An Optimized Method of Collecting Murine Peripheral Blood and Dilution Correction for Accurate Blood Cell Enumeration
Vijay Bhoopalan, Vijay Bhoopalan, Elizabeth E. Gardiner, Elizabeth E. Gardiner, Amandeep Kaur, Amandeep Kaur
Abstract
Accurate measurement of whole blood counts from mice is an essential quantitative tool across the fields of vascular cell biology. In particular, the measurement of platelet counts can be challenging as the process relies upon good phlebotomy technique, the inclusion of a sufficient amount of the appropriate anticoagulant, and very often dilution of the sample to meet the sample volume requirements of an automated analyzer. To minimize sample dilution, blood collection tubes pre-coated with the anticoagulant can be used; however, these are expensive and prone to blood clotting issues. Here, we describe a simple dilution correction method that accurately calculates blood-to-anticoagulant dilutions to generate appropriate volumes for automated blood cell analysis while minimizing blood clotting. We also discuss some simple steps that can be incorporated into blood collection methods to avoid artefacts during blood collection. Blood count data analysis involving volume correction and clot exclusion can significantly reduce variable blood cell count values among healthy untreated littermates. It also detects subtle changes in blood cell counts, mainly of platelets and RBCs in experimental settings, which can be masked in the absence of careful and precise volume correction. Blood count analysis with volume correction precisely determines mouse whole blood cell counts for investigators. The decreased variability in cell count values reduces the number of experimental animals required for meaningful analysis. © 2023 The Authors. Current Protocols published by Wiley Periodicals LLC.
Basic Protocol : An optimized method of collecting murine peripheral blood and dilution correction for accurate blood cell enumeration
This article was corrected on 19 February 2024. See the end of the full text for details.
INTRODUCTION
Platelets are the smallest and second most abundant cells in blood after red blood cells. Platelets have a primary role in preserving blood volume and maintaining hemostasis, which they achieve by rapidly forming platelet aggregates (thrombi) that seal off areas damaged by physical trauma to the vasculature and begin the wound repair process (Rayes & Jenne, 2021). As innate immune cell entities, platelet function is crucial for mounting systemic immune responses to inflammation and infection (Li et al., 2017; Mandel et al., 2022).
Mouse models have been used extensively to elevate our understanding of the mechanisms of thrombopoiesis (platelet production) and multiplex functions of platelets under physiological and pathological conditions. These mouse models represent an integral approach that underpins platelet and thrombosis research (Bourrienne et al., 2022; Han et al., 2022; Palacios-Acedo et al., 2020; Ware, 2022). Several excellent publications describe in great detail about the care and handling of laboratory mice (Donovan & Brown, 2013), as well as blood collection methods (Ahrens Kress et al., 2022; Aurbach et al., 2019; Parasuraman et al., 2010; Yang et al., 2019); however, these methods rely upon the use of skilled blood collection techniques such as retro-orbital bleeding or cardiac puncture that requires anesthesia as well as the use of specialized pre-coated anticoagulant tubes to avoid blood dilution errors.
Accuracy in the quantitation of circulating cells in peripheral blood (cell counts) is of paramount importance as an experimental readout and a verifier of on- and off-target effects of a drug or treatment. Platelet numbers and properties are usually tightly controlled (Grozovsky et al., 2015); therefore, subtle alterations to these levels and platelet function are helpful and informative parameters critical in mouse models of thrombosis, thrombocytopenia, and thrombocytosis. Studying platelets isolated from mouse models is integral to platelet research; however, platelets are sensitive to mechanical stresses implicated during blood collection and can lead to platelet activation, aggregation, and/or blood clot formation. Activated platelets also interfere with platelet function studies. Both blood clots and activated platelets engage other blood cell types, such as red and white blood cells, leading to inaccuracies in estimating cell numbers. To ensure robust reproducibility and consistency of blood cell data, the initial steps of blood collection, choice of anticoagulation, and subsequent laboratory handling of blood are highly important and should be standardized within a laboratory. We have found that the reproducibility and repeatability of our blood cell data improved significantly through the deployment of carefully planned initial steps of blood collection, anticoagulant preparation, accurate calculation of the amount of blood collected, and post-collection sample handling.
Retro-orbital blood collection from mice is routinely performed to avoid inadvertent platelet activation due to shear stress. This mode of collection uses anticoagulant-coated capillaries to minimize blood dilution. Although shear stress is not a significant concern in retro-orbital blood collection, repeated sampling at this collection site can only be performed after 2 weeks, which carries significant implications for a study design that requires blood collection at shorter intervals. Only very small volumes of blood can be collected, meaning platelet function studies are not normally possible. Cardiac puncture is another method of blood collection that uses 27-G needles and syringes filled with an anticoagulant; however, this approach exposes blood platelets to elevated shear stress and enhances the possibility of platelet activation (Rana et al., 2019) and subsequent clot formations, thus skewing the blood cell and platelet count data (Hoggatt et al., 2016). However, collecting blood from a tail vein snip into a vial containing anticoagulant overcomes the drawback of exposure to shear stress and offers multiple blood collections. This method does not enable the collection of an accurate blood volume. It introduces variability in blood dilution ratios after mixing with a standard volume of anticoagulant across samples, leading to inaccurate blood dilutions. Here, we describe a simple method to collect mouse tail vein blood, overcome inconsistencies in blood dilutions, and obtain accurate blood cell counts using an automated blood analyzer. Although the method described here relates to tail bleeding, the sample handling technique is applicable across various blood collection methods. Finally, we describe some experimental approaches using this protocol in mice where thrombocytopenia has been induced by carboplatin treatment. We use fluorescently labeled antibodies to evaluate platelet surface protein properties in control and carboplatin-treated mice. Antibodies and carboplatin are included in the materials list but are not required for the mouse blood collection protocol being described. Rather, these reagents are used to illustrate the utility of this protocol in mice where platelet counts are severely diminished.
NOTE : All protocols involving animals must be reviewed and approved by the appropriate Animal Care and Use Committee and must follow regulations for the care and use of laboratory animals.
Basic Protocol: AN OPTIMIZED METHOD OF COLLECTING MURINE PERIPHERAL BLOOD AND DILUTION CORRECTION FOR ACCURATE BLOOD CELL ENUMERATION
Tail vein blood collection is a standard non-terminal procedure frequently used in longitudinal studies involving rodents. This method is suitable for collecting small blood volumes (20 to 200 μl) and ideal for repeated blood collection within short intervals (2 to 3 days). Mouse blood collection via tail vein sampling into the anticoagulant solution often leads to variable blood dilutions, which are difficult to resolve and adjust due to small volumes of samples. Although one way might be to add a precisely measured blood volume to a known volume of anticoagulant, this is cumbersome, time-consuming, prone to errors, and, if using pipette aspiration, can induce inadvertent platelet activation through fluid shear stress caused by pipetting blood through a narrow bore tip. Shear-induced activation can trigger the coagulation cascade and platelet activation, resulting in a significant reduction in the cellular components of the blood sample and sample loss. Planned functional studies are also dramatically affected. Hence, we propose an easy, quick, and accurate sample collection method with dilution correction involving precise weighing of the collected material, which can be adapted to any laboratory animal blood collection mode.
