Determining On-Target, Off-Target, and Copy Number Status of Transgenic Events After CRISPR/Cas9 Targeted AAVS1 Safe-Harbor Modification of iPSCs Using Double-Control Quantitative Copy Number PCR
Brit-Maren Michaud Schjeide, Brit-Maren Michaud Schjeide, Gerhard Paul Püschel, Gerhard Paul Püschel
Abstract
Double-control quantitative copy number PCR (dc-qcnPCR) is a recently described tool that can be used to quantify donor DNA insertions in genetically modified monoclonal cell lines. In conjunction with an insert-confirmation PCR, the technique can quickly and easily identify clones containing on-target heterozygous or homozygous donor DNA integrations and exclude off-target insertions. The genetic manipulation of immortal cell lines is a versatile tool to elucidate cellular signaling pathways and protein functions. Despite recent advances in the precision of genetic engineering tools such as CRISPR/Cas9, transcription-activator-like effector nucleases (TALENs), and zinc-finger nucleases (ZFNs), it is still essential to verify the accurate insertion of the sequence of interest (donor DNA) into the targeted genomic DNA (gDNA) locus. This precise integration into a genetic safe harbor, and exclusion of the donor DNA from functionally relevant genes, can ensure normal cellular functionality. Current methods to analyze the specificity of donor DNA insertions either are cost-prohibitive or create dependency on manufacturers for assay design and production. The dc-qcnPCR method is a simple, yet powerful, approach that can be prepared and carried out in any laboratory equipped with standard molecular biology supplies. Here we provide step-by-step instructions to prepare and perform the dc-qcnPCR, and its companion insert-confirmation PCR, to determine donor DNA insertion numbers in monoclonal cell lines genetically modified through CRISPR/Cas9. © 2023 The Authors. Current Protocols published by Wiley Periodicals LLC.
Basic Protocol 1 : Genetic modification at AAVS1 safe harbor in induced pluripotent stem cells (IMR90-4) using CRISPR/Cas9: from plasmid design to monoclonal expansion
Support Protocol 1 : Measurement of Gaussia luciferase activity to verify reporter protein functionality
Support Protocol 2 : Verification of monoclonal expansion using immunofluorescence.
Basic Protocol 2 : Insert-confirmation PCR
Basic Protocol 3 : Design and preparation of double-control quantitative copy number PCR reagents and quantification of donor DNA integrations in genetically modified monoclonal cells
INTRODUCTION
The genetic manipulation of immortal cell lines has been used for decades to advance knowledge surrounding cellular signaling pathways and protein function. In the early 1980s, it was first suggested that the stable introduction of foreign DNA into target genomes could be assisted by homology-directed recombination (HDR). This observation was exploited to insert donor DNA segments into specific genomic loci (Folger, Wong, Wahl, & Capecchi, 1982). Genetic engineering technologies have since progressed in leaps and bounds, expanding in choice and precision. Tools such as zinc-finger nucleases (ZFNs), transcription-activator-like effector nucleases (TALENs), and most recently CRISPR/Cas9 now open doors to the precise site-directed mutagenesis of genomic DNA (Bhardwaj & Nain, 2021; Lanigan, Kopera, & Saunders, 2020; Stoddard, 2014; Waddington, Privolizzi, Karda, & O'Neill, 2016). Despite the improvements in the precision of these techniques, it is still essential to verify the accurate insertion of the sequence of interest (donor DNA/transgene) into the targeted genomic DNA (gDNA) locus in order to exclude possible disturbances in cellular functionality caused by off-target integrations in functionally relevant genes or uncontrolled overexpression of the transgene due to multiple random genomic insertions.
Current methods to analyze the efficiency and accuracy of genetically modified cells either are expensive (as with long-read whole-genome sequencing) or create dependency on manufacturers for dual-probe assay design and production, which limits the flexibility of smaller laboratories in verifying the results of their genetic engineering experiments (see discussion in Background Information). Double-control quantitative copy number PCR (dc-qcnPCR) is a simple procedure that can be prepared and carried out in any laboratory equipped with standard molecular biology equipment. The tool is used to identify on-target heterozygous or homozygous donor DNA insertions in genetically modified monoclonal cell lines. The tool takes advantage of the precision of qPCR and compares the amplification of the target donor DNA against that of autosomal and X-chromosome (ChrX) genes. The autosomal gene defines the copy number of 2, while the ChrX gene can be used to quickly verify the credibility of the experiment, by calculating the expected copy number ratio of female to male gDNA as 2:1. All reactions are compared to one using a control plasmid that contains sequences for all genes of interest and serves as a control for both differences in primer efficiency and any possible pipetting error across samples. In conjunction with an insert-confirmation PCR, the dc-qcnPCR can be utilized to quickly identify clones containing on-target heterozygous or homozygous donor DNA integrations. The determination of heterozygous or homozygous on-target insertion using the insert-confirmation PCR is also essential to exclude clones containing off-target donor DNA integrations: If the dc-qcnPCR results in a copy number greater than what is visualized on the agarose gel, those extra copies are classified as off-target insertions. We provide step-by-step instructions to illustrate this tool in the form of three basic protocols and two support protocols.
In Basic Protocol 1, we describe how to carry out a CRISPR/Cas9 double transfection method to knock in a sequence encoding the Gaussia princeps luciferase (GLuc) reporter protein, whose expression is steered by the Ef1a-HTLV promoter, in the AAVS1 safe harbor of IMR90-4 induced pluripotent stem cells (iPSC). This protocol serves as an example of how to transfect cells using CRISPR/Cas9. However, the dc-qcnPCR can be utilized to discriminate between on- and off-target integrations in any cell type, at any known target, and by any recombination method. In Support Protocol 1, we describe how to verify that the stably transfected cells are expressing GLuc by measuring luciferase activity. In Support Protocol 2, we describe how to verify successful monoclonal expansion using immunofluorescence. Once the luciferase expression and the expansion of monoclonal cell lines have been successfully confirmed, it is possible to move onto the final two basic protocols. In Basic Protocol 2, we describe how to plan and carry out the insert-confirmation PCR, which is necessary to determine if the donor DNA has been integrated into one or both alleles at the AAVS2 safe-harbor locus. Finally, in Basic Protocol 3, we describe how to prepare, plan, and implement the dc-qcnPCR to analyze the monoclonal cell lines isolated during Basic Protocol 1. The results of Basic Protocols 2 and 3 are combined to determine whether the clone of interest contains heterozygous or homozygous on-target transgene integrations and/or off-target transgene integrations. Figure 1 provides a visual summary of the protocol workflow.
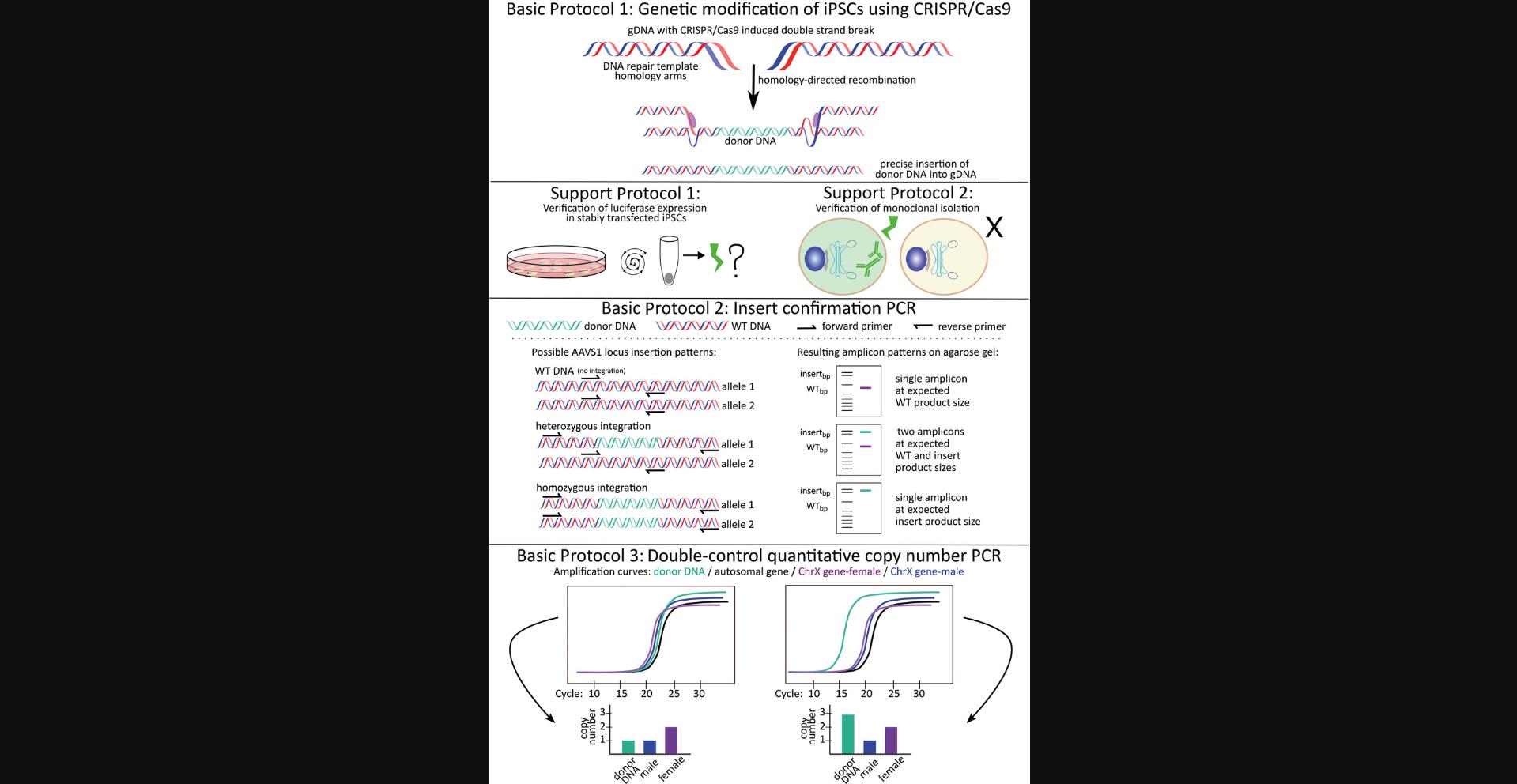
Basic Protocol 1: GENETIC MODIFICATION AT AAVS1 SAFE HARBOR IN INDUCED PLURIPOTENT STEM CELLS (IMR90-4) USING CRISPR/CAS9: FROM PLASMID DESIGN TO MONOCLONAL EXPANSION
Basic Protocol 1 describes the maintenance and transfection of IMR90-4 induced pluripotent stem cells (Yu et al., 2007). The iPSCs are genetically modified at the AAVS1 safe-harbor locus using a double-plasmid CRISPR/Cas9 transfection method. The backbone that encodes the Cas9 endonuclease is the enhanced-specificity Cas9 (eSpCas9(1.1)) plasmid, which was a gift from Feng Zhang (Slaymaker et al., 2016). The sequence of the AAVS1 safe-harbor locus T2 single guide RNA (sgRNA) site (Oceguera-Yanez et al., 2016) must be cloned into the eSpCas9(1.1) vector. The second plasmid backbone used in this protocol is pAAVS1-P-MCS, which contains sequences for the AAVS1 safe-harbor locus homology arms and the puromycin resistance gene. pAAVS1-P-MCS was a gift from Knut Woltjen (Oceguera-Yanez et al., 2016). The Ef1a-HTLV promoter and the coding sequence of the Gaussia luciferase reporter protein are cloned into the pAAVS1-P-MCS vector. These two elements are referred to as the “donor DNA” throughout the following protocols. Lipofectamine 3000 reagent, which has been specially designed to more efficiently transfect difficult cell lines, is used for the transfection. Stably transfected clones are selected with puromycin antibiotic. Once the selection is done, stably transfected monoclonal cell lines are expanded by performing single-cell dilutions in 96-well plates. An overview is provided in Figure 2 (numbers in the subheadings below refer to those in the figure).
<img src="https://static.yanyin.tech/literature_test/cpz1635-fig-0002-m.jpg" alt="Schematic of Basic Protocol 1 and Support Protocols 1 and 2. (A) Plasmid mapping of the eSpCas9(1.1)-AAVS1-T2 and pAAVS1-P-MCS no tag GLuc vectors. Summary of the three major experimental sections of Basic Protocol 1. First, prepare the plasmids necessary for the CRISPR/Cas9 double transfection. Part 1: Anneal the oligonucleotides encoding the gRNA and clone into the eSpCas9(1.1) backbone. The resulting vector, known as "eSpCas9(1.1) AAVS1-T2,” is used for the expression of Cas9 and its guide to the AAVS1 safe-harbor locus. Part 2a: Clone donor DNA in between homology arms corresponding to AAVS1 safe-harbor locus. PCR amplify the Ef1-HTLV promoter and clone into the pAAVS1-P-MCS backbone. The resulting vector is called “pAAVS1-P-MCS_Ef1-HTLV”. Part 2b: PCR amplify the coding sequence of Gaussia luciferase (Gluc) and clone into the pAAVS1-P-MCS_Ef1-HTLV” vector. The resulting vector, known as “pAAVS1-P-MCS no tag Gluc,” is used as a template for homology directed repair at the AAVS1 safe harbor. Part 3: Carry out a double plasmid transfection using the eSpCas9(1.1)-AAVS1-T2 and pAAVS1-P-MCS no tag GLuc vectors. Use selection medium to isolate clones containing a stable integration the donor DNA into their genomic DNA. Immediately carry out Support Protocols 1 and 2 in order to verify GLuc expression and to verify successful monoclonal isolation of the stably transfected cells. (B) Panel B': Simplified plasmid map of eSpCas9(1.1) vector including the AAVS1-T2 sgRNA sequence. Features included: "AmpR promoter" and "AmpR" provide ampicillin resistance for bacterial selection, the "AAVS1-T2 gRNA" is the sequence cloned into the original eSpCas9(1.1) vector during Basic Protocol 1, "gRNA scaffold" encodes the gRNA hairpin structure, "CMV enhancer" plus "chicken beta-actin promoter" drive the expression of the eSpCas9(1.1) endonuclease. Panel B'': Simplified plasmid map of pAAVS1-P-MCS no tag GLuc vector. Features included: "AmpR promoter" and "AmpR" provide ampicillin resistance for bacterial selection, the "HA-L" is the homology-arm corresponding to the left side of the AAVS1 locus, the "HA-R" is the homology-arm corresponding to the right side of the AAVS1 locus. "PuroR" confers puromycin resistance for the selection of stably transfected cells. The "Ef1-HTLV" promoter and "GLuc" coding sequence are features cloned into the vector during Basic Protocol 1. Relevant restriction enzyme sites are included (SatI, PacI, SalI, SpeI). HA, homology arm; L, left; R, right; PuroR, puromycin resistance; AmpR, ampicillin resistance. Figure created with Inkscape and SnapGene." loading="lazy" title="Details are in the caption following the image"/>
Basic Protocol 1 produces the transfected cells necessary to describe the dc-qcnPCR. The dc-qcnPCR genomic analysis tool can be implemented in any cell line and many types of targeted genetic manipulation. Note that it may be necessary to optimize this protocol depending on the needs and goals of your project (e.g., to use other cell types, genetic modification tools, modification targets, etc.).
NOTE : Care should be taken to work in a sterile environment. This protocol falls under Biosafety Level 1.
