2021). These iPSC-derived PTL cells display a polarized phenotype, express proximal tubular-specific proteins, such as megalin, and show functional characteristics of proximal tubule cells, including response to parathyroid hormone, uptake of albumin and ABCB1 transport activity (Chandrasekaran et al., 2021). PTL have been used in several transcriptomic studies which supported the utilization of these cells in toxicity assessment and prediction (Jennings et al., 2022; Nunes et al., 2022; Singh et al., 2021; Snijders et al., 2021). The human iPSC-derived PTL model was exposed to several model compounds, including cadmium (Singh et al., 2021), paraquat (Nunes et al., 2022), and bardoxolone-methyl (Snijders et al., 2021), to investigate toxicity mechanisms. Furthermore, the PTL cells were employed to study temporal effects of stress response pathways by exposure to a range of compounds known to activate specific known stress response pathways, including sodium arsenite, tunicamycin, amiodarone, and rotenone (Jennings et al., 2022). In addition to transcriptomics, activation of the HMOX1 stress response gene was monitored by exposing PTL differentiated from the fluorescent HMOX1 reporter human iPSC line (Snijders et al., 2021) to compounds reported to induce oxidative stress (Jennings et al., 2022). Overall, the human iPSC-derived PTL model shows to be promising in studying and predicting drug-induced toxicity.
Here, we describe a protocol to efficiently differentiate human iPSC into PTL, along with passaging and freezing procedures. Basic Protocol 1 describes iPSC culture methods. Basic Protocol 2 provides a detailed overview on the differentiation of iPSC into PTL. Subsequent PTL passaging and freezing methods are presented in Basic Protocol 1. Additionally, PTL can be cultured on transwells (aka filter inserts or microporous growth supports) which is described in Basic Protocol 4. Support Protocols 1, 2 and 3 provide information on different coating methods. Lastly, Support Protocol 4 explains the procedure for performing immunofluorescence staining of characteristic markers of differentiated and passaged PTL.
NOTE : We tested the above-described protocols across seven different human iPSC lines, including StemBANCC (Morrison et al., 2015) cell lines SBAD3 clone 1, SBAD2 clone 1, SFC086 clone 1, SFC018 clone 1, SFC156 clone 1, SFC840 clone 1, and SBNEO clone 1. No modifications to the protocols were required, as the described procedures worked equally well across these iPSC lines.
CAUTION : All cell culture procedures, with the exclusion of immunofluorescence staining described in Support Protocol 4, should be conducted under sterile conditions in a biosafety cabinet. Cell culture handling should be performed according to the safety rules applied to cell line used and relevant country regulations. It is critical to follow the Good Cell Culture Practice (GCCP) guidelines when working with cell cultures (Pamies et al., 2017, 2022).
NOTE : All protocols involving animals must be reviewed and approved by the appropriate Animal Care and Use Committee and must follow regulations for the care and use of laboratory animals. Appropriate informed consent is necessary for obtaining and use of human study material.
Basic Protocol 1: iPSC CULTURE
This protocol provides instructions for culturing iPSC, including non-enzymatic passaging, as well as freezing and thawing procedures. iPSC are cultured in mTeSR™1 medium on Geltrex™ human embryonic stem cell (hESC)-qualified reduced growth factor basement membrane matrix (Geltrex) coating. To achieve optimal results, iPSC should be passaged at a confluency of approximately 60 to 70% (Fig. 1). Full confluency (100%) should be avoided as this may lead to poor passaging results. Furthermore, it is recommended to maintain iPSC in small cell colonies during passaging; this can be achieved by using ethylenediaminetetraacetic acid (EDTA) and avoiding excessive pipetting.
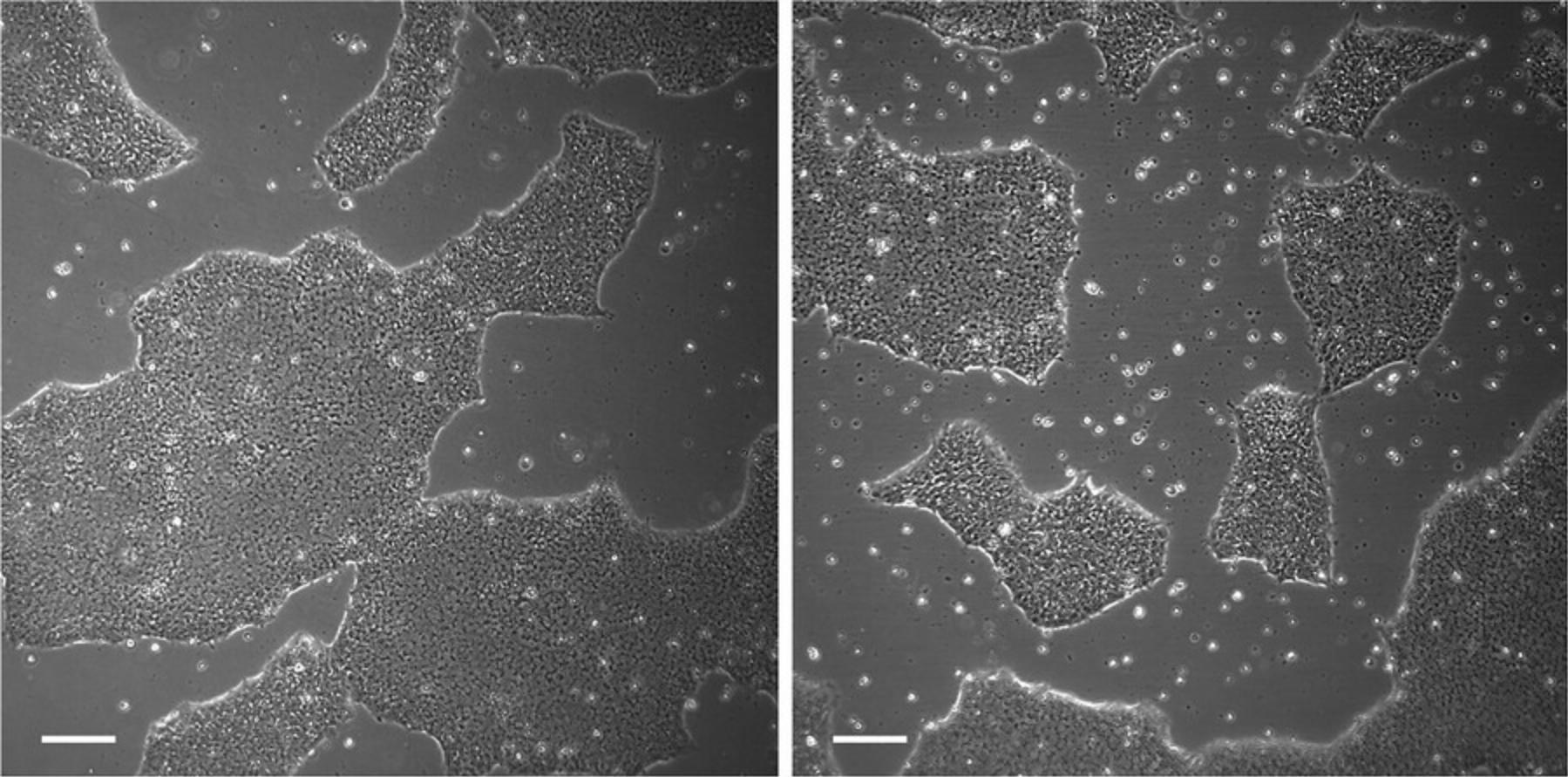
Materials
- Geltrex (Gibco, cat. no. A14133-02)
- mTeSR™1, supplemented (see recipe)
- Y-27632 dihydrochloride ROCK inhibitor, 10 mM (see recipe)
- Human iPSC, e.g., SBAD3 clone 1 (Wilmes et al., 2017), or other human iPSC lines
- Dulbecco′s phosphate-buffered saline (DPBS), no calcium, no magnesium (Gibco, cat. no. 14190-144)
- EDTA, 0.48 mM (Gibco, cat. no. 15040-066)
- iPSC freezing medium, 2× (see recipe)
-
- 6-well cell culture plate, coated with Geltrex (see Support Protocol 1)
- Water bath
- 15-ml Centrifuge tubes
- Pipet aid
- 10-ml Serological pipets
- 1000-µl Pipette
- 1000-µl Pipette tips
- 1000-µl Pipette tips, with filter
- Cryovials
- Centrifuge
- Cell culture incubator, set to 36.5°C, 5% CO2, humidified
- Biosafety cabinet
- Inverted microscope
- Vacuum pump, for aspiration
- Ice
- Cell freezing container
Thawing iPSC
All volumes described below are based on two wells of a 6-well plate. Adjust the volumes accordingly if other plate formats are used.
1.Pre-warm a Geltrex-coated plate for 15 min at 37°C, if stored at 4°C.
2.Pre-warm 4 ml mTeSR1 medium and 4 ml mTeSR1 medium supplemented with 10 µM Y-27632 dihydrochloride ROCK inhibitor for 15 min in a 37°C water bath.
3.Carefully defrost a cryovial in a 37°C water bath until only a small amount of frozen sample remains and proceed quickly to step 4.
4.Transfer the contents of the vial to a tube containing 4 ml warm mTeSR1 medium.
5.Centrifuge for 5 min at 300 × g , room temperature.
6.Aspirate the supernatant from two wells of the warm Geltrex-coated plate and transfer 1.2 ml and 1.8 ml warm mTeSR1 medium supplemented with 10 µM Y-27632 dihydrochloride ROCK inhibitor to each of the wells.
7.Carefully aspirate the supernatant from the centrifuged cells without disturbing the cell pellet.
8.Gently resuspend the cell pellet in 1 ml warm mTeSR1 medium supplemented with 10 µM Y-27632 dihydrochloride ROCK inhibitor.
9.Add 0.8 ml cell suspension to the well containing 1.2 ml medium and 0.2 ml cell suspension to the well containing 1.8 ml medium.
10.Gently rock the plate to spread the cells equally over the wells.
11.Carefully transfer the plate to the cell culture incubator.
12.Feed the cells the next day with warm mTeSR1 medium.
13.Continue to feed the cells every day with warm mTeSR1 medium until cells are 60 to 70% confluent.
Passaging iPSC using 0.48 mM EDTA
All volumes described below are based on one well of a 6-well plate. Adjust the volumes accordingly if other plate formats are used.
14.Pre-warm a Geltrex-coated plate for 15 min at 37°C, if stored at 4°C.
15.Pre-warm 3 ml mTeSR1 medium for 15 min in a 37°C water bath.
16.Aspirate medium from a 60 to 70% confluent well of iPSC.
17.Add 1 ml DPBS and aspirate.
18.Add 0.5 ml 0.48 mM EDTA, gently rock the plate to cover all cells with EDTA, and aspirate.
19.Add 0.5 ml 0.48 mM EDTA, gently rock the plate to cover all cells with EDTA, and incubate for 3 to 6 min at 36.5°C.
20.Carefully aspirate EDTA.
21.Detach the cells from the plate with 1 ml warm mTeSR1 medium with gentle pipetting.
22.Plate out cells at split ratios between 1:3 to 1:25.
-
Aspirate the supernatant from the warm Geltrex-coated plate and add warm mTeSR1 medium. The volume of medium is dependent on the selected split ratio (see below for an example).
-
Add the cell suspension to the well containing mTeSR1 medium. The volume of cell suspension is dependent on the selected split ratio (see below for an example).
23.Check the cells with an inverted microscope to see whether the cell amount is sufficient and whether most cells are in small colonies.
24.Gently rock the plate to spread the cells equally over the wells.
