A Multiplex One-Step RT-qPCR Protocol to Detect SARS-CoV-2 in NP/OP Swabs and Saliva
Joshua Bland, Joshua Bland, Ashley Kavanaugh, Ashley Kavanaugh, Lenny K. Hong, Lenny K. Hong, Omar Perez, Omar Perez, Shrihari S. Kadkol, Shrihari S. Kadkol
Abstract
Since December 2019, SARS-CoV-2 has spread extensively throughout the world, with more than 117 million reported cases and 2.6 million deaths (Johns Hopkins coronavirus resource center, https://coronavirus.jhu.edu/map.html). Detecting the virus is the first step in diagnosing the infection, followed by quarantine to prevent transmission. Nasopharyngeal/oropharyngeal swabs (NP/OP) and saliva are two specimen types that are most often analyzed to detect SARS-CoV-2 by molecular tests that detect viral RNA or by antigen/antibody tests that detect viral proteins and/or the host immune response against the virus. Compared to antigen/antibody tests, molecular tests are highly sensitive and specific for detecting the virus. A significant drawback is that specimen collection requirements are specific to each test and cannot be interchanged with another test. Some tests are qualified to be used on NP swabs or saliva, but not both specimen types. Even with NP swabs, a test may be qualified to detect the virus only with swabs collected in viral transport medium (VTM) but not in other media. These restrictive pre-analytic steps are disadvantageous in that a lab would have to develop and validate different tests for SARS-CoV-2 depending on the specimen type and collection media, with added setup cost, infrastructure, and training requirements. To overcome these problems, we developed and validated a cost-effective multiplex reverse-transcription real-time PCR assay that can be used to detect SARS-CoV-2 in different specimen types. The assay is highly sensitive and specific, can be used to detect the virus in saliva as well as NP swabs collected in different media such as VTM, saline, and commercial preservative fluid, and serves as one test for all applications. The protocol also describes an optimal laboratory setup and unidirectional workflow for detecting SARS-CoV-2 by RT-qPCR. © 2021 The Authors. Current Protocols published by Wiley Periodicals LLC.
Basic Protocol 1 : Manual viral nucleic acid extraction from NP/OP swabs collected in different media, and from saliva
Alternate Protocol 1 : Low-throughput automated extraction on the Qiagen EZ1 Advanced XL machine (1-14 samples)
Alternate Protocol 2 : High-throughput automated extraction on the Kingfisher Flex machine (1-96 samples)
Basic Protocol 2 : Multiplex RT-qPCR protocol to detect SARS-CoV-2
Alternate Protocol 3 : Multiplex one-step RT-qPCR protocol to detect SARS-CoV-2 with S and E gene probes labeled with the same fluorochrome
INTRODUCTION
Many different molecular tests to detect SARS-CoV-2 have been developed by research and clinical laboratories as well by commercial companies (Kubina & Dziedzic, 2020). However, each test has specific requirements regarding sample type, collection, storage, and analysis. As such, it is very difficult to adapt a particular test to different specimen types or changing needs. For example, the Abbott SARS-CoV-2 Real Time PCR assay can only be performed on NP/OP swabs collected in the Abbott Universal collection tube, and not in other media (Hirschhorn et al., 2021). The CDC N1/N2 assay has an emergency use authorization from the FDA only for NP/OP swabs collected in viral transport media, but not for other media types or saliva (Lu et al., 2020). Moreover, different tests need specific equipment, reagents, and consumables, and require additional personnel training. Each individual test also has its own analytical sensitivity and specificity for detecting SARS-CoV-2, making it difficult to compare results across laboratories (Fung et al., 2020). Some tests are low throughput, whereas others must be done in a high-throughput manner, making it difficult to adapt to changing test volumes. To overcome these disadvantages, we developed and validated a single highly sensitive and specific multiplex one-step RT-qPCR assay to detect SARS-CoV-2 in saliva as well as in NP/OP swabs collected in different media, using generic reagents and PCR machines that are available in any laboratory that does molecular analysis. No special training is required beyond general molecular laboratory experience. The protocols have a short turn-around-time of 3-4 hr for results, and are cost-effective. They are versatile and can be adapted to test a few samples or thousands of samples each day. Lastly, having a single protocol to detect SARS-CoV-2 in multiple specimen types helps to bring about standardization and consistency in testing across different specimen types and laboratories.
SARS-CoV-2 is a single-stranded RNA virus that is 29,903 nt long and belongs to the family Coronaviridae, order Nidovirales, genus Betacoronavirus, and lineage B (Abdelrahman, Li, & Wang, 2020; Wang et al., 2020). The positive-sense RNA genome contains open reading frames that code for structural proteins S, N, E, and M (Sartarker & Nampoothiri, 2020) and non-structural proteins (NSPs; Díaz, 2020). The protocols described here allow the detection of SARS-CoV-2 in nasopharyngeal/oropharyngeal swabs collected in different transport media such as VTM, saline, and the Abbott Universal collection tube, and in saliva. In these protocols, S and E genes of the virus and cellular RNaseP are all amplified in the same multiplex RT-qPCR reaction. We chose to include S and E genes of the virus because of their superior sensitivity when compared to the N gene. Also, having two targets decreases the likelihood of false-negative results with variants that contain mutations in the primer/probe binding regions of one or the other gene. Successful amplification of cellular RNaseP in the same reaction indicates good specimen quality, adequate nucleic acid extraction, and absence of PCR inhibition, thereby ruling out false-negative results. The data obtained is qualitative in nature, with “Detected” or “Not detected” results. RNaseP must amplify in all samples to call the result “Detected” or “Not detected.” If RNaseP fails amplification, the result is “Invalid.” An invalid result can occur because of nucleic acid degradation prior to analysis or PCR inhibitors in the sample. Failure of nucleic acid extraction can also result in loss of RNaseP amplification. If the result is invalid, analysis on a fresh specimen is indicated.
A basic protocol for manual nucleic acid extraction and alternate protocols for automated extraction are given. One of the automated protocols is for low-throughput (1-14 specimens) and the other is for high-throughput (1-96 specimens) extraction. The RT-qPCR protocol detects the S and E genes of SARS-CoV-2 and cellular RNaseP in a single multiplex reaction with high sensitivity and specificity. The protocol can be performed on NP/OP swabs collected in different media such as viral transport medium, saline, and Abbott Universal collection tubes, and on saliva. In the basic RT-qPCR protocol, the S and E probes are labeled with different fluorochromes such that the amplification from either target can be visualized separately. For example, amplification of the E but not the S gene suggests a SARS-CoV-2 variant with mutations in the primer- or probe-binding region of the S gene. In the alternate RT-qPCR protocol, the probes are labeled with the same fluorochrome, and it is not possible to distinguish amplification from S and E genes separately. Nevertheless, amplification curves are more robust because the signal intensity is additive. The basic and alternate amplification protocols are otherwise identical.
SAFETY PRECAUTIONS
- Handle all primary specimens in a BSL-2 hood with universal precautions.
- Wear disposable gowns, gloves, N95 mask, face shield, and head cover when handling specimens.
- Follow unidirectional workflow to prevent amplicon contamination. Extract specimens, prepare the RT-qPCR mastermix, add nucleic acid extract to the mastermix, and run the RT-qPCR in a thermocycler in different rooms.
- Dedicate pipettors, gowns, gloves, and masks for each area.
- Prepare mastermixes in a dead air box in Area 2; turn on UV light after use.
- Add nucleic acid to the mastermix in a dead air box in Area 3; turn on UV light after use.
- Wipe all surfaces and pipettors clean with 80% ethanol after each use.
STRATEGIC PLANNING
It is important to maintain a unidirectional workflow to prevent PCR contamination. Plan on dedicating separate rooms for nucleic acid extraction, mastermix preparation, addition of nucleic acid extract to the mastermix, and thermocycling. Do not take amplified products backwards from Area 4 into areas 3, 2, or 1. RT-PCR reagents in Area 2 are stored in a dedicated −20° freezer. Extracted nucleic acids and residual primary specimens are stored in Area 1 in a −80° freezer to prevent degradation. Discard plates containing amplified products in Area 4 in an autoclavable trash bag. Figure 1 shows an optimal laboratory setup and unidirectional workflow to prevent contamination.

Basic Protocol 1: MANUAL VIRAL NUCLEIC ACID EXTRACTION FROM NP/OP SWABS COLLECTED IN DIFFERENT MEDIA, AND FROM SALIVA
Viral nucleic acids are extracted from the specimen manually. The manual method is useful if few samples are analyzed. Specimens are lysed in lysis buffer and applied to spin columns containing a silica membrane. Viral nucleic acids bind the membrane, are washed to remove contaminants, and then eluted in buffer. This process takes about 45 min to extract 6-8 samples. At the end of the extraction process, isolated viral nucleic acid is ready for the multiplexed RT-qPCR reaction.