Materials
- Laminar flow hood, class 2, for microbiology (Mars Scanlaf, 9001020999)
- eSpCas9(1.1) (Addgene plasmid cat. no. 71814; http://n2t.net/addgene:71814; RRID Addgene_71814), supplied as bacterial stab in agar
- Luria Broth (LB) agar (see recipe)
- Ampicillin (Gibco, 11593027)
- LB medium (see recipe)
- GeneJET Plasmid Maxiprep, Midiprep, or Miniprep Kits (Thermo Fisher Scientific, K0492, K0481, and K0503)
- Glycerol (Thermo Fisher Scientific, J61059.K2)
- FastDigest restriction enzymes (Thermo Fisher Scientific, various) and buffers
- FastAP Thermosensitive Alkaline Phosphatase (1 U/µl; Thermo Fisher Scientific, EF0651)
- 10× TAE solution (see recipe)
- Agarose NEEO Ultra-Quality (Carl Roth, 2267.4)
- Ethidium bromide (Thermo Fisher Scientific, 15585011)
- MassRuler DNA ready-to-use DNA ladder mix (Thermo Fisher Scientific, SM0403) or equivalent
- Agarose-out DNA Purification Kit (Roboklon, E3540) or equivalent
- Oligonucleotide sequences for preparation of gRNA segment (Table 1; Biolegio or similar)
- T4 polynucleotide ligase (Thermo Fisher Scientific, EK0031) with buffer
- T4 DNA ligase (5 U/µl; Thermo Fisher Scientific, EL0011) with buffer
- SOC medium (see recipe)
- XL1-Blue electroporation-competent Escherichia coli cells (Agilent, 200228)
- 2× Maxima SYBR Green/ROX qPCR Master Mix (Thermo Fisher Scientific, K0222)
- PCR primers for gRNA ligation confirmation (Table 2; Biolegio)
- Phusion™ High-Fidelity DNA Polymerase (2 U/µl) Kit (Thermo Fisher Scientific, F530S; high-fidelity, proof-reading enzyme, which prepares blunt-end PCR products, McInerney, Adams, & Hadi, 2014)
- Dimethyl sulfoxide (DMSO; Thermo Fisher Scientific, J66650.AK)
- 100 mM dNTP solution set (Thermo Fisher Scientific, R0182)
- pNIFTY3/pUNO (InvivoGen, pUNO4; includes Ef1a-HTLV promoter: elongation factor-1α core promoter with stabilizing segments of human T-cell leukemia virus promoter)
- pAAVS1-P-MCS (Addgene plasmid cat. no. 80488; http://n2t.net/addgene:80488; RRID Addgene_80488)
- PCR primers for Ef1a-HTLV promoter plasmid cloning (Table 2, Biolegio)
- pCMV-Gluc-SS (NanoLight, 209; contains Gaussia luciferase [GLuc] coding sequence)
- PCR primers for GLuc plasmid cloning (Table 2, Biolegio)
- Matrigel (high concentration, growth factor reduced, Corning, 354263; reproduces laminin/collagen IV-rich basement membrane, which allows feeder-free culturing of iPSCs, Totonchi et al., 2010)
- Knockout DMEM Medium (Thermo Fisher Scientific, 10829018)
- Penicillin-Streptomycin (P/S; PAN Biotech, P06-07100)
- IMR90-4 iPSCs (Wi-Cell, iPS(IMR90)-4)
- StemMACS™ iPS-Brew XF, human (Miltenyi, 130-104-368)
- Phosphate-buffered saline (PBS; see recipe)
- 0.5 mM EDTA in PBS (EDTA/PBS solution; see recipe)
- ROCK inhibitor Y-27632 (Bertin Pharma, T1725; enhances the survival of stem cells when dissociated to single cells, Watanabe et al., 2007)
- Opti-MEM™ Reduced Serum Medium (Gibco/Thermo Fisher, 31985070)
- Lipofectamine 3000 reagent kit (Thermo Fisher Scientific, L3000015)
- Passive Lysis Buffer (5×; Promega, E194A)
- Puromycin (Thermo Scientific, J67236.XF)
- Accutase (Sigma, A6964-500ML; enzyme mixture with proteolytic and collagenolytic enzyme activities that can be used at low concentrations and is therefore less toxic for more sensitive cells, Bajpai, Lesperance, Kim, & Terskikh, 2008)
- CloneR™ (Stemcell Technologies, 5888)
- DNeasy™ Blood & Tissue Kit (Qiagen, 69504)
Oligonucleotide name | Sequence |
---|---|
AAVS1T2_eSpCas9_S | 5′-CACCGGGGCCACTAGGGACAGGAT-3′ |
AAVS1T2_eSpCas9_AS | 3′-CCCCGGTGATCCCTGTCCTACAAA-5′ |
-
S, sense (5′-3′) oligonucleotide; AS, antisense (3′-5′) oligonucleotide. BbsI sticky ends, specific to this vector, are highlighted in red.
Primer name | Primer sequence (5′ to 3′) | Product size (bp) |
---|---|---|
Ef1HTLV_PacI_F | GCGGCGTTAATTAAGATCTGTAACGGCGCAGAAC | 499 |
Ef1HTLV_SalI_R | GCGGCGGTCGACGTCAGTGGGCAGAGCGCACATC | |
GLuc_SpeI_F | GCGGCGACTAGTCTAGTCACCACCGGCCCCCTTG | 530 |
GLuc_PacI_R | GCGGCGTTAATTAACCACCATGAAGCCCACCGAGAACAACGAAG | |
eSpCas9_F-seq* | catgattccttcatatttgc | 255 |
AAVS1T2_eSpCas9_R | AAACATCCTGTCCCTAGTGGCCCC |
-
To enable the restriction enzyme to bind and cleave the DNA, all cloning primers include 5′ overhangs corresponding to the appropriate restriction enzyme recognition sequence (underlined) and a GCGGCG elongation sequence (boldface). *Primer can be used both for qPCR insert confirmation and for sequencing the eSpCas9 vector.
- Toothpicks (sterile)
- Petri dishes (Sarstedt, 82.9923.429)
- Bacterial incubator and shaker, 37°C (Eppendorf, New Brunswick™ Innova™ 42)
- Screw-cap tube (Sarstedt, 72.694.305)
- Manual single-channel pipettes (10 µl/200 µl/1000 µl)
- 10-, 200-, and 1000-µl pipette tips (Sarstedt, 70.3010, 70.3030, and 70.3050.020)
- Vortexer (Scientific Industries, 444-0954)
- 0.2-, 0.5-, and 1.5-ml polypropylene microcentrifuge tubes (Sarstedt, 72.991.102, 72.704.700, 72.690.300)
- Thermocycler (Analytik Jena, 846-2-070-215)
- Horizontal gel electrophoresis chamber (Analytik Jena, 846-025-503)
- Microwave (Toshiba, MW2-MM20PF(BK))
- Chemical balance (A&D Instruments, FZ-300i-EC)
- GelDoc Go Imager (Bio-Rad, 12009077)
- Gene Pulser II (electroporator; Bio-Rad, M1652105C)
- 2-mm electroporation cuvette (Bulldog Bio, 12358-2U)
- Water bath: Ecoline O11 with heater coil (Lauda, Hydro H 24)
- 15- and 50-ml CELLSTAR™ polypropylene tubes (Greiner, 188261/227270)
- CFX Opus 96 Real-Time System (Bio-Rad, 12011319)
- Hard-Shell™ low-profile thin-wall 96-well skirted PCR plates (Bio-Rad, HSP9601)
- Microseal™ ‘B’ adhesive seals (Bio-Rad, MSB1001)
- Brand™ Pipette tip, filter tip 0.5-20 µl (Sigma, Z740037)
- 96-, 24-, and 6-well cell culture plates (Sarstedt, 83.3924, 83.3922, and 83.3920)
- 96-well clear-bottom cell culture microplate (Greiner, 655088)
- Cell culture incubator (37°C, 5% CO2)
- Eve Automatic cell counter (NanoEnTek, E1000)
- Tabletop centrifuge (Thermo Fisher, Pico™ 21)
- Laminar flow hood, class 2 (Mars Scanlaf, 9001020999)
- Inverted tissue culture microscope (Olympus, CKX53, or equivalent)
(1) Plasmid preparation for double plasmid transfection: Cloning sgRNA sequence into vector backbone encoding Cas9 endonuclease
1.To prepare the plasmid encoding Cas9 endonuclease (eSpCas9(1.1)), streak the bacterial stab onto an LB agar plate (100 µg ampicillin/ml LB agar) using a sterile toothpick or pipette tip in a zigzag pattern while working in a sterile laminar flow hood for microbiology. Incubate the plate upside down overnight at 37°C.
2.Pick individual colonies of the plasmid-containing bacteria using a sterile toothpick or pipette tip and inoculate a liquid culture in LB medium (100 µg ampicillin/ml LB medium) in a non-air-tight vessel overnight at 37°C while shaking. The next day, use the liquid culture to isolate the eSpCas9(1.1) plasmid DNA using a miniprep, midiprep, or maxiprep plasmid isolation kit, following the manufacturer's instructions.
3.Digest the eSpCas9(1.1) vector isolated from the bacteria using the restriction enzyme BbsI for 30 min at 37°C, as described below.
Reagent | Amount |
eSpCas9(1.1) vector | 2 µg |
10× buffer | 2 µl |
10 U/µl BbsI restriction enzyme | 1 µl |
H2O | Adjust volume to 20 µl |
4.Promptly dephosphorylate the digested vector by adding 1 µl FastAP alkaline phosphatase to the reaction. Incubate for another 15 min at 37°C.
5.Inactivate the reaction at 75°C for 5 min.
6.Purify the digested vector by loading the sample into wells of a 1% agarose/TAE gel, along with DNA size ladder mix. Run the gel in 1× TAE buffer in a horizontal gel electrophoresis chamber at 130 V for 1 hr. Visualize the gel using 0.25 µl/ml ethidium bromide, or an equivalent method, using the Gel Doc EZ imager (or equivalent gel imager).
7.Precisely excise the digested vector from the gel and use an agarose gel clean-up kit (for example, from Roboklon) to purify the sample, following the manufacturer's instructions.
8.Anneal and phosphorylate the oligonucleotides (AAVS1T2_eSpCas9_S, AAVS1T2_eSpCas9_AS; see Table 1 for oligonucleotide sequences) encoding the AAVS1-T2 recognition sequence as described below. Incubate the reaction in a thermocycler at 37°C for 30 min and 95°C for 5 min, and then allow the sample to slowly cool to 25°C by ramping down by 5°C per min.
Reagent | Amount (µl) |
100 µM AAVS1T2_eSpCas9_S | 1 |
100 µM AAVS1T2_eSpCas9_AS | 1 |
10× T4 ligation buffer | 1 |
T4 polynucleotide kinase | 0.5 |
H2O | Adjust volume to 10 µl |
9.Ligate the eSpCas9(1.1) vector from step 7 and the annealed and phosphorylated AAVS1-T2 oligonucleotides from step 8, as described below. Incubate the reaction at 22°C for 1 hr.
Reagent | Amount |
Digested, dephosphorylated, gel-purified eSpCas9(1.1) vector | 50 ng |
1:250 dilution of AAVS1-T2 annealed oligonucleotides | 1 µl |
10× T4 ligation buffer | 1 µl |
T4 DNA ligase | 1 µl |
H2O | Adjust volume to 10 µl |
10.Inactivate the reaction at 65°C for 10 min
11.Warm SOC medium to 37°C and place the transformation cuvette and cuvette holder on ice.
12.Add 1 µl ligation product to 50 µl electrocompetent XL1-Blue E. coli cells.
13.Immediately transfer ligation and E. coli mixture to the 2-mm electroporation cuvette.
14.Quickly slide the cuvette into the shocking chamber of the Gene Pulser II electroporator using the cold cuvette rack. Be sure to dry the cuvette and rack before pulsing the cells.
15.Apply an electrical field of 2.5 kV, with a capacitance of 25 µF and a time constant of 5 ms, to the competent cells in order to create pores in the cell walls through which the ligation product can pass.
16.Quickly add 1 ml warm SOC medium to the transformed cells, transfer to 15-ml conical tube, and incubate the transformed cells while shaking (225 rpm) at 37°C for 1 hr.
17.Evenly spread 100 µl transformed cells on an LB agar plate (100 µg ampicillin/ml LB agar) using a sterile spreading device.
18.Incubate the agar plate upside down at 37°C overnight.
19.Pick six to eight colonies using a sterile toothpick or pipette tip and place each into 5 ml LB medium with antibiotic (100 µg ampicillin/ml medium). Swirl, cover with a sterile lid, and incubate overnight at 37°C while shaking.
20.Isolate plasmid DNA from the bacterial culture using a miniprep kit following the manufacturer's instructions. Expect an extracted plasmid concentration ranging from 100 to 400 ng/µl.
21.Confirm the successful insertion of the annealed oligonucleotides by qPCR, performed as described below using thin-wall 96-well skirted PCR plates with adhesive seals and a CFX Opus 96 Real-Time System. Include non-transformed empty vector as negative control, plus non-template controls (NTC). If samples amplify, run those samples on an agarose gel (2% agarose/TAE) to verify their size. Run the gel in 1× TAE buffer in a horizontal gel electrophoresis chamber at 130 V for 1 hr. If PCR products are the expected size (255 bp), send the vector for Sanger sequencing verification. A simplified plasmid map of the eSpCas9(1.1) vector including the AAVS1-T2 gRNA sequence is illustrated in Figure 2, panel B′. See Table 2 for PCR primer sequences.
- Reaction components:
Reagent | Amount |
2× SYBR green Master Mix | 5 µl |
10 µM eSpCas9_F-seq (located in vector) | 0.25 µl |
10 µM AAVS1T2_eSpCas9_AS oligonucleotide | 0.25 µl |
1:1000 dilution of mini-prepped plasmid | 1 µl |
H2O | Adjust volume to 10 µl |
Step | Duration | Temperature | Process |
1 | 600 sec | 95°C | (denaturation) |
40 cycles of: | 20 sec | 95°C | (denaturation) |
20 sec | 60°C | (annealing) | |
20 sec | 72°C | (extension) | |
1 | 10 sec | 95°C | (melting curve) |
Ramp-up: | 5 sec/step | 65°C to 95°C | (melting curve) |
22.Once sequence is verified, inoculate a liquid culture of the bacteria containing the correct vector in 50-250 ml (depending on the plasmid isolation kit size) LB medium with antibiotic (100 µg ampicillin/ml medium) overnight at 37°C while shaking. Use the liquid culture to isolate the pAAVS1-P-MCS AAVS1-T2 plasmid DNA using a midiprep or maxiprep plasmid isolation kit, following the manufacturer's instructions.
(2a) Plasmid preparation for double plasmid transfection: Cloning Ef1a-HTLV promoter into vector backbone containing AAVS1 homology arms
23.To prepare the plasmid containing the AAVS1 homology arms (pAAVS1-P-MCS), streak the bacterial stab obtained from Addgene onto an LB agar plate with antibiotic (100 µg ampicillin/ml agar) using a sterile toothpick or pipette tip in a zigzag pattern and incubate the plate overnight at 37°C.
24.Pick individual colonies of the plasmid-containing bacteria using a sterile toothpick or pipette tip and inoculate a liquid culture in 5 ml LB medium with antibiotic (100 µg ampicillin/ml medium). Shake overnight at 37°C. Use the liquid culture to isolate the pAAVS1-P-MCS plasmid DNA using a miniprep plasmid isolation kit, following the manufacturer's instructions.
25.Carry out a double digestion of the pAAVS1-P-MCS vector obtained in step 24 using the restriction enzymes PacI and SalI for 15 min at 37°C, as described below.
Reagent | Amount |
pAAVS1-P-MCS vector | 2 µg |
10× FastDigest buffer | 2 µl |
PacI FastDigest restriction enzyme | 1 µl |
SalI FastDigest restriction enzyme | 1 µl |
H2O | Adjust volume to 20 µl |
26.Promptly dephosphorylate the digested vector by adding 1 µl Fast AP to the reaction. Incubate for another 15 min at 37°C.
27.Inactivate the reaction at 75°C for 5 min.
28.Purify the digested vector by loading the sample into wells of a 1% agarose/TAE gel. Run the gel in 1× TAE buffer in a horizontal gel electrophoresis chamber at 130 V for 1 hr. Visualize the gel using 0.25 µl/ml ethidium bromide or by an equivalent method.
29.Precisely excise the digested vector from the gel and use a gel clean-up kit to purify the sample, following the manufacturer's instructions.
30.Selectively amplify the Ef1a-HTLV promoter sequence (see Table 2 for PCR primer sequences).
PCR reaction components:
Reagent | Amount |
5× GC Phusion buffer | 10 µl |
10 mM dNTPs | 1 µl |
10 µM Ef1HLTV_PacI_F primer | 2.5 µl |
10 µM Ef1HTLV_SalI_R primer | 2.5 µl |
DMSO | 1.5 µl |
2 U/µl Phusion polymerase | 0.5 µl |
pNIFTY3/pUNO plasmid DNA (includes Ef1a-HTLV promoter) | 10 ng |
H2O | Adjust volume to 50 µl |
PCR protocol:
Step | Duration | Temperature | Process |
1 | 180 sec | 98°C | (denaturation) |
35 cycles of: | 30 sec | 98°C | (denaturation) |
20 sec | 61°C | (annealing) | |
60 sec | 72°C | (extension) | |
1 | 600 sec | 72°C | (extension). |
31.Run PCR reaction on an agarose gel (2% agarose/TAE) at 130 V for 1 hr, visualize with ethidium bromide (0.25 µl/ml), and precisely excise the desired products from the gel.
