Taste-Associative Learning in Rats: Conditioned Immunosuppression with Cyclosporine A to Study the Neuro-Immune Network
Stephan Leisengang, Stephan Leisengang, Manfred Schedlowski, Manfred Schedlowski, Martin Hadamitzky, Martin Hadamitzky, Laura Lückemann, Laura Lückemann
behaviorally conditioned immunosuppression
conditioned taste avoidance
cyclosporine A
learned immune responses
rats
taste-immune associative learning
Abstract
The pharmacological effects of an immunosuppressive drug, such as cyclosporine A (CsA), can be learned and retrieved by humans and animals when applying associative learning paradigms. This principle is based on Pavlovian conditioning, in which repeated presentation of an “unconditioned stimulus” (US; here, the drug CsA) is paired with exposure to a “conditioned stimulus” (CS; here, the novel taste of saccharin). Re-exposure to the CS at a later time leads to an avoidance behavior. Concomitantly, using this paradigm, animals exposed to the CS (saccharin) display immunosuppression, reflected by reduced splenic T-cell proliferation and diminished interleukin-2 and interferon-γ expression and release in ex vivo cultured splenocytes, mimicking the pharmacological effects of the US (CsA). Notably, this paradigm of taste-immune associative learning demonstrates the impressive abilities of the brain to detect and store information about an organism's immunological status and to retrieve this information, thereby modulating immunological functions via endogenous pathways. Moreover, conditioned pharmacological effects, obtained by means of associative learning, have been successfully implemented as controlled drug-dose reduction strategies as a supportive treatment option to optimize pharmacological treatment effects for patients’ benefit. However, our knowledge about the underlying neurobiological and immunological mechanisms mediating such learned immunomodulatory effects is still limited. A reliable animal model of taste-immune associative learning can provide novel insights into peripheral and central nervous processes. In this article, we describe protocols that focus on the basic taste-immune associative learning paradigm with CsA and saccharin in rats, where conditioned peripheral immunosuppression is determined in ex vivo cultured splenocytes. The behavioral protocol is reliable and adaptable and may pave the road for future studies using taste-immune associative learning paradigms to gain deeper insight into brain-to-immune-system communication. © 2022 The Authors. Current Protocols published by Wiley Periodicals LLC.
Basic Protocol 1 : Taste-immune associative learning with cyclosporine A
Basic Protocol 2 : Splenocyte isolation and cultivation to study stimulation-induced cytokine production
INTRODUCTION
Cyclosporine A (CsA) is an immunosuppressive drug that is frequently used to treat autoimmune diseases and allergies or to prevent rejection of organs after transplantation (White, 1982). The immune response is attenuated via inhibition of both T-cell activation and release of T cell–associated cytokines [e.g., interleukin (IL)-2, interferon (IFN)-γ] (Steinbach, Reedy, Cramer, Perfect, & Heitman, 2007). Interestingly, this immunopharmacological effect of CsA can be conditioned in rats using a taste-immune associative learning paradigm (Hadamitzky, Lückemann, Pacheco-López, & Schedlowski, 2020). In this scenario, repeated administration of an active drug together with a specific pleasant taste in a so-called acquisition phase results in reduced consumption of this taste, known as conditioned taste avoidance (CTA). When the animal is re-exposed to the taste at a later time but without administration of the drug (retrieval phase), the CTA is accompanied by changes in the peripheral immune response mimicking the initial immunopharmacological action of the drug, called a conditioned response (CR). In this case, the taste represents the conditioned stimulus (CS), whereas the drug is the unconditioned stimulus (US). This paradigm of taste-immune associative learning demonstrates the impressive abilities of the brain to detect and store information about an organism's immunological status and to retrieve this information, thereby modulating immunological functions via endogenous pathways.