Materials
-
QiaAmp Viral RNA Mini kit (Qiagen, cat. no. 52904)
-
Specimen:
- Nasopharyngeal/oropharyngeal swabs are collected by trained personnel using standard operating procedures :
- NP/OP swabs (Puritan Rayon tipped applicators 25-800 R 50; Fisher Scientific, cat. no. 22-029-502) collected in viral transport medium (M4RT; Thermofisher, cat. no. R12505), sterile saline (Fisher Scientific, cat. no. Z1376), or Abbott Universal collection tube (Abbott, cat. no. 09N77-055)
- The Abbott multi-collect specimen collection kit tube can also be used ( cat. no . 09K12).
- M4RT and the Abbott tubes are pre-filled with collection medium.
- For saline, aliquot 2 ml sterile saline into 15-ml sterile polypropylene tubes (Fisher Scientific, cat. no. 12-565-268)
- Saliva is collected by having the subject drool about 2 ml of saliva into a 50-ml polypropylene tube (Fisher Scientific, cat. no. 12-565-270)
- Supernatants from cell culture fluids can also be used
-
Absolute ethanol (200 proof)
-
80% ethanol in a spray bottle (1 vol absolute ethanol + 4 vol of distilled water)
-
Freshly prepared bleach, 0.5%, in a spray bottle (1 vol household bleach + 9 vol cold water)
-
Personal protective equipment (see Safety Precautions)
-
Laminar flow hood, BSL-2
-
Pipettors: 20-200 µl, 100-1000 µl
-
Pipette tips: 200 µl, 1000 µl, DNase RNase free with aerosol filters
-
1.5-ml microcentrifuge tubes, DNase/RNase free
-
Vortex, placed in the BSL-2 hood
-
Minicentrifuge (Fisher Scientific, cat. no.12-006-901), placed in the BSL-2 hood
-
Microcentrifuge, placed on a table top outside the hood
-
1.5-ml microcentrifuge tubes
-
Tube racks to fit 1.5-ml microcentrifuge tubes
Before starting extraction
1.Wear personal protective equipment including a N95 mask covered with a blue surgical mask. Wear a disposable lab coat, gloves, head cover, and face shield.
2.Turn on the laminar flow hood.
3.Prepare carrier RNA stock solution (from materials in QiaAmp Viral RNA Mini kit) as follows:
Add 310 μl Buffer AVE to 310 μg lyophilized carrier RNA, dissolve by pipetting, aliquot and store at −20°C.
4.Add 25 ml of absolute ethanol to the bottle labeled AW1 (from QiaAmp kit) and mix well.
5.Add 30 ml absolute ethanol to the bottle labeled AW2 (from QiaAmp kit) and mix well.
6.Prepare buffer AVL-carrier RNA mix as follows:
For each sample: Add 5.6 µl of carrier RNA stock to 560 µl of buffer AVL (from QiaAmp kit).
7.All solutions must be at room temperature.
8.In the BSL-2 hood, pipet 560 µl of Buffer AVL-carrier RNA mix into a 1.5-ml microcentrifuge tube.
9.Add 140 µl of specimen to the Buffer AVL-carrier RNA mix in the tube. Mix well by pipetting up and down gently three times, and vortex for 15 s.
10.Incubate at room temperature for 10 min.
11.Spin the tubes in a minicentrifuge for 5 s to collect all the contents at the bottom of the tube.
12.Add 560 µl absolute ethanol to the tube. Mix by pulse vortexing for 15 s. Solution must be homogeneous after vortexing.
13.On a bench top outside the hood, for each specimen, place a labeled QiaAmp mini column in a rack (column sitting inside the collection tube).
14.Add 630 µl of solution from step 12 to the column, taking care not to wet the rim of the column.
15.Close the cap and microcentrifuge 1 min at 6000 × g.
16.Place the column in a fresh 2-ml collection tube and discard the tube containing the flowthrough.
17.Add the remaining 630 µl of solution from step 12 to the column.
18.Close the cap and microcentrifuge 1 min at 6000 × g.
19.Discard the flowthrough in the collection tube and place the column back in the same collection tube.
20.Add 500 µl of buffer AW1 (prepared in step 4) to the column.
21.Close the cap and microcentrifuge 1 min at 6000 × g.
22.Discard the flowthrough in the collection tube and place the column back in the same collection tube.
23.Add 500 µl of buffer AW2 (prepared in step 5) to the column.
24.Close the cap and microcentrifuge at full speed (20,000 × g) for 1 min.
25.Discard the flowthrough in the collection tube and place the column back in the same collection tube.
26.Close the cap and microcentrifuge at full speed (20,000 × g) again for 1 min to remove all traces of ethanol.
27.Transfer the column to a fresh 1.5-ml microcentrifuge tube. Discard the flowthrough in the collection tube.
28.Add 50 µl of Buffer AVE to the column. Incubate at room temperature for 1 min.
29.Centrifuge at full speed (20,000 × g) for 1 min to elute the viral nucleic acid.
30.Discard the column, label the tube, and store extracted nucleic acid at −80°C.
31.Wipe down the hood with freshly prepared bleach (0.5%) followed by 80% ethanol. Turn on the UV light.
Alternate Protocol 1: LOW-THROUGHPUT AUTOMATED EXTRACTION ON THE QIAGEN EZ1 ADVANCED XL MACHINE (1-14 SAMPLES)
In this automated method, the specimen is lysed in lysis buffer and extracted on a nucleic acid extractor. Viral nucleic acid is purified by binding to magnetic beads and eluted in buffer at the final step. The Qiagen EZ1 Advanced machine can extract 14 samples in an hour. At the end of the extraction process, isolated viral nucleic acid is ready for the multiplexed RT-qPCR reaction.
Additional Materials (also see Basic Protocol 1)
-
EZ1 Virus Mini Kit v2.0 (Qiagen, cat. no. 955134)
-
Buffer AVL (Qiagen, cat. no. 19073)
-
EZ1 Advanced XL machine (Qiagen)
-
EZ1 Advanced XL Virus Card v2.0 (Qiagen)
Before starting extraction
1.Wear personal protective equipment including a N95 mask covered with a blue surgical mask. Wear a disposable lab coat, gloves, head cover and face shield.
2.Turn on the laminar flow hood.
3.Prepare carrier RNA stock solution (from materials in EZ Virus Mini Kit) as follows:
Add 310 μl Buffer AVE to the tube containing lyophilized carrier RNA, dissolve by pipetting up and down, aliquot, and store at 4°C.
4.Prepare carrier RNA in a 1.5-ml microcentrifuge tube as follows:
For each sample: Add 3.6 µl of carrier RNA stock (also from EZ Virus Mini Kit) solution to 56.4 µl of buffer AVE, and mix by pipetting up and down.
5.All solutions must be at room temperature.
6.In a BSL-2 hood, pipet 200 µl of buffer AVL into a labeled 2-ml tube (supplied in the EZ Virus Mini kit).
7.Add 200 µl of specimen to the same labeled 2-ml tube, and pipet up and down gently to mix well (volume = 400 µl).
8.Insert the EZ1 Advanced XL virus card 2.0 into the slot in the machine.
9.Turn on the EZ1 Advanced XL machine.
10.Press start.
11.Open the instrument door.
12.Invert the reagent cartridges three times and tap on the bench to collect the contents to the bottom. Insert the supplied 2-ml tube at the back of each cartridge. Insert the cartridges into the reagent racks on the machine.
13.Follow the on-screen instructions for work table setup. Insert 1.5-ml elution tubes in the first row, filter tips in the second row, carrier RNA in the third row, and sample tubes in the fourth row.
14.Close the instrument door.
15.Press the start button.
16.After the protocol ends (∼45 min), open the instrument door.
17.Remove the elution tubes containing viral nucleic acids from the first row. Label the elution tubes and store the nucleic acids at −80°C.
18.Discard the remaining tubes.
19.Wipe down the hood with freshly prepared bleach (0.5%) followed by 80% ethanol. Turn on the UV light.
Alternate Protocol 2: HIGH-THROUGHPUT AUTOMATED EXTRACTION ON THE KINGFISHER FLEX MACHINE (1-96 SAMPLES)
In this automated method, the specimen is lysed in lysis buffer and extracted on a nucleic acid extractor. Viral nucleic acid is purified by binding to magnetic beads and eluted in buffer at the final step. The Kingfisher Flex machine can extract up to 96 samples in about half an hour. At the end of the extraction process, isolated viral nucleic acid is ready for the multiplexed RT-qPCR reaction.