32.Purify the amplicon with a gel clean-up kit according to the manufacturer's instructions.
33.Double digest the Ef1-HTLV PCR product with the PacI and SalI restriction enzymes as described below, at 37°C for 15 min. Inactivate the enzymes at 70°C for 5 min.
Reagent | Amount |
Ef1-HTLV PCR product | 20 µl |
10× FastDigest buffer | 2.5 µl |
PacI restriction enzyme | 1 µl |
SalI restriction enzyme | 1 µl |
H2O | Adjust volume to 25 µl |
34.Run digestion reaction on an agarose gel (2% agarose/TAE) at 130 V for 1 hr, visualize with ethidium bromide (0.25 µl/ml), and precisely excise the desired products from the gel.
35.Purify the amplicon with a gel clean-up kit according to the manufacturer's instructions.
36.Ligate the pAAVS1-P-MCS vector from step 29 and the gel purified Ef1-HTLV promoter from step 35 as described below, in a 3:1 insert to vector molar ratio. Incubate the reaction at 22°C for 1 hr.
Reagent | Amount |
Digested, dephosphorylated, gel-purified pAAVS1-P-MCS vector | 50 ng |
Digested, gel-purified PCR product | 13.6 ng |
10× T4 ligation buffer | 1 µl |
T4 DNA ligase | 1 µl |
H2O | Adjust volume to 10 µl |
37.Inactivate the reaction at 65°C for 10 min
38.Follow steps 11-19 to perform the bacterial transformation with the ligation of interest.
39.Isolate plasmid DNA of the new “pAAVS1-promEf1-HTLV” vector from the bacterial culture using a miniprep kit, following the manufacturer's instructions.
40.Check the successful insertion by double digest, using the reaction composition detailed below and incubating the reaction at 37°C for 15 min.
Reagent | Amount |
Plasmid DNA | 5 µl |
10× FastDigest buffer | 2 µl |
PacI restriction enzyme | 1 µl |
SalI restriction enzyme | 1 µl |
H2O | Adjust volume to 20 µl |
41.Run digestion on an agarose gel (2% agarose/TAE) at 130 V for 1 hr, and visualize with ethidium bromide (0.25 µl/ml). If the digested products are the expected size, send the vector for Sanger sequencing verification.
42.Once sequence is verified, inoculate a liquid culture of the bacteria containing the correct vector in LB medium (100 µg ampicillin/ml LB medium) overnight at 37°C while shaking. Use the liquid culture to isolate the pAAVS1-promEf1-HTLV plasmid DNA using a midiprep or maxiprep plasmid isolation kit, following the manufacturer's instructions.
(2b) Plasmid preparation for double plasmid transfection: Cloning coding sequence of Gaussia luciferase reporter into vector backbone containing Ef1-HTLV promoter in between AAVS1 homology arms
43.Carry out a double digestion of sequence-verified pAAVS1-promEf1-HTLV vector from step 42 using the restriction enzymes SpeI and PacI for 15 min at 37°C, as described below.
Reagent | Amount |
pAAVS1-promEf1-HTLV vector | 2 µg |
10× FastDigest buffer | 2 µl |
SpeI restriction enzyme | 1 µl |
PacI restriction enzyme | 1 µl |
H2O | Adjust volume to 20 µl |
44.Optional : Promptly dephosphorylate the digested vector by adding 1 µl Fast AP to the reaction. Incubate for another 15 min at 37°C.
45.Inactivate the reaction at 75°C for 5 min.
46.Purify the digested vector by loading the sample into wells of a 1% agarose/TAE gel. Run the gel in 1× TAE buffer in a horizontal gel electrophoresis chamber at 130 V for 1 hr. Visualize the gel using 0.25 µl/ml ethidium bromide or by an equivalent method.
47.Precisely excise the digested vector from the gel and use a gel clean-up kit to purify the sample.
48.Selectively amplify the Gaussia luciferase (GLuc) coding sequence from the pCMV-GLuc-SS plasmid, as described below. See Table 2 for PCR primer sequences.
- PCR reaction components:
Reagent | Amount |
5× GC Phusion buffer | 10 µl |
10 mM dNTPs | 1 µl |
10 µM GLuc_SpeI_F primer | 2.5 µl |
10 µM GLuc_PacI_R primer | 2.5 µl |
2 U/µl Phusion polymerase | 0.5 µl |
Plasmid DNA | 10 ng |
H2O | Adjust volume to 50 µl |
Step | Duration | Temperature | Process |
1 | 180 sec | 98°C | (denaturation) |
35 cycles of: | 30 sec | 98°C | (denaturation) |
20 sec | 72°C | (annealing) | |
60 sec | 72°C | (extension) | |
1 | 600 sec | 72°C | (extension) |
49.Run PCR reaction on an agarose gel (2% agarose/TAE) at 130 V for 1 hr, visualize with ethidium bromide (0.25 µl/ml), and precisely excise the desired products from the gel.
50.Purify the amplicon with a gel clean-up kit, according to the manufacturer's instructions.
51.Double digest the GLuc PCR product with the SpeI and PacI restriction enzymes as described below, at 37°C for 15 min.
Reagent | Amount |
GLuc PCR product | 20 µl |
10× FastDigest buffer | 2.5 µl |
SpeI restriction enzyme | 1 µl |
PacI restriction enzyme | 1 µl |
H2O | Adjust volume to 25 µl |
52.Inactivate the enzymes at 70°C for 5 min.
53.Run digestion reaction on an agarose gel (2% agarose/TAE) at 130 V for 1 hr, visualize with ethidium bromide (0.25 µl/ml), and precisely excise the desired products from the gel.
54.Purify the amplicon with a gel clean-up kit, according to the manufacturer's instructions.
55.Ligate the pAAVS1-promEf1-HTLV vector from step 47 and the gel purified, double-digested GLuc coding sequence from step 54 as described below, in a 3:1 insert-to-vector molar ratio. Incubate the reaction at 22°C for 1 hr.
Reagent | Amount |
Digested, dephosphorylated, gel-purified pAAVS1-promEf1-HTLV vector | 50 ng |
Digested, gel-purified PCR product | 13.2 ng |
10× T4 ligation buffer | 1 µl |
T4 DNA ligase | 1 µl |
H2O | Adjust volume to 10 µl |
56.Inactivate the reaction at 65°C for 10 min.
57.Follow steps 11-19 to perform the bacterial transformation with the ligation of interest.
58.Isolate plasmid DNA, now called “AAVS1-Ef1HTLV-Gluc,” from the bacterial culture using a miniprep kit, following the manufacturer's instructions.
59.Check the successful insertion by double digest, as described below, incubating the reaction at 37°C for 15 min. Run digestion on an agarose gel (2% agarose/TAE) at 130 V for 1 hr, visualize with ethidium bromide (0.25 µl/ml). If the digested products are the expected size, send the vector for Sanger sequencing verification. A simplified plasmid map of pAAAVS1-Ef1-HLTV_GLuc is illustrated in Figure 2B′′.
Reagent | Amount |
Plasmid DNA | 5 µl |
10× FastDigest buffer | 2 µl |
SpeI restriction enzyme | 1 µl |
PacI restriction enzyme | 1 µl |
H2O | Adjust volume to 20 µl |
60.Once sequence is verified, inoculate a liquid culture of the bacteria containing the correct vector in 50 ml to 250 ml (depending on the plasmid isolation kit size) LB medium with antibiotic (100 µg ampicillin/ml medium) overnight at 37°C while shaking. Use the liquid culture to isolate the AAVS1-Ef1HTLV-GLuc plasmid DNA using a midiprep or maxiprep plasmid isolation kit, following the manufacturer's instructions.
Standard cell culture maintenance
61.Working in a laminar flow hood, culture undifferentiated IMR90-4 cells on Matrigel-coated plates in StemMACs™ iPS-Brew XF human cell culture medium containing 1% P/S in feeder-free conditions at 37°C and 5% CO2. Change medium every 2 days.
62.Split cells 1:40 when they reach ∼80% confluency: Aspirate the old medium and wash the cells by carefully adding 2 ml PBS to the well. Gently swirl the PBS, then aspirate and add 1 ml EDTA/PBS solution. Incubate the cells for 5 min at room temperature.
63.Gently aspirate the EDTA/PBS solution.
64.Resuspend and plate the split cells in fresh StemMACs™ iPS-Brew XF human cell culture medium supplemented with 1 µM Y-27632 ROCK inhibitor.
(3) Double plasmid transfection of IMR90-4 iPSCs with eSpCas9(1.1)-AAVS1-T2 and AAVS1-Ef1HTLV-GLuc
65.Carry out the IMR90-4 transfection according to the Lipofectamine 3000 transfection protocol. Seed cells (2 × 105/well) in two wells of a 6-well plate and begin the transfection once the cells reach 60% confluency.
66.Add the plasmids in a 1:4 ratio of eSpCas9(1.1)-AAVS1-T2 plasmid to pAAVS1-Ef1HTLV-Gluc. Combine the two transfection master mixes, master mix 1 and master mix 2, as described below. Incubate the combined mixture at room temperature for 15 min and then add to cells containing fresh standard StemMACs™ iPS-Brew XF medium.
Reagent | Quantity per well |
Master mix 1 | |
Opti-MEM medium | 125 µl |
Lipofectamine 3000 reagent | 7.5 µl |
Master mix 2 | |
Opti-MEM medium | 125 µl |
eSpCas9(1.1)-AAVS1T2 | 625 ng |
pAAVS1-Ef1HLTV-Gluc | 1875 ng |
P3000 reagent | 5 µl |
67.Incubate the cells and transfection reagents at 37°C in 5% CO2 for 48 hr.
68.After 48 hr, lyse the cells in one of the wells using 1× Passive Lysis Buffer and transfer to a 1.5-ml microcentrifuge tube, to verify transient luciferase expression. See Support Protocol 1 for luciferase activity measurement procedure.
69.Using the second well, begin the antibiotic selection process of cells that have incorporated a stable genomic integration of donor DNA: Remove the transfection medium and apply standard StemMACs™ iPS-Brew XF medium containing 500 ng/ml puromycin.
70.Change the selection medium every 2 days.
71.Once most cells have died and only a few healthy clusters remain, count the remaining cells with a standard cell culture microscope.
72.Wash cells with PBS.
73.Incubate the cells with Accutase at 37°C for 5 min. Collect the detached cells in a 15-ml conical tube and centrifuge 3 min at 250 × g , room temperature.
74.Resuspend the cells in a dilution of 1 cell/100 µl in monoclonal selection medium (CloneR reagent diluted as per manufacturer's instructions in StemMACs™ iPS-Brew XF medium supplemented with 500 ng/ml puromycin) and seed into wells in Matrigel-coated 96-well plates for single-cell dilution and monoclonal isolation.
75.Change the monoclonal selection medium every 3 days by carefully aspirating the old medium and replacing with 100 µl of fresh selection medium. It is more important to avoid disturbing the cells than to remove 100% of the old medium; therefore, just quickly and efficiently aspirate from one corner of the well. Carefully observe the cells to be sure that each well of the 96-well plate does not contain more than 1 colony per well.
76.Once a cluster of ∼10-20 healthy cells has grown in a well of the 96-well plate, transfer these cells to a well of a Matrigel-coated 24-well plate using EDTA/PBS solution as described in steps 62-64.Include 1 µM Y-27632 ROCK inhibitor and 500 ng/ml puromycin in the fresh splitting medium. It is no longer necessary to use the ClonerR supplement.
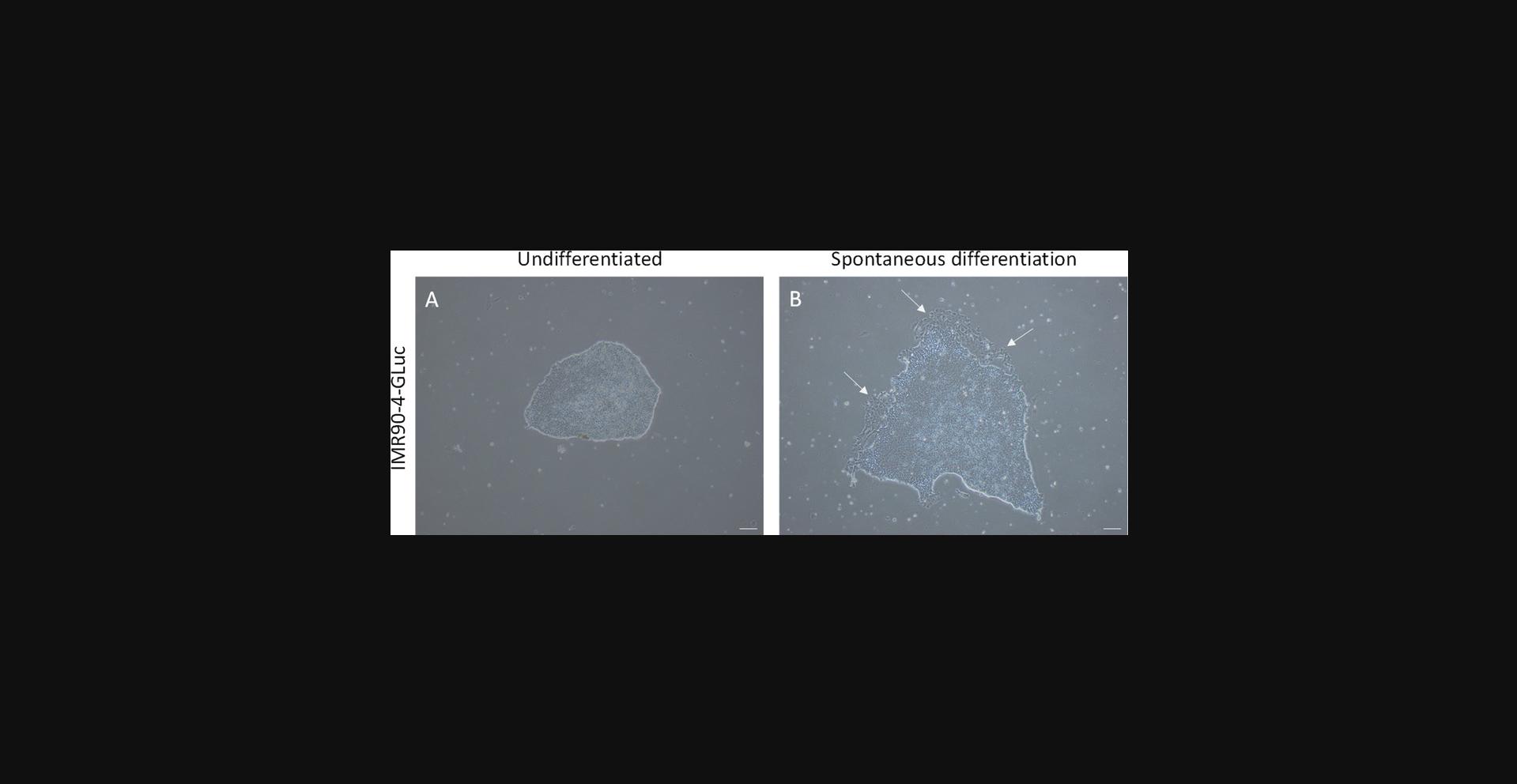
77.Once cells in the 24-well plate have reached ∼60-70% confluency, transfer the cells from one well of the 24-well plate into one well of a Matrigel-coated 6-well plate using EDTA/PBS solution as described in steps 62-64.Include 1 µM Y-27632 ROCK inhibitor and 500 ng/ml puromycin in the fresh splitting medium.
78.Once cells in the 6-well plate have reached ∼70% confluency, all cells can be split into six wells of a Matrigel-coated 6-well plate using EDTA/PBS solution as described in steps 62-64 for propagation, freezing, and cellular analysis.
79.In order to analyze the copy number of the inserted donor sequence, extract gDNA from the monoclonal cell lines using Qiagen DNeasy™ Blood and Tissue extraction kit (or equivalent kit), according to the manufacturer's instructions.