Our understanding of the immunobiological and neurobiological mechanisms in the periphery and brain mediating and orchestrating such learned immunomodulatory effects is still limited. Reliable animal models can provide the opportunity not only to study the impact of conditioned effects on the immune response but also to characterize the role of specific central nervous system (CNS) structures and pathways involved in brain-immune communication.
Here, we present an established model of taste-immune associative learning in rats, which has been applied in distinct approaches to study the effects on conditioned immunopharmacological functions (Exton, von Hörsten, Schult, et al., 1998; Exton, von Hörsten, Vöge, et al., 1998; Hörbelt, Martínez-Gómez, Hadamitzky, Schedlowski, & Lückemann, 2019; Lueckemann et al., 2016), the extinction of observed outcomes (Hadamitzky, Bösche, Engler, Schedlowski, & Engler, 2015), and use of low-dose medication to block extinction (Hadamitzky, Bösche, et al., 2016), as well as to study the role of specific CNS regions and neuro-immune pathways that modulate peripheral immune responses (Hadamitzky, Bösche, et al., 2016; Hadamitzky, Orlowski, et al., 2016). Moreover, this approach has successfully been applied in animal models of organ transplantation (Exton, von Hörsten, Schult, et al., 1998; Hadamitzky, Bösche, et al., 2016), autoimmune diseases (Lückemann, Stangl, Straub, Schedlowski, & Hadamitzky, 2020), and cancer. The presented protocols focus on the basic taste-immune associative learning paradigm with CsA and saccharin, where conditioned peripheral immunosuppression is determined in ex vivo cultured splenocytes.
The approach is as follows. After an initial training phase, where animals learn to drink water for 15 min at 9 a.m. and again at 5 p.m. each day, a sweet-tasting saccharin solution (the CS) is presented together with a CsA injection (the US) in three association trials during acquisition (Fig. 1A). This results in a CTA, which persists for several days, even when solely the CS is re-presented without CsA in the following retrieval phase (Fig. 1B). Pharmacological effects of CsA, as well as conditioned immunosuppressive effects, are examined in peripheral immune organs such as the spleen (Fig. 1C). Ex vivo cultivation and stimulation of splenocytes with anti-CD3 induce the production of T cell–associated cytokines (IL-2, IFN-γ), which is diminished in rats treated with CsA (“US-G.”), representing the pharmacological effect, as well as in conditioned animals (“CS-G.”), indicative of taste-immune associative learning (Fig. 1D).