Materials
-
100 mg/kg carboplatin solution (cat. no. C2538, Sigma-Aldrich; see recipe; not essential for blood collection)
-
Mice of interest (C57BL/6 mice for this study)
-
0.5 M ethylenediaminetetraacetic acid (EDTA; pH 7.4; cat no. 0215252290, MP Biomedicals; see recipe)
-
1× phosphate-buffered saline (PBS; pH 7.4; cat no. D8537, Sigma-Aldrich; see recipe**)**
-
Fluorescein isothiocyanate (FITC) conjugated anti-mouse CD41 (cat. no. 553848, BD Bioscience; not essential for blood collection)
-
Allophycocyanin (APC) conjugated anti-mouse P-Selectin (cat. no. 148304, Biolegend; not essential for blood collection)
-
Phycoerythrin (PE) conjugated anti-mouse active-αIIbβ3 JONA (cat. no. M023-2, Emfret Analytics; not essential for blood collection)
-
Appropriate PPE (lab coat, gloves, surgical mask, and safety goggles)
-
500-μl centrifuge tubes (#AXYPCR-05-C, Axygen)
-
Analytical electronic weighing balance
-
Winged infusion sets butterfly scalp vein 23-G × 3/4″ short needles (Terumo Surflo)
-
Mechanical restraint device (#NC1272190, Fisher Scientific)
-
Heat source (heat lamp or heating pad)
-
200-µl pipette tips cut approximately 1 cm from the tip
-
Automated blood analyzer (ADVIA 2120 Hematology systems, Siemens)
Preparation of blood collection tubes for tail bleed
1.Coat a 500-µl centrifuge tube with EDTA solution by repeatedly dispensing and aspirating roughly 600 µl of 0.5 M EDTA solution into the tube five to six times (Fig. 1).
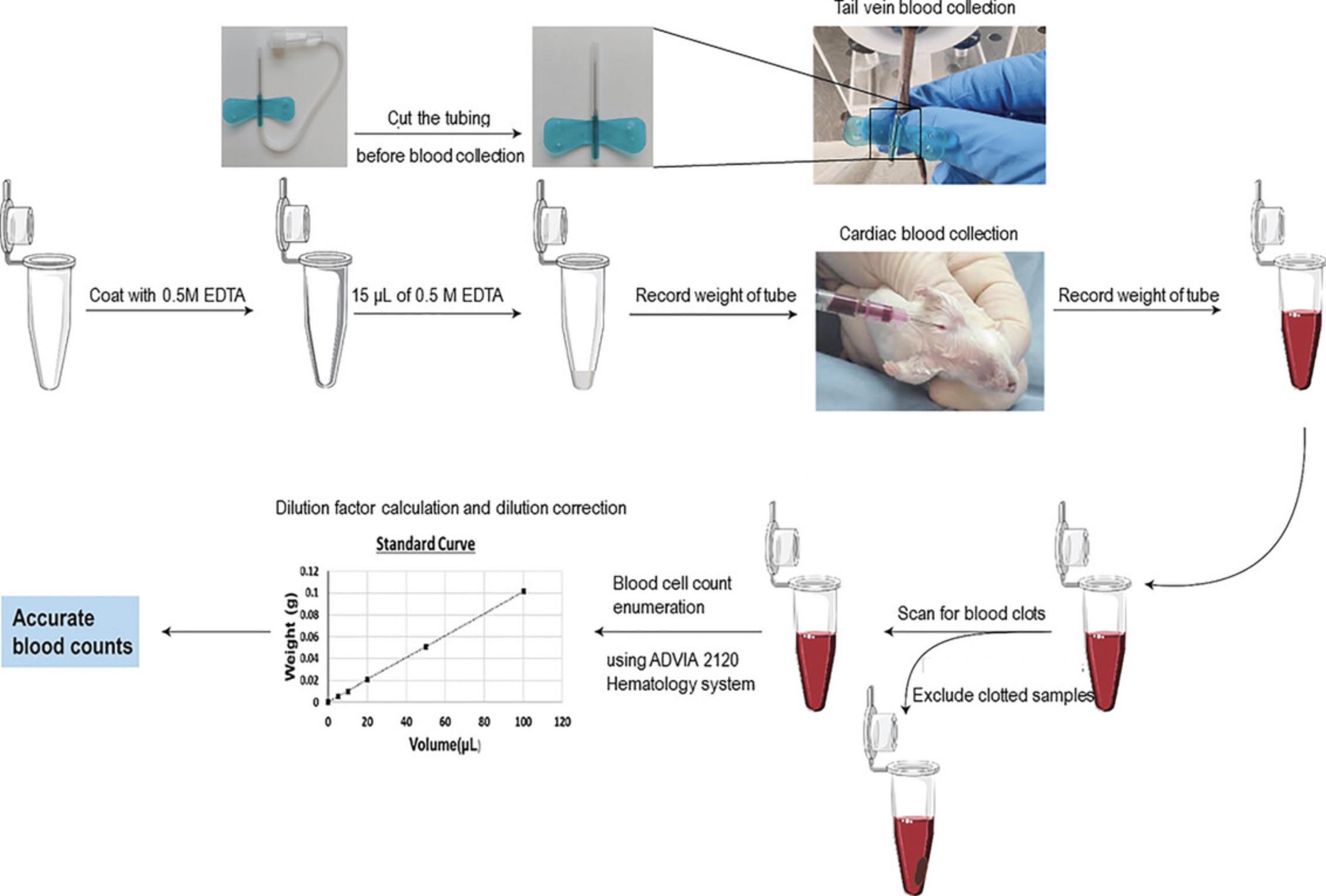
2.Add 15 μl of 0.5 M EDTA to each pre-coated microcentrifuge tube.
3.Record the weight of all the microcentrifuge tubes containing EDTA using an electronic weighing balance.
4.Cut the tubing attached to 23-G butterfly needles and have the needles ready for blood collection.
Blood collection by tail vein bleed
5.Place the mice in a cage and expose them to a warming lamp for 1 to 2 min to increase the visibility of the tail vein.
6.Place a mouse in a mechanical restrainer with the tail extending out.
7.Locate one of the two lateral tail veins in the middle third of the ventral tail. Carefully insert a 23-G butterfly needle into the tail vein (Liu et al., 2019).