Support Protocol 1: MEASUREMENT OF GAUSSIA LUCIFERASE ACTIVITY TO VERIFY FUNCTIONALITY OF REPORTER PROTEIN
This protocol describes how to measure Gaussia luciferase activity using the substrate coelenterazine. This process is not meant to quantify the luciferase expression, but rather to verify that the selected clone generally expresses luciferase, and therefore can be defined as a clone of interest and should be propagated and analyzed in the later support and basic protocols. The cells should be harvested as described and lysed, and the luciferase activity should be measured in a single-tube- or plate-reading luminometer.
NOTE : Care should be taken to work in a sterile environment. This protocol falls under Biosafety Level 1.
Materials
-
Coelenterazine (Carl Roth, 4094.3)
-
99.8% ethanol, for analysis (Carl Roth, 9065.1)
-
0.5 mM EDTA in PBS (see recipe)
-
Monoclonal iPSCs (Basic Protocol 1)
-
StemMACS™ iPS-Brew XF, human (Miltenyi, 130-104-368)
-
PBS (see recipe)
-
Passive Lysis Buffer 5×; Promega, E194A)
-
Manual single-channel pipettes
-
0.2-, 0.5-, and 1.5-ml polypropylene microcentrifuge tubes (Sarstedt, 72.991.102, 72.704.700, and 72.690.300)
-
10-, 200-, and 1000-µl pipette tips (Sarstedt, 70.3010, 70.3030, and 70.3050.020)
-
Tabletop centrifuge (Thermo Fisher, Pico™ 21)
-
Vortexer (Scientific Industries, 444-0954)
-
Ice machine
-
White 96-well flat-bottom microplate (Greiner, 655073)
-
FLUOstar Omega microplate reader (BMG Labtech, FLUOstar Omega) or equivalent
1.Resuspend synthetic coelenterazine in ethanol at 2 mM concentration (stock solution).
2.Two hours before performing the luminescence measurement, dilute the 2 mM stock to 10 µM in water and store in the dark until use. Use cellular lysates to first confirm transient expression of luciferase in newly transfected cells. Later on, set aside some cells from the monoclonal cell lines, generally during a splitting step as described in step 77 of the Basic Protocol 1, to confirm whether they express GLuc before starting to expand them.
3.Prepare lysates by harvesting a clone at 50%-70% confluency that has been incubated in StemMACS iPS-Brew XF at 37°C, 5% CO2 (as described in steps 62-63 of Basic Protocol 1). Dissociate the cells from the cell culture plate using 0.5 mM EDTA in PBS, resuspend the cells in 1 ml of fresh cell culture medium, remove 100 µl of the cell suspension, and use these cells in the next step.
4.Centrifuge the cells for 3 min at 250 × g , room temperature.
5.Aspirate the medium, resuspend the cells in 1 ml PBS, and centrifuge 3 min at 250 × g , room temperature.
6.Aspirate the PBS and resuspend and incubate the cells in 20 µl of 1× Passive Lysis Buffer for 10 min on ice.
7.Centrifuge the lysed cells 3 min at 15,000 × g , room temperature to clear cellular debris.
8.Measure luciferase activity: Place 20 µl lysate in a white-walled white-bottomed 96-well plate, automatically inject 100 µl of the 10 µM coelenterazine solution into the sample well, and immediately measure light for 10 sec.
Support Protocol 2: VERIFICATION OF MONOCLONAL EXPANSION USING IMMUNOFLUORESCENCE
This protocol describes how to verify that the transfected and isolated cells from Basic Protocol 1 are monoclonal. This step is essential for the correct analysis of the dc-qcnPCR. If care was taken in carrying out the single-cell dilution and subsequent expansion of the cell lines, the expanded clonal line should have originated from a single cell and therefore the ratio of the inserted donor DNA to the autosomal gene should be equal between all isolated cells. If the cell population is impure, this will affect the ratio of copy numbers of GLuc and the autosomal gene and make it impossible to accurately determine whether the clone contains off-target integrations of donor DNA conjugate.
NOTE : Care should be taken to work in a sterile environment. This protocol falls under Biosafety Level 1.
Materials
-
KnockOut DMEM (Thermo Fisher Scientific, 10829018)
-
Matrigel (high concentration, growth factor reduced; Corning, 354263; reproduces laminin-/collagen-IV-rich basement membrane, which allows feeder-free culturing of iPSCs, Totonchi et al., 2010)
-
4% paraformaldehyde (PFA; see recipe)
-
PBS (see recipe)
-
Immunofluorescence permeabilization buffer (0.25% PBS-T; see recipe)
-
Anti-Gaussia luciferase (rabbit; NEB, E8023)
-
Anti-rabbit IgG(H+L)-Alexa Fluor 488 (Invitrogen, A32731)
-
Immunofluorescence blocking solution (see recipe)
-
DAPI (see recipe)
-
VECTASHIELD™ Antifade Mounting Medium (Vector Laboratories, H-1000-10)
-
Clear nail polish
-
Manual single-channel pipettes
-
10-, 200-, and 1000-µl pipette tips (Sarstedt, 70.3010, 70.3030, 70.3050.020)
-
Vortexer (Scientific Industries, 444-0954)
-
24-well plate (Sarstedt, 83.3922)
-
Microscope cover glasses (Paul Marienfeld GmbH & Co. KG, 111520)
-
Cell culture incubator (37°C/5% CO2; Panasonic, MCO-170AICUVH-PE)
-
Rocker 3D (IKA, 444-0817)
-
Refrigerator
-
Small-gauge needle (Carl Roth, C724.1; any needle of gauge <20 can be used)
-
Curved tweezers (Carl Roth, 2856.1)
-
Microscope slides (Carl Roth, H868)
-
Aluminum foil
-
Software: Fiji/ImageJ
-
Fluorescence microscope (Leica, DM6 B, or equivalent)
1.Prepare Matrigel-coated coverslips in 24-well plates by transferring one sterile coverslip into each plate well (or however many wells are necessary for the experiment), adding 300 µl of a 1:100 dilution of Matrigel in KnockOut DMEM medium on top of each coverslip, and incubating the plate for at least 2 hr at room temperature. Aspirate the Matrigel solution and immediately plate 100,000 cells/well onto the coverslips. Cultivate the cells under standard conditions at 37°C and 5% CO2 (see Basic Protocol 1, step 61).
2.After the cells have adhered to the coverslip, fix them by aspirating out the standard medium and adding 300 µl of 4% paraformaldehyde. Incubate at room temperature for 10 min.
3.Wash the coverslips three times, for 10 min each, in PBS.
4.Aspirate the last PBS wash and permeabilize the cells in immunofluorescence permeabilization buffer (0.25% PBS-T) for 10 min at room temperature.
5.Aspirate the permeabilization buffer and block the cells in immunofluorescence blocking solution for 60 min at room temperature.
6.Dilute the anti-Gaussia luciferase primary antibody 1:1000 in immunofluorescence blocking solution.
7.Incubate the cells in 300 µl diluted primary antibody at 4°C overnight. Alternatively, to reduce antibody usage, the coverslips can be removed from the 24-well plate and incubated, cell side down, in 25 µl antibody dilution, placed as dots on Parafilm, at 4°C overnight.
8.The next day, carefully transfer the cover slips back into 24-well plates, cell side up, and remove excess antibodies by washing three times, for 10 min each, in PBS.
9.Dilute the Alexa Fluor 488-conjugated anti-rabbit secondary antibodies 1:1000 in immunofluorescence blocking solution and incubate at room temperature for 1 hr.
10.Aspirate the secondary antibody solution and incubate the cells in 300 nM DAPI, diluted in PBS, for 5 min.
11.Wash the cover slips three times, for 10 min each, in PBS.
12.Mount the cover slips on glass microscope slides using 3.2 µl VECTASHIELD™ Antifade Mounting Medium and seal by applying clear nail polish to the perimeter of the cover slip.
13.Image the cell preparations with a fluorescence microscope and verify that all captured cells express the reporter of interest (Fig. 3).
Basic Protocol 2: INSERT-CONFIRMATION PCR
This protocol describes how to carry out the insert-confirmation PCR using the extracted gDNA of monoclonal cell lines. The insert-confirmation PCR is necessary to confirm that the donor DNA has indeed been inserted into the AAVS1 safe-harbor locus. This test does not exclude off-target integrations, but only confirms on-target integrations. Here it is essential to design the PCR primers to be 5′ and 3′ of the safe-harbor locus, preferentially completely flanking the homology arm sequences found on the transfection plasmid. If this is not possible, no more than one PCR primer may be found in one of the homology arm sequences, and the other primer must be outside the region of homology-directed repair (Fig. 4). These rules serve to exclude the accidental amplification of off-target inserted donor DNA sequence. Once clones are identified as containing on-target donor DNA insertions, the PCR products containing the inserts should be Sanger sequenced to verify the amplification of the correct genomic locus.
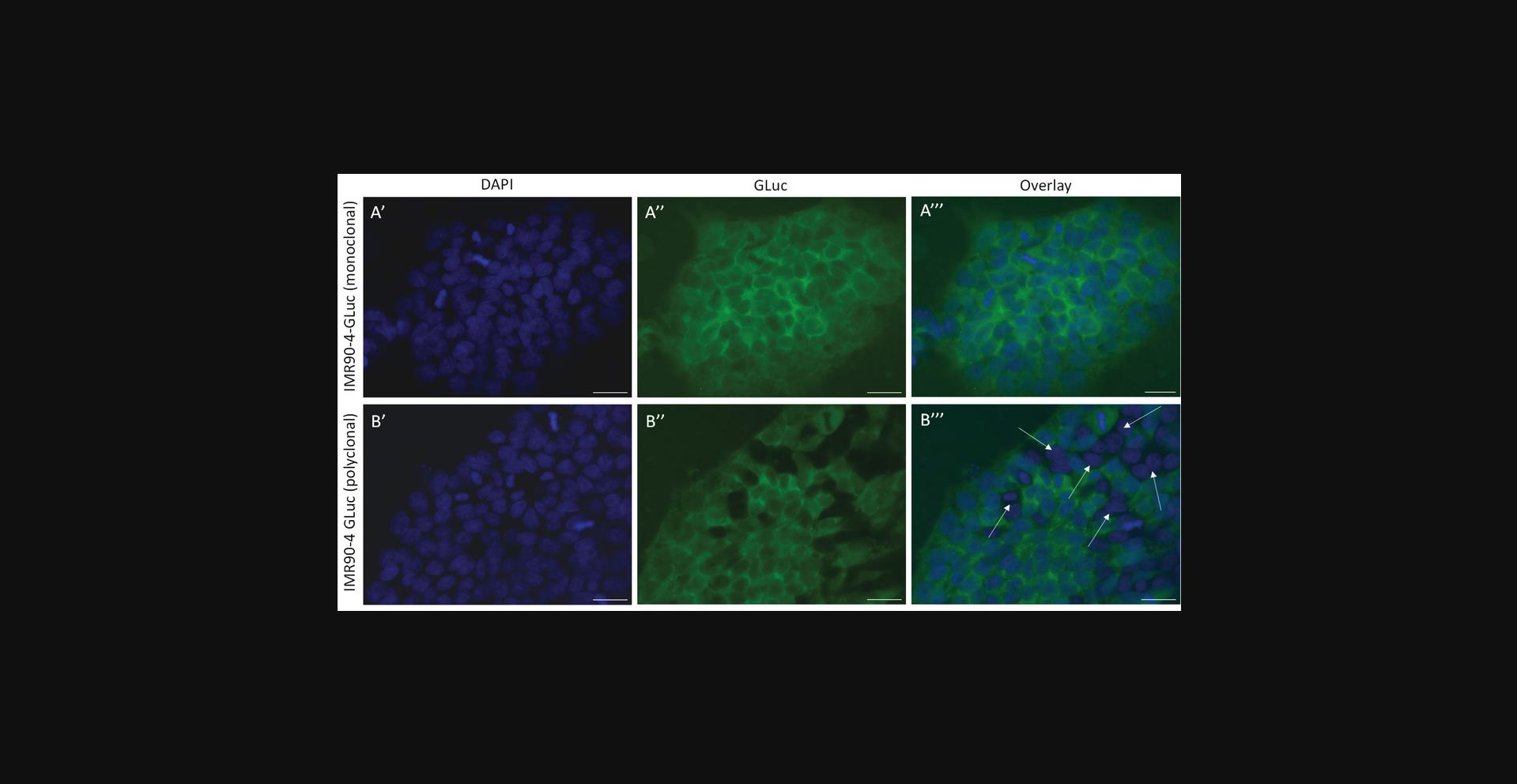
Materials
- Phusion™ High-Fidelity DNA Polymerase (2 U/µl) Kit (Thermo Fisher Scientific, F530S; high-fidelity, proof-reading enzyme that prepares blunt-end PCR products, McInerney et al., 2014)
- 100 mM dNTP solution set (Thermo Fisher Scientific, R0182)
- Dimethyl sulfoxide (DMSO; Thermo Fisher Scientific, J66650.AK)
- PCR primers used for insert-confirmation PCR (Table 3, Biolegio)
- gDNA (Basic Protocol 1)
- 10× TAE solution (see recipe)
- Agarose NEEO Ultra-Quality (Carl Roth, 2267.4)
- Ethidium bromide (Thermo Fisher Scientific, 15585011)
- DNA ladder: e.g., MassRuler DNA ladder ready-to-use (Thermo Fisher Scientific, SM0403)
- Agarose-out DNA Purification Kit (Roboklon, E3540)
Primer name | Primer sequence (5′ to 3′) | Product size, WT (bp) | Product size, insertion (bp) |
---|---|---|---|
5′probeWT_F | TTCAGGTTCCGTCTTCCTCC | 850 | 2950 |
Rpcr-wt-3'HA | AGGATCCTCTCTGGCTCCAT |
- Vortexer (Scientific Industries, 444-0954)
- Manual single-channel pipettes
- 0.2-, 0.5-, and 1.5-ml polypropylene microcentrifuge tubes (Sarstedt, 72.991.102, 72.704.700, and 72.690.300)
- 10-, 200-, and 1000-µl pipette tips (Sarstedt, 70.3010, 70.3030, and 70.3050.020)
- Thermocycler (with a gradient function; Analytik Jena, 846-2-070-215)
- Horizontal gel electrophoresis chamber (Analytik Jena, 846-025-503)
- Microwave (Toshiba, MW2-MM20PF(BK))
- Chemical balance (A&D Instruments, FZ-300i-EC)
- Gel Doc™ Go Imager (Bio-Rad, 12009077)
- Sanger sequencing source
1.Amplify gDNA extracted from each monoclonal cell line (Basic Protocol 1, step 79) using the insert-confirmation PCR protocol described below. See Table 3 for PCR primer sequences.
- PCR reaction components:
Reagent | Amount |
5× GC Phusion buffer | 10 µl |
10 mM dNTPs | 1 µl |
10 µM 5′probeWT_F primer | 2.5 µl |
10 µM Rpcr-wt-3’HA primer | 2.5 µl |
DMSO | 1.5 µl |
2 U/µl Phusion polymerase | 0.5 µl |
gDNA | 10-20 ng |
H2O | Adjust volume to 50 µl |
Step | Duration | Temperature | Process |
1 | 30 sec | 98°C | (denaturation) |
35 cycles of: | 45 sec | 98°C | (denaturation) |
45 sec | 70°C | (annealing) | |
120 sec | 72°C | (extension) | |
1 | 600 sec | 72°C | (extension). |
2.Visually inspect the PCR products by loading the samples into wells of a 1% agarose/TAE gel alongside the appropriate DNA ladder. Run the gel in 1× TAE buffer in a horizontal gel electrophoresis chamber at 130 V for 1 hr. Visualize the gel using 0.25 µl/ml ethidium bromide or by an equivalent method.
3.Analyze the gel by assessing the amplicon patterns as described and exemplified in Figure 4, whereby an amplified product of WT gDNA is expected to be 850 bp and an amplified product of gDNA including the donor DNA is expected to be almost 3000 bp.
4.Use this heterozygous/homozygous insertion data to fully interpret the results of the dc-qcnPCR in Basic Protocol 3.
5.Identify PCR products including donor DNA insertions and excise them from the gel. Purify the excised gel bands using a gel-purification kit and perform Sanger sequencing to confirm the accuracy and specificity of the insert sequence in the AAVS1 safe harbor.