25.Carefully transfer the plate to the cell culture incubator.
26.Feed the cells the second day after passaging with warm mTeSR1 medium.
27.Continue to feed the cells every day with warm mTeSR1 medium.
28.Passage iPSC once 60 to 70% confluency is reached.
Freezing iPSC
All volumes described below are based on one well of a 6-well plate. Adjust the volumes accordingly if other plate formats are used.
29.Pre-cool one cryovial in a cold cell freezing container.
30.Pre-cool 0.5 ml 2× iPSC freezing medium and 0.5 ml mTeSR1 medium on ice.
31.Follow steps 16 to 20 of Passaging iPSC using 0.48 mM EDTA in Basic Protocol 1.
32.Detach the cells from the plate with 0.5 ml ice-cold mTeSR1 medium with gentle pipetting.
33.Transfer 0.5 ml cell suspension to one pre-cooled cryovial in the cell freezing container.
34.Add 0.5 ml ice-cold 2× iPSC freezing medium to the cell suspension in the cryovial and pipette up and down once.
35.Store the cell freezing container containing the cryovial at −80°C overnight.
36.Transfer the cryovial to liquid nitrogen storage the next day for long-term storage.
Basic Protocol 2: iPSC-DERIVED PTL DIFFERENTIATION
This protocol outlines the procedure to differentiate iPSC into PTL within 14 days. PTL can be differentiated on either growth factor reduced Geltrex or de-cellularized extracellular matrix (ECM) derived from cultured RPTEC/TERT1 cells, or from cultured human dermal fibroblasts fHDF/TERT166, as previously described (Chandrasekaran et al., 2021; Murphy et al., 2023).
Extra care should be taken regarding the starting confluency of the iPSC (Fig. 1), the seeding density of the cells on day 0, and the precise timing of the feeding intervals up to day 6.PTL should reach confluency on day 3 of the differentiation (Fig. 2). Occasionally, holes in the monolayer may be observed on day 6 after FGF-9 treatment. However, as long as the cells grow back into a confluent monolayer by day 8, this is not a problem. If holes persist after day 8, discard the culture and start a new differentiation.
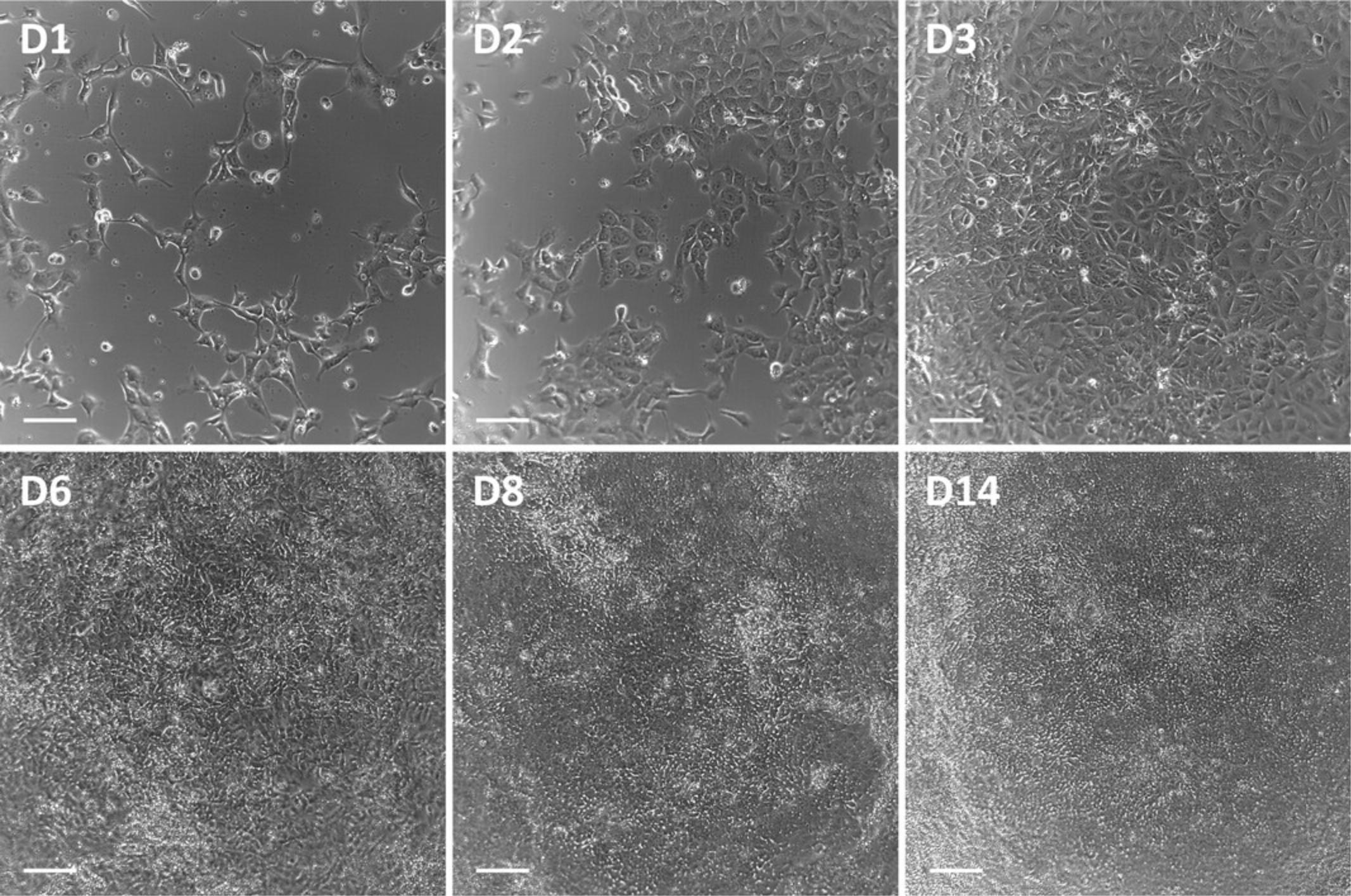
PTL are considered fully differentiated after 14 days, which should be confirmed by immunofluorescence staining of proximal tubular-specific markers as well as markers for tight junctions to confirm polarization (Fig. 3, see Support Protocol 4).
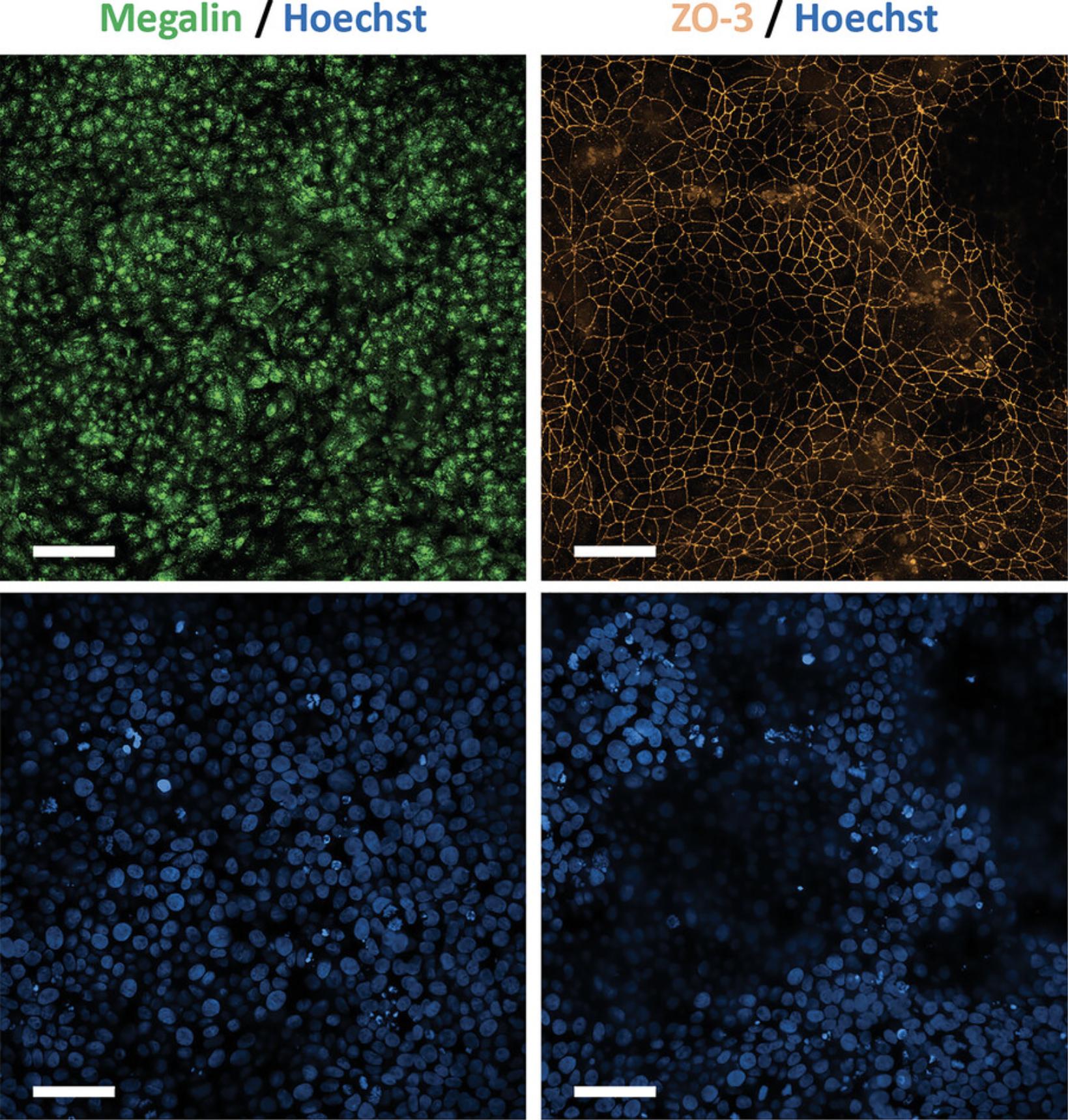
Materials
- PTL-A medium (see recipe)
- Human iPSC, e.g., SBAD3 clone 1 (Wilmes et al., 2017), or other human iPSC lines
- DPBS, no calcium, no magnesium (Gibco, cat. no. 14190-144)
- StemPro™ accutase™ cell dissociation reagent (Gibco, cat. no. A11105-01)
- CHIR99021, 5 mM (see recipe)
- TTNPB, 5 mM (see recipe)
- Y-27632 dihydrochloride ROCK inhibitor, 10 mM (see recipe)
- Trypan blue solution, 0.4% (Millipore Sigma, cat. no. T8154)
- PTL-B medium (see recipe)
- FGF-9, 0.1 mg/ml (see recipe)
-
- 6-well cell culture plate, coated with Geltrex (see Support Protocol 1), or alternatively coated with RPTEC/TERT1 or fHDF/TERT166-ECM (see Support Protocol 2)
- Pipet aid
- 10-ml Serological pipets
- 1000-µl Pipette
- 1000-µl Pipette tips
- 1000-µl Pipette tips, with filter
- 50-ml Centrifuge tubes
- Water bath
- Cell culture incubator, set to 36.5°C, 5% CO2, humidified
- Biosafety cabinet
- Inverted microscope
- Vacuum pump, for aspiration
- Centrifuge
- Cell counter
Day 0 (seeding day)
All volumes described below are based on one well of a 6-well plate. Adjust the volumes accordingly if other plate formats are used.
1.Pre-warm PTL-A medium in a 37°C water bath.