Additional Materials (also see Basic Protocol 1)
-
MagMAX™ Viral/Pathogen Nucleic Acid Isolation Kit (Fisher Scientific, cat. no. A42352)
-
80% ethanol, 150 ml/96 samples in a DNase-/RNase-free bottle
-
15-ml sterile polypropylene tubes (Fisher Scientific, cat. no. 12-565-268)
-
50-ml sterile polypropylene tubes (Fisher Scientific, cat. no. 12-565-270)
-
Reagent reservoir, 25 ml (Fisher Scientific, cat. no. 04-100-517)
-
Reagent reservoir, 55 ml (Fisher Scientific, cat. no. 12-111-085)
-
Pipettors: 2-20 µl, 20-200 µl, 100-1000 µl
-
Multichannel pipettor (Fisher Scientific, cat. no. FA10015TG or equivalent)
-
Pipette tips: 20 µl, 200 µl, 1000 µl, DNase RNase free with aerosol filters
-
96-well deep-well plates (Fisher Scientific, cat. no. 22-387-031)
-
Microamp clear adhesive film (Fisher Scientific, cat. no. 43-063-11)
-
Kingfisher Flex machine (Thermofisher)
-
Kingfisher 96 tip comb (Thermofisher, cat. no. 97002534)
Before starting extraction
1.Wear personal protective equipment including a N95 mask covered with a blue surgical mask. Wear a disposable lab coat, gloves, head cover, and face shield.
2.Turn on the laminar flow hood.
3.All solutions must be at room temperature.
4.Vortex the bottle containing the binding beads for 15 s to re-suspend.
5.Prepare binding bead mix from ingredients in the MagMAX kit in a sterile 15-ml or 50-ml polypropylene tube:
- For one sample: 265 µl binding solution + 10 µl binding beads.
- For 24 wells: 7.31 ml binding solution + 276 µl binding beads.
- For 48 wells: 14.6 ml binding solution + 552 µl binding beads.
- For 96 wells: 29.2 ml binding solution + 1104 µl binding beads.
- Once prepared, invert the tube to mix thoroughly and pour into the reagent reservoir.
6.Fill four 96-well deep-well plates and label as follows:
- Wash Plate 1—Wash buffer (from MagMAX kit), 500 µl/well
- Wash Plate 2—80% ethanol, 500 µl/well
- Wash Plate 3—80% ethanol, 500 µl/well
- Elution Plate—Elution solution (from MagMAX kit), 60 µl/well.
7.In the BSL-2 hood, add 10 µl of proteinase K (from the MagMAX kit) to each well of a 96-well deep-well plate (label as Sample Plate).
8.Add 200 µl of specimen to each well of the Sample Plate, and mix well by pipetting up and down gently.
9.Add 275 µl of binding bead mix to each well of the Sample Plate using a multichannel pipettor.
10.Select the MVP_3Wash_200 Flex program on the Kingfisher machine.
11.Start the run, load the prepared plates in the respective positions when prompted by the instrument.
12.After the run completes (∼25 min), remove the elution plate (Plate 5). Seal the plate with adhesive film and store the plate at −80°C.
13.Wipe down the hood with freshly prepared bleach (0.5%) followed by 80% ethanol. Turn on the UV light.
Basic Protocol 2: MULTIPLEX ONE STEP RT-qPCR PROTOCOL TO DETECT SARS-CoV-2
This protocol detects the S and E genes of SARS-CoV-2 and the cellular RNaseP gene in a multiplex one-step RT-qPCR reaction with primers and probes that bind the S and E genes of SARS-CoV-2.The S and E gene probes are labeled with HEX and FAM fluorochromes, respectively, and as such amplification of each target can be visualized separately. In addition, the reaction also contains primers and probe to amplify the RNaseP gene/mRNA. The RNaseP probe is labeled with Cy5.Amplification of the RNaseP gene indicates good sample quality, adequate nucleic acid extraction, and absence of PCR inhibitors in the sample, thus ruling out false negative results. If done properly, every specimen should show an amplification curve for RNaseP. Specimens that contain SARS-CoV-2 show PCR amplification curves for both S and E genes (see Fig. 2). In an occasional positive specimen, only the S or the E amplification curve is present. This can occur if the viral load in the specimen is low (Ct value > 32) or if there are mutations in the primer/probe binding regions of one or the other gene. For example, if there are mutations or deletions in the primer/probe-binding regions of the S gene, then only the E gene will amplify, indicating the presence of a variant in the specimen.
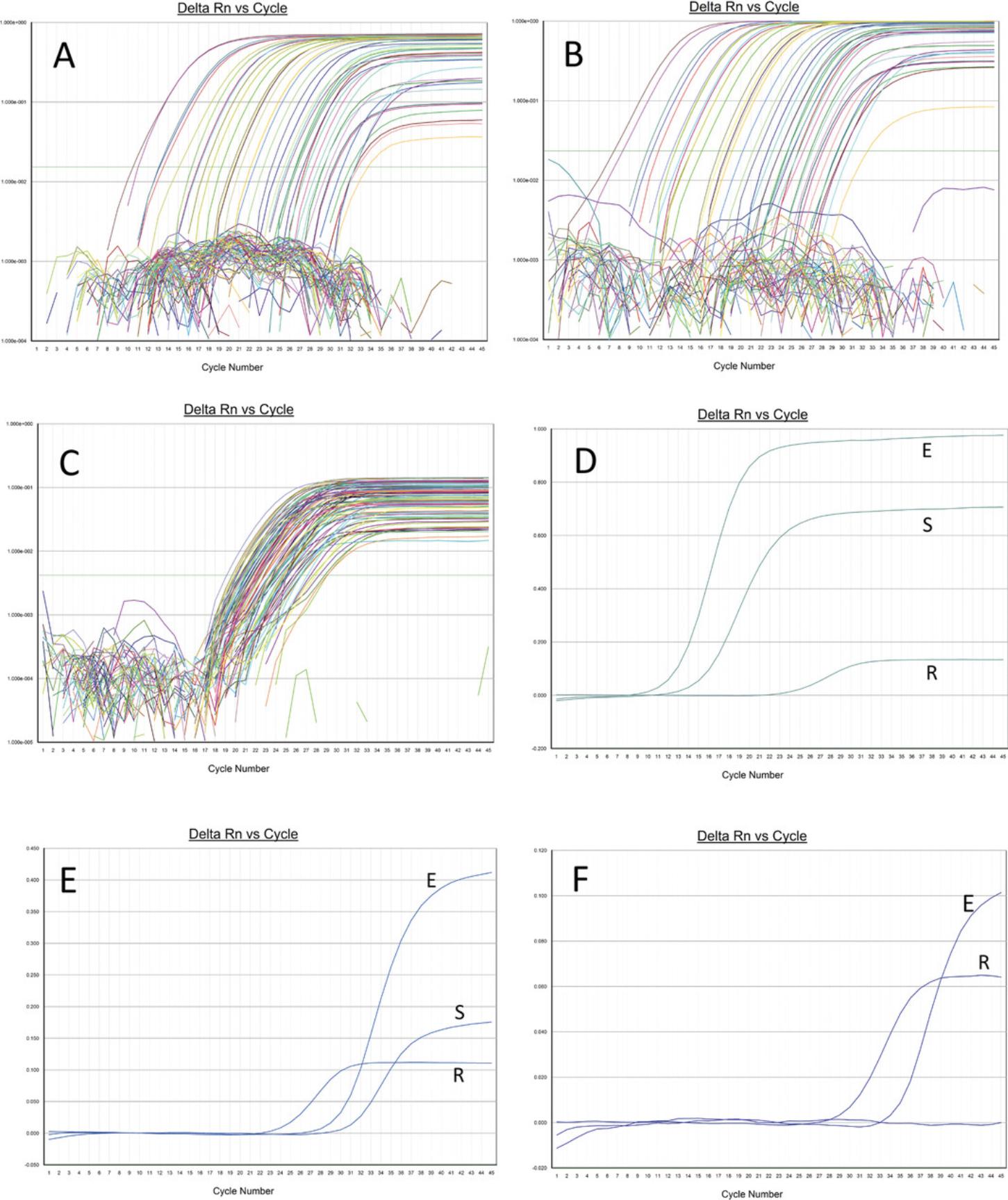
Materials
- 1 M Tris·HCl buffer, pH 7.5 (Thermofisher, cat. no. 15567027)
- Primers and probes (see Table 1, custom ordered)
- Primers must be purified by desalting. Probes must be purified by HPLC. The dual-labeled probes are labeled with a fluorochrome on the 5 ′ end and a quencher on the 3 ′ end. During the annealing/extension step of PCR, the probe binds the antisense strand of DNA. As the forward primer is extended, the probe is hydrolyzed by the 5 ′ exonuclease activity of Taq polymerase, separating the fluorochrome from the quencher. The emission from the fluorochrome is then captured by the detector.