Basic Protocol 3: DESIGN AND PREPARATION OF DOUBLE-CONTROL QUANTITATIVE COPY NUMBER PCR REAGENTS FOLLOWED BY QUANTIFICATION OF DONOR DNA INTEGRATIONS IN GENETICALLY MODIFIED MONOCLONAL CELLS
This protocol describes how to prepare the control plasmids for the dc-qcnPCR, organize the qPCR of the gDNA extracted from the monoclonal cell lines, and calculate and interpret the results of the dc-qcnPCR. It is necessary to have at least one control plasmid that contains the sequences of the donor DNA, an autosomal gene, and a ChrX gene (in the following Basic Protocol 3, these sequences are specifically GLuc , CHOP , and RBBP7 , as explained in detail below). To double-check the quality of the control plasmid, it is reasonable to prepare a second plasmid containing the sequences of the donor DNA, the same autosomal gene, and a different ChrX gene. In the following protocol, these sequences are described as GLuc , CHOP , and HPRT1 specifically. Thus, segments of the autosomal gene DNA damage inducible transcript 3 (CHOP) and the ChrX genes hypoxanthine phosphoribosyltransferase 1 (HPRT1) or RB binding protein 7, chromatin remodeling factor (RBBP7) are included in the control plasmids. Both plasmids can be analyzed separately by qPCR. The qPCR plate will include the amplification of each control plasmid using the primer sets for the donor DNA (GLuc), the autosomal gene (CHOP), and the appropriate ChrX gene (HPRT1 or RBBP7). The qPCR plate will also include the gDNA extracted from the monoclonal cell lines of interest, which will also be amplified using the primer sets for the donor DNA (GLuc), the autosomal gene (CHOP), and the appropriate ChrX gene (HPRT1 or RBBP7). The ChrX control is most useful in the monoclonal cell lines if they are male-derived cells, and therefore should have one copy of the selected gene. If the cell line is female-derived, the copy number should be equal to that of the autosomal gene and the detection of a single copy number difference cannot be calculated. Therefore, it is also recommended that high-quality gDNA from a female and a male be included on the qPCR plate, which should be amplified using the primer sets from the autosomal gene and the appropriate ChrX gene. These data can be used as a control to verify that the method calculates the correct ratio of ChrX genes in females and males as 2:1 (Fig. 5).
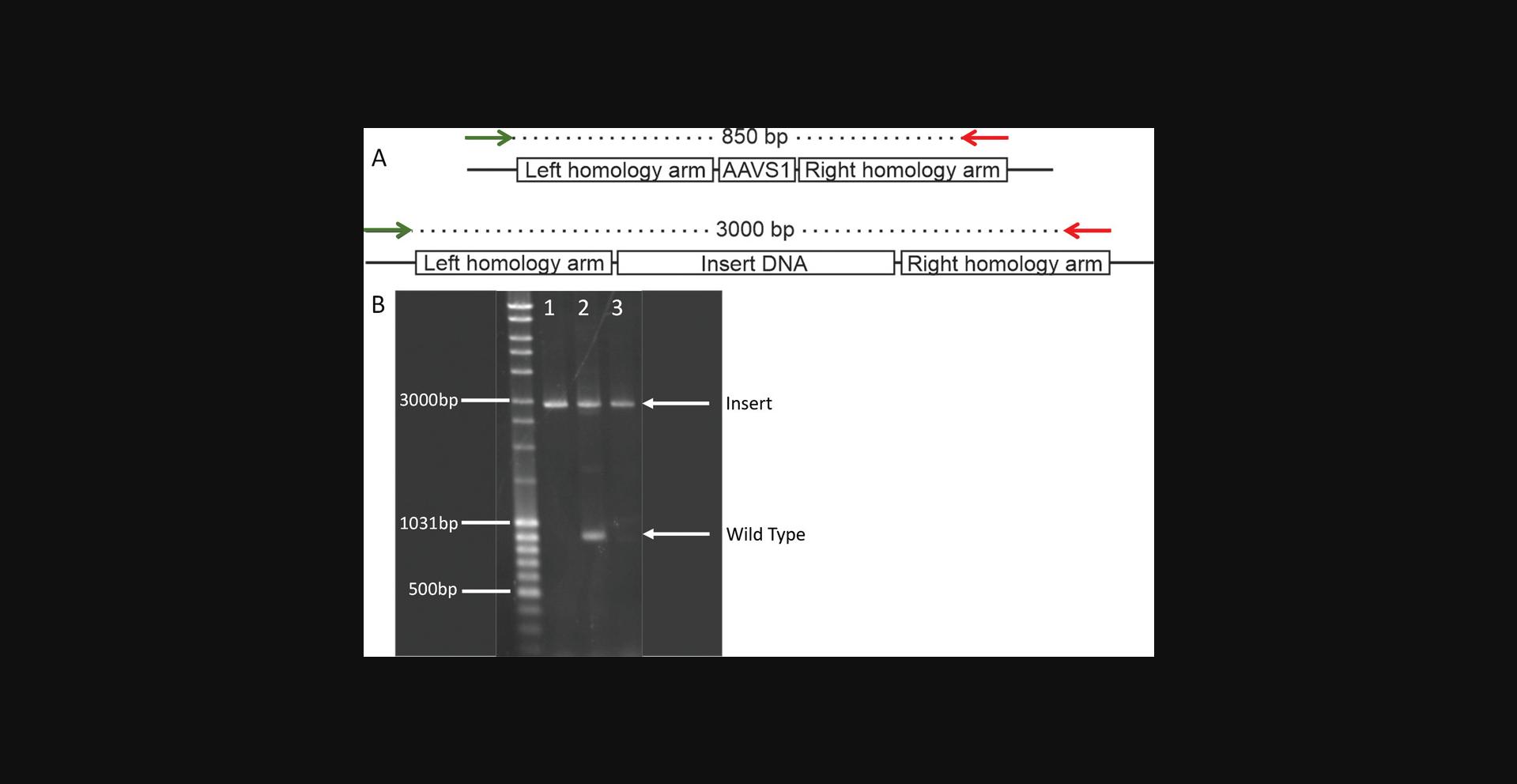
Materials
-
gDNA (Basic Protocol 1)
-
AAVS1-Ef1HTLV-Gluc plasmid (Basic Protocol 1)
-
PCR primers used for CHOP plasmid cloning (Table 4, Biolegio)
-
Phusion™ High-Fidelity DNA Polymerase (2 U/µl) Kit (Thermo Fisher Scientific, F530S)
-
100 mM dNTP solution set (Thermo Fisher Scientific, R0182)
-
Dimethyl sulfoxide (DMSO; Thermo Fisher Scientific, J66650.AK)
-
T4 polynucleotide kinase (Thermo Fisher Scientific, EK0031)
-
pAAVS1-Ef1HTLV-Gluc vector (Basic Protocol 1)
-
FastDigest restriction enzymes (Thermo Fisher Scientific, various) and buffers
-
FastAP Thermosensitive Alkaline Phosphatase (1 U/µl; Thermo Fisher Scientific, EF0651)
-
10× TAE solution (see recipe)
-
Agarose NEEO Ultra-Quality (Carl Roth, 2267.4)
-
Ethidium bromide (Thermo Fisher Scientific, 15585011)
-
MassRuler DNA ready-to-use DNA ladder (Thermo Fisher Scientific, SM0403)
-
Agarose-out DNA Purification Kit (Roboklon, E3540)
-
T4 DNA ligase (5 U/µl; Thermo Fisher Scientific, EL0011) with buffer
-
SOC medium (see recipe)
-
XL1-Blue Electroporation-Competent Cells (Agilent, 200228)
-
LB agar (see recipe)
-
Ampicillin (Gibco, 11593027)
-
LB medium (see recipe)
-
GeneJET Plasmid Miniprep Kit (Thermo Fisher Scientific, K0503)
-
PCR primers used for HPRT1 and RBBP7 plasmid cloning (Table 4; Biolegio)
-
Maxima SYBR Green/ROX qPCR Master Mix (2×; Thermo Fisher Scientific, K0222)
-
PCR primers used for dc-qcnPRC analysis (Table 4; Biolegio)
Primer name | Sequence | Product size (bp) |
---|---|---|
Gaussia luciferase | ||
GLuc_F | CCCCTTGATCTTGTCCACCT | 299 |
GLuc_R | GCACGCCCAAGATGAAGAAG | |
Autosomal control gene | ||
hCHOP-F | CAGAACCAGCAGAGGTCACA | 200 |
hCHOP-R | AGCTGTGCCACTTTCCTTTC | |
X-chromosome genes | ||
xRBBP7_F | AAATTTCACTGACAGGGCCG | 264 |
xRBBP7_R | GGCCATCTCAATTTGTCCCG | |
xHPRT1_F | GGGCTAGACTTTTGAGGGACA | 250 |
xHPRT1_R | AGTCCTAATCGGCCATTACTGA |
- Tabletop centrifuge (step 18; Thermo Fisher, Pico™ 21)
- 10-, 200-, and 1000-µl pipette tips (Sarstedt, 70.3010, 70.3030, and 70.3050.020)
- 0.2-, 0.5-, and 1.5-ml polypropylene microcentrifuge tubes (Sarstedt, 72.991.102, 72.704.700, and 72.690.300)
- Manual single-channel pipettes
- Thermocycler (Analytik Jena, 846-2-070-215)
- Water bath: Ecoline O11 with heater coil (Lauda, Hydro H 24)
- Microwave (Toshiba, MW2-MM20PF(BK))
- Chemical balance (A&D Instruments, FZ-300i-EC)
- Horizontal gel electrophoresis chamber (Analytik Jena, 846-025-503)
- Gel Doc Go Imager (Bio-Rad, 12009077)
- Gene Pulser II (electroporator; Bio-Rad, M1652105C)
- 20-mm electroporation cuvette (Bulldog Bio, 12358-2U)
- Screw-cap tube (Sarstedt, 72.694.305)
- 15- and 50-ml CELLSTAR™ Polypropylene tubes (Greiner, 188261/227270)
- Laminar flow hood, class 2, for microbiology (Mars Scanlaf, 9001020999)
- Petri dishes (Sarstedt, 82.9923.429)
- Bacterial incubator and shaker, 37°C (Eppendorf, New Brunswick™ Innova™ 42)
- Toothpick, sterile
- CFX Opus 96 Real-Time System (Bio-Rad, 12011319)
- Microseal™ ‘B’ adhesive seals (Bio-Rad, MSB1001)
- Hard-Shell™ low-profile thin-wall 96-well skirted PCR plates (Bio-Rad, HSP9601)
- Excel or similar graphing software (Microsoft)
Preparation of double-control plasmids for dc-qcnPCR
1.Prepare to add segment of CHOP autosomal genetic sequence to the AAVS1-Ef1HTLV-Gluc plasmid using blunt-end ligation.
2.Amplify the segment of CHOP genetic sequence from the gDNA of IMR90-4 cells, as described below. See Table 4 for PCR primer sequences.
- PCR reaction components:
Reagent | Amount |
5× GC Phusion buffer | 10 µl |
10 mM dNTPs | 1 µl |
10 µM hCHOP_F primer | 2.5 µl |
10 µM hCHOP_R primer | 2.5 µl |
2 U/µl Phusion polymerase | 0.5 µl |
gDNA | 10 ng |
H2O | Adjust volume to 50 µl |
Step | Duration | Temperature | Process |
1 | 180 sec | 98°C | (denaturation) |
35 cycles of: | 30 sec | 98°C | (denaturation) |
20 sec | 60°C | (annealing) | |
60 sec | 72°C | (extension) | |
1 | 600 sec | 72°C | (extension). |
3.Phosphorylate the PCR product by incubating the reaction described below at 37°C for 30 min.
Reagent | Amount (µl) |
PCR product | 20 |
10× T4 ligation buffer | 2 |
T4 polynucleotide kinase | 1 |
H2O | Adjust volume to 25 µl |
4.Digest the pAAVS1-Ef1HTLV-Gluc vector with a single-cutting blunt-end enzyme (PmlI) at 37°C for 15 min, according to the table below.
Reagent | Amount |
pAAVS1-Ef1HTLV-Gluc vector | 2 µg |
10× FastDigest buffer | 2 µl |
PmlI restriction enzyme | 1 µl |
H2O | Adjust volume to 20 µl |
5.Promptly dephosphorylate the digested vector by adding 1 µl Fast AP to the reaction. Incubate for another 15 min at 37°C.
6.Inactivate the reaction at 75°C for 5 min.
7.Run the digested and dephosphorylated vector (Basic Protocol 3, step 6) and the phosphorylated PCR product (Basic Protocol 3, step 3) on an agarose gel (1% agarose/TAE) at 100 V for 1 h, visualize with ethidium bromide (0.25 µl/ml), and precisely excise the desired products from the gel.
8.Purify the vector and amplicon with a gel clean-up kit, according to the manufacturer's instructions.
9.Ligate the pAAVS1-Ef1HTLV**-** Gluc vector and the gel-purified, phosphorylated CHOP sequence as described below, in a 3:1 insert to vector molar ratio. Incubate the reaction at 22°C for 1 hr and then inactivate at 65°C for 10 min.
Reagent | Amount |
Digested, dephosphorylated, gel-purified pAAVS1-Ef1HTLV-Gluc vector | 50 ng |
Phosphorylated PCR product | 4.6 ng |
10× T4 ligation buffer | 1 µl |
T4 DNA ligase | 1 µl |
H2O | Adjust volume to 10 µl |
10.Warm SOC medium to 37°C and place the transformation cuvette and guide on ice.
11.Add 1 µl ligation product to 50 µl electrocompetent XL1-Blue E. coli cells.
12.Immediately transfer ligation and E. coli mixture to the 2-mm electroporation cuvette.
13.Quickly slide the cuvette into the shocking chamber using the cold cuvette rack. Be sure to dry the cuvette and rack before pulsing the cells.
14.Apply an electrical field of 2.5 kV, with a capacitance of 25 µF and a time constant of 5 ms, to the competent cells in order to create pores in the cell walls through which the ligation product can pass.
15.Quickly add 1 ml warm SOC medium to the transformed cells, transfer to a 15-ml conical tube, and incubate the transformed cells while shaking at 37°C for 1 hr. Do not fully tighten the lid of the tube.
16.Working in a sterile laminar flow hood, spread 100 µl transformed cells on an LB agar plate (100 µg ampicillin/ml LB agar) using a sterile device. Incubate upside down at 37°C overnight.
17.Pick six to eight colonies using a sterile toothpick or pipet tip and place in LB medium with antibiotic (100 µg ampicillin/ml LB medium). Swirl, cover with a loosely attached sterile lid, and incubate overnight at 37°C while shaking.
18.Isolate plasmid DNA, now called “AAVS1-Ef1HTLV-Gluc-CHOP,” from the bacterial culture using a miniprep kit, following the manufacturer's instructions.
19.Check the successful insertion by digestion with a restriction enzyme that is found once in the original plasmid and once in the CHOP insert sequence (in this case either SmaI or SacI). Incubate the reaction at 37°C for 15 min.
Reagent | Amount |
pAAVS1-Ef1HTLV-Gluc-CHOP vector | 5 µl |
10× FastDigest buffer | 2 µl |
SacI restriction enzyme | 1 µl |
H2O | Adjust volume to 20 µl |
20.Run digested vector on an agarose gel (1% agarose/TAE) at 130 V for 1 hr and visualize with ethidium bromide (0.25 µl/ml).
21.Add a segment of genetic sequence found on the X chromosome to the AAVS1-Ef1HTLV-Gluc-CHOP plasmid using blunt-end ligation. This plasmid already contains the coding sequence of the GLuc segment of interest and CHOP autosomal control. Follow steps 2-17 above, using HPRT1 (PCR primers xHPRT1_F and xHPRT1_R) in place of CHOP (in step 2) and the following restriction enzyme and ligation mixtures. See Table 4 for PCR primer sequences.
- Vector restriction digestion:
Reagent | Amount |
pAAVS1-Ef1HTLV-Gluc-CHOP vector | 2 µg |
10× FastDigest buffer | 2 µl |
PsiI restriction enzyme | 1 µl |
H2O | Adjust volume to 20 µl |
Reagent | Amount |
Digested, dephosphorylated, gel-purified pAAVS1-Ef1HTLV-Gluc-CHOP vector | 50 ng |
Phosphorylated PCR product | 5.6 ng |
10× T4 ligation buffer | 1 µl |
T4 DNA ligase | 1 µl |
H2O | Adjust volume to 10 µl |
22.Isolate plasmid DNA, now called “AAVS1-Ef1HTLV-Gluc-CHOP-HPRT1” or “Control-HPRT1,” from the bacterial culture using a miniprep kit, following the manufacturer's instructions.