8.Discard the first drop of blood and collect approximately 5 to 6 drops (∼45 to 60 μl) of blood into a labeled pre-weighed anticoagulant tube.
9.Gently tap and invert the tube to ensure blood mixes with the anticoagulant.
10.Use clean tissues to apply pressure to the puncture site for about a minute and ensure the bleeding stops before returning the mouse to the cage.
11.Reweigh and record the weight of the microcentrifuge tubes containing anticoagulated blood after blood collection.
Standard curve for blood dilutions and weights
12.For each tube, subtract the weight before drawing blood from the weight after drawing blood. This is the weight of the blood in each tube.
13.Prepare a standard curve of the sample blood weight versus the volume of blood. To do this, add various known volumes (15 to 150 µl) of anticoagulated blood into 0.5-ml vials and record each weight using an analytical weighing balance. The standard curve needs to be generated only once per mouse strain and used for correcting blood dilution.
14.Plot the weight versus volume data using a graphing program (e.g., Prism GraphPad or MS Excel) to obtain a standard curve, as shown in Figure 2.
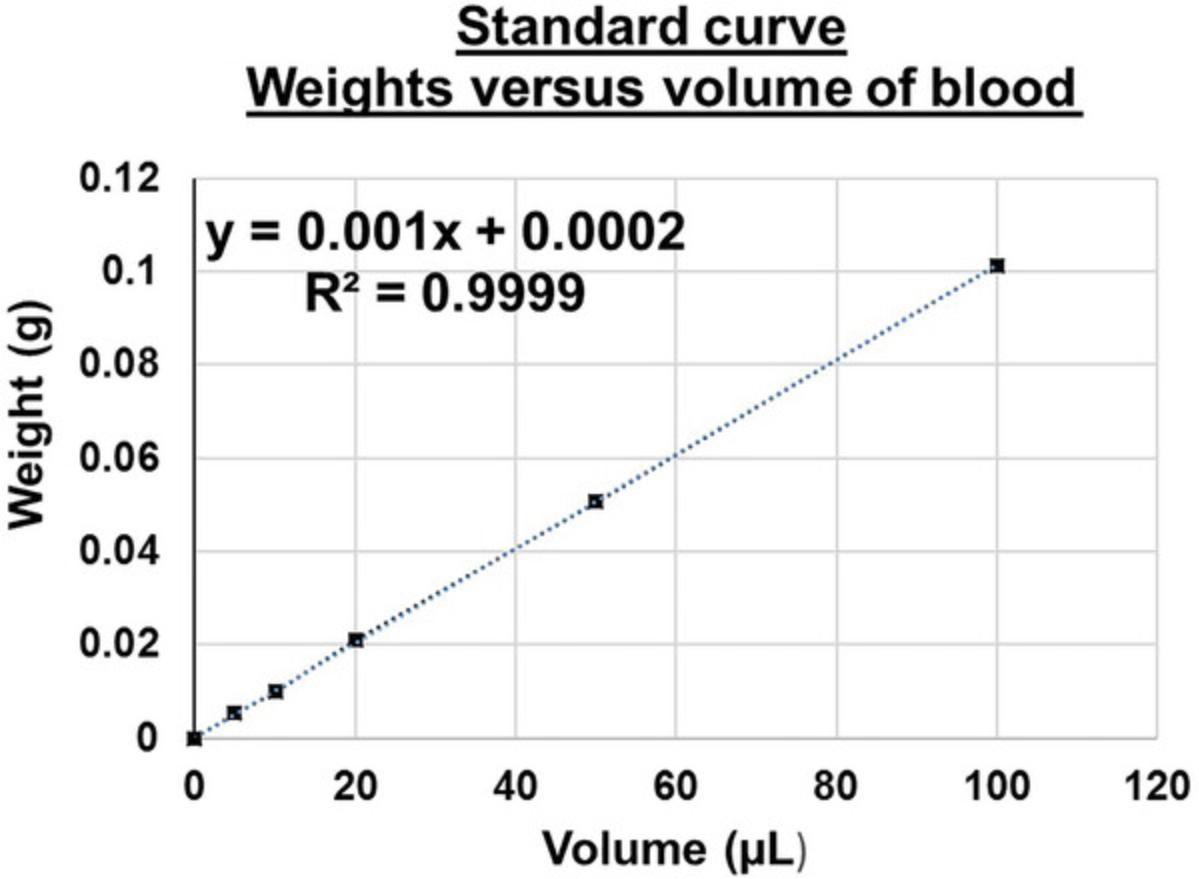
15.Calculate the equation of the straight-line curve as shown below.
y= mx + c (x: volume of blood, y: weight of blood, m= slope/gradient, and c= constant) Volume of blood (x) = [weight of blood (y) – (c)]/ Slope of the line (m) |
16.For routine analysis, a table can be prepared in MS Excel to calculate unknown blood volumes from the weights of the tubes recorded before and after blood collection.
17.Calculate the dilution factor for each blood sample, as shown in Table 1.
Sample name | Weight before (W1) (g) | Weight after (W2) (g) | Final weight of blood (W2–W1) | Volume of blood (V1) (µl) | Vol. of anticoagulant EDTA (µl) | Total vol. blood+ anticoagulant (V2) (µl) | Dilution factor of blood (V2/V1) | Dilution factor for ADVIA | Final dilution factor |
---|---|---|---|---|---|---|---|---|---|
Ml | 0.508 | 0.565 | 0.057 | 56.8 | 15 | 71.8 | 1.26 | 4 | 5.04 |
M2 | 0.509 | 0.581 | 0.072 | 71.8 | 15 | 86.8 | 1.21 | 4 | 4.84 |
M3 | 0.504 | 0.579 | 0.075 | 74.8 | 15 | 89.8 | 1.20 | 4 | 4.80 |
M4 | 0.511 | 0.568 | 0.057 | 56.8 | 15 | 71.8 | 1.26 | 4 | 5.04 |
M5 | 0.52 | 0.589 | 0.069 | 68.8 | 15 | 83.8 | 1.22 | 4 | 4.88 |
M6 | 0.516 | 0.542 | 0.026 | 25.8 | 15 | 40.8 | 1.58 | 4 | 6.32 |
M7 | 0.516 | 0.578 | 0.062 | 61.8 | 15 | 76.8 | 1.24 | 4 | 4.96 |
M8 | 0.507 | 0.544 | 0.037 | 36.8 | 15 | 51.8 | 1.41 | 4 | 5.64 |
M9 | 0.511 | 0.586 | 0.075 | 74.8 | 15 | 89.8 | 1.20 | 4 | 4.80 |
M10 | 0.512 | 0.574 | 0.062 | 61.8 | 15 | 76.8 | 1.24 | 4 | 4.96 |
M11 | 0.510 | 0.58 | 0.07 | 69.8 | 15 | 84.8 | 1.21 | 4 | 4.84 |
M12 | 0.511 | 0.565 | 0.054 | 53.8 | 15 | 68.8 | 1.28 | 4 | 5.12 |
M13 | 0.511 | 0.563 | 0.052 | 51.8 | 15 | 66.8 | 1.29 | 4 | 5.16 |
M14 | 0.507 | 0.522 | 0.015 | 14.8 | 15 | 29.8 | 2.01 | 4 | 8.05 |
Screening of blood samples for clots
18.Blood clots should be exceedingly rare if employing the appropriate amount of anticoagulant and correct sample handling technique. Visually screen the blood samples for clots by gently tumble-rolling the microcentrifuge tube containing blood at eye level and closely looking for evidence of blood clots (Brake et al., 2019).