2.Pre-warm plates coated with either RPTEC/TERT1-ECM, fHDF/TERT166-ECM, or Geltrex for 15 min at 37°C, if stored at 4°C.
3.Aspirate medium from a 60 to 70% confluent well of iPSC.
4.Add 1 ml DPBS and aspirate.
5.Add 0.5 ml accutase, gently rock the plate to cover all cells with accutase, and incubate for approximately 2 to 4 min at 36.5°C.
6.Detach the cells from the plate with gentle pipetting.
7.Transfer the detached cells to a tube containing 10 ml warm PTL-A medium.
8.Centrifuge for 5 min at 300 × g , room temperature.
9.Carefully aspirate the supernatant without disturbing the cell pellet.
10.Resuspend the cell pellet in 1.5 ml freshly prepared warm PTL-A medium supplemented with 3 µM CHIR99021, 1 µM TTNPB, and 10 µM Y-27632 dihydrochloride ROCK inhibitor.
11.Count the live cells in a cell counter using a 1:1 ratio of cell suspension and 0.4% trypan blue solution.
12.Prepare a stock of cells at a seeding density of 3.5 × 104 cells/cm2 in warm PTL-A medium supplemented with 3 µM CHIR99021, 1 µM TTNPB, and 10 µM Y-27632 dihydrochloride ROCK inhibitor.
13.From this stock, plate out cells into the desired plate formats.
14.Gently rock the plate to spread the cells equally over the wells.
15.Carefully transfer the plate to the cell culture incubator.
Day 2
16.After 42 hr (± 2 hr), replace the medium with freshly prepared warm PTL-A medium supplemented with 1 µM TTNPB only.
Day 3
17.After a further 30 hr (± 2 hr), replace the medium with freshly prepared warm PTL-B medium supplemented with 10 ng/ml FGF-9.
Day 6
18.After a further 48 to 65 hr, replace the medium with warm PTL-B medium.
Day 7 and onward
19.Feed the cells every 2 to 3 days with warm PTL-B medium.
Day 14 to Day 20
20.PTL cells are differentiated and ready to be used or passaged (see Basic Protocol 3).
Basic Protocol 3: PTL PASSAGING, CULTURING, AND FREEZING
At the end of the PTL differentiation protocol (see step 20 of Basic Protocol 2), the cells can only be passaged once as PTL will lose their differentiation markers after any additional passage. It is possible to either plate the cells out into the desired plate format or freeze them in liquid nitrogen for long-term storage. Please note that after thawing, the cells cannot be passaged anymore and should be used immediately after the maturation period.
Since differentiated PTL cells produce their own ECM, no coating is required when replating PTL (Chandrasekaran et al., 2021). Nevertheless, for smaller formats such as a 96-well plate, or smaller, a coating is recommended. In such cases, we recommend one of the following coatings: RPTEC/TERT1 or fHDF/TERT166-ECM coating (see Support Protocol 2) (Chandrasekaran et al., 2021; Murphy et al., 2023), or human collagen IV coating (see Support Protocol 3).
For subsequent assays, it is recommended that PTL are used between day 7 and 15 after replating (Fig. 4). It is essential to include the TGF-beta type I receptor inhibitor GW788388 hydrate in the cell culture medium at all times, as in its absence the passaged cells will lose their polarization (Chandrasekaran et al., 2021). It is advised to assess the maturation status of passaged PTL by staining of the proximal tubular-enriched marker megalin and the tight junction marker tight junction protein 3 (TJP3 aka ZO-3) to confirm polarization prior to conducting experiments (Fig. 5, see Support Protocol 4).
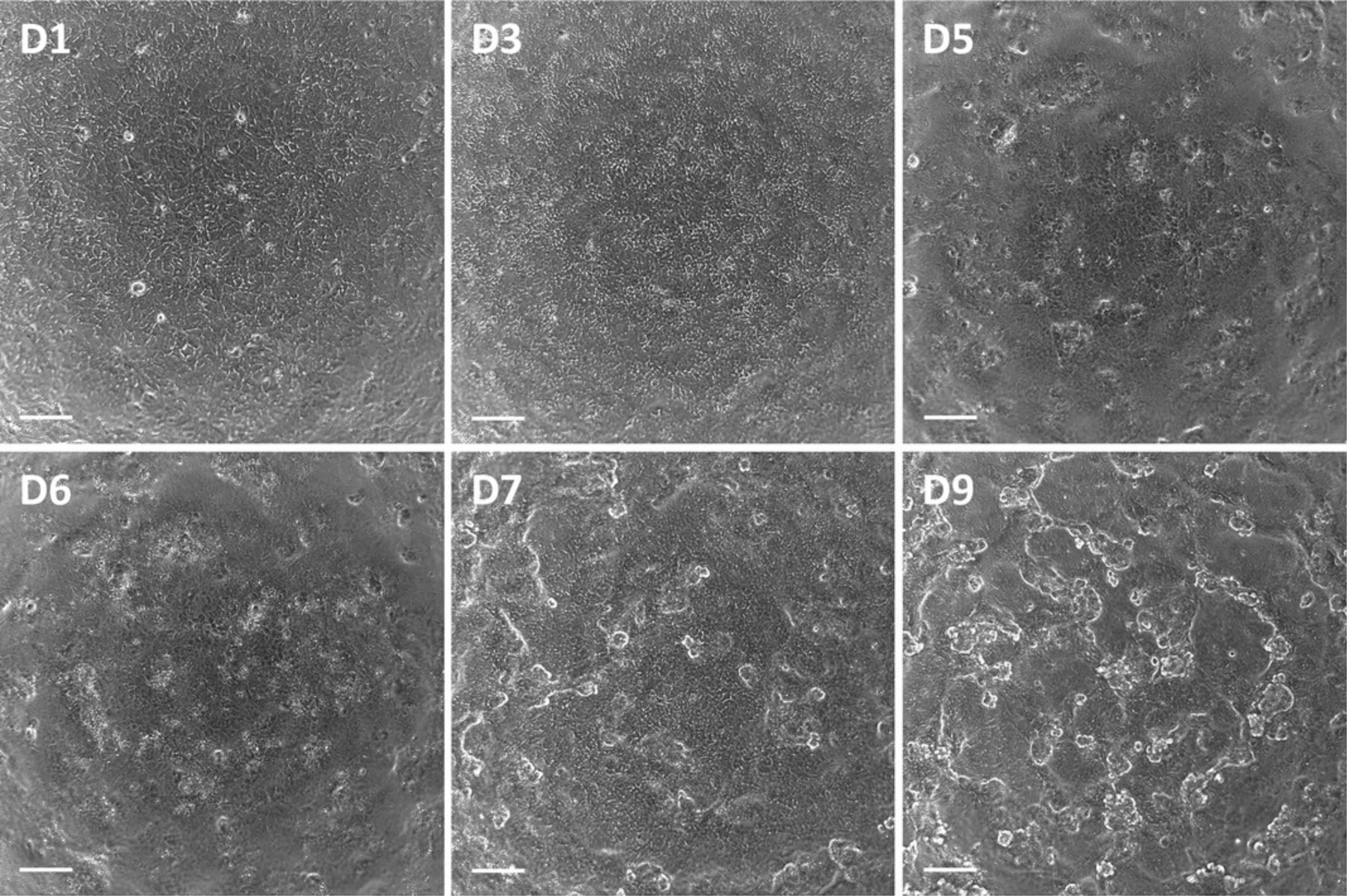
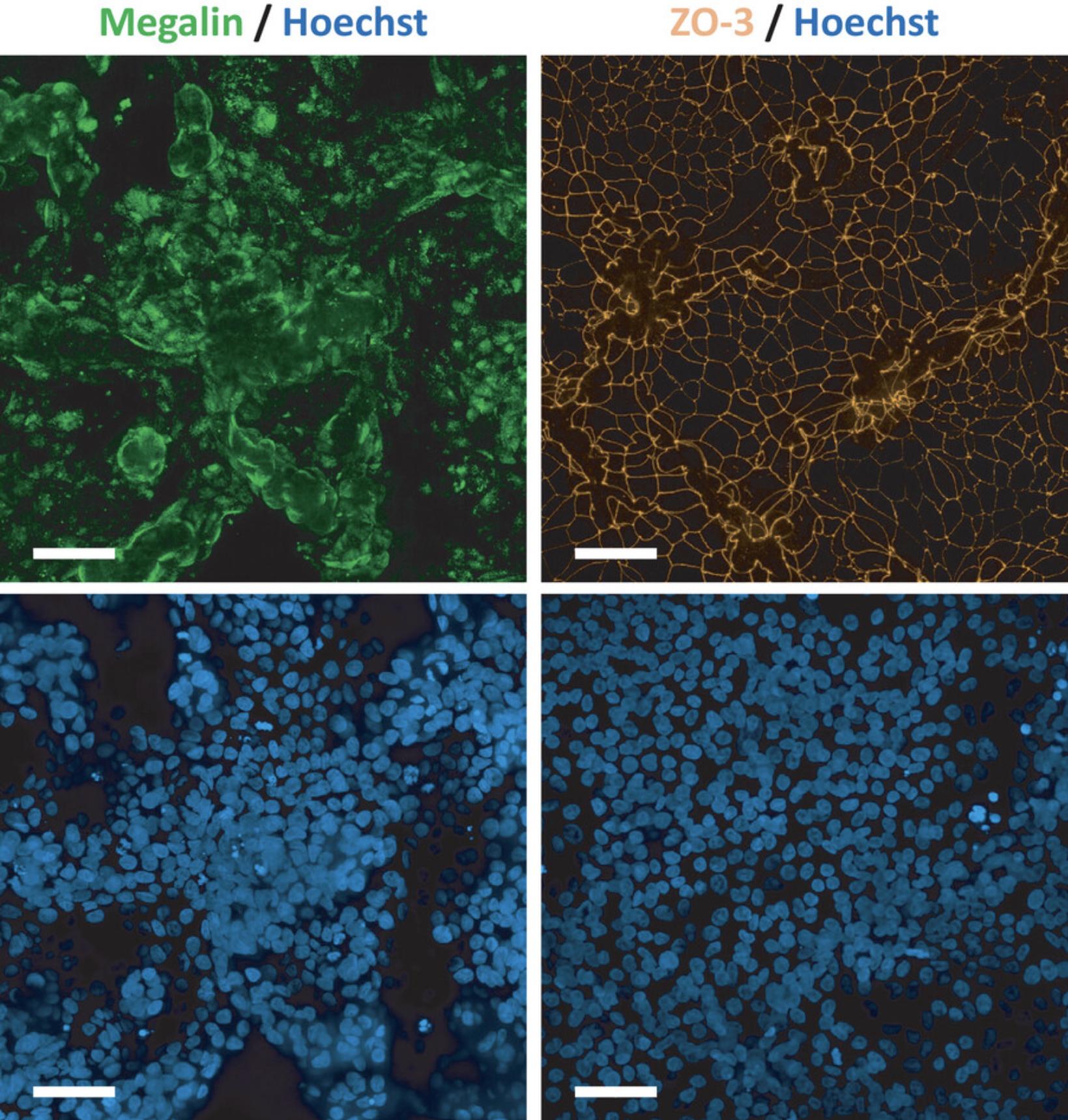
Materials
- PTL-B medium (see recipe)
- TrypLE™ express enzyme, 1× (Gibco, cat. no. 12604-013)
- Differentiated PTL day 14 to 20 (see step 20 of Basic Protocol 2)
- DPBS, no calcium, no magnesium (Gibco, cat. no. 14190-144)
- EDTA, 0.48 mM (Gibco, cat. no. 15040-066)
- Fetal bovine serum (FBS) (Gibco, cat. no. 10270-106)
- Deoxyribonuclease I (DNase I), 10,000 Kunitz units/ml (see recipe)
- GW788388 hydrate, 1 mM (see recipe)
- Y-27632 dihydrochloride ROCK inhibitor, 10 mM (see recipe)
- Trypan blue solution, 0.4% (Millipore Sigma, cat. no. T8154)
- PTL freezing medium (see recipe)
- PTL-A medium (see recipe)
-
- 6-well cell culture plate
- Optional: coated with RPTEC/TERT1 or fHDF/TERT166-ECM (see Support Protocol 2), or human collagen IV (see Support Protocol 3)
- Pipet aid
- 10-ml Serological pipets
- 1000-µl Pipette
- 1000-µl Pipette tips
- 1000-µl Pipette tips, with filter
- 50-ml Centrifuge tubes
- Water bath
- Cell culture incubator, set to 36.5°C, 5% CO2, humidified
- Biosafety cabinet
- Inverted microscope
- Vacuum pump, for aspiration
- Centrifuge
- Cell counter
- Ice
- Cryovials
- Cell freezing container
Passaging and culturing PTL
All volumes described below are based on one well of a 6-well plate. Adjust the volumes accordingly if other plate formats are used.