- Probes must be protected from light by covering the tube with aluminum foil when kept outside the freezer.
Sequence identity | |||||
---|---|---|---|---|---|
Primer/probe | Sequence, 5′>3′ | SARS-CoV-2 | SARS-CoV | MERS | Amplicon |
Sgene-F2 | AACTCAATTACCCCCTGCATAC | 22/22 | 14/22 |
0/22 22/22 deleted |
167 bp |
Sgene-R2 | TAGTACCATTGGTCCCAGAGACA | 23/23 |
2/23 18/23 deleted |
15/23 | |
SgeneProbe2 (HEX) | HEX TCAGATCCTCAGTTTTACATTCAACTCAGGACTTG BHQ1 | 35/35 | 21/35 | 10/35 | |
Egene-F2 | TCATTCGTTTCGGAAGAGACAG | 22/22 | 21/22 | 11/22 | 103 bp |
Egene-R2 | GCGCAGTAAGGATGGCTAGT | 20/20 | 20/20 | 12/20 | |
EgeneProbe2 (FAM) | FAM AGCGTACTTCTTTTTCTTGCTTTCGTGGTATTCT BHQ1 | 34/34 | 34/34 | 10/34 | |
RNaseP-F | AGATTTGGACCTGCGAGCG | 65 bp | |||
RNaseP-R | GAGCGGCTGTCTCCACAAGT | ||||
RNaseP probe (Cy5) | Cy5 TTCTGACCTGAAGGCTCTGCGCG BHQ1 |
-
aThe S and E gene primers and probe were designed using Oligo v6.64 software using the SARS-CoV-2 reference sequence, NC_045512.2. The RNaseP primers and probe are CDC-designed sequences. The Sgene primers and probe are specific to SARS-CoV-2. The Egene primers and probe will detect SARS-CoV in addition to SARS-CoV-2. The S and E gene primers and probe will not detect MERS
- Dedicated lab coats (for each different Area)
- Real-time PCR machine (ABI7500FastDx, ABI7500, Quantstudio 7 Flex or equivalent)
- The real-time PCR machine must be calibrated to detect HEX, FAM, and Cy5 fluorochromes.
- Dead air boxes for Areas 2 and 3 (AirClean 600 PCR workstation or equivalent; https://www.aircleansystems.com/products/dead-air-box)
- Vortex, placed in the dead air box
- 1.5-ml microcentrifuge tubes, DNase-/RNase-free (Fisher Scientific, cat. no. 05-408-129)
- 2.0-ml microcentrifuge tubes, DNase-/RNase-free (Fisher Scientific, cat. no. 05-408-138)
- Minicentrifuge (Fisher Scientific, cat. no. 12-006-01), placed in the dead air box
- PCR-Cooler, 0.2 ml (Eppendorf, cat. no. 022510509 or equivalent), for Area 2 and Area 3
- MicroAmp™ Fast Optical 96-Well Reaction Plate or equivalent
- Aluminum foil
- Microamp clear adhesive film (Fisher Scientific, cat. no. 43-063-11)
- Pipettors: 2-20 µl, 20-200 µl, 100-1000 µl
- Multichannel pipettor (Fisher Scientific cat. no. FA10015TG or equivalent)
- Pipette tips: 20 µl, 200 µl, 1000 µl, DNase RNase free with aerosol filters
- Mini Plate Spinner Centrifuge (Fisher Scientific, cat. no. 14-100-141/143)
Diluting the primers and probe
1.Prepare 10 mM Tris·HCl, pH 7.5, from the 1 M stock solution as follows:
Add 100 µl of 1 M Tris·HCl, pH 7.5, to 9.9 ml of distilled water, and mix well.
2.Add 10 mM Tris·HCl, pH 7.5, to the primer tubes to a final concentration of 100 pmol/µl (stock primers).
3.Dilute the primers to the working concentrations in distilled water as follows:
- Sgene-F2 and Sgene R2: 10 pmol/µl
- Egene-F2 and Egene R2: 1 pmol/µl
- RNaseP-F and RNaseP-R: 0.5 pmol/µl.
4.Add 10 mM Tris·HCl, pH 7.5, to the probe tubes to a final concentration of 100 pmol/µl (stock probes).
5.Dilute the probes to the working concentrations as follows in 10 mM Tris·HCl, pH 7.5:
- SgeneProbe2 (HEX): 5 pmol/µl
- EgeneProbe2 (FAM); 2 pmol/µl
- RNaseP Probe (Cy5): 0.5 pmol/µl.
6.Store all primers and probes at −20°C in Area 2 (see Strategic Planning).
Setting up the one step RT-qPCR program and run file and on the real-time PCR machine
7.Open the real-time PCR software program.
8.Set up a one-step RT-qPCR program with the following conditions:
- Step 1: 50o 20 min (reverse transcription step)
- Step 2: 95o 3 min (initial denaturation)
- Step 3: 95o 10 s (denaturation)
- Step 4: 56o 20 s (annealing/extension)
- 45 cycles of PCR starting from step 3.
- Fluorescence must be acquired at each PCR cycle in step 4.
9.Set up the PCR plate map on the real-time PCR machine and save in a folder on the computer attached to the machine. Enter specimen IDs for each well that will be analyzed.
10.Select HEX/FAM/Cy5 detectors for each well.
11.Print out the plate map and take to Area 3.
Perform steps (RT-qPCR)
The following steps are performed in Area 2
12.Wear the dedicated lab coat in Area 2.
13.Turn off the light inside the box to prevent damage to the probes.
14.Thaw the TaqPath RT-qPCR mix and working dilutions of the primers/probe inside the dead air box.
15.Wipe the tubes dry, briefly vortex to mix, and spin in the minicentrifuge to collect the contents at the bottom of the tube.
16.Prepare the mastermix in the dead air box in Area 2 in a 1.5-ml or 2-ml microcentrifuge tube according to Table 2.
Multiply the “Per reaction” values by the number of specimens that need to be analyzed. Add 2-4 reactions extra to cover for pipetting loss.
Per reaction | Amt./reaction | Conc. | |
---|---|---|---|
4× TaqPath 1-Step RT-qPCR mix, CG | 6.25 µl | 1× | |
Sgene-F2 (10 pmol/µl) | 1.00 µl | 10 pmol | 0.4 μM |
Sgene-R2 (10 pmol/µl) | 1.00 µl | 10 pmol | 0.4 μM |
SgeneProbe2 HEX (5 pmol/µl) | 1.00 µl | 5 pmol | 0.2 μM |
Egene-F2 (1 pmol/µl) | 1.50 µl | 1.5 pmol | 0.06 μM |
Egene-R2 (1 pmol/µl) | 1.50 µl | 1.5 pmol | 0.06 μM |
EgeneProbe2 FAM (2 pmol/µl) | 1.00 µl | 2.0 pmol | 0.08 μM |
RNaseP-F (0.5 pmol/µl) | 1.00 µl | 0.5 pmol | 0.02 μM |
RNaseP-R (0.5 pmol/µl) | 1.00 µl | 0.5 pmol | 0.02 μM |
RNaseP Probe Cy5 (0.5 pmol/µl) | 1.00 µl | 0.5 pmol | 0.02 μM |
PCR-grade water | 3.75 µl | ||
Total | 20.00 µl |
17.Place a MicroAmp™ Fast Optical 96-Well Reaction Plate in the PCR-cooler box inside the dead air box.
18.Pipet 20 µl of the mastermix into each well of the PCR plate.
19.Add 5 µl of PCR-grade water to the well that will be designated “NTC” (no template control).
20.Cover the PCR plate with aluminum foil.
21.Return all reagents to the −20°C freezer.
22.Remove the PCR plate from the dead air box, close the dead air box, and turn on the UV light.
23.Remove the lab coat and keep in Area 2 itself.
24.Take the PCR plate to Area 3.
The following steps are performed in Area 3
25.Wear the dedicated lab coat in Area 3.
26.Place the PCR plate containing the mastermix on the PCR-cooler inside the dead air box.
27.Add 5 µl of specimen nucleic acid extract to each well according to the plate map that was previously prepared.
28.Add 5 µl of positive control extract to the well designated as “positive control.”
29.Seal the PCR plate with adhesive film.
30.Spin the plate for 10 s in the Mini Plate Spinner centrifuge to collect the contents to the bottom of the wells.