19.Exclude all the clotted blood samples from blood count measurements and analysis to ensure accurate blood cell numbers.
Dilution correction of blood cell count using the standard curve
20.Mix 50 μl of anticoagulated blood with 150 μl of 1× PBS (4-fold dilution).
21.Correct the blood cell counts obtained from the automated analyzer by multiplying each value by the appropriate dilution factor, as shown in Table 2.
Females | Males | ||||
---|---|---|---|---|---|
Hematology Parameter | Units x | Corrected data | Uncorrected data | Corrected data | Uncorrected data |
White blood cell count | 103 cells/μl | 9.52 ± 3.1 | 8.23 ± 2.7 | 9.5 ± 3.2 | 8.0 ± 2.4 |
Red blood cell count | 106 cells/μl | 10.1 ± 1.001 | 8.4 ± 0.9 | 10.6 ± 1.1 | 8.6 ± 0.9 |
Hemoglobin | g/dl | 184.1 ± 18.1 | 150.5 ± 14.9 | 192.8 ± 18.3 | 158.5 ± 16.6 |
Mean cell volume | fl | 48.2 ± 1.1 | 48.3 ± 1.1 | 48 ± 0.6 | 48.1 ± 0.7 |
Platelets | 103 cells/μl | 1231.3 ± 306.8 | 1037.8 ± 252.4 | 1276.8 ± 187.4 | 1058.5 ± 187.4 |
Mean platelet volume | fl | 6.2 ± 1.9 | 6.1 ± 2.0 | 6.5 ± 2.3 | 6.2 ± 2.3 |
Neutrophils | 103 cells/μl | 0.68 ± 0.31 | 0.3 ± 0.3 | 0.5 ± 0.3 | 0.38 ± 0.3 |
Lymphocytes | 103 cells/μl | 8.6 ± 2.6 | 7.5 ±2.3 | 8.2 ± 3.3 | 7.1 ± 2.5 |
Monocytes | 103 cells/μl | 0.4 ± 0.6 | 0.5 ± 0.8 | 0.3 ± 0.3 | 0.36 ± 0.4 |
Eosinophils | 103 cells/μl | 0.2 ± 0.1 | 0.2 ± 0.1 | 0.15 ± 0.09 | 0.13 ± 0.1 |
REAGENTS AND SOLUTIONS
100 mg/kg carboplatin solution (cat. no. C2538, Sigma-Aldrich)
- Dissolve 10 mg of carboplatin in 100 µl of 0.9% saline solution.
0.5 M EDTA in 1× tris-buffered saline, pH 7.4
- Mix 186.1 g of Na2EDTA in 700 ml distilled water.
- Heat and stir the solution to approximately 60°C in a fume hood and add 10 M NaOH dropwise until the solution clarifies and the salt dissolves completely.
- Add 100 ml of 10× tris-saline buffer with pH 7.4 and readjust pH to 7.4 using 1M HCl/NaOH.
- Make final volume up to 1 liter, aliquot, and store at RT.
1× phosphate buffered saline (1× PBS, pH 7.4)
- Dissolve 8 g NaCl, 1.42 g Na2HPO4, 0.2 g KCl, and 1.8 g KH2PO4 in 900 ml distilled water.
- Adjust pH to 7.4 using 1 M HCl or 1 M NaOH as required.
- Make final volume up to 1 liter.
10× tris-buffered saline (1.5 M NaCl containing 0.2 M tris·HCl; pH 7.4)
- Dissolve 24 g of tris base and 88 g of NaCl in 500 ml double distilled water.
- Adjust pH to 7.4 with 12 M HCl.
- Make the final volume up to 1 liter with double distilled water.
COMMENTARY
Background Information
Here, we describe a simple method for the routine collection and analysis of blood cell counts with improved accuracy. This method is easy to follow and has utility in detecting subtle changes in blood cell numbers and parameters. Using this technique, we can maintain appropriate blood–anticoagulant ratios and avoid variations in the properties of the platelets and other blood cells for downstream experiments (Table 2). Although routinely used, steps such as pipetting can alter the resting state of the blood cells, specifically platelets, and would introduce artifacts in the results (Table 3). It is necessary to exclude samples showing visible clots to avoid introducing inaccuracies and skewing of the data (Fig. 3A-C). Out first-hand experience shows that if the quality of the blood collected is good, this significantly reduces animal numbers required to achieve high-quality data with low coefficients of variation; we recommend three to four mice per group to confidently determine differences of 10% in platelet numbers. By minimizing/removing blood clot artifacts and increasing the precision of dilution correction, we can obtain accurate cell count data, helping reduce costs and animal numbers. (Fig. 4A and B).