23.Check the successful insertion by digestion with a restriction enzyme whose recognition site is found once in the original plasmid and once in the HPRT1 insert sequence (SphI). Incubate the reaction at 37°C for 15 min.
Reagent | Amount |
pAAVS1-Ef1HTLV-Gluc-CHOP-HPRT1 vector | 5 µl |
10× FastDigest buffer | 2 µl |
SphI restriction enzyme | 1 µl |
H2O | Adjust volume to 20 µl |
24.Run digested vector on an agarose gel (1% agarose/TAE) at 130 V for 1 hr, visualizing with ethidium bromide (0.25 µl/ml).
Quantification of donor DNA integrations in genetically modified monoclonal cells (dc-qcnPCR)
25.When testing the dc-qcnPCR tool for the first time, it is a good idea to design a couple of plasmid controls with the same autosomal sequence, but varying ChrX sequences.
26.Dilute the control plasmid(s) designed in step 25 in a 1:10 dilution series of five samples, starting with a 1:1000 dilution.
27.Amplify each of the diluted plasmids with the primer sets for GLuc, CHOP , HPRT1 , and RBBP7 (see Table 4 for sequences and expected product sizes), as well as one exemplary gDNA extraction from a monoclonal cell line, according to the tables below using thin-wall 96-well skirted PCR plates with adhesive seals and a CFX Opus 96 Real-Time System.
- Reaction components:
Reagent | Amount |
2× SYBR Green Master Mix | 5 µl |
10 µM forward primer | 0.25 µl |
10 µM reverse primer | 0.25 µl |
Dilution of mini-prepped plasmid or 10 ng/µl gDNA | 1 µl |
H2O | Adjust volume to 10 µl |
Step | Duration (sec) | Temperature | Step |
1 | 600 sec | 95°C | (denaturation) |
40 cycles of: | 20 sec | 95°C | (denaturation) |
20 sec | 60°C | (annealing) | |
20 sec | 72°C | (extension) | |
1 | 10 sec | 95°C | (melting curve) |
Ramp-up: | 5 sec/step | 65°C to 95°C | (melting curve) |
28.Determine which plasmid dilution amplifies within the same cycle threshold (Ct) range as the exemplary 10 ng of gDNA. This dilution will be the control plasmid to include in all further dc-qcnPCR runs.
29.Prepare the qPCR plate to amplify four sets of sequences: GLuc , CHOP , HPRT1 , and RBBP7. For each of these sequences, include the control plasmids (Control-HPRT1 and Control-RBBP7), the samples under investigation, and non-template controls (see Fig. 6 for example plate layout).
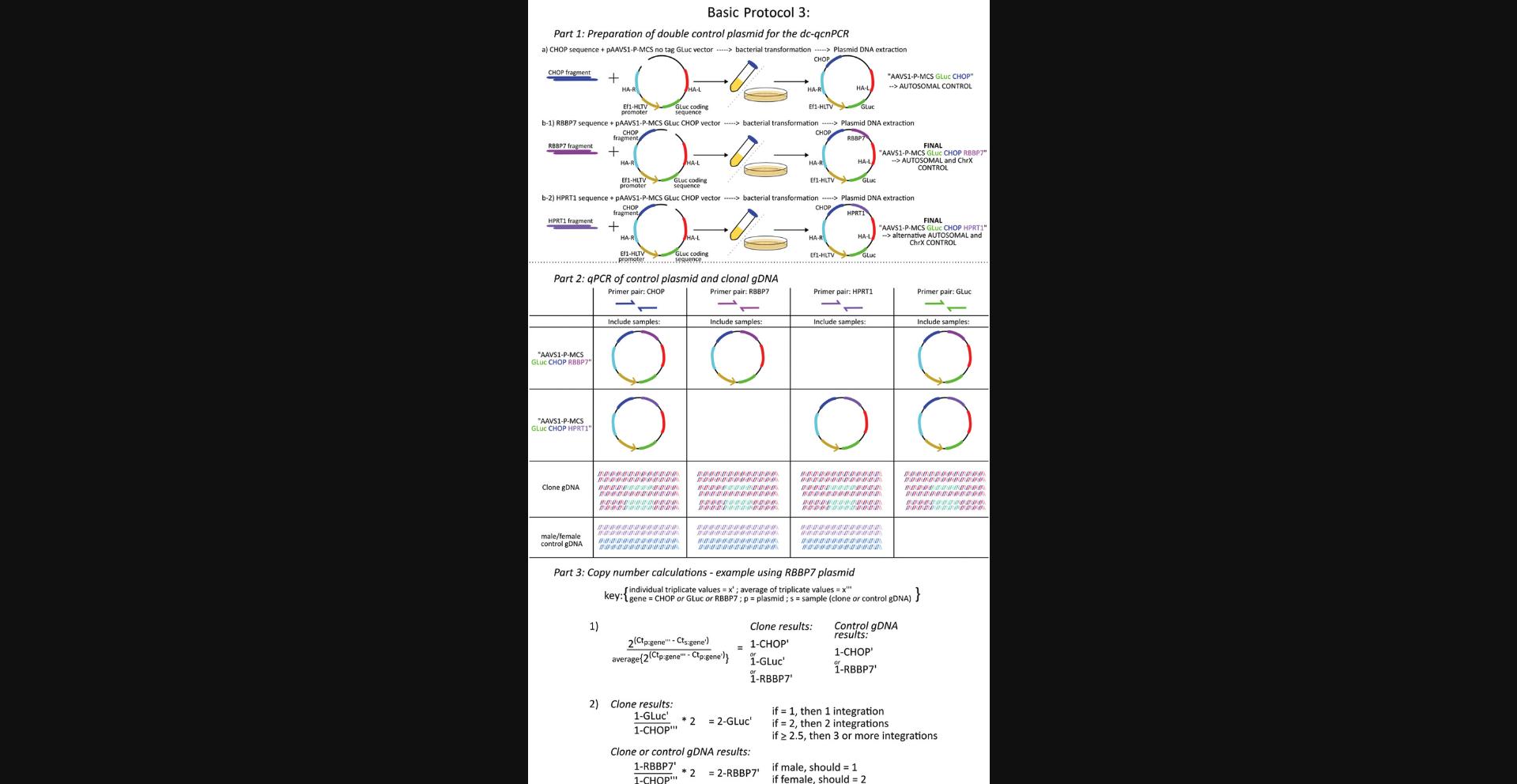
30.Run all samples in triplicate using the master mix and qPCR thermocycling program described in Basic Protocol 3, step 27.
31.Collect Ct values for each sample and sequence and normalize the sample amplification to the plasmid amplification within each gene. Primer efficiency was normalized by comparing each gene of interest with the same plasmid.
32.The normalization relative to CHOP designated which Ct value, within each sample, equals 2, because autosomal genes possess two alleles.
33.The calculations in Basic Protocol 3, steps 31 and 32, allow the primer efficiency of each primer set to be normalized by comparing each gene of interest within the same plasmid (equation 1). The normalization relative to CHOP designates which Ct value, within each sample, equals 2, because autosomal genes possess two alleles (equation 2). The normalization of RBBP7 to CHOP verifies that the ChrX gene equals 2 in females and 1 in males. Female and male gDNA should always be included as a positive control during qPCR analyses (Fig. 7).
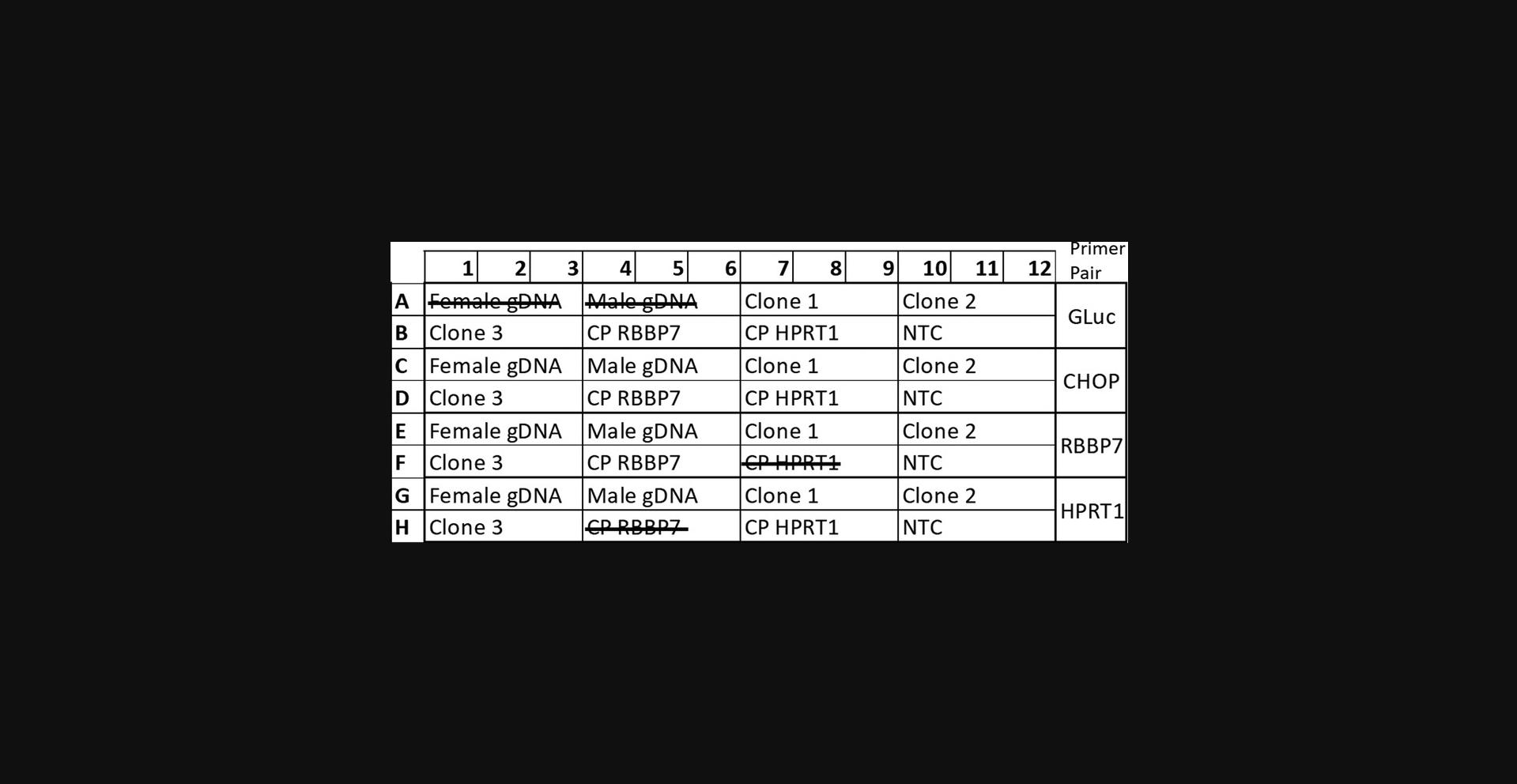
REAGENTS AND SOLUTIONS
4′,6-Diamidino-2-phenylindole dihydrochloride (DAPI; Roth, 6843) working solution (300 µM)
- DAPI stock: Resuspend 10 mg DAPI in 1 ml DMSO (DMSO, Roth, A994.1).
- DAPI working solution: Add 10.51 µl DAPI stock to 989.51 µl PBS (see recipe).
- Store both DAPI stock and working solution at –20°C in the dark. Solutions will remain stable for at least a year.
EDTA (0.5 mM)/PBS
- Mix:
- 14.6 mg ethylenediamine tetraacetic acid (EDTA; ≥99% p.a.; Roth, 8040.2)
- 100 ml PBS (see recipe)
- Sterile filter and store up to several months at 4°C.
Immunofluorescence blocking solution
- Mix:
- 5 ml fetal bovine serum (PAN Biotech, P30-3306)
- 1 g albumin fraction V (Roth, 8076.3)
- 250 µl Triton™X (Roth, 3051.2)
- PBS (see recipe) to 100 ml
- Sterile filter and store up to several months at 4°C.
Immunofluorescence permeabilization buffer (0.25% PBS-T)
- Mix:
- 250 µl Triton™X (Roth, 3051.2)
- 100 ml PBS (see recipe)
- Store up to several months at 4°C.
Luria broth (LB) ampicillin agar plates
- Mix:
- 32 g LB agar (Thermo Scientific, 22700041)
- H2O to 1000 ml
- Autoclave mixture. Remove the bottle while it is still hot and partially submerge in 60°C water bath. Allow the gel mix to cool until you can touch the bottle (∼60°C). Under a sterile hood, add 1 ml of 100 mg/ml sterile-filtered ampicillin and carefully swirl the bottle to mix. Carefully pour the liquid agar into the sterile petri dishes. Allow the dishes to solidify and dry (leave in the sterile hood overnight at room temperature). Cover the dishes and place in a plastic bag. The bag can be stored at 4°C for several months.
Luria broth (LB) medium, pH 7.3
- Mix:
- 5 g yeast extract (Roth, 2904.3)
- 10 g tryptone (Thermo Scientific, LP0042B)
- 10 g NaCl (Roth, HN00.2)
- H2O to 1000 ml
- Adjust pH to 7.3 using NaOH
- Sterilize solution by autoclaving
- Store up to several months at 4°C.
Mg2+ solution, 2 M (for SOC medium)
- 20.33 g MgCl2 (Roth, KK36.3)
- 12.4 g MgSO4 (Roth, P027.2)
- H2O adjust volume to 100 ml
- Sterile filter and store up to several months at room temperature.
Paraformaldehyde, pH 6.9, 4%
- Add 40 g paraformaldehyde (PFA; Roth, 0335.3) to ∼800 ml of PBS at 60°C. Do not allow the solution to boil. Slowly adjust the pH with NaOH while stirring. Once the PFA has dissolved, add PBS to 1000 ml, and finally adjust the pH with small amounts of HCl. Sterile filter and store up to several months at –20°C.
Phosphate-buffered saline (PBS) solution, pH 7.4
- Mix:
- 8 g NaCl (Roth, HN00.2)
- 201 mg KCl (Roth, P017.1)
- 1.42 g Na2HPO4 (Roth, T877.1)
- 1.58 g KH2PO4 (Roth, 3904.1)
- H2O adjust volume to 1000 ml
- Adjust pH to 7.4
- Sterilize by autoclaving
- Solution can be stored for several months at room temperature.
Super optimal broth (SOB) medium, pH 7.0 (for SOC medium)
- Mix:
- 20 g tryptone (Thermo Scientific, LP0042B)
- 5 g yeast extract (Roth, 2904.3)
- 584 mg NaCl (Roth, HN00.2)
- 201.3 mg KCl (Roth, P017.1)
- H2O to 1000 ml
- Adjust pH to 7.0 with NaOH and autoclave to sterilize
- Store up to several months at 4°C.
Super optimal broth with catabolite repression (SOC) medium
- Mix:
- 10 µl 2 M Mg2+ solution (see recipe)
- 3.6 mg glucose (Roth, X997.2)
- Add SOB medium (see recipe) to 1 ml
- Store up to 1 month at 4°C.
Tris-acetate EDTA buffer (TAE), 10×
- Mix:
- 60.57 g Tris base (Roth, 4855.2)
- 16.406 g sodium acetate (Roth, 6773.2)
- 5.845 g EDTA (Roth, 8040.2)
- H2O to 1000 ml
- Sterilize solution by autoclaving
- Store up to several months at room temperature.