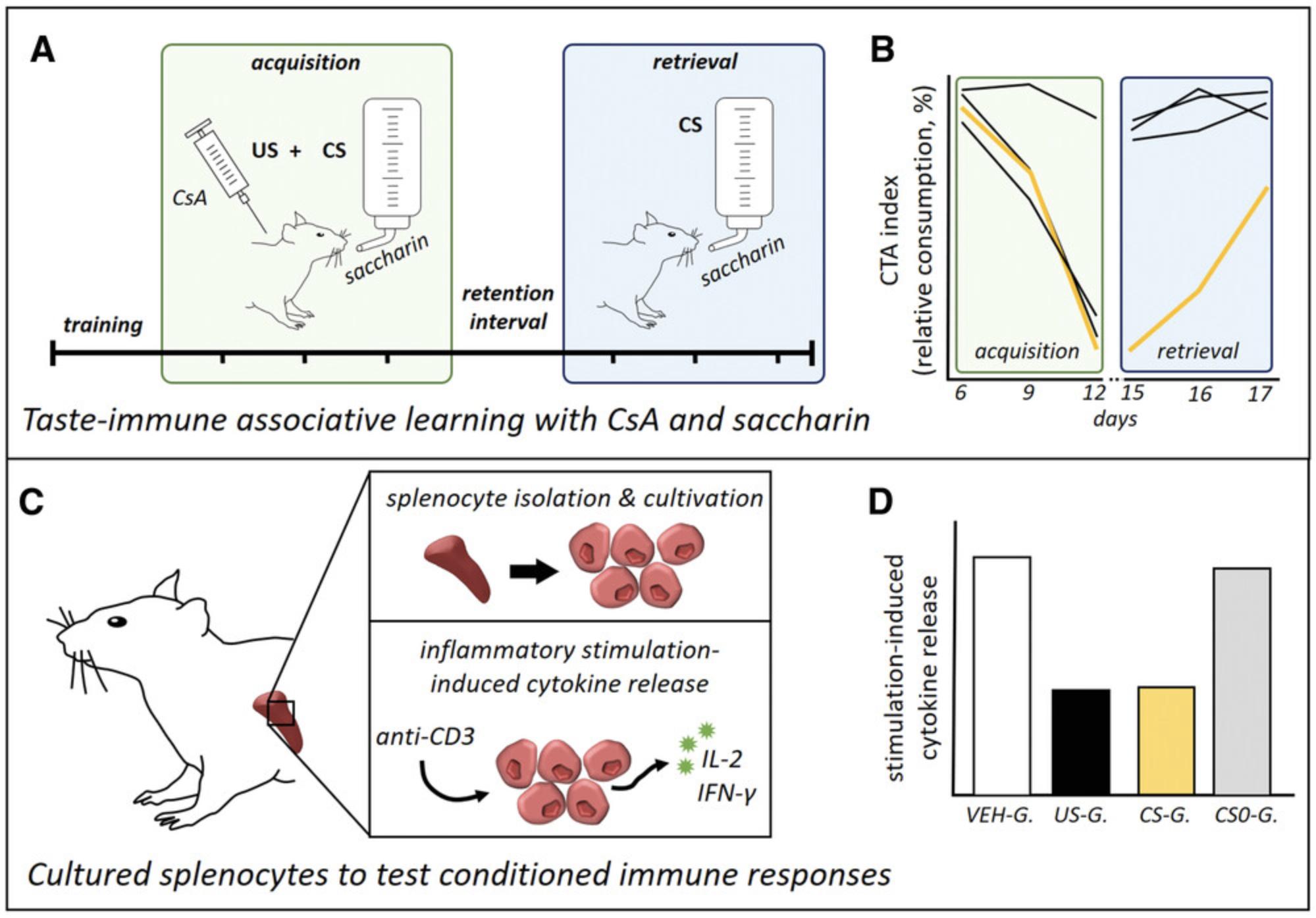
In Basic Protocol 1, we describe the principal steps to induce a state of conditioned immunosuppression, applying the taste-immune associative learning paradigm with CsA as the US and saccharin as the CS (Fig. 1A and 1B). In Basic Protocol 2, we introduce the ex vivo stimulation of cultured splenocytes with anti-CD3 and the detection of stimulation-induced expression and release of IL-2 and IFN-γ as important readout parameters to examine immunopharmacological and conditioned effects (Fig. 1C, 1D).
The reproducible protocol of conditioned immunosuppression in rats (Basic Protocol 1) constitutes a reliable tool to gain further insights into the underlying mechanisms and pathways of brain-to-immune communication. Given that its applicability has also been shown for humans (Goebel et al., 2002), conditioned immunopharmacological effects may be implemented as controlled drug-dose reduction strategies supporting the efficacy of medical treatments for patients’ benefit (Hadamitzky & Schedlowski, 2022).
STRATEGIC PLANNING
To analyze the success of a taste-immune associative learning paradigm, predetermination of specific treatment groups is an essential step, and adjustments might be required if the protocol is used with different drugs or disease models. Basic Protocol 1 comprises four treatment groups, namely “CS-G.”, “US-G.”, “CS0-G.”, and “VEH-G.” (see Fig. 2). The conditioned group (“CS-G.”) receives the CS (saccharin) in the acquisition phase, combined with injection of the drug CsA (US). In the retrieval phase, animals are re-exposed to the CS without the US to detect conditioned effects. Moreover, one group, “US-G.”, is treated with CsA in the acquisition phase as well as in the retrieval phase to analyze pharmacological effects. An additional group, “CS0-G.”, undergoes acquisition with the US/CS pairing but without a subsequent evocation of the CR. Therefore, these animals receive water instead of saccharin in the retrieval phase. This group is essential to distinguish between (putative) residual drug effects in the retrieval phase and true CS-evoked conditioned effects. Finally, a vehicle group (“VEH-G.”) should be implemented as an untreated control. Animals in this group are not exposed to the US, but rather only to the CS, during acquisition and retrieval.