Problem | Possible causes | Solutions | |
---|---|---|---|
1. | Restricted blood flow from the needle | Blood clot formation in the needle | Remove the needle and collect the blood dropwise directly from the site of the incision. |
Needle could be coated with anticoagulant. | |||
2. | Mouse vein not visible | Sub-optimal vasodilation | Increase the intensity of light. |
Mouse tail can be wiped with ethanol wipes just before blood collection to increase the visibility of the mouse tail vein. | |||
Allow a recovery period (at least 5 min) between collections. | |||
Extend exposure to warmth (but not more than 2 min). | |||
3. | Low blood volume | Sub-optimal temperature | Increase the length of exposure to warmth to improve vein visibility and yield of blood |
4. | Formation of blood clots | Insufficient anticoagulant | Increase the volume of the anticoagulant |
Insufficient mixing | Increase mixing of the sample immediately after drawing blood | ||
5. | Formation of micro-clots | Excessive shear exposure during pipetting and tapping tubes containing blood | Use tips with ends cut off to reduce shear-mediated platelet activation. |
Ensure complete mixing of blood with anticoagulant immediately after blood collection. | |||
6. | Persistent blood clotting | Consider increasing the anticoagulant volume | |
pH changes in EDTA solution | Adjust the pH of the anticoagulant to 7.4 | ||
Inadequate mixing of blood and anticoagulant | Make sure to gently mix/flick each vial immediately after blood collection to ensure complete mixing of blood with the anticoagulant | ||
7. | Hemolysis | Physical trauma | Avoid applying pressure or physically manipulating the tail to increase the blood yield. This can cause blood cell lysis. |
Adjust the pH of the anticoagulant to 7.4 | |||
Repeated use of the same needle | Use one needle per mouse. Blood clots formed in the needle barrel can contribute to hemolysis. |
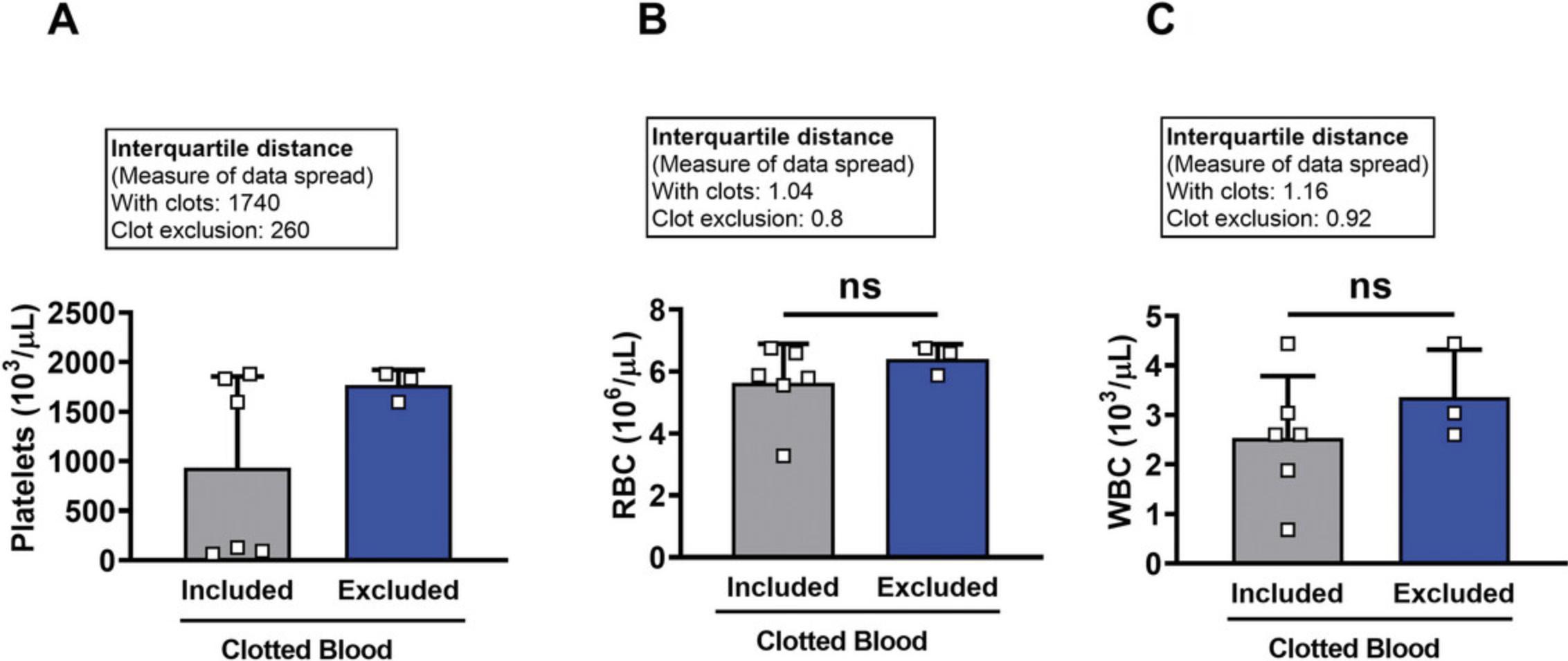
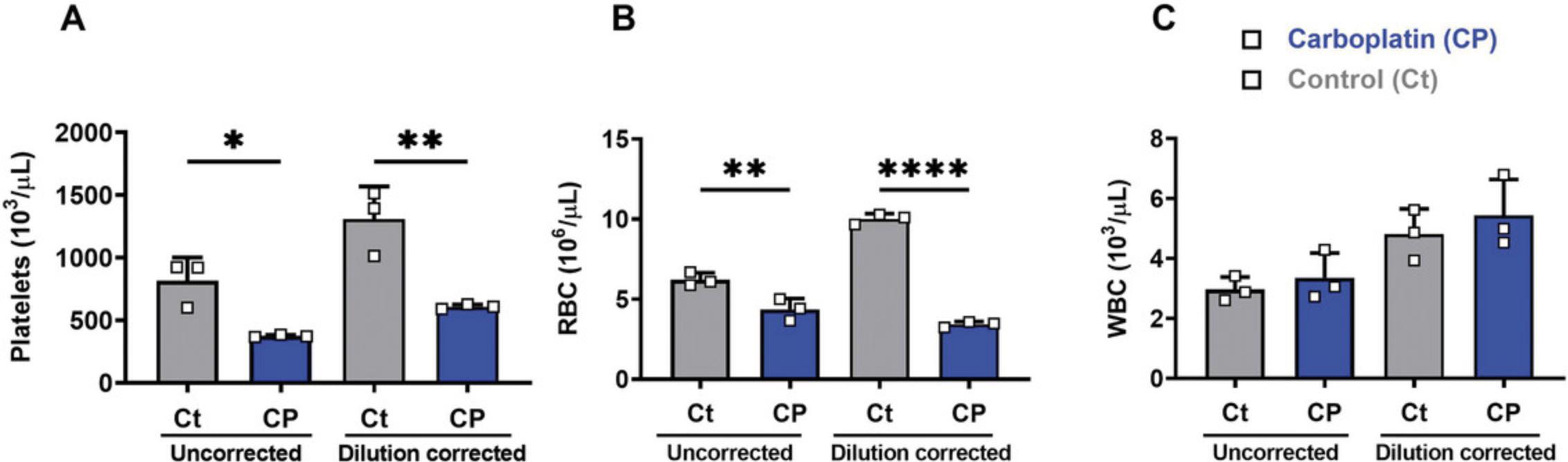
This protocol utilizes the tail bleed route of blood collection and dilution correction for routine blood count measurements. However, the protocol can be adapted to other routes of blood collection, including cardiac puncture. Although the retro-orbital blood collection technique yields higher blood volumes and avoids shear-induced blood clotting in samples, this method is not permitted in several countries due to the risk of animals enduring significant pain and discomfort, notably if the operator lacks expertise (Golde et al., 2005). Moreover, retro-orbital bleeding procedures can ethically be performed only once every fortnight, hindering research that requires more frequent blood cell and component monitoring and demanding a larger animal cohort to enable repetitive blood counts. Cardiac puncture is typically a terminal procedure that enables collection of larger volumes (>200 µl) of blood. However, mice have to be anesthetized before blood collection, which requires training and practice to perform on a routine basis. They may affect blood components if functional assays are also to be performed. The collection of similar volumes of cardiac blood to achieve a similar anticoagulant dilution is challenging to achieve as the volume of blood obtained from cardiac bleeds will depend significantly on the age, sex, and size of each mouse. In addition, hemolysis is more common in blood samples collected with a needle and syringe, such as in cardiac puncture, which significantly compromises the quality of blood samples and can limit subsequent functional assays. Collecting blood into pre-weighed tubes coated with anticoagulants removes the need to use expensive commercial blood collection tubes and widens the options for different anticoagulants. It is cost-effective and does not require significant experience or expertise to perform the procedure.