COMMENTARY
Background Information
Genetic engineering is an essential technique in the molecular biologist's handbook, artificially creating situations that can be exploited to learn immeasurable amounts of information about cellular processes. The highly acclaimed and accurate CRISPR/Cas9 technology is joined by other important genetic engineering tools, such as rare-cutting meganucleases (Stoddard, 2014), TALENs (Bhardwaj & Nain, 2021) and ZFNs (Kim, Cha, & Chandrasegaran, 1996), as well as retroviruses or transposon vectors such as “Sleeping Beauty” and “piggybac,” which were derived from fish and insect systems, respectively, and optimized to generate genetically modified mammalian cell lines (Lanigan et al., 2020). However, despite the wide selection of methods and their growing acclaim, it is impossible to assume that these tools will produce only accurately modified cell lines (Hendel, Fine, Bao, & Porteus, 2015; Lanigan et al., 2020; Manghwar et al., 2020). If the aim of the genetic modification is to study the effects of modulated expression of the gene of interest under otherwise normal conditions, it is essential to verify that the genetic modification did not create unwanted new conditions through off-target transgene insertions. Therefore, it is necessary not only to confirm that the genetic modification took place as expected, but also to analyze the transgenic cells for off-target modifications.
Quantitative PCR has been shown to reliably monitor small changes in gene expression through the amplification of cDNA fragments (Arya et al., 2005). Therefore, the same instrument should also be capable of detecting small variations in gDNA copy number. Based on this hypothesis, we validated the sensitivity of the qPCR and developed the autosomal/ChrX double-control quantitative copy number PCR (dc-qcnPCR) to detect one, two, or more copies of a sequence of interest (Schjeide, Schenke, Seeger, & Püschel, 2022). The insert-confirmation PCR is an essential partner to the dc-qcnPCR. If the dc-qcnPCR detects only one copy of the transgene and the insert-confirmation PCR amplifies one wild-type (WT) allele and one transgene allele, the on-target insertion was heterozygous. If the dc-qcnPCR detects two copies of the transgene, but the insert-confirmation PCR again amplifies one WT allele and one transgene allele, this indicates there was one on-target insertion and one off-target insertion. On the other hand, if the insert-confirmation PCR amplifies only a transgene product, this means that the integration took place in both alleles and the two copies of the transgene are homozygous at the target locus. This test must be performed in a monoclonal cell population. A result of greater than two copies of the donor DNA clearly indicates off-target integrations; any result of two copies or less could indicate either homozygous or heterozygous integrations, but could also be due to an artificial reduction of copy number through contamination from unstably transfected cells. Therefore, after transfection, all cells should be subjected to monoclonal isolation. In Support Protocol 2, we describe how to confirm the expression of Gaussia luciferase in the clonal population using immunofluorescence. The addition of high-quality gDNA from female and male in the analysis, as well as our recommendation to create more than one control plasmid, also provide a general monitoring of the genomic health of the cell. The verification of the expected ratios of autosomal to sex chromosomes can confirm, at least in the inspected regions, the normal diploid state of the cell.
Various methods already exist to analyze off-target donor DNA integrations or to characterize modified cell lines. These methods offer some advantages, but also have their limitations. The Southern blot is a standard method designed to detect specific sequences in digested gDNA separated by gel electrophoresis (Southern, 1975). We found, however, that when using non-isotope-based strategies, the method was not sensitive enough to detect single copies of functionally verified Gaussia luciferase coding DNA (Schjeide et al., 2022). More recent advances to assess off-target modifications involve detection of potential Cas off-target inserts followed up by next-generation sequencing: for example, genome-wide, unbiased identification of DSBs enabled by sequencing (GUIDE-seq; Tsai et al., 2015); discovery of in situ Cas off-targets and verification by sequencing (DISCOVER-seq; Wienert et al., 2019); circularization for in vitro reporting of cleavage effects by sequencing (CIRCLE-seq; Tsai et al., 2017); and linear amplification-mediated high-throughput genome-wide translocation sequencing (LAM-HGTGTS; Frock et al., 2014). These methods offer the advantage of possibly detecting any mutations caused by DSBs at putative off-target Cas sites, and not only off-target transgene integrations (see next paragraph for the limitations of the dc-qcnPCR). However, they do not physically screen the whole genome for off-target integrations and also involve methods that may be technically and/or financially out of reach for many smaller laboratories. Furthermore, even if whole-genome sequencing (WGS) were to be utilized, not only would the researchers need enough computational power to analyze the resulting data, but the read length of most next-generation sequencing platforms would not allow the detection of multi-kilobase donor DNA segments between long homology arms (Hu, Chitnis, Monos, & Dinh, 2021). The most ideal method to analyze off-target effects would likely be long-read sequencing (Höijer et al., 2020), but this method is once again limited by specialized materials and computational burden. Other methods also taking advantage of PCR have been established to efficiently measure copy-number variation, such as the digital droplet PCR (Hindson et al., 2011) and dual-probe amplification assays (Mehrotra, 2016). Again, these methods have been shown to be useful, but the digital droplet PCR requires specific instruments for droplet generation and reading, which can be an expensive addition to the laboratory, and some dual-probe amplification systems create a dependency on the manufacturer for the number of reactions produced and require a new assay for each gene of interest.
The greatest advantages of the dc-qcnPCR tool are its simplicity and flexibility. The control plasmids can be prepared with multiple sequences of interest at a scale that will cover thousands of reactions. The method for confirming the transfection accuracy takes advantage of the same skills necessary to perform that transfection: the design and preparation of plasmids. Once the control plasmids are prepared, it is only necessary to organize all of the necessary controls along with the samples of interest on the qPCR plate. A limitation of this tool is that although it can be exploited to detect the specific target modification in the cells, as in the example detailed here involving the insertion of the coding sequence of Gaussia luciferase into the AAVS1 safe-harbor locus, it cannot detect off-target double-strand breaks that may have resulted in point mutations rather than integration of the donor DNA. Nonetheless, it has proven to be a relevant and useful tool for detecting off-target integrations, which, depending on cell type, have been shown to still occur frequently (Schjeide et al., 2022). The greatest benefit of this workflow is that each protocol takes advantage of techniques and devices that are commonly found in most standard molecular biology laboratories, or at least neighboring laboratories.
Critical Parameters
There are multiple critical parameters for each of the protocols presented here. In Basic Protocol 1, it is absolutely essential to work in a sterile environment and pay close attention to the IMR90-4 cells. For the most part, these iPSCs grow well on Matrigel-coated plates, but it is absolutely necessary to continuously monitor them for any signs of spontaneous differentiation—as evidenced by cells growing outside their usual round cluster (Fig. 8). Methods to rescue plates with partial spontaneous differentiation are noted in the Troubleshooting section. Differentiation can happen truly spontaneously, but it is also caused by cellular stress: be sure to regularly provide fresh medium and minimize handling of the cells. During the single-cell isolation, it is critical to remain patient. Regularly check each well of the 96-well plate to verify that surviving clones grew from only one source cell and to note each clone's speed of growth and health. Some clones will grow quickly and others more slowly. The timeline for the expansion of the monoclonal line is completely dependent on the individual clone. The extraction of genomic DNA from the monoclonal cell lines of interest must be of high quality. At the time of these experiments, centrifuging the empty DNA binding column of the Qiagen DNeasy Kit after the washing steps was not recommended. However, we observed that carrying out an extra drying centrifugation step greatly improved the A 260/A 280 ratio, indicating a purer gDNA extraction. The minimum acceptable A 260/A 280 ratio for the purpose of dc-qcnPCR is 1.7.
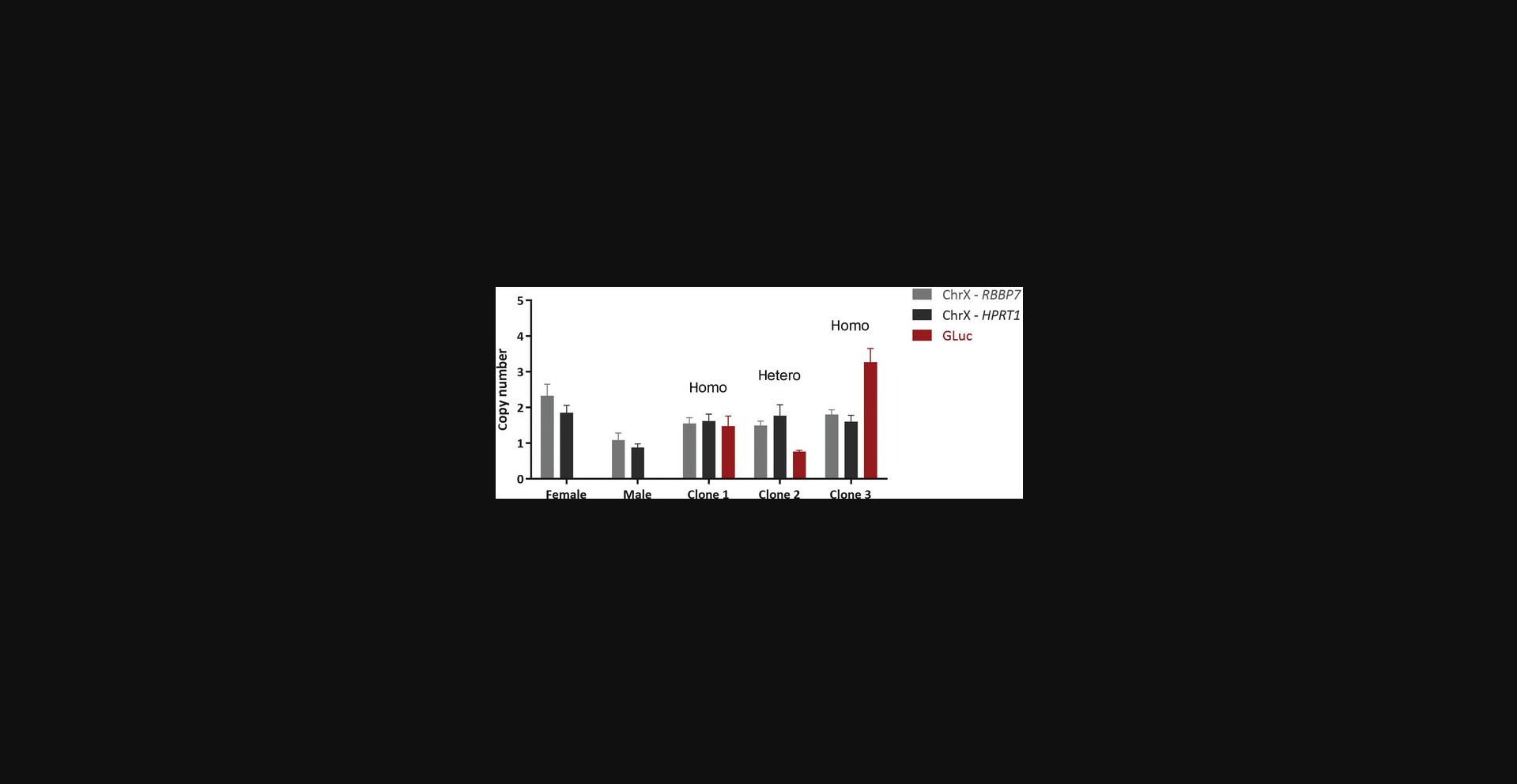
For Support Protocol 2, while inspecting the expression of the reporter gene by immunofluorescence, be sure to assess multiple growth areas on the coverslips. Because the cells grow in clusters (as seen in Fig. 3), it may be that some clusters originate from a clone expressing the reporter gene, while a neighboring cluster might originate from a non-reporter-gene-expressing cell. It is crucial to establish monoclonal cell lines, for both downstream functional experiments as well as the dc-qcnPCR.
In Basic Protocol 2, the design of the PCR primers is key to the functionality of the insert confirmation. The aim should be to amplify the entire transgene target region, including both full-length homology arms, in order to capture all possible modifications through HDR, as well as any unmodified, wild-type allele(s). In this example, despite extensive optimization, it was not possible to design primers that completely flanked both homology arms (Fig. 4A). It is necessary, however, to have at least one primer either 5′ or 3′ of the homology arm. In our case, the second primer was close to the flanking region, but within the right homology arm. As discussed in the Troubleshooting section, it may be necessary to design multiple amplicons covering the full homology arm/donor DNA sequence lengths. If multiple fragments must be amplified separately, the primer pairs must be designed so that the amplicons overlap each other. Furthermore, it is essential to include a primer set that will amplify the wild-type locus, in order to identify potential heterozygous insertions or completely unmodified alleles. It is good practice to sequence the insert area to confirm that the amplified region was not random.
For Basic Protocol 3, we found it important and helpful to include high-quality female and male gDNA in the qPCR analysis, which can be used to determine the copy number of the X chromosome control gene. The successful calculation of a copy number of 2 in the female gDNA and 1 in the male gDNA serves to verify the quality of the control plasmids and the proper calculation technique. Furthermore, we calculate the copy number of the ChrX gene in each genomic DNA extraction from each monoclonal cell lines in order to validate the quality of the gDNA extraction and to check for possible chromosomal abnormalities. In this example, the IMR90-4 cells were derived from a female donor, so the expected ChrX copy number was 2. It is especially useful to include the external female and male gDNA on the plate if the cell line of interest is derived from a female donor. In this case it is still possible to use the calculated ChrX copy number as a comparative tool, but it cannot be used to verify the difference of a single copy of the sequence of interest in the clonal gDNA.
Troubleshooting
See Table 5 for the commonly encountered problems, possible causes, and solutions.
Problem | Possible cause | Solution |
---|---|---|
The AAVS1-T2 oligonucleotides do not anneal | The antisense oligo was designed complimentary to the sense oligo, but was then converted into a reverse complement as if it were a reverse PCR primer | The sense oligo should be designed as 5′ to 3′; the antisense oligo should be complementary to the sense oligo, designed 3′ to 5′. Both oligos should be ordered exactly as designed. |
Very few colonies grow from the transformation of the ligation reaction between eSpCas9(1.1) linearized vector backbone and annealed AAVS1-T2 oligos | The ligation of short oligonucleotide fragments can be inefficient |
Add 2-3 µl of the ligation reaction between eSpCas9(1.1) linearized vector backbone and annealed AAVS1-T2 oligos. Extend the digestion time of the eSpCas9(1.1) vector up to 2 h to allow all vector copies to be fully digested. |
IMR90-4 cells do not die in selection medium | The cells are too confluent (>80% confluency) and are protecting each other from the puromycin | Split the transfected cells 1:4 and immediately resume with the selection medium. During splitting, be sure to include Y-27632 ROCK inhibitor (which enhances the survival of stem cells when they are dissociated to single cells), as in the standard protocol. |
IMR90-4 cells spontaneously differentiate | Cells were seeded too sparsely (<5%) | Adjust the splitting ratio to prevent cells from being forced to grow alone. |
Cells were dissociated for too long | Decrease dissociation time and resuspension intensity, allow the cells to remain in clumps of 3-10 cells. | |
Cells have become too confluent | Mark areas of differentiation, and while aspirating the EDTA-PBS solution, place the Pasteur pipet directly on these differentiated areas and remove them from the cell population. | |
Cells have been in culture too long | Start the culture over with a freshly thawed cryovial of cells. | |
gDNA extracted from monoclonal cell lines is not of high quality, which is essential for the dc-qcnPCR | Extraction quality is poor as a result of inefficient removal of residual contaminants | Add an extra centrifugation step (15,000 × g for 2 min) after the last column washing step. |
Insert-confirmation PCR: Unsuccessful amplification of area surrounding the homology arms | The insert-confirmation PCR will generally involve a long amplification target, as it should include the amplification of the inserted donor DNA, which can be several kilobase pairs long, as well as the full sequences of both the right and left homology arms |
Include DMSO, Q, or Betaine in the PCR reaction. Use a temperature gradient to optimize for reaction annealing temperature. Design and test multiple primer pairs for a greater range of flexibility. Increase the duration of the extension step for longer ranges. Use DNA polymerase especially designed for long PCR. Design a combination of amplification areas that overlap and cover the entire homology arm/donor DNA area. At least two of the amplicons must contain an internal primer within the donor DNA and an external primer flanking the homology arm sequence. In this case, additional primers must be designed to amplify WT DNA in order to detect unmodified alleles. |
After carrying out the dc-qcnPCR calculation in Basic Protocol 3, step 31 and 32, the ChrX copy number ratio of the female/male sample is not exactly 2 or 1; small variations in the expected results can lead to confusion about their interpretation | Possibly due to accessibility of surrounding genomic sequence of the amplified fragments |
Consider creating new control plasmid with the autosomal and ChrX fragments in different chromosomal localization. If the dc-qcnPCR is prepared using genomic fragments not discussed in this protocol, it may be necessary to optimize both the autosomal genomic sequence as well as the ChrX sequence. Choose gene segments that have been shown in your lab to be easily amplified. Prepare the dc-qcnPCR control plasmids as described in Basic Protocol 3, so that they include your donor DNA, the sequence of an autosomal fragment, and the sequence of a ChrX fragment. First run a proof-of-concept experiment with the new control plasmid to verify that the difference between copy number of the ChrX sequence can be accurately determined in high-quality genomic DNA from male and female donors. Once verified, the new control plasmid can be used as described in Basic Protocol 3. |
The melting curve of the dc-qcnPCR has more than one peak | Possible nonspecific amplification products are being coamplified during the reaction |
Verify the amplification of the sample(s) by agarose gel electrophoresis. If multiple products are identified on the gel, either re-determine the optimal and specific annealing temperature for your existing primers or redesign your primers. |
The calculation of ChrX copy number results in 1 for females and 0.5 for males | This result is likely due to the calculation of the quotient of autosomal to ChrX copy number | Multiply the results by 2 (the known copy number of the autosomal sequence). |
Understanding Results
In Basic Protocol 1, one's ability to isolate the stably transfected clones can improve with time and practice. The first transfection carried out in our group resulted in the isolation of ∼15 monoclonal cell lines; after the fifth transfection, we were able to isolate up to 50 monoclonal cell lines. The key is to develop a feeling for the cells and always to be available to rescue a clone that may, for example, be growing faster than expected.