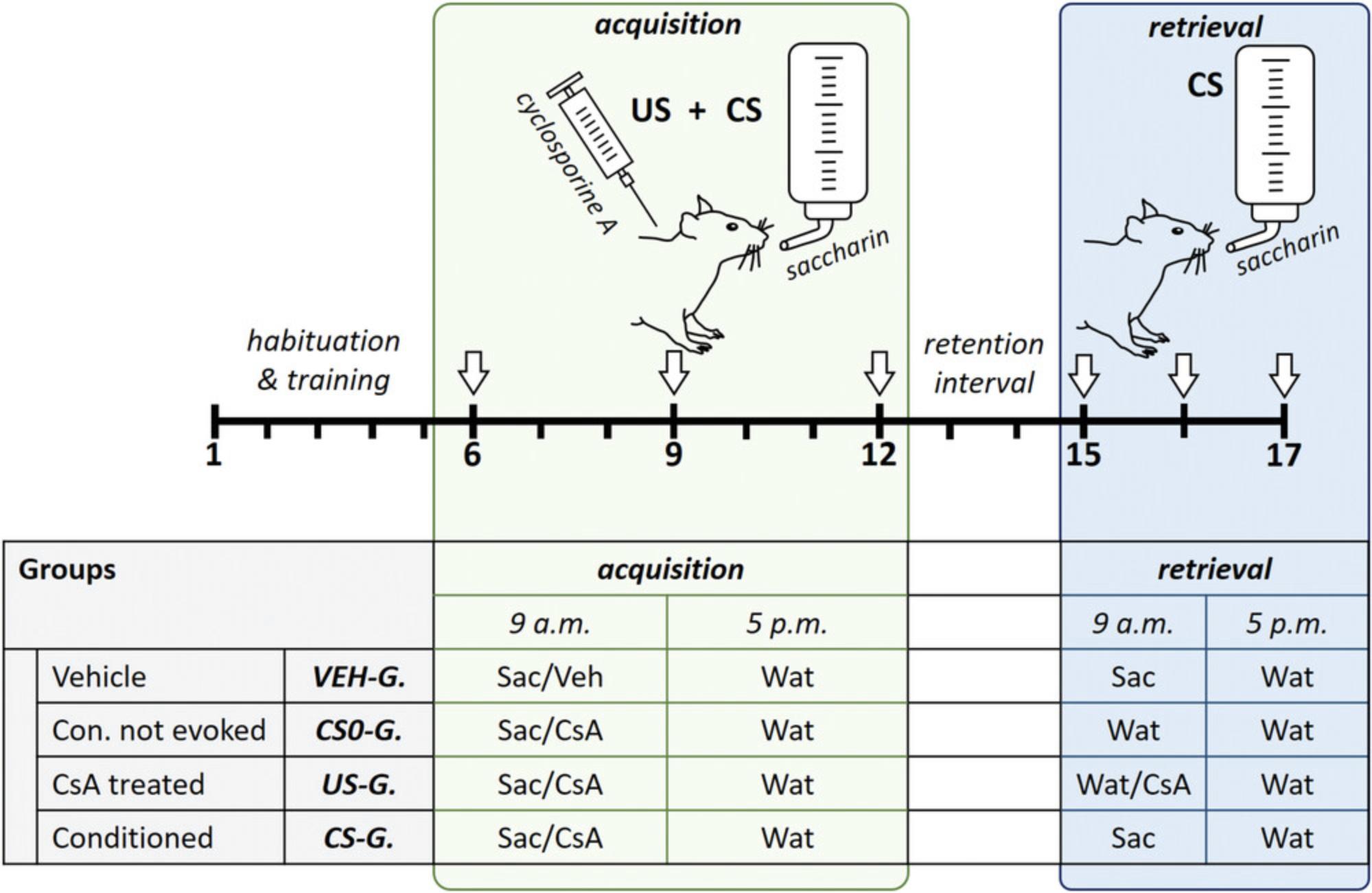
Per experiment, the total number of animals should not exceed 16 rats (four groups with four animals each). For statistical analyses, the experiment should be repeated at least three times to achieve a number of 12 animals per group.