1.Pre-warm PTL-B medium and 1× TrypLE in a 37°C water bath.
2.Aspirate medium from a well containing differentiated PTL day 14 to 20 (see step 20 of Basic Protocol 2).
3.Add 1 ml DPBS and aspirate.
4.Add 0.5 ml 0.48 mM EDTA, gently rock the plate to cover all cells with EDTA, and aspirate.
5.Add 1 ml warm 1× TrypLE, gently rock the plate to cover all cells with TrypLE, and incubate for approximately 5 to 7 min at 36.5°C.
6.Detach the cells from the plate with gentle pipetting.
7.Transfer the detached cells to a tube containing 5 ml freshly prepared DPBS supplemented with 10% FBS.
8.Centrifuge for 5 min at 4°C at 300 × g , room temperature.
9.Carefully aspirate the supernatant without disturbing the cell pellet.
10.Resuspend the cell pellet in 1 ml freshly prepared DPBS supplemented with 20 Kunitz units/ml Dnase I.
11.Leave the cell suspension at room temperature for approximately 3 min.
12.Add 4 ml DPBS to the cell suspension in the tube.
13.Centrifuge for 5 min at 4°C at 300 × g , room temperature.
14.Carefully aspirate the supernatant without disturbing the cell pellet.
15.Resuspend the cell pellet in freshly prepared warm PTL-B medium supplemented with 1 µM GW788388 hydrate, 10 µM Y-27632 dihydrochloride ROCK inhibitor, and 2% FBS.
16.Count the live cells in a cell counter using a 1:1 ratio of cell suspension to 0.4% trypan blue solution.
17.Prepare a stock of cells at a seeding density between 8 to 35 × 104 cells/cm2.
18.From this stock, plate out cells into the desired plate formats.
19.Gently rock the plate to spread the cells equally over the wells.
20.Carefully transfer the plate to the cell culture incubator.
21.Feed the cells the next day with warm PTL-B medium supplemented with 1 µM GW788388 hydrate.
22.Continue to feed the cells every 2 to 3 days with warm PTL-B medium supplemented with 1 µM GW788388 hydrate.
23.The cells are ready for use between day 7 and 15 after replating.
Freezing PTL
24.Pre-cool cryovials in a cold cell freezing container.
25.Pre-cool PTL freezing medium on ice.
26.Pre-warm 1× TrypLE in a 37°C water bath.
27.Follow steps 2 to 14 of passaging and culturing PTL in Basic Protocol 3.
28.Resuspend the cell pellet in ice-cold PTL freezing medium.
29.Divide the cell suspension over the pre-cooled cryovials in the cell freezing container, with 1 ml per vial.
30.Store the cell freezing container containing the cryovials at −80°C overnight.
31.Transfer the cryovials to liquid nitrogen storage the next day for long-term storage.
Thawing PTL
32.Pre-warm PTL-A medium and PTL-B medium in a 37°C water bath.
33.Carefully defrost a cryovial containing passaged PTL in a 37°C water bath until only a small amount of frozen sample remains and proceed quickly to step 34.
34.Transfer the contents of the vial to a tube containing 5 ml warm PTL-A medium.
35.Follow steps 13 to 23 of Passaging and culturing PTL in Basic Protocol 3.
Basic Protocol 4: PTL CULTURING ON TRANSWELLS
This protocol describes the culturing of PTL on transwells, also known as microporous growth supports, or filters. Transwells allow separate treatment conditions of apical (AP) and basolateral (BL) compartments, as well as transepithelial electrical resistance (TEER) measurements. It is recommended to passage or thaw PTL onto transwells. As described in Basic Protocol 3, a coating is not required when replating PTL. When using transwells, coating with human collagen IV is preferred.
PTL have been tested on transwells with pore sizes of 1.0 or 0.4 µm and a polyethylene terephthalate (PET) or polycarbonate (PC) membrane. PET transwells are preferred, as this membrane material makes it easier to view the cells by phase contrast microscopy. Optimal AP and BL volumes for transport studies are 1.5 ml and 2 ml, respectively, for 6-well PET transwells (membrane surface area: 4.5 cm2 and well surface area: 9.6 cm2) (Wilmes et al., 2014), and should be adjusted accordingly when using different transwells. This corresponds to 100 µl AP and 400 µl BL for 24-well PET transwells (membrane surface area: 0.3 cm2 and well surface area: 1.9 cm2).
Materials
- PTL-B medium (see recipe)
- TrypLE™ express enzyme, 1× (Gibco, cat. no. 12604-013)
- Differentiated PTL day 14 to 20 (see step 20 of Basic Protocol 2)
- GW788388 hydrate, 1 mM (see recipe)
- Y-27632 dihydrochloride ROCK inhibitor, 10 mM (see recipe)
- Fetal bovine serum (FBS) (Gibco, cat. no. 10270-106)
- DPBS, no calcium, no magnesium (Gibco, cat. no. 14190-144)
- EDTA, 0.48 mM (Gibco, cat. no. 15040-066)
- Deoxyribonuclease I (DNase I), 10,000 Kunitz units/ml (see recipe)
- Trypan blue solution, 0.4% (Millipore Sigma, cat. no. T8154)
- PTL-A medium (see recipe)
-
- 6-well PET transwells (Millipore, cat. no. MCRP06H48) in 6-well cell culture plate or 24-well PET transwells (Sarstedt, cat. no. 83.3932.101) in 24-well cell culture plate; optional: coated with human collagen IV (see Support Protocol 3)
- Water bath
- Forceps
- Pipet aid
- 10-ml Serological pipets
- 1000-µl Pipette
- 1000-µl Pipette tips
- 1000-µl Pipette tips, with filter
- 50-ml Centrifuge tubes
- Cell culture incubator, set to 36.5°C, 5% CO2, humidified
- Biosafety cabinet
- Inverted microscope
- Vacuum pump, for aspiration
- Centrifuge
- Cell counter
PTL culturing on transwells
The recommended AP/BL volume is 1.5/2 ml for 6-well transwells and 100/400 µl for 24-well transwells. When plating out cells, it is recommended to increase the AP volume from 1.5 to 2 ml for 6-well transwells and from 100 to 200 µl for 24-well transwells to avoid the transwell from drying out overnight, as media will run faster through the transwell until a monolayer is formed.
1.Pre-warm PTL-B medium and 1× TrypLE in a 37°C water bath.
2.Place the required amount of transwells one by one into the cell culture plate by using sterile forceps.
3.Add freshly prepared warm PTL-B medium supplemented with 1 µM GW788388 hydrate, 10 µM Y-27632 dihydrochloride ROCK inhibitor, and 2% FBS to the wells of the plate.
4.Either passage or thaw PTL:
-
For passaging, follow steps 2 to 17 of Passaging and culturing PTL in Basic Protocol3.
-
For thawing, follow steps 32 to 34 of Thawing PTL and then steps 13 to 17 of Passaging and culturing PTL in Basic Protocol3.
5.From this stock, plate out cells into the transwells.
6.Follow steps 20 to 23 of Passaging and culturing PTL in Basic Protocol 3.
Support Protocol 1: PREPARATION OF GELTREX-COATED CELL CULTURE PLATES
This support protocol describes the preparation of Geltrex-coated cell culture plates. The following instructions are based on 2 mg aliquots that have previously been prepared from the stock bottle. It is important to note that the protein concentration of the product may differ from batch-to-batch when obtained from the supplier. Therefore, each lot has to be aliquoted accordingly in order to prepare 2 mg aliquots.
Materials
- DMEM/F-12, HEPES (Gibco, cat. no. 11330032)
- Geltrex™ lactose dehydrogenase elevating virus (LDEV)-free, hESC-qualified, reduced growth factor basement membrane matrix, 2 mg (see recipe)
-
- Ice
- 6-well cell culture plate
- Biosafety cabinet
- Pipet aid
- 10-ml Serological pipets
- 25-ml Serological pipets
- 1000-µl Pipette
- 1000-µl Pipette tips
- 50-ml Centrifuge tubes
- Parafilm® M sealing film
Geltrex coating
All volumes described below are based on four 6-well plates, totaling 24 wells. Adjust the volumes accordingly if other plate formats are used.
1.Pre-cool 25 ml DMEM/F-12 on ice.
2.Thaw a 2 mg Geltrex aliquot on ice.
3.Add the 2 mg Geltrex aliquot to 25 ml ice-cold DMEM/F-12 to reach a concentration of 0.08 mg/ml, mix by inverting the tube.
4.Immediately add 1 ml of the Geltrex solution per well of a 6-well plate (9.6 cm2) to reach a coating concentration of 0.0083 mg/cm2.
5.Gently rock the plate to spread Geltrex over the wells.
6.Incubate for 1 hr at room temperature.
7.Plate is ready for use or can be stored at 4 ° C for up to 14 days. For storage, add an additional 1 ml DMEM/F-12 to each well on top of the coating solution and seal the plate with parafilm to avoid evaporation.
Support Protocol 2: PREPARATION OF RPTEC/TERT1 OR fHDF/TERT166-ECM-COATED CELL CULTURE PLATES
ECM-coated cell culture plates can be prepared by culturing cell lines in plates with subsequent de-cellularization. We tested the cell lines RPTEC/TERT1 and fHDF/TERT166.Both models are immortalized with hTERT and were obtained from Evercyte GmbH (Murphy et al., 2023; Wieser et al., 2008).
RPTEC/TERT1 cells were cultured as previously described (Aschauer et al., 2013), and ECM was harvested 4 days after reaching confluency as previously described (Chandrasekaran et al., 2021). ECM from fHDF/TERT166 cells was harvested immediately after confluency was reached as previously described (Murphy et al., 2023).
Materials
- RPTEC/TERT1 or fHDF/TERT166 (Evercyte GmbH) cells cultured in 6-well cell culture plates, or desired plate format
- Sterile water, high purity
- Ammonia solution, 35 mM (see recipe)
- DPBS, no calcium, no magnesium (Gibco, cat. no. 14190-144)
-
- Biosafety cabinet
- Vacuum pump, for aspiration
- Shaker
- Pipet aid
- 10-ml Serological pipets
- 1000-µl Pipette
- 1000-µl Pipette tips
- Inverted microscope
- Parafilm® M sealing film
RPTEC/TERT1 or fHDF/TERT166-ECM coating
All volumes described below are based on one well of a 6-well plate. Adjust the volumes accordingly if other plate formats are used. RPTEC/TERT1 should be cultured until 4 days after reaching confluency and fHDF/TERT166 cultured until confluent.