The following steps are performed in Area 4
31.Insert the PCR plate in the real-time PCR machine and start the run. The run takes about 1 hr 30 min.
Run analysis
32.Click the Auto-baseline option.
33.Click the “Analyze” button.
34.Adjust the threshold to the mid log phase of the positive curves (between 0.01 and 0.05 on the ABI machines).
Alternate Protocol 3: MULTIPLEX ONE-STEP RT-qPCR PROTOCOL TO DETECT SARS-CoV-2 WITH S AND E PROBES LABELED WITH THE SAME FLUOROCHROME
The alternate protocol is exactly the same as Basic Protocol 2, except that the S and E gene probes are labeled with the same fluorochrome, FAM. As such, a single amplification curve is observed in SARS-CoV-2-positive cases, and amplification from the S and E genes cannot differentiated. The advantage over the Basic Protocol 2 is that the signal is additive and more robust. The internal control RNaseP signal can still be differentiated because the probe is labeled with Cy5. This alternate protocol is useful if there is no need to differentiate between amplification of the S and E genes.
Additional Materials (also see Basic Protocol 2)
- Alternate SgeneProbe2 labeled with FAM,
- SgeneProbe2 (FAM): FAM TCAGATCCTCAGTTTTACATTCAACTCAGGACTTG BHQ1
In Basic Protocol 2, under “Setting up the one step RT-qPCR program and run file and on the real-time PCR machine,” at step 10, select FAM/Cy5 detectors for each well. Under “Perform RT-qPCR,” step 16, replace HEX-labeled SgeneProbe2 with FAM-labeled SgeneProbe2 at the same concentration.
COMMENTARY
Background Information
SARS-CoV-2 is a highly infectious betacoronavirus that has spread throughout the world. Controlling the infection starts with detecting the virus followed by isolation of infected individuals (Kucharski et al., 2020). Molecular and antigen/antibody tests can detect SARS-CoV-2. Molecular tests are superior to antigen/antibody tests because of their high sensitivity and specificity. Molecular tests can also detect the virus in the early asymptomatic phase after exposure when an individual shows no clinical signs of infection, but can spread the virus to others. Antigen/antibody tests become positive only after individuals becomes symptomatic. As such, molecular tests are the preferred method to detect the infection.
SARS-CoV-2 is a single-stranded positive-sense RNA virus 29,903 nucleotides in length (Abdelrahman et al., 2020; Wang et al., 2020). The RNA genome is enclosed within a lipid envelope that contains the Spike(S) and Envelope (E) proteins. The nucleocapsid protein (N) is associated with the RNA genome inside the virus. The virus attaches to ACE2 receptors on the host cell surface using the Spike protein. Cleavage of the Spike protein into S1 and S2 units by cell surface receptor TMPRSS2 is essential for attachment to the host cell surface. Once attached, S2 mediates fusion of the viral envelope with the host cell membrane, thereby releasing the viral genome into the host cell cytoplasm (Shang et al., 2020; Harrison, Lin, & Wang, 2020). The RNA genome codes for a polyprotein that is cleaved into several smaller proteins by the protease, 3CLPro (Zhang et al., 2020). RNA-dependent RNA polymerase (RdRp) transcribes the viral genome in the initial steps of replication, followed by translation of viral proteins. The genome and the proteins are then packaged into nascent virions. These virions are released upon rupture of the cell membrane and infect adjacent cells to cause widespread infection of epithelial surfaces. The initial site of infection is believed to be the epithelial cells lining the mouth along with the ductal and acinar cells of the minor salivary glands (Liu et al., 2011). The mucosal cells lining the tongue, oropharynx, and nasopharynx can also be sites of initial infection. At this timepoint, the individual can be asymptomatic or show minor symptoms such as sore throat, running nose, loss of taste and smell, mild fever, and malaise. Almost 85% of individuals recover without serious sequalae. In 10%-15% of individuals, the viral infection spreads to the lower respiratory tract and into the lungs. The virus infects pneumocytes that line the alveoli, causing cell lysis that leads to disruption of the alveolar basement membrane. Inflammation ensues, with resultant pneumonia that can resolve with supportive therapy or progress to adult respiratory distress syndrome (ARDS). A subset of patients progress towards intractable ARDS and ultimately die despite ventilator support.
Nasopharyngeal/oropharyngeal swabs and saliva are most often analyzed to detect SARS-CoV-2 infection (Azzi et al., 2020; Butler-Laporte et al., 2021; Wang et al., 2020). Broncho-alveolar lavage fluid is also an excellent specimen type to detect SARS-CoV-2, but is infrequently collected because of the invasive nature of collection. With high viral loads such as in early symptomatic patients, saliva and NP/OP swabs most often yield positive results. However, in the early asymptomatic or late convalescent phase of infection, one or the other specimen type may be positive. In such cases, it may be necessary to test the individual repeatedly to detect the infection.
One-step reverse transcription and real-time PCR (RT-qPCR) is a highly sensitive and specific technique to detect SARS-CoV-2. Other molecular methods such as transcription-mediated amplification (Gorzalski et al., 2020), loop-mediated amplification (Lamb, Bartolone, Ward, & Chancellor, 2020), and CRISPR (Ding et al., 2020) based methods have been described. However, one-step RT-qPCR has the advantage of being a frequently used technique that is highly sensitive and specific to detect the virus within a short turn-around time of about 3-4 hr. Materials and instrumentation for real-time PCR are readily available in most laboratories, whereas the other molecular methods mentioned require special reagents and esoteric protocols to execute. Moreover, both reverse transcription and real-time PCR are performed in the same reaction without the need for separate steps, thereby reducing hands-on time and laboratory error. Just like other RNA viruses, SARS-CoV-2 tends to mutate over time. Hence, it is prudent to design protocols that detect more than one target to avoid false negative results. Individual viral targets can be detected in separate reactions or within the same reaction. For example, the CDC-designed assay detects different regions of the N gene of the virus in two separate reactions. Both reactions have to be positive to call the virus detected. A third reaction is used to detect an internal control target, RNaseP. RNaseP is a cellular gene that should be present in all samples. If RNaseP amplifies, it means that the sample was adequate, nucleic acid extraction was optimal, and there was no RT-PCR inhibition ruling out false negative results. Disadvantages of analyzing SARS-CoV-2 targets and the internal control in separate reactions are that few specimens can be analyzed in a run, as well as the added cost of additional reagents and consumables. Multiplex PCR reactions allow the detection of many targets in the same reaction, thereby increasing throughput and reducing cost. For example, on a 96-well PCR plate, if two SARS-CoV-2 targets and an internal control were to be analyzed as individual reactions, only 30 specimens could be analyzed in a run. In a multiplex format, 94 specimens can be analyzed in a run with just one third the amount of RT-PCR reagents. However, multiplex RT-PCR reactions are more complex to design because, unlike a reaction with a single set of primers and probe, multiplex reactions contain many primers and probes that could interact with each other, thereby producing nonspecific amplification or primer dimers. Careful attention to primer/probe design and optimization of RT-PCR conditions are needed to avoid these problems and result in highly sensitive and specific assays. The protocol reported in this article detects two targets of SARS-CoV-2 (S and E genes) and the internal control RNaseP all within the same reaction. The primers and probes were designed to avoid nonspecific amplification, and their concentrations were optimized to obtain near equivalent amplification of both targets including the internal control. Initially, we tested different combinations of S, E, N, RdRp, and Orf1ab in multiplex RT-qPCR assays, along with the internal control RNaseP. S and E targets with RNaseP gave us the most optimal amplification curves, and hence this combination was chosen for the multiplexing.
We used the TaqPath 1-Step RT-qPCR mix in our protocol (Thermofisher) because it gave us the most optimal results. Reagents from other vendors can also be used after the assay has been adequately validated. The composition of the mixes is proprietary, and differences in composition can affect the results.
The real-time PCR probes used in the protocol are dual-labeled probes that have a fluorochrome attached to the 5′ end and a quencher at the 3′ end. In the native state, the quencher absorbs any fluorescence emitted by the fluorochrome. In the annealing phase of PCR, both the forward primer and the probe bind the antisense strand of cDNA that was generated by reverse transcription. The probe binds downstream of the forward primer. During the extension phase of PCR, as Taq polymerase extends the forward primer, it encounters the downstream probe attached to the antisense strand. The 5′ exonuclease activity of Taq polymerase hydrolyses the probe, thereby releasing the fluorochrome into the PCR solution. The fluorochrome is now separated from the quencher and emits its fluorescence when exited by a certain wavelength of light. The emitted fluorescence is then captured by a detector and processed by the software. At each cycle of PCR, the emitted fluorescence is additive and results in a sigmoidal curve because PCR amplification is a logarithmic process. After the instrument software analyzes the run, the threshold, a horizontal line that runs across the PCR curves, is generally placed in the early logarithmic portion of the curves. The cycle number at which the PCR curve cuts the threshold line is called the Ct value. The Ct value is inversely proportional to the starting viral load. If a specimen contained a high viral load, the Ct value will be early, between 15 and 30. If a specimen contained a low viral load, the Ct value will generally be >30.