The method is also well suited to avoid unnecessary platelet activation and clot formation; hence, it can be adapted specifically for experiments involving platelet activation studies using platelet receptor agonists ( Fig. 5A and B). Our method has direct applicability in platelet research, and it can enable investigators in related research fields (e.g., innate and adaptive immunology) to improve the quality of the blood collected and positively affect the results obtained in their field-specific studies. As platelet functional assays are relatively demanding and sensitive to platelet pre-activation, the suggested protocol is designed for platelet studies requiring resting or inactivated platelets that can be treated with agonists. We have shown that this technique can significantly overcome the variations introduced via blood–anticoagulant dilution and collection (Moorhead et al., 2016).
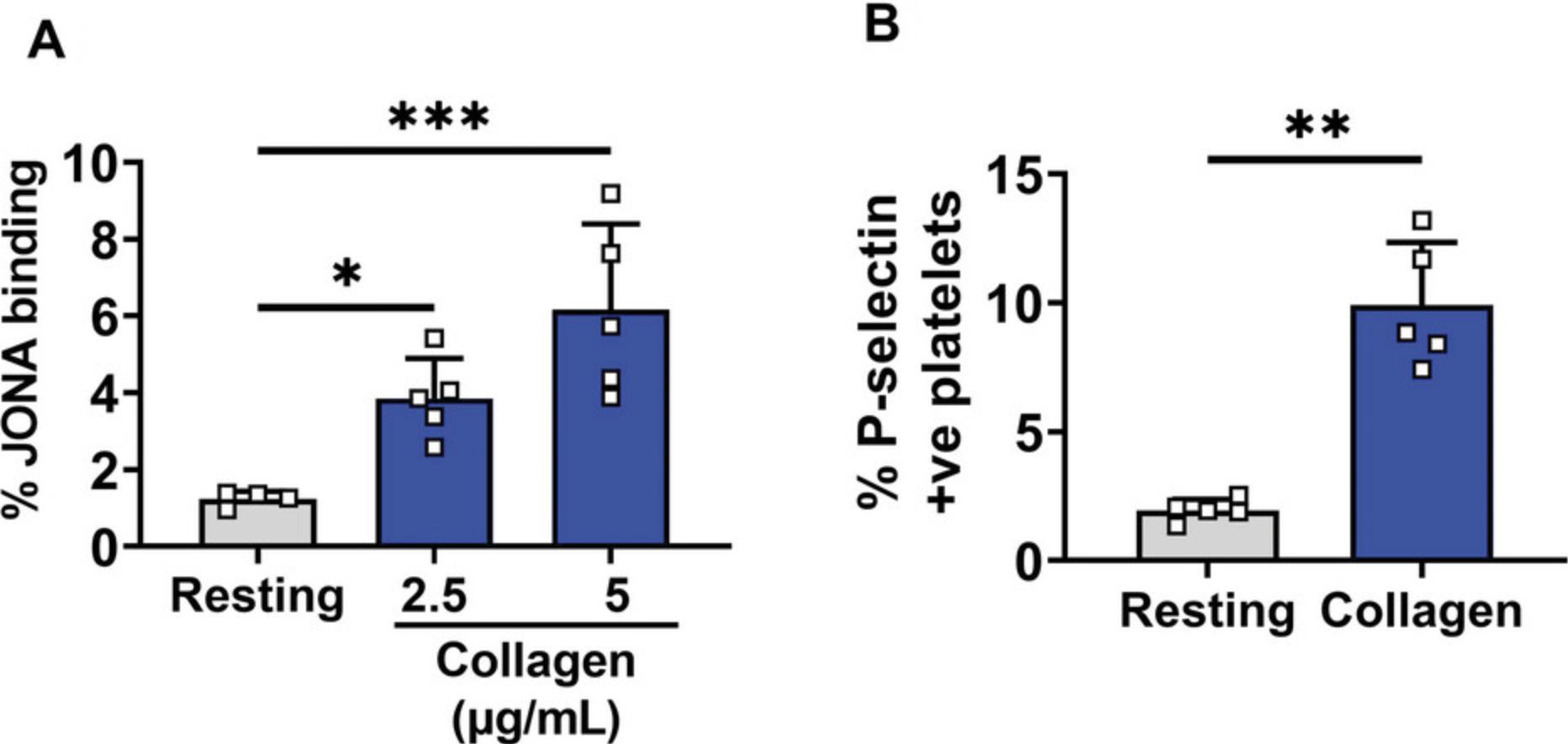
Critical Parameters
To remain consistent, it is essential to carry out blood cell enumeration within 1 to 2 hr of blood collection. Storing anticoagulant-treated blood in a static condition for an extended period can lead to hemolysis, affecting RBC parameters in the count and rendering the data unusable, and this is problematic, especially in longitudinal studies. The choice of anticoagulant should be based on the additional experimental requirements of the samples. EDTA and trisodium citrate are reliable and robust anticoagulants for routine blood analyzer enumeration. In our experience, plasma samples prepared from blood isolated using different anticoagulants can behave differently in various ELISAs, for example, to measure cytokines. As a potent anticoagulant and metal ion chelator, EDTA is not recommended when blood samples are also needed for platelet functional assays, as EDTA prevents platelet function and necessitates recalcification because most platelet assays require Ca2+. Recalcification can be achieved more readily when trisodium citrate is used as the anticoagulant. Anticoagulants such as acid citrate dextrose (ACD), which permits centrifugation and resuspension of platelets in a range of physiological buffers, or heparin, can also be used. However, the volume of anticoagulants must be optimized for mouse blood collection to prevent clotting and platelet activation (Grill et al., 2020). All blood collection techniques can be stressful to the animals; however, techniques that require further interventions, such as anesthesia, introduce additional steps to the procedure, and other modes of blood collection should only be used if large volumes of blood are required (>200 μl). It is recommended that the same individual bleeds the mice for an experiment to reduce the variation introduced by handling of the mice and ensure reproducibility of the data.