1.Aspirate medium from a well containing confluent cells.
2.Add 1 ml sterile water and aspirate.
3.Add 1 ml 35 mM ammonia solution and incubate for 5 min while shaking at 750 rpm.
4.Aspirate ammonia solution.
5.Add 1 ml sterile water and aspirate.
6.Add 1 ml DPBS and aspirate.
7.Repeat step 6 three more times, for a total of four washes.
8.Add 2 ml DPBS.
9.Check the well with an inverted microscope to confirm that all cells detached.
10.Plate is ready for use or can be stored at 4°C for up to 1 month. For storage, seal the plate with parafilm to avoid drying out.
Support Protocol 3: PREPARATION OF HUMAN COLLAGEN IV-COATED CELL CULTURE PLATES
This support protocol describes the preparation of human collagen IV-coated cell culture plates. Before plating out cells onto human collagen IV-coated plates, it is important to wash the coated wells with DPBS at least three times. This step ensures the removal of any residual acetic acid from the coating procedure.
If transwells have been coated with human collagen IV, it is necessary to wash not only the transwells themselves but also the wells in which the transwells are positioned. Furthermore, if a plate with coated transwells is to be stored at 4°C, it is advised to add DPBS to the wells in addition to prevent the transwells from drying out.
Materials
- Collagen IV from human placenta, 1 mg/ml (see recipe)
- Sterile water, high purity
- DPBS, no calcium, no magnesium (Gibco, cat. no. 14190-144)
-
- 6-well cell culture plate
- Pipet aid
- 10-ml Serological pipets
- 1000-µl Pipette
- 200-µl Pipette
- 1000-µl Pipette tips
- 200-µl Pipette tips
- 50-ml Centrifuge tubes
- Cell culture incubator
- Biosafety cabinet
- Vacuum pump, for aspiration
- Parafilm® M sealing film
Human collagen IV coating
All volumes described below are based on one well of a 6-well plate. Adjust the volumes accordingly if other plate formats are used.
1.Thaw a 1 mg/ml collagen IV aliquot at room temperature.
2.Add 48 µl 1 mg/ml collagen IV to 2 ml sterile water to reach a concentration of 24 µg/ml and mix by inverting the tube.
3.Add 2 ml of the collagen IV solution to one well of a 6-well plate (9.6 cm2) to reach a coating concentration of 5 µg/cm2.
4.Gently rock the plate to spread collagen IV over the well.
5.Incubate for 1 hr at 36.5°C.
6.Aspirate the supernatant from the coated well.
7.Add 1 ml DPBS and aspirate.
8.Repeat step 7 one more time, for a total of two washes.
9.Add 2 ml DPBS.
10.Plate is ready for use or can be stored at 4 ° C for up to 14 days. For storage, seal the plate with parafilm to avoid drying out.
Support Protocol 4: IMMUNOFLUORESCENCE STAINING
This support protocol describes the procedure for performing immunofluorescence staining of PTL for the proximal tubular marker megalin to confirm successful differentiation and for the tight junction marker ZO-3 to assess polarization. Before subsequent use of PTL, it is critical to check whether the PTL display a polarized phenotype. Upon full polarization of PTL, ZO-3 staining should be observed exclusively at the tight junctions and not in the cytoplasm or nuclei (Fig. 3, Fig. 5). Nuclear or cytoplasmic ZO-3 staining is observed in non-matured PTL (Chandrasekaran et al., 2021) (Fig. 6). To properly visualize the tight junction staining, it is recommended to use at least a 40× objective or higher. Although PTL grow in a monolayer, they may exhibit a wavy growth pattern. As such, it is recommended to combine several planes into a stack to obtain a clear and in-focus image.
Materials
- PTL cultured on a PhenoPlate 96-well microplate (PerkinElmer, cat. no. 6055302, or equivalent)
- Paraformaldehyde (PFA), 4% (see recipe)
- 1× PBS, diluted from 10× PBS with sterile water (see recipe)
- Blocking buffer, 100% (see recipe)
- ZO-3 (D57G7) XP® rabbit mAb, 1:1600 (Cell Signaling Technology, cat. no. 3704)
- Human megalin/LRP2 mouse antibody, 1:100 (R&D Systems, cat. no. MAB9578)
- Donkey anti-rabbit IgG (H + L) highly cross-adsorbed secondary antibody, Alexa Fluor™ 546, 1:1000 (Invitrogen, cat. no. A10040)
- Donkey anti-mouse IgG (H + L) highly cross-adsorbed secondary antibody, Alexa Fluor™ 488, 1:1000 (Invitrogen, cat. no. A21202)
- Hoechst 33342, 10 mg/ml, 1:10,000 (Invitrogen, cat. no. H3570)
-
- Fume hood
- 10-µl Pipette
- 200-µl Pipette
- 10-µl Pipette tips
- 300-µl Pipette tips, with filter
- 15-ml Centrifuge tubes
- Vacuum pump, for aspiration
- Shaker (optional)
- Parafilm® M sealing film
- Aluminum foil
- Fluorescent microscope
- Imaging software
Cell fixation
All volumes described below are based on one well of a 96-well plate. Adjust the volumes accordingly if other plate formats are used.
1.Fix differentiated PTL day 14 to 20 (see step 20 of Basic Protocol 2) or passaged PTL day 7 to 15 (see step 23 of Basic Protocol 3) with cold 2 to 4% PFA for 30 min in a fume hood. Either aspirate medium and add 50 µl 4% PFA or add 100 µl 4% PFA directly to the well containing 100 µl medium.
2.Remove fixative.
3.Add 100 µl 1× PBS and aspirate.
4.Repeat step 3 one more time, for a total of two washes.
5.Add 100 µl 1× PBS.
Blocking and antibody staining
6.Aspirate 1× PBS.
7.Add 100 µl freshly prepared 100% blocking buffer and incubate for 30 min at room temperature.
8.Prepare primary and secondary antibody solutions in freshly prepared 10% blocking buffer, using the dilutions indicated for each antibody, and add Hoechst 33342 to the secondary antibody solution.
- Prepare 10% blocking buffer by diluting 100% blocking buffer with 1× PBS.
- For the primary antibody solution, add anti-ZO-3 antibody in a dilution of 1:1600 and anti-megalin antibody in a dilution of 1:100 to 10% blocking buffer.
- For the secondary antibody solution, add anti-rabbit-Alexa Fluor 546 and anti-mouse-Alexa Fluor 488, both in a dilution of 1:1000, and Hoechst 33342 in a dilution of 1:10,000, to 10% blocking buffer.
9.Aspirate 100% blocking buffer.
10.Add 50 µl primary antibody solution or 10% blocking buffer (for the control well) and incubate for 1 hr at room temperature.
11.Aspirate primary antibody solution and 10% blocking buffer.
12.Add 100 µl 1× PBS and aspirate.
13.Repeat step 12 one more time, for a total of two washes.
14.Add 50 µl secondary antibody solution and incubate for 35 min at room temperature.
15.Aspirate secondary antibody solution.
16.Add 100 µl 1× PBS and aspirate.
17.Repeat step 16 one more time, for a total of two washes.
18.Add 100 µl 1× PBS.
19.Plate is ready for image acquisition or can be stored at 4°C. For storage, seal the plate with parafilm and wrap in aluminum foil to prevent drying out and to protect from light respectively.
REAGENTS AND SOLUTIONS
Prepare all reagents and solutions using sterile water of high purity such as MilliQ or Barnstead filtered, and autoclaved. Ensure that all cell culture reagents remain sterile by preparing in a biosafety cabinet using aseptic technique. When working with PFA, use a fume hood.
Ammonia solution, 35 mM
- 49.875 ml Sterile water, high purity
- 0.125 ml Ammonia solution, 25% (Supelco, cat. no. 1054321011)
- Store at 4°C.
Blocking buffer, 100%
- 5% Bovine serum albumin (BSA) (Millipore Sigma, cat. no. A7030)
- 1% Triton X-100 (Millipore Sigma, cat. no. T8787)
- 1× PBS (dilute 10× PBS with sterile water)
- Combine ingredients; prepare fresh.
CHIR99021, 5 mM
- 1 mg CHIR99021 (Abcam, cat. no. ab120890)
- 430 µl Dimethyl sulfoxide (DMSO) (Millipore Sigma, cat. no. D8418)
- Reconstitute 1 mg CHIR99021 (465.34 g/mol) in 430 µl DMSO to get a concentration of 5 mM.
- Make aliquots of 20 µl and store at −20°C.
Collagen IV from human placenta, 1 mg/ml
- 2.85 ml Glacial acetic acid (CAS no. 64-19-7)
- 97.15 ml Sterile water, high purity
- 0.2 µm Polyether sulfone (PES) syringe filter, sterile
- 5 mg Collagen IV from human placenta (Millipore Sigma, cat. no. C7521)
- Prepare 0.5 M acetic acid by adding 2.85 ml glacial acetic acid to 97.15 ml sterile water. Mix well and filter sterilize using a sterile PES syringe filter with a pore size of 0.2 µm.
- Reconstitute 5 mg collagen IV in 5 ml 0.5 M acetic acid to get a concentration of 1 mg/ml.
- Incubate for 30 min in a 37°C water bath with occasional mixing.
- Make aliquots and store at −20°C.
Deoxyribonuclease I (DNase I), 10,000 Kunitz units/ml
- 43.83 mg Sodium chloride (NaCl) (CAS no. 7647-14-5)
- 5 ml Sterile water, high purity
- 0.2 µm Polyether sulfone (PES) syringe filter, sterile
- DNase I, 15 KU (Millipore Sigma, cat. no. D5025)
- Prepare 0.15 M NaCl by adding 43.83 mg NaCl to 5 ml sterile water. Mix well and filter sterilize using a sterile PES syringe filter with a pore size of 0.2 µm.
- Reconstitute 15 KU DNase I in 1.5 ml 0.15 M NaCl to get a concentration of 10,000 Kunitz units/ml DNase I.
- Make aliquots and store at −20°C.
Epidermal growth factor (EGF), human recombinant, 10 µg/ml
- 57 µl Glacial acetic acid (CAS no. 64-19-7)
- 100 ml Sterile water, high purity
- 25 mg BSA (Millipore Sigma, cat. no. A9418)
- 200 µg EGF, human recombinant (Millipore Sigma, cat. no. E9644)
- 0.2 µm Polyether sulfone (PES) syringe filters, sterile
- Prepare 10 mM acetic acid by adding 57 µl glacial acetic acid to 100 ml sterile water, mix well and filter sterilize using a sterile PES syringe filter with a pore size of 0.2 µm.
- Prepare 0.1% BSA in 10 mM acetic acid by adding 25 mg BSA to 25 ml 10 mM acetic acid, mix well and filter sterilize using a sterile PES syringe filter with a pore size of 0.2 µm.
- Reconstitute 200 µg EGF in 20 ml 10 mM acetic acid containing 0.1% BSA to get a concentration of 10 µg/ml.
- Make aliquots of 1 ml and store at −20°C. This is a 1000× stock.
FGF-9, human recombinant, 0.1 mg/ml
- 20 µg FGF-9, human recombinant (Gibco, cat. no. PHG0194)
- 200 µl Sterile water, high purity
- Reconstitute 20 µg FGF-9 in 200 µl sterile water to get a concentration of 0.1 mg/ml.
- Make aliquots of 5 µl and store at −20°C, avoid freeze-thaw.