Basic Protocol 1: TASTE-IMMUNE ASSOCIATIVE LEARNING WITH CYCLOSPORINE A
This basic protocol comprises the fundamental steps of the taste-immune associative learning paradigm in rats to obtain a conditioned immune response mimicking the effects of the immunosuppressive drug CsA. Therefore, rats learn to associate the taste of saccharin with the pharmacological effect of CsA during the acquisition phase. Subsequently, the CR can be observed during the retrieval phase, when saccharin is re-presented without CsA (Fig. 2).
The strict drinking regimen twice a day is required to ensure adequate consumption of saccharin. For habituation of rats to the restrictive drinking procedure twice daily (9 a.m. and 5 p.m.), an initial training phase is needed (days 1 to 5). On days 6, 9, and 12 (acquisition), the rats are injected intraperitoneally with a therapeutic dose of CsA (20 mg/kg) after the drinking session in the morning (9 a.m.). The repetition every 3 days results in a conditioned avoidance of the taste (CTA), with reduced consumption of the saccharin, indicative of a strong association of the taste (CS) with the drug (US). The intermediate days between injections, as well as between acquisition and retrieval, guarantee adequate excretion of CsA to avoid residual pharmacological effects. Re-exposure to saccharin at subsequent retrieval (days 15 to 17) results not only in CTA but also, more importantly, in conditioned immunosuppression mimicking the initial pharmacological CsA effects. Conditioned immunosuppressive effects can be detected in ex vivo cultured splenocytes, which we describe in Basic Protocol 2.To interpret results adequately, at least four groups should be implemented (for details, see Strategic Planning): “VEH-G.”, “CS0-G.”, “US-G.”, and “CS-G.”.
NOTE : The following procedures were approved by the Institutional Animal Care and Use Committee (IACUC) of the Medical Faculty, University Duisburg-Essen. Users must also acquire approval from an equivalent committee and obtain all relevant permissions before performing the following steps on any animal.
Materials
-
16 rats (Dark Agouti DA/HanJ, male, ∼ 250 g; Janvier)
-
0.2% (w/v) saccharin solution (see recipe)
-
100 mg/ml CsA stock solution (see recipe)
-
Sterile saline (0.9% NaCl; Fresenius Kabi)
-
Hank's balanced salt solution (HBSS; Gibco Life Technologies, cat. no. 14170-088), 4°C
-
Oxygen (10 l; 200 bar; Oxyparat C. Allihn GmbH)
-
Isoflurane (Piramal Critical Care B.V., cat. no. 2800026)
-
Single housing cages (16 total)
-
Drinking bottles (250 ml; 16 bottles for water, 16 bottles for saccharin solution)
-
Scale (Mettler Toledo, cat. no. PB3001-SFACT)
-
1-ml syringes (F-Injekt, B. Braun, cat. no. 9166017V)
-
26G cannula (26G × 1″, B. Braun, cat. no. 4657683)
-
Anesthesia unit (Compact 150 Rodent Anesthesia Machine, Midmark, model no. 91800121, serial no. V2262460)
-
Guillotine
-
Surgical equipment for spleen harvesting (sharp scissors and forceps)
-
50-ml centrifuge tubes (Greiner Bio-One, cat. no. 227261)
Prior to experiment
1.Plan four groups, with four animals per group: “VEH-G.”, “CS0-G.”; “US-G.”, and “CS-G.”.