RNaseP, a cellular gene, is analyzed in the multiplex RT-qPCR reaction as an internal control in each specimen to prevent false negative results for SARS-CoV-2. A false negative result for SARS-CoV-2 can occur if (1) the specimen was not from a human source; (2) nucleic acid extraction failed; or (3) the specimen contained RT-qPCR inhibitors. The Ct value for RNaseP must be <32 to be considered valid. RNaseP is a cellular gene that is endogenous to each specimen because NP/OP swabs as well as saliva contain host inflammatory cells and epithelial cells that are shed from the mucosal surface. As such, RNaseP amplification confirms that the sample was indeed derived from a human source, and at the same time indicates that nucleic acid extraction was adequate and that there were no RT-PCR inhibitors. Therefore, amplification of RNaseP rules out false negative results for SARS-CoV-2. The primers for RNaseP are located in exon 1 and amplify both the gene and RNaseP cDNA derived after reverse transcription. In many other protocols including some that have an FDA Emergency Use Authorization (EUA), an exogenous synthetic RNA is spiked into the sample, carried through extraction, and analyzed by RT-qPCR. This approach is good only to rule out extraction failure and PCR inhibition. However, it does not control for sample adequacy or integrity. For example, if the sample analyzed is just plain water, a negative result will be generated for SARS-CoV-2, but the spiked internal control will amplify, indicating optimum assay performance, while in reality the sample itself was not adequate to begin with. The amount of RNaseP primers was purposefully kept low and optimized to get unequivocal amplification, yet not compete out S or E gene amplification in the multiplex RT-qPCR reaction. As such, the analytical sensitivity of S and E detection is high and is not impeded by RNaseP amplification.
Analytical sensitivity
The analytical sensitivity or the Limit of Detection (LOD) is the lowest amount of virus/ml of specimen that can be detected with a 95% frequency. To determine the LOD, we obtained quantified heat-inactivated whole virus from ATCC (VR-1986HK) and spiked known amounts of virus into negative specimens, extracted them, and then analyzed them with the protocol. The analytical sensitivity was determined to be 375 copies/ml (Fig. 3). Below this level, the detection frequency was < 95%.
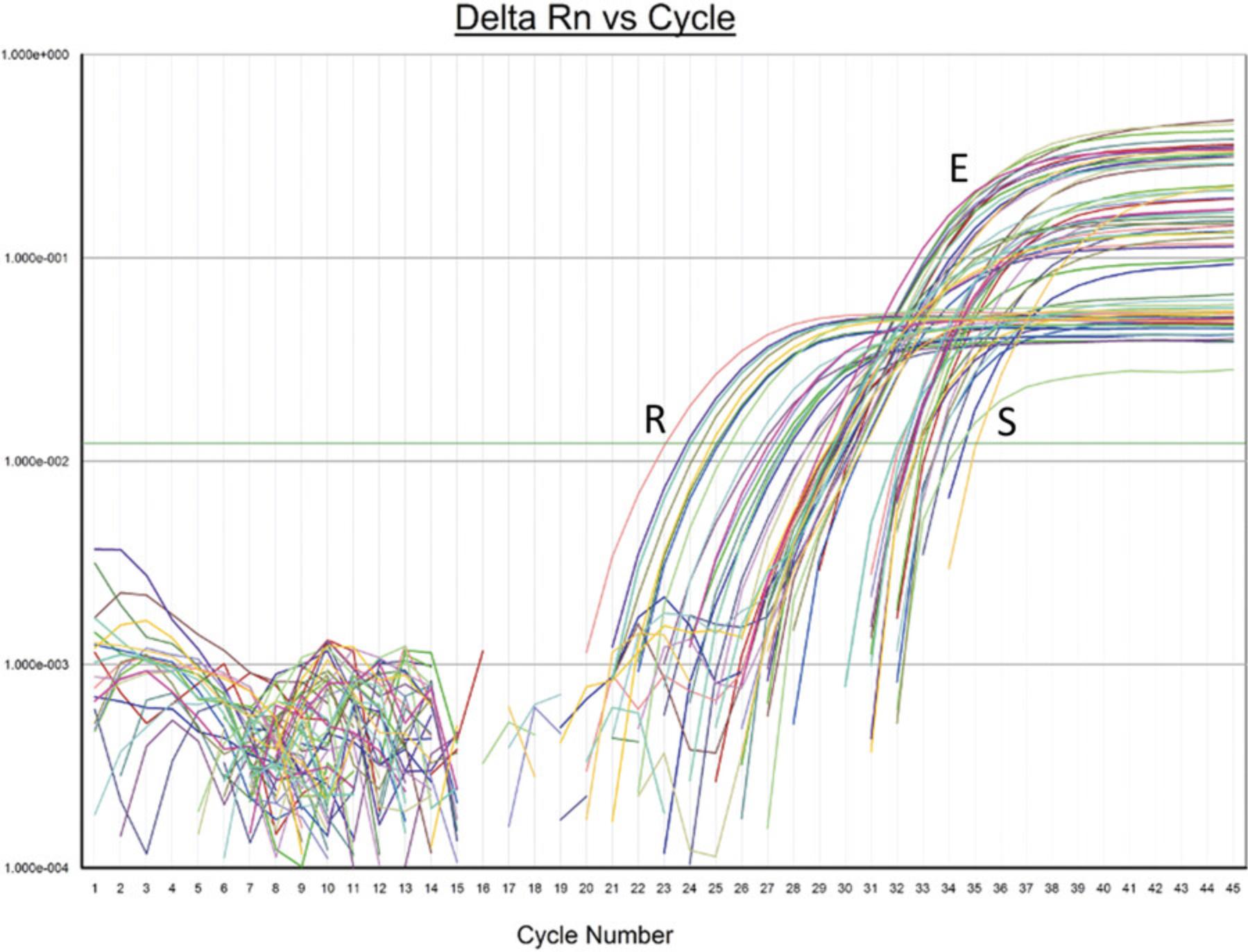
Analytical specificity
We analyzed specimens from previous years that were positive for common respiratory pathogens—influenza A and B; parainfluenza; RSV; hMPV; the other human coronaviruses 229E, NL63, OC43, and HKU1; adenovirus; rhinovirus; Streptococcus pneumoniae , and Haemophilus influenzae. All these specimens were negative by the multiplex RT-qPCR assay, indicating that the assay is highly specific. In silico alignment analysis using the NCBI BLAST program shows that the Sgene primers are specific to SARS-CoV-2 and will not amplify SARS-CoV or MERS. The Egene primers will in addition amplify SARS-CoV, but not MERS. This is not a problem in the current pandemic because SARS-CoV is not circulating at the present time.
Validation of the E/S/RNaseP multiplex RT-qPCR protocol against the CDC N1/N2/RNaseP assay and the Abbott m2000 SARS-CoV-2 real-time PCR assay (FDA EUA assays)
The CDC and Abbott m2000 assays are qualified only for NP/OP swabs and not saliva. NP/OP swabs collected in M4RT viral transport medium or saline were extracted by the EZ1 Advanced method (low throughput) as described in Alternate Protocol 1 and analyzed by the CDC N1/N2/RNaseP assay according to the package insert for initial clinical testing. Nucleic acid extracts were then re-analyzed by the multiplex E/S/RNaseP RT-qPCR protocol (25 positives, 20 negatives each for M4RT and saline collections). The positive and negative percent agreements were 100% with the CDC assay. NP/OP swabs collected in Abbott Universal collection tubes were initially analyzed by the Abbott m2000 real-time PCR assay for clinical testing. The specimens were re-extracted on the Kingfisher machine (high throughput) according to Alternate Protocol 2 and analyzed by the multiplex E/S/RNaseP RT-qPCR protocol (50 positives, 44 negatives). The positive and negative percent agreements were 96% and 100%, respectively, with the Abbott m2000 assay results. These results show that the E/S/RNaseP multiplex RT-qPCR is equivalent to two assays that have received EUA authorization from the FDA.