Geltrex™ LDEV-free, hESC-qualified, reduced growth factor basement membrane matrix, 2 mg (Gibco, cat. no. A14133-02)
- Place the 5 ml Geltrex stock on ice and place in the fridge to thaw overnight.
Always keep the stock on ice, it will gel quickly at room temperature!
- Pre-cool a box with sterile 200 µl pipette tips at −20°C.
- Pre-cool sterile 1.5 ml tubes on ice.
- Retrieve the lot specific protein concentration from the ‘Certificate of Analysis’ (between 12 and 18 mg/ml).
Attention: Product has a batch-to-batch variation.
- Calculate aliquot volume (z) needed to prepare 25 ml at 0.08 mg/ml = 2 mg per aliquot.
- z in µl = (25 ml * 0.08 mg/ml)/(stock protein concentration in mg/ml)
- Example: (25 ml * 0.08 mg/ml)/(15.9 mg/ml) = 125.8 µl
- Mix the 5 ml stock by inverting carefully.
Keep the time at room temperature to a minimum.
- Prepare z µl aliquots of Geltrex by pipetting into pre-cooled 1.5 ml tubes using sterile pre-cooled pipette tips.
Use a new cold pipette tip every five aliquots to avoid gelling inside the tip.
- Store at −20°C.
Do not re-freeze an aliquot.
GW788388 hydrate, 1 mM
- 5 mg GW788388 hydrate (Millipore Sigma, cat. no. SML0116)
- 11.75 ml DMSO (Millipore Sigma, cat. no. D8418)
- Reconstitute 5 mg GW788388 hydrate (425.48 g/mol) in 11.75 ml DMSO to get a concentration of 1 mM.
- Make aliquots of 100 µl and store at −20°C.
Hydrocortisone, 36 µg/ml
- 1 mg Hydrocortisone (Millipore Sigma, cat. no. H0135)
- 1 ml Ethanol, absolute (VWR, cat. no. 20821.365 or equivalent)
- 27 ml DPBS, no calcium, no magnesium (Gibco, cat. no. 14190-144)
- Reconstitute 1 mg hydrocortisone in 1 ml Ethanol absolute. Add 27 ml DPBS to get a concentration of 36 µg/ml (total volume: 28 ml).
- Make aliquots of 1 ml and store at −20°C. This is a 1000× stock.
Insulin, human recombinant, 5 mg/ml
- 100 mg Insulin (Gibco, cat. no. A11382II)
- 20 ml Sterile water, high purity
- 1 N Hydrochloric acid (HCl)
- 1 N Sodium hydroxide (NaOH)
- 0.2 µm Polyether sulfone (PES) syringe filter, sterile
- Weigh in 100 mg insulin, add 20 ml sterile water to get a concentration of 5 mg/ml.
- Adjust to pH 3 with 1 N HCl and mix using a magnetic stir plate.
Lower the pH to dissolve the insulin.
- Readjust the pH to 7.1 with 1 N NaOH.
- Filter sterilize using a sterile PES syringe filter with a pore size of 0.2 µm.
- Make aliquots of 1 ml and store at −20°C. This is a 1000× stock.
iPSC freezing medium, 2×
- 60% Embryonic stem-cell tested FBS (Gibco, cat. no. 16141-061)
- 20% Dimethyl sulfoxide (DMSO) (Millipore Sigma, cat. no. D8418)
- 20% mTeSR1 (see recipe)
mTeSR™1 (STEMCELL Technologies, cat. no. 85850)
- The mTeSR™1 Complete Kit comes with 400 ml mTeSR™1 Basal Medium and 100 ml mTeSR™1 5× Supplement.
- Thaw the 100 ml 5× Supplement bottle overnight in the fridge.
- Add 100 ml 5× Supplement carefully to the 400 ml Basal Medium bottle.
- Invert several times to mix.
- Store for up to 2 weeks at 4°C, or prepare aliquots and store at −20°C.
Paraformaldehyde (PFA), 4%
- 200 ml Sterile water, high purity
- 10 g PFA (Millipore Sigma, cat. no. P6148)
- 50 µl 10 N NaOH
- 25 ml 10× PBS (see recipe)
- Pre-heat 200 ml sterile water to 60°C.
- Add 10 g PFA while stirring the warm water.
Attention: PFA fumes are toxic. All work should be done in a fume hood.
- Add 50 µl of 10 N NaOH, stir the solution until it is fully dissolved.
- Add 25 ml of 10× PBS and mix.
- Add sterile water to a final volume of 250 ml.
- Aliquot and freeze at −20°C for long-term storage.
Phosphate-buffered saline (PBS), 10×
- 80 g NaCl (CAS no. 7647-14-5)
- 2 g KCl (CAS no. 7447-40-7)
- 14.4 g Na2HPO4 • 2H2O (CAS no. 10028-24-7)
- 2.4 g KH2PO4 (CAS no. 7778-77-0)
- 800 ml Sterile water, high purity
- 1 N HCl
- Combine ingredients and adjust pH to 7.4 with 1 N HCl.
- Add sterile water to a final volume of 1 L.
- Autoclave and store at room temperature.
PTL-A medium
- 500 ml DMEM, no glucose (Gibco, cat. no. 11966-025)
- 500 ml Ham's F-12 Nutrient Mix (Gibco, cat. no. 21765-029)
- 10 ml 100× GlutaMAX, final concentration 2 mM (Gibco, cat. no. 35050-038)
- 1 ml 5 mg/ml Insulin, final concentration 5 µg/ml (see recipe)
- 1 ml 5 mg/ml Transferrin, final concentration 5 µg/ml (see recipe)
- 1 ml 5 µg/ml Sodium selenite, final concentration 5 ng/ml (see recipe)
- Combine ingredients and store at 4°C.
PTL-B medium
- 1 L PTL-A medium
- 1 ml 10 µg/ml EGF, final concentration 10 ng/ml (see recipe)
- 1 ml 36 µg/ml Hydrocortisone, final concentration 36 ng/ml (see recipe)
- Combine ingredients and store at 4°C.
PTL freezing medium
- 80% PTL-A medium (see recipe)
- 10% FBS (Gibco, cat. no. 10270-106)
- 10% DMSO (Millipore Sigma, cat. no. D8418)
Sodium selenite, 5 µg/ml
- 100 mg Sodium selenite (Millipore Sigma, cat. no. S5261)
- 50 ml Sterile water, high purity
- 0.2 µm Polyether sulfone (PES) syringe filter, sterile
- Prepare a 2 mg/ml pre-stock solution by adding 100 mg sodium selenite to 50 ml sterile water.
- Filter sterilize using a sterile PES syringe filter with a pore size of 0.2 µm.
- Make aliquots of 1 ml and store at −20°C.
- To make the 5 µg/ml stock, add 150 µl of 2 mg/ml sodium selenite to 60 ml sterile water.
- Make aliquots of 1 ml and store at −20°C. This is a 1000× stock.
Transferrin, human recombinant, 5 mg/ml
- 100 mg Transferrin, human recombinant (Millipore Sigma, cat. no. T3705)
- 20 ml DPBS, no calcium, no magnesium (Gibco, cat. no. 14190-144)
- 0.2 µm Polyether sulfone (PES) syringe filter, sterile
- Add 100 mg transferrin to 20 ml DPBS for a concentration of 5 mg/ml. Gently invert the tube to fully dissolve the transferrin.
- Filter sterilize using a sterile PES syringe filter with a pore size of 0.2 µm.
- Make aliquots of 1 ml and store at −20°C. This is a 1000× stock.
TTNPB, 5 mM
- 10 mg TTNPB (Millipore Sigma, cat. no. T3757)
- 1.148 ml DMSO (Millipore Sigma, cat. no. D8418)
- Prepare a 25 mM pre-stock solution by reconstituting 10 mg TTNPB (348.48 g/mol) in 1.148 ml DMSO.
- Make aliquots of 50 µl and freeze at −20°C.
- To prepare a 5 mM stock, add 50 µl of 25 mM TTNPB to 200 µl DMSO.
- Make aliquots of 10 µl and store at −20°C.
Y-27632 dihydrochloride, Rho kinase inhibitor, 10 mM (ROCK inhibitor)
- 10 mg Y-27632 dihydrochloride, Rho kinase inhibitor, 10 mM (ROCK inhibitor) (Abcam, cat. no. ab120129)
- 3 ml Sterile water, high purity
- Reconstitute 10 mg ROCK inhibitor (333.77 g/mol) in 3 ml sterile water for a concentration of 10 mM. Molecular weight depends on batch. Check batch molecular weight in Certificate of Analysis.
- Make aliquots of 50 µl and store at −20°C.
COMMENTARY
Background Information
To date, several protocols have been established to differentiate human iPSC into kidney organoids containing multiple cell phenotypes (Freedman et al., 2015; Morizane et al., 2015; Taguchi et al., 2014; Takasato et al., 2015, 2016). While multicellular 3D cultures are well-suited for certain applications, including developmental studies, they may display specific challenges for toxicity testing and transport studies when compared to single cell type monolayer cell culture systems. (1) It is difficult to control and/or calculate local chemical concentrations in non-perfused multicellular 3D renal organoids. Since chemicals are applied to the external media of the organoid, penetration within the tissue may not be uniform and may decrease towards the center. Thus, target cells may be exposed to much lower concentration of the applied chemical than desired. (2) The side of chemical application (apical or basolateral) is not controlled in 3D renal organoids. In monolayer cell cultures, cells can either be cultured on solid or microporous growth supports and the chemical can be exposed to either the apical or basolateral domain as desired. (3) Mixed population cells may not readily be suitable to down-stream bulk applications, such as western blotting, metabolomics or gene analysis (e.g., transcriptomics). Although single cell analysis can overcome this issue, it is more expensive and time-consuming. Monolayer cultures of target renal cells negate many of these issues, require less time to produce, have greater application flexibility, and higher throughput. On the other hand, the presence of other cell types, as seen in multicellular organoids, will allow cellular communication and may contribute to increased maturity of the individual cell types present.
Only few protocols that derive monocultures of renal proximal tubular cells exist and a major advantage of the protocol described above, compared to other differentiation protocols, is the extended stability of these cells and the possibility to passage them. Hence, with regards to toxicity testing, it is possible to perform repeated-dose toxicity testing for up to 7 days using differentiated PTL cells. Moreover, passaging makes it possible to replate the cells onto desired plate formats, including transwells or organ-on-chip devices, or to freeze them down for long-term storage. Culturing of PTL on transwells allows exposure to compounds applied on either apical or basolateral side. Nevertheless, the iPSC-derived PTL model also displays some limitations. Subculturing is currently limited to one single passage only. Furthermore, PTL may not be fully matured and functional organic anion and cation transport, as well as phase I and II metabolism, have not yet been fully characterized.
Compared to primary human renal proximal tubule cells, iPSC-derived PTL lack the expression of tight junction protein claudin 2 (Aschauer et al., 2013; Chandrasekaran et al., 2021). However, like primary proximal tubular cells, PTL express other tight junction proteins, including ZO-3 and occludin, and can therefore establish a tight and contact-inhibited monolayer (Aschauer et al., 2013; Chandrasekaran et al., 2021; Wieser et al., 2008). Furthermore, PTL display brush border structures with microvilli (Chandrasekaran et al., 2021). Similar to primary proximal tubular cells, PTL express megalin whereas RPTEC/TERT1 cells do not (Chandrasekaran et al., 2021; Hosokawa et al., 2018; Nielsen et al., 2016). Additionally, PTL display functional characteristics of proximal tubule cells, including megalin-mediated uptake of albumin, parathyroid hormone response, and functional ABCB1 transport activity (Chandrasekaran et al., 2021; Wieser et al., 2008).