2.Place 16 rats into single housing cages 7 days prior to start of the experiment and label cages with the respective numbers 1 to 16.Provide ad libitum access to food and tap water until water restriction regimen starts at day 1 (training, steps 5 to 8).
3.Label water and saccharin bottles for each animal (32 drinking bottles total) according to their respective experimental ID (water: W1 to W16; saccharin: S1 to S16). Fill up all bottles with corresponding solution (i.e., tap water or 0.2% saccharin solution) and weigh them prior to conditioning procedure (baseline value).
4.Remove water bottles in the evening (5 p.m.) prior to the start of the training phase.
Training: Days 1 to 5
5.Start training phase by placing the labeled water bottles (W1 to W16) in the respective animals’ cages at 9 a.m. (morning session) and at 5 p.m. (evening session) and allow rats to drink for 15 min.
6.Remove and weigh bottles immediately after each drinking session.
7.Monitor rats’ drinking behavior per drinking session over the complete experiment by calculating the volume of consumed water as the difference in bottle weights between drinking sessions.
8.Refill bottles when necessary. Weigh refilled bottles to generate a new baseline value.
Acquisition: Days 6 to 12
9.Prepare injections for all treatment groups, with CsA for 12 animals (“CS0-G.”, “US-G.”, and “CS-G.”) and sterile saline (0.9% NaCl) for 4 animals (“VEH-G.”). Dilute 50 µl of 100 mg/ml CsA stock solution in 950 µl sterile saline to reach a final concentration of 5 mg/ml, corresponding to 20 mg/kg for one rat of 250 g.
10.Place prepared saccharin bottles (S1 to S16) into the cages at 9 a.m. in the morning session of day 6 and allow rats to drink for 15 min. Remove saccharin bottles immediately after the drinking session.
11.Taking one rat after the other out of their home cages immediately after the drinking session, inject CsA (see step 9) or sterile saline intraperitoneally using a 1-ml syringe with a 26G cannula (for details about parenteral injections in laboratory rodents, see Current Protocols article: Donovan & Brown, 1995), as follows:
- “CS0-G.”: CsA (20 mg/kg)
- “US-G.”: CsA (20 mg/kg)
- “CS-G.”: CsA (20 mg/kg)
- “VEH-G.”: Sterile saline (0.9% NaCl)
12.Place rats back into their respective home cages after each injection.
13.Weigh all bottles and note liquid consumption (see step 7).
14.Provide water in evening session (5 p.m.) for 15 min. Immediately after the session, remove and weigh all bottles and note consumed water volume (see step 7).
15.Repeat this CS-US pairing (steps 9 to 14) on days 9 and 12.
16.Provide water in morning and evening sessions of intermediate days (days 7, 8, 10, 11) for 15 min and note consumed water volume (see step 7).
Retention interval: Days 13 and 14
17.Place water bottles into the cages in the morning and evening sessions during the retention interval (days 13 and 14). Allow rats to drink for 15 min. Remove and weigh all bottles after each drinking session and note consumed water volume (see step 7).
Retrieval: Days 15 to 17
18.Prepare injections of CsA to a final volume of 1 ml (see step 9) for the pharmacological control group “US-G.” (four animals).
19.Place saccharin bottles into the cages of the rats of the “CS-G.” and “VEH-G.” groups in the morning session (9 a.m.) on day 15 (Fig. 2). Provide water bottles to the rats of the “CS0-G.” and “US-G.” groups at the same time. Allow rats to drink for 15 min.
20.Inject “US-G.” animals with CsA (20 mg/kg) intraperitoneally immediately after the drinking session as in step 11.
21.Remove and weigh all bottles after each drinking session and note liquid consumption (see step 7).