Troubleshooting
Statistical Analysis
Statistical comparison of blood cell counts involves running parametric Student t -tests or non-parametric Mann–Whitney tests, determined by the normality of the data, to evaluate differences between the mean values of each mouse group for mouse strains, treatments, and genotypes. These tests identify any significant differences between the groups. In cases where more than two groups were being compared, we used one-way ANOVA (normal distribution) or the Kruskal–Wallis test (non-normal distribution) with corrections for multiple comparisons.
Understanding Results
If collected into solutions or tubes that lack or have inappropriate levels of anticoagulation, blood cells, plasma proteins, and the collection vessel conspire to activate the coagulation cascade. This results in the formation of thrombin, which leads to uncontrolled platelet and leukocyte activation, generation of fibrin, and formation of variable blood clots. As emphasized in our current protocol (step 18), it is essential to exclude samples that show visible blood clots from murine blood cell count measurements and analyses because these samples provide variable and highly inaccurate results. For example, in cardiac puncture blood samples collected into insufficient anticoagulants, micro- and macroscopic blood clots form, and the data spread (interquartile distance) is extensive compared to data acquired from appropriately anticoagulated samples (Fig. 3A-C). These results show that the data spread is not due to any experimental variation but is a combined artifact of insufficient anticoagulation and poor blood collection techniques that led to apparent reductions in platelet, RBC, and WBC counts. To achieve appropriate anticoagulation, a critical factor is the correction of blood dilution. As a positive control, we show that in mouse tail vein blood samples from carboplatin-treated mice [a drug known to reduce platelet and RBC counts (McElroy et al., 2015)], with dilution correction, we reduced the spread of replicate data and improved the significance of p values (Fig. 4A-C).
Platelet function was tested in mouse blood collected using the current protocol. Tail vein blood samples collected into trisodium citrate anticoagulant were activated using collagen, an agonist for platelet functional receptor glycoprotein (GP) VI. It is well established that once activated, platelets degranulate and express proteins such as P-selectin on their surface (Hsu-Lin et al., 1984), which can be detected using flow cytometry. In addition, platelet activation by ligand engagement of the GPVI receptor triggers conformational (activating) changes in the fibrinogen receptor αIIbβ3, present on the platelet surface (Fullard, 2004). Active αIIbβ3 can be detected on mouse platelets using the JON-A antibody. Untreated, resting platelets (inactivated) had little or no surface P-selectin expression and JON-A antibody binding. However, an increase in both activation markers was observed upon activation with collagen. These data suggest that the blood collection technique and further laboratory handling did not activate platelets (Fig. 5A-C).
Time Considerations
This protocol involves the (1) preparation of anticoagulant-coated microcentrifuge tubes, (2) pre- and post-collection weighing of the tubes, and (3) preparation of butterfly needles, all of which can be done before the day of blood collection. On the day of the experiment, an experienced technician can collect blood from 20 mice and carry out blood count measurements using an automated counter followed by dilution correction in approximately 2 h.
Acknowledgments
The authors thank Sarah Hicks, Sidra Ali, Samina Nazir, Nadine Hein, and Megan Pavy for their help in mice handling and sample collection. This work was supported by the National Health and Medical Research Council of Australia.
Open access publishing facilitated by Australian National University, as part of the Wiley - Australian National University agreement via the Council of Australian University Librarians.
Author Contributions
Vijay Bhoopalan : conceptualization, data curation, investigation, methodology, writing original draft, writing review and editing; Elizabeth E. Gardiner : conceptualization, data curation, funding acquisition, investigation, methodology, project administration, resources, supervision, writing original draft, writing review and editing; Amandeep Kaur : conceptualization, data curation, formal analysis, methodology, supervision, writing original draft, writing review and editing.
Conflict of Interest
The authors have no conflicts of interest to declare.
Open Research
Data Availability Statement
The data, tools, and material that support the protocol are available from the corresponding author upon reasonable request.
Literature Cited
- Ahrens Kress, A. P., Zhang, Y., Kaiser-Vry, A. R., & Sauer, M. B. (2022). A comparison of blood collection techniques in mice and their effects on welfare. Journal of the American Association for Laboratory Animal Science: JAALAS , 61, 287–295. https://doi.org/10.30802/aalas-jaalas-21-000129
- Aurbach, K., Spindler, M., Haining, E. J., Bender, M., & Pleines, I. (2019). Blood collection, platelet isolation and measurement of platelet count and size in mice-a practical guide. Platelets , 30, 698–707. https://doi.org/10.1080/09537104.2018.1528345
- Bain, B. J. (2005). Diagnosis from the blood smear. New England Journal of Medicine , 353, 498–507. https://doi.org/10.1056/NEJMra043442
- Bourrienne, M. C., Gay, J., Mazighi, M., & Ajzenberg, N. (2022). State of the art in cerebral venous sinus thrombosis animal models. Journal of Thrombosis and Haemostasis , 20, 2187–2196. https://doi.org/10.1111/jth.15816
- Brake, M. A., Ivanciu, L., Maroney, S. A., Martinez, N. D., Mast, A. E., & Westrick, R. J. (2019). Assessing Blood Clotting and Coagulation Factors in Mice. Current Protocols in Mouse Biology , 9, e61. https://doi.org/10.1002/cpmo.61
- Donovan, J., & Brown, P. (2013). Care and handling of laboratory mice. Current Protocols in Microbiology , 31, A.3n.1–a.3n.18. https://doi.org/10.1002/9780471729259.mca03ns31
- Fullard, J. F. (2004). The role of the platelet glycoprotein IIb/IIIa in thrombosis and haemostasis. Current Pharmaceutical Design , 10, 1567–1576. https://doi.org/10.2174/1381612043384682
- Golde, W. T., Gollobin, P., & Rodriguez, L. L. (2005). A rapid, simple, and humane method for submandibular bleeding of mice using a lancet. Lab Animal , 34, 39–43. https://doi.org/10.1038/laban1005-39
- Grill, A., Kiouptsi, K., Karwot, C., Jurk, K., & Reinhardt, C. (2020). Evaluation of blood collection methods and anticoagulants for platelet function analyses on C57BL/6J laboratory mice. Platelets , 31, 981–988. https://doi.org/10.1080/09537104.2019.1701185
- Grozovsky, R., Giannini, S., Falet, H., & Hoffmeister, K. M. (2015). Regulating billions of blood platelets: Glycans and beyond. Blood , 126, 1877–1884. https://doi.org/10.1182/blood-2015-01-569129
- Han, T., Tang, H., Lin, C., Shen, Y., Yan, D., Tang, X., & Guo, D. (2022). Extracellular traps and the role in thrombosis. Frontiers in Cardiovascular Medicine , 9, 951670. https://doi.org/10.3389/fcvm.2022.951670
- Hoggatt, J., Hoggatt, A. F., Tate, T. A., Fortman, J., & Pelus, L. M. (2016). Bleeding the laboratory mouse: Not all methods are equal. Experimental Hematology , 44, 132–137.e131. https://doi.org/10.1016/j.exphem.2015.10.008
- Hsu-Lin, S., Berman, C. L., Furie, B. C., August, D., & Furie, B. (1984). A platelet membrane protein expressed during platelet activation and secretion. Studies using a monoclonal antibody specific for thrombin-activated platelets. Journal of Biological Chemistry , 259, 9121–9126.