Critical Parameters
An efficient and successful PTL differentiation starts with high-quality undifferentiated iPSC. Passaging of iPSC at high densities should be avoided, as this may negatively impact cell attachment and growth and may lead to spontaneous differentiation. This is not only critical for routine iPSC culture but also for the PTL differentiation. The starting confluency of iPSC dictates whether the PTL differentiation may be successful. Using iPSC with a confluency above 80% decreases the likeliness of producing high-quality PTL cells. It should also be noted that, in contrast to several other iPSC differentiation protocols, all differentiation factors are added at the time of cell seeding (day 0) and no extra day in mTeSR1 medium for cell attachment is performed.
Another critical parameter is the seeding density of cells on day 0 of the differentiation. If cells are seeded at too low of a density, confluency on day 3 may not be achieved. If cells are seeded at too high of a density, cells will already reach confluency by day 2 and FGF-9 treatment may lead to holes in the monolayer that will most likely not recover by day 8. With a correct seeding density, confluency should be reached on day 3 as shown in Figure 2. It is important to follow the precise timing of applying the growth factors and small molecules during the initial 6 days of the differentiation.
An essential parameter for passaging PTL is the inclusion of the TGF-beta type I receptor inhibitor GW788388 hydrate in the cell culture medium at all times, as in its absence passaged PTL will de-differentiate and lose their polarization (Chandrasekaran et al., 2021). The differentiation and maturation status of both unpassaged and passaged PTL should be checked before usage in experiments via immunofluorescence staining of the proximal tubular-enriched marker megalin to confirm a successful PTL differentiation, and the tight junction marker ZO-3 to confirm polarization. ZO-3 localization at the tight junctions, rather than in the cytoplasm or nuclei, is absolutely critical. It is not advised to use non-polarized PTL in subsequent assays.
Troubleshooting
An overview of common problems, their possible causes, and recommended solutions regarding iPSC culture, PTL differentiation, and PTL passaging is listed in Table 1.
Problem | Possible cause | Solution |
---|---|---|
iPSC culture | ||
Poor cell attachment after thawing. | ROCK inhibitor was omitted or cells were not frozen properly. | Thaw high-quality iPSC using ROCK inhibitor for a maximum of 24 hr. |
Poor results after passaging, e.g., poor cell growth, poor cell attachment, or high number of dead cells. | Cells were too confluent. | Passage cells at 60-70% confluency. |
Cells were pipetted too much. | Do not pipet the cells up and down too much; use gentle pipetting. Passage them in small cell colonies using EDTA. | |
Cells were not fed daily. | Feed daily, except for 1 day after passaging. | |
mTeSR1 or Geltrex-coated plates were not prepared or stored properly. | Prepare fresh mTeSR1 medium. Prepare fresh Geltrex-coated plates, do not let them dry out. | |
PTL differentiation | ||
Cells are not confluent at the end of day 3. | iPSC were too confluent or stressed prior to differentiation. | If the cell monolayer displays large holes, discard culture and start again. Use iPSC at 60-70% confluency. If the cell monolayer displays small holes, monitor cells closely. If cells do not form a confluent monolayer by day 8, discard culture and start again. |
Non-optimal seeding density on day 0. Optimal seeding density may vary between different iPSC lines. | Adjust seeding density if needed. | |
Feeding intervals during differentiation were not correct. | Follow the feeding intervals strictly. | |
Cell monolayer displays holes on day 6. | This may happen in response to FGF-9 treatment, or iPSC were too confluent or stressed prior to differentiation. | If remaining cells re-grow to form a confluent monolayer by day 8, continue as normal. If cells do not form a confluent monolayer by day 8, discard culture and start again. Use iPSC at 60-70% confluency. |
Non-optimal seeding density on day 0. Optimal seeding density may vary between different iPSC lines. | Adjust seeding density if needed. | |
Feeding intervals during differentiation were not correct. | Follow the feeding intervals strictly. | |
Cells do not stain for megalin on day 14. | Cells are not PTL. Differentiation protocol was not followed correctly. | Repeat the differentiation and follow protocol precisely. |
Immunofluorescence staining protocol was not performed correctly. | Repeat staining. | |
Cells are not polarized on day 14. | PTL are not in a confluent monolayer. | It is not advised to use non-polarized PTL for any experimental work. Discard culture and start again. |
iPSC were too confluent or stressed prior to differentiation. | Use iPSC at 60-70% confluency. | |
Non-optimal seeding density on day 0. Optimal seeding density may vary between different iPSC lines. | Adjust seeding density if needed. | |
Feeding intervals were not correct. | Follow the feeding intervals strictly. | |
PTL passaging | ||
PTL do not survive after passaging onto collagen IV-coated plate. | Plate was not washed; remaining traces of acetic acid are toxic to PTL. | Wash collagen IV-coated plate at least three times before seeding cells. |
PTL are not confluent by day 3. | Non-optimal seeding density was used to plate out cells after passaging. Note that seeding densities may vary between donors. | Discard cells and repeat the passaging protocol with a higher seeding density. |
PTL dedifferentiate and are non-polarized after passaging. | GW788388 hydrate was omitted from medium. | After passaging, add GW788388 hydrate at all times to the medium. |
PTL detached after passaging. | This may happen especially with smaller plate formats. | Repeat the passaging protocol with ECM or collagen IV-coated plates. |
PTL on transwells | ||
Transwell dried out overnight after seeding cells. | Cells did not form a monolayer yet, so more liquid passed through the transwell. | Increase the volume when seeding cells in the transwell. |
Cells are growing in the cell culture well below the transwell. | Feeding was not done carefully, and cells were transferred from the transwell to the cell culture well. | Carefully feed the transwell and the bottom well one after the other, make sure to change tips and use different medium aliquots. |
Transwell is broken. | Inspect integrity of the transwell and discard broken transwell. |
Understanding Results
To confirm a successful PTL differentiation, immunofluorescence staining of PTL for proximal tubular-specific markers as well as markers for tight junctions to confirm polarization should be performed. For proper assessment of the tight junction staining, the use of at least a 40× objective, or higher, is recommended. Since PTL may display a wavy growth-pattern, it is recommended to analyze several planes. For better visibility, several planes may be combined into a stack. Typical planes that we have observed include approximately 8 to 16 µm and approximately 12 to 26 µm for unpassaged and passaged PTL, respectively. Figures 3 and 5 show representative immunofluorescent images of the proximal tubular-specific marker megalin (green) and tight junction marker ZO-3 (orange) in unpassaged and passaged PTL, respectively. Note that the localization of ZO-3 is exclusively in the tight junctions in fully differentiated PTL whereas a cytoplasmic and/or nuclear staining of ZO-3 is observed in non-matured PTL (Chandrasekaran et al., 2021), as shown in Figure 6. Nuclear staining of ZO-3 may also appear in proliferating cells, which was previously shown in RPTEC/TERT1 cells (Aschauer et al., 2013). In subsequent assays, PTL should only be used in a fully differentiated state.
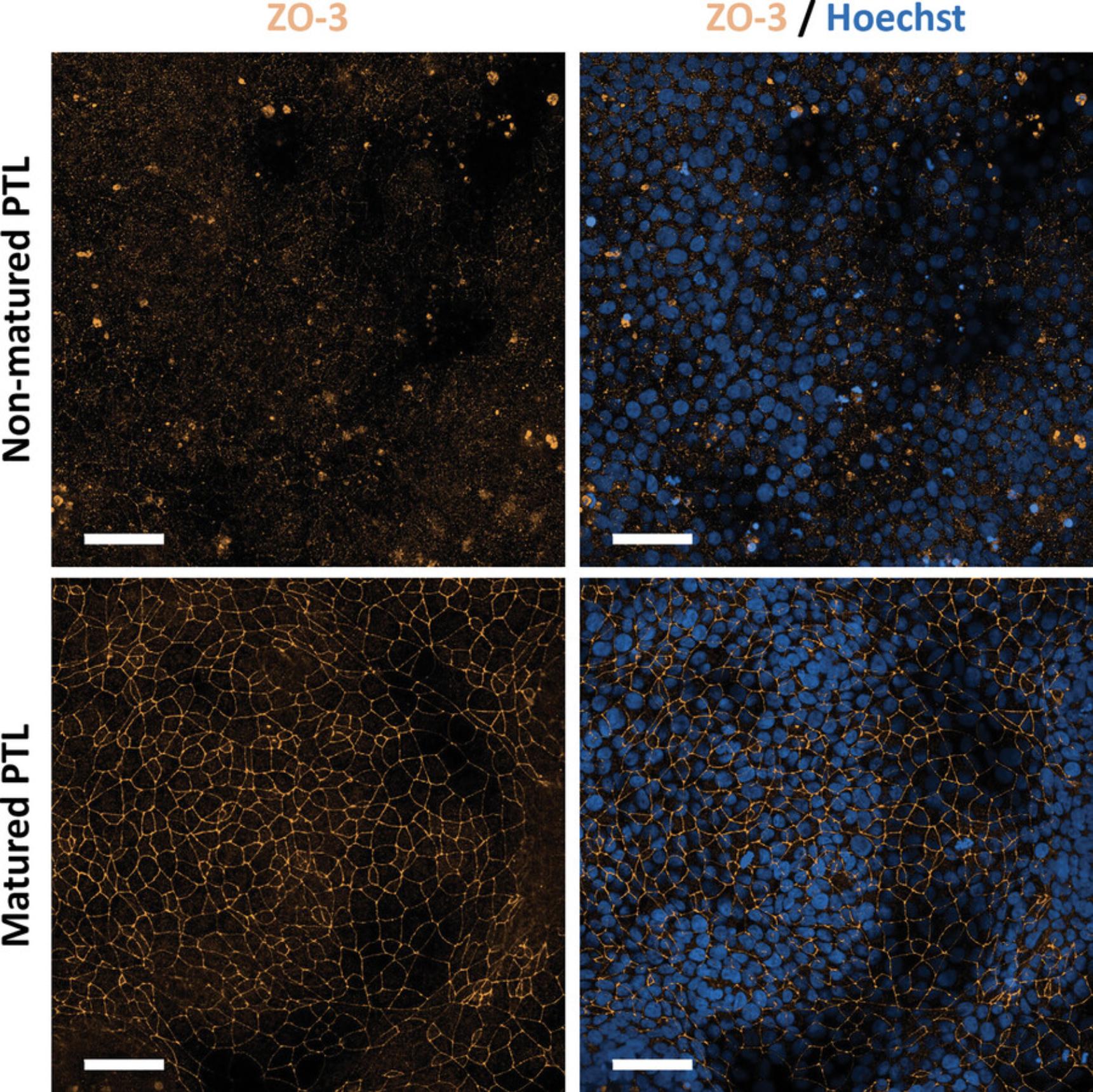
Time Considerations
iPSC should be passaged every 3 to 4 days. The PTL differentiation protocol takes a minimum of 14 days. Differentiated PTL cells can be used from day 14 until day 20 of the PTL differentiation. After passaging or thawing PTL, it takes a minimum of 7 days for cells to mature again after proliferation. Passaged PTL should therefore be used from day 7 until day 15 after replating. Preparation of Geltrex-coated or human collagen IV-coated plates takes approximately 1 hr 30 min in total, which includes a 1 hr incubation step before the coated plates are ready for use. Preparation of RPTEC/TERT1 or fHDF/TERT166-ECM-coated cell culture plates takes approximately 45 to 60 min. However, this excludes the culturing of the cell lines until confluency is reached which also needs to be considered. Immunofluorescence staining will take approximately 3 hr in total, which includes several long incubation steps. However, this excludes image acquisition which may vary depending on user experience.