22.Repeat this procedure (steps 18 to 21) on days 16 and 17.
Tissue harvesting: Day 17
23.Prepare anesthesia unit, guillotine, and surgical equipment for spleen harvesting. Transfer 10 ml cold HBSS to 50-ml centrifuge tubes, with one tube per animal (16 tubes, labeled 1 to 16). Keep tubes on ice to preserve the collected spleens for subsequent splenocyte isolation (see Basic Protocol 2).
24.Terminate experiment 1 hr after the last CS re-exposure (day 17, 10 a.m.) and proceed with one animal after the other as follows:
-
Expose rat to oxygen and then anesthetize rat with isoflurane (for details, see Current Protocols article: Davis,2008).
-
Euthanize rat by decapitation with the guillotine (for details, see Current Protocols article: Donovan & Brown,2006).
-
Open abdominal cavity lengthwise with sharp scissors.
-
Isolate spleen and, using forceps, transfer it into one of the 50-ml centrifuge tubes filled with 10 ml cold HBSS (see step 23).
-
Store spleens in HBSS on ice until all 16 spleens have been isolated and proceed with splenocyte isolation and stimulation for immunological analyses (see Basic Protocol2).
Evaluation of the conditioned taste avoidance (CTA)
25.Assemble generated data on liquid consumption (water and saccharin) over the time course of the complete experiment.
26.Calculate mean amount of consumed water per drinking session from all water drinking sessions and set this mean water consumption as 100%.
27.Evaluate relative liquid consumption in the morning sessions of the acquisition days (days 6, 9, and 12) and the retrieval days (days 15, 16, and 17) independently for each animal and each day by comparison to the mean water consumption (100%).
28.Illustrate obtained data graphically as the CTA index (see Understanding Results).
Basic Protocol 2: SPLENOCYTE ISOLATION AND CULTIVATION TO STUDY STIMULATION-INDUCED CYTOKINE PRODUCTION
The pharmacological mechanism of CsA is based on its inhibitory effect on leukocyte proliferation via blockade of calcineurin, resulting in suppressed production of T cell–associated cytokines, such as IL-2 and IFN-γ (see details in Background Information). Thus, pharmacological CsA effects, as well as conditioned immunosuppressive effects mimicking CsA, can be investigated in tissues of the lymphatic system, such as the spleen. Therefore, isolated splenocytes from rats subjected to the taste-immune associative learning paradigm described in Basic Protocol 1 are stimulated with anti-CD3 to induce T-cell proliferation and upregulation of the expression and release of IL-2 and IFN-γ, which are diminished by CsA.
The following protocol explains the process of splenocyte isolation and cultivation, stimulation with anti-CD3, and detection of stimulation-induced changes in cytokine mRNA levels and release by means of qPCR and ELISA, respectively.
This protocol is established for rat splenocytes but can also be adapted to cells from other lymphatic organs, such as lymph nodes.
NOTE : All steps in this protocol require sterile technique. Additionally, use cooled media (HBSS and splenocyte cultivation medium) throughout.
NOTE : Experiments involving PCR require extremely careful technique to prevent contamination.