Critical Parameters
It is important to observe good laboratory practices when performing this protocol. Because PCR can amplify the starting template billions of times, it is critically important to avoid template and amplicon contamination by following a unidirectional work flow as suggested in the Strategic Planning section. Separate rooms or segregated areas must be designated for nucleic acid extraction, master mix preparation, addition of nucleic acid extracts to the PCR plate, and thermocycling on a real-time PCR machine. If possible, the room where mastermix is prepared should be under positive pressure, and the room where thermocycling is done should be under negative pressure. Gowns, gloves, pipettors, and consumables must be designated for the different areas, and must not be interchanged. Addition of PCR mastermix and nucleic acid extracts must be done in dead air boxes in their respective rooms. The dead air boxes should have the UV light turned on after use. The surfaces and pipettors must be wiped clean with 80% ethanol after each use.
Every specimen must be considered infectious, and it is critically important to follow universal precautions when handling the specimens in the BSL-2 hood. Gowns, gloves, N95 mask, and face shield must be worn. The surface of the BSL-2 hood must be wiped down with diluted bleach followed by 80% ethanol after each use. To prevent degradation, nucleic acids must be extracted as soon as possible, generally within 2-4 hr after collection. If this is not possible, freeze the specimen at −80°C for later extraction. Specimens can be extracted after an indefinite period of time if frozen at −80oC. Store extracted nucleic acids at −80oC to prevent degradation.
Freeze primers, probes, and mastermix reagents in small aliquots to avoid repeated freeze-thawing that may lead to degradation and loss of enzymatic activity. Protect the probes from light by covering with aluminum foil. It is important not to pipet up and down when adding template to the wells of the PCR plate containing the mastermix. Pipetting can lead to frothing and result in unreliable results. It is important not to touch the pipet tips to other wells or surfaces, to prevent contamination. Pipetting technique is important and can be learnt only through experience.
A positive and negative control must be included in every run. The positive control amplification indicates that all components of the protocol are working as expected. If the positive control fails amplification, the results from the samples are unreliable. Set up the RT-qPCR protocol again with a fresh aliquot of the positive control. The negative control reaction contains water as template. The water should be from the same aliquot that was used to make the mastermix. A negative control that does not show any amplification curve indicates that there was no contamination in the run. If an amplification curve is seen in the negative control, negate the results and start over by re-extracting the specimens. It is necessary to investigate the source of contamination, and only after the issue is resolved should testing of specimens start again.
When setting up the real-time PCR program, choose the appropriate detectors for the wells depending on the fluorochromes that are attached to the probes. On ABI machines, HEX is detected by the VIC detector. If not done correctly, the instrument will not collect data from that detector.
Troubleshooting
Please see Table 3.
Problem | Possible cause | Solution |
---|---|---|
No RNaseP amplification or RNaseP Ct >32 from a sample, but other samples show amplification curves with Ct < 32 |
Sample not analyzed within 2-4 hr after collection causing nucleic acid degradation Sample stored improperly in the refrigerator (4o) or at room temperature, and not at −80°C causing nucleic acid degradation |
Analyze sample when fresh |
Extraction failure | Re-extract sample and analyze | |
RT-PCR inhibitors in the sample | Obtain fresh specimen and analyze | |
Multiple amplification curves bunching up together with similar Ct values | Contamination | Re-extract and analyze after thorough cleaning and decontamination of all areas |
Negative control shows amplification curve for RNaseP or S/E genes | Contamination | Re-extract and analyze after thorough cleaning and decontamination of all areas |
No amplification curves in all samples—including positive control | Mastermix prepared incorrectly | Prepare mastermix correctly |
Primers/probes degraded | Prepare fresh dilutions of primers/probes from stock solutions | |
Enzymes in TaqPath mastermix degraded | Use an unopened aliquot of Taqpath mastermix | |
Improperly selected dye detector | Select the correct dye detector in the RT-qPCR setup | |
Odd-looking amplification curves that are not logarithmic | Look at the multicomponent plot. Dye lines not horizontal and or are irregular | Repeat analysis from nucleic acid eluate |
Understanding Results
A successful run should generate logarithmic curves for RNaseP in every specimen (Fig. 2C). For each specimen, the RNaseP amplification curve must be sigmoidal when looked at in the linear mode on the Y axis and have a Ct value of <32.An RNaseP Ct value >32 generally indicates nucleic acid degradation. The amplification curves for the S and E genes must also be sigmoidal when looked at in the linear mode (Fig. 2D and E). If the RNaseP Ct is <32 and there is no amplification curve for the S and E genes, then it means that SARS-CoV-2 RNA is not present in the specimen above the detection limit of the assay (375 copies/ml).
Interpretation of results
Please see Table 4.
Sgene (HEX) | Egene (FAM) | RNaseP (Cy5) | Result | Comments |
---|---|---|---|---|
Present | Present | Present | Positive | SARS-CoV-2 RNA is present in the specimen |
Absent | Absent | Present | Negativea | SARS-CoV-2 RNA is absent in the specimen |
Absent | Present | Present | Positive |
SARS CoV-2 RNA is present in the specimen Possible variant with mutation in Sgene primer/probe binding sites, if Ct of Egene curve is < 30 Very low viral load (if Ct > 32) Repeat analysis and sequence to confirm |
Present | Absent | Present | Positive |
SARS CoV-2 RNA is present in the specimen Possible variant with mutation in Egene primer/probe binding sites if Ct of Sgene curve is <30 Very low viral load (if Ct > 32) Repeat analysis and sequence to confirm |
Absent | Absent | Absent | Invalid | See troubleshooting (Table 3) |
- a A negative result does not rule out the presence of SARS-CoV-2 RNA below the analytical sensitivity of the protocol. A negative result can also occur if there are sequence alterations in the primer/probe binding sites of the S and E genes resulting in failure of amplification.
Note about B.1.1.7, B.1.351, and P.1 variants
The B.1.1.7, B.1.351, and P.1 variants of SARS-CoV-2 contain multiple mutations and are of concern because of their enhanced capacity for transmission and possibly increased virulence. We determined if the protocol could detect these variants as efficiently as the reference strain. The primers (Sgene-F2, Sgene-R2) and probe sequence (SgeneProbe2) are 100% identical to B.1.351, the South African variant, and as such the Sgene part of the protocol should work as efficiently as for the reference strain of SARS-CoV-2 to detect this variant. However, there is a 1-nt mismatch in the Sgene-R2 when compared to B.1.1.7, the London variant, and a 1-nt mismatch in Sgene-F2 compared to P.1, the Brazil variant. We have confirmed using Sgene in vitro transcribed RNA from B.1.1.7 and P.1 that the assay is as efficient in detecting these variants as the reference strain. The Egene primers and probe are 100% identical to the Egenes of the three variants. Nevertheless, it is prudent to check the primer/probe sequences for alignment with SARS-CoV-2 sequences deposited in Genbank or GISAID databases periodically to determine alignment to new variants.
Time Considerations
Basic Protocol Basic Protocol 1: Viral nucleic acid extraction
Manual, 45 min for 6-8 specimens
Low throughput (Qiagen EZ1), 1 hr for 14 specimens
High throughput (Kingfisher), 1 hr for 96 specimens
Basic Protocol Basic Protocol 2: Multiplex RT-qPCR
Preparing the mastermix, 30 min
Adding nucleic acid extracts to PCR plate, 30 min
PCR run on the thermocycler, 2 hr
Acknowledgments
This work was supported by the Department of Pathology at the University of Illinois at Chicago, Chicago, IL. We are very grateful to Dr. Fred Behm (Department Head), Dr. Sally Campbell-Lee (Director of Clinical Pathology), and Mr. Peter Gashkoff (Administrative Director) for their unflinching support. We are also grateful to the entire staff of the Molecular Pathology Laboratory, Carol Dodge (Manager), Lynn Terrile, and Mei Shen (Supervisors) and Jyothimayi Ryali, Ermias Weldeyohannes, Adrian Tira, and, Meihong Zhang for their support.
Author Contributions
Joshua Bland: Conceptualization, Data curation, Formal analysis, Investigation, Methodology, Project administration, Resources, Software, Supervision, Validation, Writing-review & editing, Ashley Kavanaugh: Data curation, Formal analysis, Methodology, Project administration, Resources, Supervision, Validation, Writing-review & editing, Lenny K. Hong: Formal analysis, Methodology, Resources, Validation, Writing-review & editing, Omar Perez: Formal analysis, Methodology, Resources, Writing-review & editing, Shrihari S. Kadkol: Conceptualization, Data curation, Formal analysis, Funding acquisition, Investigation, Methodology, Project administration, Resources, Software, Supervision, Validation, Visualization, Writing-original draft, Writing-review & editing.
Conflict of Interest
There are no conflicts of interest to report for any of the authors.