Support Protocols 1 and 2 are control steps to validate (1) that the cells you are spending so much time caring for are indeed expressing your protein of interest and (2) that individual differences in transfection between individual clones do not become a disturbing factor in the dc-qcnPCR (establishment of the monoclonal cell line). As illustrated in Figure 4, the overlay of the DAPI channel with the fluorescence channel of interest can expose which cells are indeed expressing the protein of interest, and which are not. It is imperative to visually inspect all areas on the cover slip including the cells in order to exclude a possible polyclonal population.
As described in Basic Protocol 2, this test amplifies the area flanking the target locus. Based upon the PCR fragment sizes, it is possible to assign three different categories: no insertion, heterozygous insertion, or homozygous insertion (5). The knowledge gained from the insert-confirmation PCR is necessary to define the type of insertion in a clone that was determined to have a copy number of 2. The copy number information alone could mean either (1) that the clone has two off-target transgene insertions, (2) that the clone has one off-target and one on-target insertion, or (3) the clone has a homozygous transgene insertion at the locus of interest. If the insert-confirmation PCR results in the amplification of only wild-type alleles, this points to case (1); if it results in the amplification of one wild-type allele and one transgene allele, this points to case (2); and if it results in the amplification of only the transgene allele, this points to case (3). The same rationale can be used if the dc-qcnPCR determines the clone has a copy number of 1. This indicates that a heterozygous insertion has been made, and with the help of the insert-confirmation PCR, it can be determined if this was an on- or off-target insertion.
In order to interpret the results of the dc-qcnPCR, it is essential to understand the underlying concept. The normalization of the primer efficiency and the concentration of the samples, as well as the “definition” of what is copy number n = 1, are important factors to consider in the attempt to quantify unknown copy numbers. To assist with the first two factors, the control plasmids are constructed to contain an autosomal gene sequence, the genetic sequence for one of the ChrX genes, and the sequence of interest. This plasmid can be included and amplified with each primer pair, alongside any clonal gDNA, to normalize both primer efficiency and any pipetting errors. Once the amplification of each product has been normalized between genes and samples (Basic Protocol 3, step 31, equation 1), it is necessary to define the copy number. We know that in diploid cells, the copy number of autosomal genes is n = 2, and the copy number of sex-linked genes is n = 2 in females or n = 1 in males. Therefore, we can translate the abstract result of equation 1 (the normalized amplification of the genetic segment of the sample) into the specifically defined result of equation 2 (Basic Protocol 3, step 32) by dividing the normalized amplification of GLuc by the normalized amplification of the autosomal gene (CHOP). This provides a ratio of unknown:CHOP copy number (x :2). This quotient remains slightly abstract: if it equals 1, that actually means that the copy-number ratio between GLuc and CHOP was 2:2. Therefore, we recommend multiplying the quotient by 2 to calculate the actual copy number of GLuc in the samples (Fig. 7). For each sample, we recommend calculating the copy number of the ChrX gene included in the plasmid control in order to verify the functionality of assay: in female-derived cells, the ChrX gene should have a copy number of 2, whereas in male-derived cells, the ChrX gene should have a copy number of 1.
Using each of the basic and support protocols, it is possible to detect single copy number differences in stably integrated transgenes and to establish if each clone of interest contains only on-target heterozygous or homozygous integrations, or if any off-target transgene integrations exist in the genome. Specifically, the combined knowledge of the insert-confirmation PCR (Fig. 4B) and the dc-qcnPCR (Fig. 7) can now be used to define the transgene status of the three example clones. Figure 4B shows that clones 1 and 3 both have homozygous insertions of the donor DNA into the AAVS1 safe harbor, while clone 2 has the correct insertion in one allele, but still retains one wild-type allele. In Figure 7, we can see that the copy number of clone 1 is 2, the copy number of clone 2 is 1, and the copy number of clone 3 is at least 3. Therefore, we can conclude that clone 1 has homozygous insertions at the AAVS1 safe harbor with no off-target donor DNA integrations, and clone 2 has a heterozygous insertion at the AAVS1 safe harbor with no off-target donor DNA integrations. Clone 3, on the other hand, does have a homozygous insertion of the donor DNA at the AAVS1 safe harbor, but also has at least one off-target donor DNA insertion. Therefore clone 3 would be excluded from further experiments.
Time Considerations
Basic Protocol 1 will take a minimum of 6 weeks to carry out, and only if certain steps are carried out in parallel. The protocol can also take up to 3 months from start to finish. The IMR90-4 cells can be brought into culture while the vectors for the double transfection are being prepared. However, it is important that the iPSCs not be cultured for >2-3 weeks before the transfection in order to keep the number of passages as low as possible. Ideally the cells should be passaged no more than three or four times before transfection. If spontaneous differentiation (see Fig. 8) occurs regularly, fresh cells should be thawed and brought into culture for the transfection. The preparation of the Cas9 expression vector and the AAVS1 homology arm vector will take at least 1 week. After transfection, the IMR90-4 cells that are stably expressing the gene of interest should be selected within 7-10 days. The bulk of the time necessary for this protocol is for the monoclonal isolation. Careful attention must be paid to each clone, and the state of each clone will be unique; therefore, it is necessary to multitask. Some clones will be ready for expansion within only a couple of weeks of the preparation of the single-cell dilution, while others will take at least a month to reach the same stage. During this time, several hours should be reserved each day for inspecting the clones under the cell culture microscope and for changing medium or expanding the cells.
As each monoclonal cell line grows enough to be transferred to the 6-well plate, Support Protocols 1 and 2 can be carried out immediately on those cells. Cells can be set aside and lysed in order to test for reporter protein expression. This step takes very little time and is essential to verify successful the stable transfection of the reporter gene. It is also essential to verify that the cells that are being expanded are indeed monoclonal. The immunofluorescence protocol takes 2 days to carry out, with one overnight incubation step.
The optimization of the insert-confirmation PCR will likely take the most time for Basic Protocol 2, especially if the amplification area is tricky. If optimization is needed to successfully amplify the area of interest, it may be necessary to run several temperature gradients or redesign primer pairs, which can take several days, including delivery times. However, once the PCR is optimized, the protocol will only take 1 day to carry out, as it is only necessary to prepare and run the PCR and then load and run the agarose gel.
For Basic Protocol 3, the largest proportion of time will be needed to prepare the control vectors for the dc-qcnPCR. The vectors can, however, be prepared within 1 week if a good plan is made, because the protocol includes multiple overnight incubation steps. Once the control vectors have been prepared and the vector dilution has been optimized, the dc-qcnPCR itself takes very little time to carry out. The qPCR must be well thought-out and organized, but after that it is only a matter of pipetting and running the qPCR. The exact duration of the experiment is dependent on the number of clones that need to be analyzed, and therefore the number of 96-well plates that need to be prepared. The qPCR itself runs ∼2 hr. The calculations of the copy number can be streamlined with the preparation of template Excel sheet, so that the Ct values of each qPCR run only need to be copied into the correct cells and the values will be automatically calculated.
Therefore, once the cloning process is finished and the monoclonal cell lines are established, it can take as few as 1-2 days to carry out the insert-confirmation PCR and the dc-qcnPCR in order to determine whether the clones contain on-target heterozygous or homozygous inserts, or if any off-target integrations may affect the cell lines.
Acknowledgments
This research was funded by a grant from the German Federal Ministry of Education and Research, 031L0132A/B.
Open access funding enabled and organized by Projekt DEAL.
Author Contributions
Brit-Maren Schjeide : Conceptualization, data curation, formal analysis, investigation, methodology, validation, visualization, writing—original draft. Gerhard Püschel : Formal analysis, funding acquisition, methodology, project administration, resources, supervision, writing—review editing
Conflict of Interest
The authors declare no conflict of interest. The funders had no role in the design of the study; in the collection, analyses, or interpretation of data; in the writing of the manuscript; or in the decision to publish the results.
Open Research
Data Availability Statement
The data and tools that support the protocol are available from the corresponding author upon reasonable request.
Literature Cited
- Arya, M., Shergill, I. S., Williamson, M., Gommersall, L., Arya, N., & Patel, H. R. H. (2005). Basic principles of real-time quantitative PCR. Expert Review of Molecular Diagnostics , 5(2), 209–219. doi: 10.1586/14737159.5.2.209
- Bajpai, R., Lesperance, J., Kim, M., & Terskikh, A. V. (2008). Efficient propagation of single cells Accutase-dissociated human embryonic stem cells. Molecular Reproduction and Development , 75(5), 818–827. doi: 10.1002/mrd.20809
- Bhardwaj, A., & Nain, V. (2021). TALENs-an indispensable tool in the era of CRISPR: A mini review. Journal, Genetic Engineering & Biotechnology, 19(1), 125. doi: 10.1186/s43141-021-00225-z
- Folger, K. R., Wong, E. A., Wahl, G., & Capecchi, M. R. (1982). Patterns of integration of DNA microinjected into cultured mammalian cells: Evidence for homologous recombination between injected plasmid DNA molecules. Molecular and Cellular Biology , 2, 16.
- Frock, R. L., Hu, J., Meyers, R. M., Ho, Y.-J., Kii, E., & Alt, F. W. (2014). Genome-wide detection of DNA double-stranded breaks induced by engineered nucleases. Nature Biotechnology , 33(2), 179–186. doi: 10.1038/nbt.3101
- Hendel, A., Fine, E. J., Bao, G., & Porteus, M. H. (2015). Quantifying on- and off-target genome editing. Trends in Biotechnology , 33(2), 132–140. doi: 10.1016/j.tibtech.2014.12.001
- Hindson, B. J., Ness, K. D., Masquelier, D. A., Belgrader, P., Heredia, N. J., Makarewicz, A. J., … Colston, B. W. (2011). High-throughput droplet digital PCR system for absolute quantitation of DNA copy number. Analytical Chemistry , 83(22), 8604–8610. doi: 10.1021/ac202028g
- Höijer, I., Johansson, J., Gudmundsson, S., Chin, C.-S., Bunikis, I., Häggqvist, S., … Ameur, A. (2020). Amplification-free long-read sequencing reveals unforeseen CRISPR-Cas9 off-target activity. Genome Biology , 21(1), 290. doi: 10.1186/s13059-020-02206-w
- Hu, T., Chitnis, N., Monos, D., & Dinh, A. (2021). Next-generation sequencing technologies: An overview. Human Immunology , 82(11), 801–811. doi: 10.1016/j.humimm.2021.02.012
- Kim, Y. G., Cha, J., & Chandrasegaran, S. (1996). Hybrid restriction enzymes: Zinc finger fusions to Fok I cleavage domain. Proceedings of the National Academy of Sciences of the United States of America , 93(3), 1156–1160. doi: 10.1073/pnas.93.3.1156
- Lanigan, T. M., Kopera, H. C., & Saunders, T. L. (2020). Principles of genetic engineering. Genes , 11(3), 291. doi: 10.3390/genes11030291
- Manghwar, H., Li, B., Ding, X., Hussain, A., Lindsey, K., Zhang, X., & Jin, S. (2020). CRISPR/Cas systems in genome editing: Methodologies and tools for sgRNA design, off-target evaluation, and strategies to mitigate off-target effects. Advanced Science , 7(6), 1902312. doi: 10.1002/advs.201902312
- McInerney, P., Adams, P., & Hadi, M. Z. (2014). Error rate comparison during polymerase chain reaction by DNA polymerase. Molecular Biology International , 2014, 287430. doi: 10.1155/2014/287430
- Mehrotra, M. (2016). PCR-based detection of DNA copy number variation. In Methods in Molecular Biology , 1392, 27–32. doi:10.1007/978-1-4939-3360-0_3
- Oceguera-Yanez, F., Kim, S.-I., Matsumoto, T., Tan, G. W., Xiang, L., Hatani, T., & Woltjen, K. (2016). Engineering the AAVS1 locus for consistent and scalable transgene expression in human iPSCs and their differentiated derivatives. Methods , 101, 43–55. doi: 10.1016/j.ymeth.2015.12.012
- Schjeide, B.-M. M., Schenke, M., Seeger, B., & Püschel, G. P. (2022). Validation of a novel double control quantitative copy number PCR method to quantify off-target transgene integration after CRISPR-induced DNA modification. Methods and Protocols , 5(3), 43. doi: 10.3390/mps5030043
- Slaymaker, I. M., Gao, L., Zetsche, B., Scott, D. A., Yan, W. X., & Zhang, F. (2016). Rationally engineered Cas9 nucleases with improved specificity. Science , 351(6268), 84–88. doi: 10.1126/science.aad5227
- Southern, E. M. (1975). Detection of specific sequences among DNA fragments separated by gel electrophoresis. Journal of Molecular Biology , 98(3), 503–517. doi: 10.1016/S0022-2836(75)80083-0
- Stoddard, B. L. (2014). Homing endonucleases from mobile group I introns: Discovery to genome engineering. Mobile DNA , 5(1), 7. doi: 10.1186/1759-8753-5-7
- Totonchi, M., Taei, A., Seifinejad, A., Tabebordbar, M., Rassouli, H., Farrokhi, A., … Baharvand, H. (2010). Feeder- and serum-free establishment and expansion of human induced pluripotent stem cells. International Journal of Developmental Biology , 54(5), 877–886. doi: 10.1387/ijdb.092903mt
- Tsai, S. Q., Nguyen, N. T., Malagon-Lopez, J., Topkar, V. V., Aryee, M. J., & Joung, J. K. (2017). CIRCLE-seq: A highly sensitive in vitro screen for genome-wide CRISPR–Cas9 nuclease off-targets. Nature Methods , 14(6), 607–614. doi: 10.1038/nmeth.4278
- Tsai, S. Q., Zheng, Z., Nguyen, N. T., Liebers, M., Topkar, V. V., Thapar, V., … Joung, J. K. (2015). GUIDE-seq enables genome-wide profiling of off-target cleavage by CRISPR-Cas nucleases. Nature Biotechnology , 33(2), 187–197. doi: 10.1038/nbt.3117
- Waddington, S. N., Privolizzi, R., Karda, R., & O'Neill, H. C. (2016). A broad overview and review of CRISPR-Cas technology and stem cells. Current Stem Cell Reports , 2(1), 9–20. doi: 10.1007/s40778-016-0037-5
- Watanabe, K., Ueno, M., Kamiya, D., Nishiyama, A., Matsumura, M., Wataya, T., … Sasai, Y. (2007). A ROCK inhibitor permits survival of dissociated human embryonic stem cells. Nature Biotechnology , 25(6), 681–686. doi: 10.1038/nbt1310
- Wienert, B., Wyman, S. K., Richardson, C. D., Yeh, C. D., Akcakaya, P., Porritt, M. J., … Corn, J. E. (2019). Unbiased detection of CRISPR off-targets in vivo using DISCOVER-Seq. Science , 364(6437), 286–289. doi: 10.1126/science.aav9023
- Yu, J., Vodyanik, M. A., Smuga-Otto, K., Antosiewicz-Bourget, J., Frane, J. L., Tian, S., & Thomson, J. A. (2007). Induced pluripotent stem cell lines derived from human somatic cells. Science , 318(5858), 1917–1920. doi: 10.1126/science.1151526