- Li, J. L., Zarbock, A., & Hidalgo, A. (2017). Platelets as autonomous drones for hemostatic and immune surveillance. Journal of Experimental Medicine , 214, 2193–2204. https://doi.org/10.1084/jem.20170879
- Liu, X., Li, H., Wu, J., Yu, Y., Zhang, M., Zou, W., & Gu, Y. (2019). Modified blood collection from tail veins of non-anesthetized mice with a vacuum blood collection system and eyeglass magnifier. Journal of Visualized Experiments: JoVE , 144, e59136. https://doi.org/10.3791/59136
- Mandel, J., Casari, M., Stepanyan, M., Martyanov, A., & Deppermann, C. (2022). Beyond hemostasis: Platelet innate immune interactions and thromboinflammation. International Journal of Molecular Sciences , 23, 3868. https://doi.org/10.3390/ijms23073868
- McElroy, P. L., Wei, P., Buck, K., Sinclair, A. M., Eschenberg, M., Sasu, B., & Molineux, G. J. (2015). Romiplostim promotes platelet recovery in a mouse model of multicycle chemotherapy-induced thrombocytopenia. Experimental Hematology , 43, 479–487. https://doi.org/10.1016/j.exphem.2015.02.004
- Moorhead, K. A., Discipulo, M. L., Hu, J., Moorhead, R. C., & Johns, J. L. (2016). Alterations due to dilution and anticoagulant effects in hematologic analysis of rodent blood samples on the Sysmex XT-2000iV. Veterinary Clinical Pathology , 45, 215–224. https://doi.org/10.1111/vcp.12338
- Palacios-Acedo, A. L., Mege, D., Crescence, L., Panicot-Dubois, L., & Dubois, C. (2020). Cancer animal models in thrombosis research. Thrombosis Research , 191, S112–s116. https://doi.org/10.1016/s0049-3848(20)30407-2
- Parasuraman, S., Raveendran, R., & Kesavan, R. (2010). Blood sample collection in small laboratory animals. Journal of Pharmacology and Pharmacotherapeutics , 1, 87–93. https://doi.org/10.4103/0976-500X.72350
- Rana, A., Westein, E., Niego, B., & Hagemeyer, C. E. (2019). Shear-dependent platelet aggregation: Mechanisms and therapeutic opportunities. Frontiers in Cardiovascular Medicine , 6, 141. https://doi.org/10.3389/fcvm.2019.00141
- Rayes, J., & Jenne, C. N. (2021). Platelets: Bridging thrombosis and inflammation. Platelets , 32, 293–294. https://doi.org/10.1080/09537104.2021.1889310
- Ware, J. (2022). Murine models of glycoprotein Ib-IX. Platelets , 33, 811–816. https://doi.org/10.1080/09537104.2021.2022115
- Yang, H., Wu, C., Liu, F., Wang, M., Zou, P., He, Y., Liu, Q., Zhou, Q., & Zhou, S. (2019). Blood collection through subclavian vein puncture in mice. Journal of Visualized Experiments , 147, e59556. https://doi.org/10.3791/59556
CORRECTIONS
In this publication, the following corrections have been made:
In Table 1, in the column titled “Dilution Factor of Blood (V2-V1)”, “(V2-V1)” has been replaced with “(V2/V1).”
In column 1, row 5, “MS” has been replaced with “M5”; in row 8, “MS” has been replaced with “M8”; in row 10, “MIO” has been replaced with “M10”, and in row 11, “MII” has been replaced with “M11”.
In column 2, row 5, “0.052” has been replaced with “0.52”; row 7, “0.507” has been replaced with “0.516”; row 8 “0.511” has been replaced with “0.507”, row 9 “0.512” has been replaced with “0.511”, row 10, “0.510” has been replaced with “0.512”; row 11, “0.511” has been replaced with “0.510”, and in row 13 “0.507” has been replaced with “0.511”.
In column 3, row 14, “22” has been replaced with “0.522”.
In column 8, row 14, “12.01” has been replaced with “2.01”.
In column 10, row 1: “5.26” has been replaced with “5.04”; row 2: “5.21” has been replaced with “4.84”; row 3: “5.20” has been replaced with “4.80”; row 4: “5.26” has been replaced with “5.04”; row 5: “5.22” has been replaced with “4.88”; row 6: “5.58” has been replaced with “6.32”; row 7: “5.24” has been replaced with “4.96”; row 8: “5.41” has been replaced with “5.64”; row 9: “5.20” has been replaced with “4.80”; row 10: “5.24” has been replaced with “4.96”; row 11: “5.21” has been replaced with “4.84”; row 12: “5.28” has been replaced with “5.12”; row 13: “5.29” has been replaced with “5.16”, and in row 14: “6.01” has been replaced with “8.05”.
Finally, on Page 6 step 17, line 8, the sentence “This dilution factor was added to the standard ADVIA dilution factor (1:4) to obtain the final dilution factor for the blood.” has been replaced with “This dilution factor was multiplied by the standard ADVIA dilution factor (1:4) to obtain the final dilution factor for the blood.”
The current version online now includes these corrections and may be considered the authoritative version of record.
Citing Literature
Number of times cited according to CrossRef: 1
- Naima Mammate, Fatima Ezzahra El Oumari, Hamada Imtara, Salim Belchkar, Ramzi A. Mothana, Hinde E.L. Fatemi, Mohammed Danouche, Sara Er-rahmani, Nabil Boucetta, Omar M. Noman, Mahmoud Tarayrah, Tarik Sqalli Houssaini, The Anti-urolithiatic effect of the roots of Saussurea costus (falc) Lipsch agonist ethylene glycol and magnesium oxide induced urolithiasis in rats, Saudi Pharmaceutical Journal, 10.1016/j.jsps.2024.101967, 32 , 3, (101967), (2024).