Open Research
Data Availability Statement
The data that support the findings of this study are available on request from the corresponding author. The data are not publicly available due to privacy or ethical restrictions.
Literature Cited
- Abdelrahman, Z., Li, M., & Wang, X. (2020). Comparative review of SARS-CoV-2, SARS-CoV, MERS-CoV, and influenza a respiratory viruses. Frontiers in Immunology , 11, 552909. doi: 10.3389/fimmu.2020.552909.
- Azzi, L., Maurino, V., Baj, A., Dani, M., d'Aiuto, A., Fasano, M., … Alberio, T. (2020). Diagnostic salivary tests for SARS-CoV-2. Journal of Dental Research , 100(2), 115–123. doi: 10.1177/0022034520969670.
- Butler-Laporte, G., Lawandi, A., Schiller, I., Yao, M., Dendukuri, N., McDonald, E. G., … Lee, T. C. (2021). Comparison of saliva and nasopharyngeal swab nucleic acid amplification testing for detection of SARS-CoV-2: A systematic review and meta-analysis. JAMA Internal Medicine , 181(3), 353–360. doi: 10.1001/jamainternmed.2020.8876.
- Díaz, J. (2020). ARS-CoV-2 molecular network structure. Frontiers in Physiology , 11, 870. doi: 10.3389/fphys.2020.00870.
- Ding, X., Yin, K., Li, Z., Lalla, R. V., Ballesteros, E., Sfeir, M. M., & Liu, C. (2020). Ultrasensitive and visual detection of SARS-CoV-2 using all-in-one dual CRISPR-Cas12a assay. Nature Communications , 11(1), 4711. doi: 10.1038/s41467-020-18575-6.
- Fung, B., Gopez, A., Servellita, V., Arevalo, S., Ho, C., Deucher, A., … Miller, S. (2020). Direct comparison of SARS-CoV-2 analytical limits of detection across seven molecular assays. Journal of Clinical Microbiology , 58(9), e01535–20. doi: 10.1128/JCM.01535-20.
- Gorzalski, A. J., Tian, H., Laverdure, C., Morzunov, S., Verma, S. C., VanHooser, S., & Pandori, M. W. (2020). High-throughput transcription-mediated amplification on the Hologic Panther is a highly sensitive method of detection for SARS-CoV-2. Journal of Clinical Virology , 129, 104501. doi: 10.1016/j.jcv.2020.104501.
- Harrison, A. G., Lin, T., & Wang, P. (2020). Mechanisms of SARS-CoV-2 transmission and pathogenesis. Trends in Immunology , 41(12), 1100–1115. doi: 10.1016/j.it.2020.10.004.
- Hirschhorn, J. W., Kegl, A., Dickerson, T., Glen, W. B. Jr, Xu, G., Alden, J., & Nolte, F. S. (2021). Verification and validation of SARS-CoV-2 assay performance on the Abbott m2000 and Alinity m systems. Journal of Clinical Microbiology , 59, JCM.03119-20. doi: 10.1128/JCM.03119-20.
- Kubina, R., & Dziedzic, A. (2020). Molecular and serological tests for COVID-19 a comparative review of SARS-CoV-2 coronavirus laboratory and point-of-care diagnostics. Diagnostics (Basel, Switzerland) , 10(6), 434. doi: 10.3390/diagnostics10060434.
- Kucharski, A. J., Klepac, P., Conlan, A. J. K., Kissler, S. M., Tang, M. L., Fry, H., … Edmunds, W. J. (2020). Effectiveness of isolation, testing, contact tracing, and physical distancing on reducing transmission of SARS-CoV-2 in different settings: A mathematical modelling study. CMMID COVID-19 working group. The Lancet Infectious Diseases , 20(10), 1151–1160. doi: 10.1016/S1473-3099(20)30457-6.
- Lamb, L. E., Bartolone, S. N., Ward, E., & Chancellor, M. B. (2020). Rapid detection of novel coronavirus/Severe Acute Respiratory Syndrome Coronavirus 2 (SARS-CoV-2) by reverse transcription-loop-mediated isothermal amplification. PLoS One , 15(6), e0234682. doi: 10.1371/journal.pone.0234682.
- Liu, L., Wei, Q., Alvarez, X., Wang, H., Du, Y., Zhu, H., … Chen, Z. (2011). Epithelial cells lining salivary gland ducts are early target cells of severe acute respiratory syndrome coronavirus infection in the upper respiratory tracts of rhesus macaques. Journal of Virology , 85(8), 4025–4030. doi: 10.1128/JVI.02292-10.
- Lu, X., Wang, L., Sakthivel, S. K., Whitaker, B., Murray, J., Kamili, S., … Lindstrom, S. (2020). US CDC real-time reverse transcription PCR panel for detection of Severe Acute Respiratory Syndrome Coronavirus 2. Emerging Infectious Diseases , 26(8), 1654–1665. doi: 10.3201/eid2608.201246.
- Satarker, S., & Nampoothiri, M. (2020). Structural proteins in Severe Acute Respiratory Syndrome Coronavirus-2. Archives of Medical Research , 51(6), 482–491. doi: 10.1016/j.arcmed.2020.05.012.
- Shang, J., Wan, Y., Luo, C., Ye, G., Geng, Q., Auerbach, A., & Li, F. (2020). Cell entry mechanisms of SARS-CoV-2. Proceedings of the National Academy of Sciences , 117(21), 11727–11734. doi: 10.1073/pnas.2003138117.
- Wang, M. Y., Zhao, R., Gao, L. J., Gao, X. F., Wang, D. P., & Cao, J. M. (2020). SARS-CoV-2: Structure, biology, and structure-based therapeutics development. Frontiers in Cellular and Infection Microbiology , 10, 587269. doi: 10.3389/fcimb.2020.587269.
- Wang, W., Xu, Y., Gao, R., Lu, R., Han, K., Wu, G., & Tan, W. (2020). Detection of SARS-CoV-2 in different types of clinical specimens. JAMA , 323(18), 1843–1844. doi: 10.1001/jama.2020.3786.
- Zhang, L., Lin, D., Sun, X., Curth, U., Drosten, C., Sauerhering, L., … Hilgenfeld, R. (2020). Crystal structure of SARS-CoV-2 main protease provides a basis for design of improved α-ketoamide inhibitors. Science , 368, 409–412. doi: 10.1126/science.abb3405.
Internet Resources
- https://www.cdc.gov/urdo/downloads/SpecCollectionGuidelines.pdf
- https://www.cdc.gov/coronavirus/2019-ncov/lab/guidelines-clinical-specimens.html
- https://static.yanyin.tech/literature/current_protocol/10.1002/cpz1.145/attachments/Appropriate%20Collection%20and%20Handling%20Methods%20of%20Respiratory%20Specimens.pdf
Detailed procedures to collect NP/OP swabs.
Specimen collection—saliva.
Genbank SARS-CoV-2 genomes database.
GISAID SARS-CoV-2 genomes database.
Citing Literature
Number of times cited according to CrossRef: 4
- Ali Vahidnia, Dennis Souverein, Sjoerd M. Euser, Milly Haverkort, Elise Noordhuis, Thomas Z. I. van Zijl, Jayant Kalpoe, Jan C. Sinnige, Bjorn L. Herpers, Similar Sensitivity of SARS-CoV-2 Detection in Oropharyngeal/Nasopharyngeal and Saliva Samples on the Hologic Panther Platform, Diagnostics, 10.3390/diagnostics13030347, 13 , 3, (347), (2023).
- Sushma M. Bhosle, Julie P. Tran, Shuiqing Yu, Jillian Geiger, Jennifer D. Jackson, Ian Crozier, Anya Crane, Jiro Wada, Travis K. Warren, Jens H. Kuhn, Gabriella Worwa, Duplex One-Step RT-qPCR Assays for Simultaneous Detection of Genomic and Subgenomic RNAs of SARS-CoV-2 Variants, Viruses, 10.3390/v14051066, 14 , 5, (1066), (2022).
- Blanka Wolszczak-Biedrzycka, Anna Bieńkowska, Stanisław Maksymowicz, Adaptation of medical laboratory scientists to workplace hazards – experiences from the COVID-19 pandemic, Frontiers in Public Health, 10.3389/fpubh.2022.997049, 10 , (2022).
- Phimon Atsawasuwan, Dhammacari Martin Del Campo, Laura Martin Del Campo, Grace Viana, Sriram Ravindran, Veerasathpurush Allareddy, Shrihari Kadkol, Positivity rates of SAR-CoV-2 infection in orthodontic patients at the orthodontic clinic, University of Illinois Chicago, PLOS ONE, 10.1371/journal.pone.0270311, 17 , 6, (e0270311), (2022).