Author Contributions
Tamara Meijer : Data curation, writing—original draft, writing—review and editing; Elisabeth Naderlinger : Data curation, writing—original draft, writing—review and editing; Paul Jennings : Methodology, writing—review and editing, Anja Wilmes : Conceptualization, methodology, supervision, writing—original draft, writing—review and editing.
Conflict of Interest
The authors declare no conflict of interest.
Open Research
Data Availability Statement
Data is available on reasonable request from the authors.
Literature Cited
- Aschauer, L., Gruber, L. N., Pfaller, W., Limonciel, A., Athersuch, T. J., Cavill, R., Khan, A., Gstraunthaler, G., Grillari, J., Grillari, R., Hewitt, P., Leonard, M. O., Wilmes, A., & Jennings, P. (2013). Delineation of the key aspects in the regulation of epithelial monolayer formation. Molecular and Cellular Biology , 33(13), 2535–2550. https://doi.org/10.1128/MCB.01435-12
- Awdishu, L., & Mehta, R. L. (2017). The 6R's of drug induced nephrotoxicity. BMC Nephrology , 18(1), 124. https://doi.org/10.1186/s12882-017-0536-3
- Chandrasekaran, V., Carta, G., da Costa Pereira, D., Gupta, R., Murphy, C., Feifel, E., Kern, G., Lechner, J., Cavallo, A. L., Gupta, S., Caiment, F., Kleinjans, J. C. S., Gstraunthaler, G., Jennings, P., & Wilmes, A. (2021). Generation and characterization of iPSC-derived renal proximal tubule-like cells with extended stability. Scientific Reports , 11(1), 11575. https://doi.org/10.1038/s41598-021-89550-4
- El Nahas, A. M., & Bello, A. K. (2005). Chronic kidney disease: The global challenge. Lancet , 365(9456), 331–340. https://doi.org/10.1016/S0140-6736(05)17789-7
- Freedman, B. S., Brooks, C. R., Lam, A. Q., Fu, H., Morizane, R., Agrawal, V., Saad, A. F., Li, M. K., Hughes, M. R., Werff, R. V., Peters, D. T., Lu, J., Baccei, A., Siedlecki, A. M., Valerius, M. T., Musunuru, K., McNagny, K. M., Steinman, T. I., Zhou, J., … Bonventre, J. V. (2015). Modelling kidney disease with CRISPR-mutant kidney organoids derived from human pluripotent epiblast spheroids. Nature Communications , 6, 8715. https://doi.org/10.1038/ncomms9715
- Hosokawa, S., Hosokawa, K., Ishiyama, G., Ishiyama, A., & Lopez, I. A. (2018). Immunohistochemical localization of megalin and cubilin in the human inner ear. Brain Research , 1701, 153–160. https://doi.org/10.1016/j.brainres.2018.09.016
- Jennings, P. (2015). The future of in vitro toxicology. Toxicology in Vitro , 29(6), 1217–1221. https://doi.org/10.1016/j.tiv.2014.08.011
- Jennings, P., Aschauer, L., Wilmes, A., & Gstraunthaler, G. (2014). Renal cell culture. A. Bal-Price & P. Jennings (Eds.), In Vitro Toxicology Systems (pp. 79–101). Humana Press. https://doi.org/10.1007/978-1-4939-0521-8
- Jennings, P., Carta, G., Singh, P., da Costa Pereira, D., Feher, A., Dinnyes, A., Exner, T. E., & Wilmes, A. (2022). Capturing time-dependent activation of genes and stress-response pathways using transcriptomics in iPSC-derived renal proximal tubule cells. Cell Biology and Toxicology , https://doi.org/10.1007/s10565-022-09783-5
- Limonciel, A., Wilmes, A., Aschauer, L., Radford, R., Bloch, K. M., McMorrow, T., Pfaller, W., van Delft, J. H., Slattery, C., Ryan, M. P., Lock, E. A., & Jennings, P. (2012). Oxidative stress induced by potassium bromate exposure results in altered tight junction protein expression in renal proximal tubule cells. Archives of Toxicology , 86(11), 1741–1751. https://doi.org/10.1007/s00204-012-0897-0
- Morizane, R., Lam, A. Q., Freedman, B. S., Kishi, S., Valerius, M. T., & Bonventre, J. V. (2015). Nephron organoids derived from human pluripotent stem cells model kidney development and injury. Nature Biotechnology , 33(11), 1193–1200. https://doi.org/10.1038/nbt.3392
- Morrison, M., Klein, C., Clemann, N., Collier, D. A., Hardy, J., Heisserer, B., Cader, M. Z., Graf, M., & Kaye, J. (2015). StemBANCC: Governing access to material and data in a large stem cell research consortium. Stem Cell Reviews and Reports , 11(5), 681–687. https://doi.org/10.1007/s12015-015-9599-3
- Murphy, C., Naderlinger, E., Mater, A., Kluin, R. J. C., & Wilmes, A. (2023). Comparison of human recombinant protein coatings and fibroblast-ECM to Matrigel for induced pluripotent stem cell culture and renal podocyte differentiation. ALTEX - Alternatives to Animal Experimentation , 40(1), 141–159. https://doi.org/10.14573/altex.2112204
- Nielsen, R., Christensen, E. I., & Birn, H. (2016). Megalin and cubilin in proximal tubule protein reabsorption: From experimental models to human disease. Kidney International , 89(1), 58–67. https://doi.org/10.1016/j.kint.2015.11.007
- Nunes, C., Singh, P., Mazidi, Z., Murphy, C., Bourguignon, A., Wellens, S., Chandrasekaran, V., Ghosh, S., Zana, M., Pamies, D., Thomas, A., Verfaillie, C., Culot, M., Dinnyes, A., Hardy, B., Wilmes, A., Jennings, P., Grillari, R., Grillari, J., … Exner, T. (2022). An in vitro strategy using multiple human induced pluripotent stem cell-derived models to assess the toxicity of chemicals: A case study on paraquat. Toxicology in Vitro , 81, 105333. https://doi.org/10.1016/j.tiv.2022.105333
- Pamies, D., Bal-Price, A., Simeonov, A., Tagle, D., Allen, D., Gerhold, D., Yin, D., Pistollato, F., Inutsuka, T., Sullivan, K., Stacey, G., Salem, H., Leist, M., Daneshian, M., Vemuri, M. C., McFarland, R., Coecke, S., Fitzpatrick, S. C., Lakshmipathy, U., … Hartung, T. (2017). Good Cell Culture Practice for stem cells and stem-cell-derived models. ALTEX - Alternatives to Animal Experimentation , 34(1), 95–132. https://doi.org/10.14573/altex.1607121
- Pamies, D., Leist, M., Coecke, S., Bowe, G., Allen, D. G., Gstraunthaler, G., Bal-Price, A., Pistollato, F., de Vries, R. B. M., Hogberg, H. T., Hartung, T., & Stacey, G. (2022). Guidance document on Good Cell and Tissue Culture Practice 2.0 (GCCP 2.0). ALTEX - Alternatives to Animal Experimentation , 39, 30–70. https://doi.org/10.14573/altex.2111011
- Rauch, C., Jennings, P., & Wilmes, A. (2014). Use of induced pluripotent stem cells in drug toxicity screening. A. Bal-Price & P. Jennings (Eds.), In Vitro Toxicology Systems (pp. 335–350). Humana Press. https://doi.org/10.1007/978-1-4939-0521-8
- Singh, P., Chandrasekaran, V., Hardy, B., Wilmes, A., Jennings, P., & Exner, T. E. (2021). Temporal transcriptomic alterations of cadmium exposed human iPSC-derived renal proximal tubule-like cells. Toxicology in Vitro , 76, 105229. https://doi.org/10.1016/j.tiv.2021.105229
- Snijders, K. E., Fehér, A., Táncos, Z., Bock, I., Téglási, A., van den Berk, L., Niemeijer, M., Bouwman, P., le Dévédec, S. E., Moné, M. J., van Rossom, R., Kumar, M., Wilmes, A., Jennings, P., Verfaillie, C. M., Kobolák, J., Ter Braak, B., Dinnyés, A., & van Water, B. (2021). Fluorescent tagging of endogenous Heme oxygenase-1 in human induced pluripotent stem cells for high content imaging of oxidative stress in various differentiated lineages. Archives of Toxicology , 95(10), 3285–3302. https://doi.org/10.1007/s00204-021-03127-8
- Taguchi, A., Kaku, Y., Ohmori, T., Sharmin, S., Ogawa, M., Sasaki, H., & Nishinakamura, R. (2014). Redefining the in vivo origin of metanephric nephron progenitors enables generation of complex kidney structures from pluripotent stem cells. Cell Stem Cell , 14(1), 53–67. https://doi.org/10.1016/j.stem.2013.11.010
- Takahashi, K., & Yamanaka, S. (2006). Induction of pluripotent stem cells from mouse embryonic and adult fibroblast cultures by defined factors. Cell , 126(4), 663–676. https://doi.org/10.1016/j.cell.2006.07.024
- Takasato, M., Er, P. X., Chiu, H. S., & Little, M. H. (2016). Generation of kidney organoids from human pluripotent stem cells. Nature Protocols , 11(9), 1681–1692. https://doi.org/10.1038/nprot.2016.098
- Takasato, M., Er, P. X., Chiu, H. S., Maier, B., Baillie, G. J., Ferguson, C., Parton, R. G., Wolvetang, E. J., Roost, M. S., de Sousa Lopes, S. M. C., & Little, M. H. (2015). Kidney organoids from human iPS cells contain multiple lineages and model human nephrogenesis. Nature , 526(7574), 564–568. https://doi.org/10.1038/nature15695
- Wieser, M., Stadler, G., Jennings, P., Streubel, B., Pfaller, W., Ambros, P., Riedl, C., Katinger, H., Grillari, J., & Grillari-Voglauer, R. (2008). hTERT alone immortalizes epithelial cells of renal proximal tubules without changing their functional characteristics. American Journal of Physiology-Renal Physiology , 295(5), F1365–F1375. https://doi.org/10.1152/ajprenal.90405.2008
- Wilmes, A., Aschauer, L., Limonciel, A., Pfaller, W., & Jennings, P. (2014). Evidence for a role of claudin 2 as a proximal tubular stress responsive paracellular water channel. Toxicology and Applied Pharmacology , 279(2), 163–172. https://doi.org/10.1016/j.taap.2014.05.013
- Wilmes, A., Bielow, C., Ranninger, C., Bellwon, P., Aschauer, L., Limonciel, A., Chassaigne, H., Kristl, T., Aiche, S., Huber, C. G., Guillou, C., Hewitt, P., Leonard, M. O., Dekant, W., Bois, F., & Jennings, P. (2015). Mechanism of cisplatin proximal tubule toxicity revealed by integrating transcriptomics, proteomics, metabolomics and biokinetics. Toxicology in Vitro , 30(1), 117–127. https://doi.org/10.1016/j.tiv.2014.10.006
- Wilmes, A., Rauch, C., Carta, G., Kern, G., Meier, F., Posch, W., Wilflingseder, D., Armstrong, L., Lako, M., Beilmann, M., Gstraunthaler, G., & Jennings, P. (2017). Towards optimisation of induced pluripotent cell culture: Extracellular acidification results in growth arrest of iPSC prior to nutrient exhaustion. Toxicology in Vitro , 45(3), 445–454. https://doi.org/10.1016/j.tiv.2017.07.023