Materials
-
HBSS (Gibco Life Technologies, cat. no. 14170-088), 4°C
-
Isolated spleens (see Basic Protocol 1, step 24e)
-
PharM Lyse (BioLegend, cat. no. 420301), diluted 1:10 in sterile water
-
Splenocyte cultivation medium (see recipe), 4°C
-
1 mg/ml anti-CD3, mouse anti-rat monoclonal antibody (mAb; clone G4.18, BD Pharmingen, cat. no. 554829)
-
IL-2 and IFN-γ ELISA kits (e.g., Rat IL-2 Quantikine ELISA Kit, R&D Systems, cat. no. R2000, and Rat IFN-γ Legend Max ELISA Kit, BioLegend, cat. no. 439007)
-
RNeasy Mini Kit (Qiagen, cat. no. 74106)
-
MultiScribe reverse transcriptase (Thermo Fisher Scientific, cat. no. 4311235)
-
Random hexamer primers
-
Takyon mastermix (Takyon No ROX Probe Mastermix dTTP, Eurogentec, cat. no. UF-NPMT-C0701)
-
Gene expression assays (Thermo Fisher Scientific):
- IL-2 (Rn00587673_m1)
- IFN-γ (Rn00594078_m1)
- 18s (#4319413E)
-
Laminar flow hood (HERA Safe, Heraeus, type HS18, serial no. 99115953)
-
50-ml centrifuge tubes (Greiner Bio-One, cat. no. 227261)
-
Petri dishes (Greiner Bio-One, cat. no. 628161; 16 total)
-
20-ml syringe plungers (BD Discardit, cat. no. 300296; 16 total)
-
Refrigerated centrifuge, 4°C, with plate adaptor
-
Sterile 70-µm nylon cell strainers (EASYstrainer, Greiner Bio-One, cat. no. 542070; 16 total)
-
Hematology analyzer (Vet ABC, HORIBA ABX, model no. SCIL VET, serial no. 804AB12278) or hemocytometer
-
96-well flat-bottom microtiter plate (MicroApm, Applied Biosystems® by Life Technologies™ Corporation, cat. no. 4346906)
-
Humidified 37°C, 5% CO2 incubator (Thermo Fisher Scientific, Heracell 240)
-
1.5-ml reaction tubes (Greiner Bio-One, cat. no. 616 201)
-
Plate reader (Plate Reader FLUOstar Optima, BMG Labtech GmbH, cat. no. S/N 413-3219)
-
PCR machine (7500 FAST Real-Time PCR System, Applied Biosystems, cat. no. 4387777)
Splenocyte isolation and cultivation
1.Prepare a sterile work area under a laminar flow hood.
2.Label 50-ml centrifuge tubes as 1 to 16, with each number used twice (for a total of 32 labeled tubes).
3.Add 10 ml cold HBSS to a petri dish.
4.Transfer one isolated spleen (from Basic Protocol 1, step 24e) into the petri dish filled with HBSS.
5.Pestle whole spleen with a 20-ml syringe plunger in the petri dish until most of the tissue is disrupted.
6.Transfer cell suspension to the corresponding 50-ml centrifuge tube (numbered 1 to 16, from step 2) and add 25 ml cold HBSS.
7.Store cell suspension on ice and proceed with next spleen until all spleens are disrupted.
8.Centrifuge all samples for 8 min at 350 × g , 4°C.
9.Discard supernatants and resuspend cell pellets in 3 ml PharM Lyse (diluted 1:10 in sterile water). Incubate for 5 min at room temperature to lyse all red blood cells.
10.Add 25 ml cold splenocyte cultivation medium to each sample.
11.Centrifuge cells for 8 min at 350 × g , 4°C.
12.Discard supernatants and resuspend cell pellets in 5 ml cold splenocyte cultivation medium.
13.Place sterile 70-µm nylon cell strainers on fresh 50-ml centrifuge tubes (labeled 1 to 16 in step 2).
14.Filter cell suspension through the cell strainer on the proper tube and add 25 ml cold splenocyte cultivation medium.
15.Centrifuge cells (8 min at 350 × g , 4°C), discard supernatants, and resuspend cell pellets in 5 ml cold splenocyte cultivation medium.
16.Determine number of cells per milliliter using a hematology analyzer or hemocytometer and adjust cell suspension to a final concentration of 5 × 106 cells/ml. Proceed to determination of stimulation-induced cytokine release (steps 17 to 23) or to determination of stimulus-induced changes in cytokine mRNA expression (steps 24 to 29).
Determination of stimulation-induced cytokine release
17.Transfer 100 µl of the adjusted cell suspension of each sample into each of three wells of a 96-well flat-bottom microtiter plate (see Fig. 3 for an exemplary 96-well plate design).
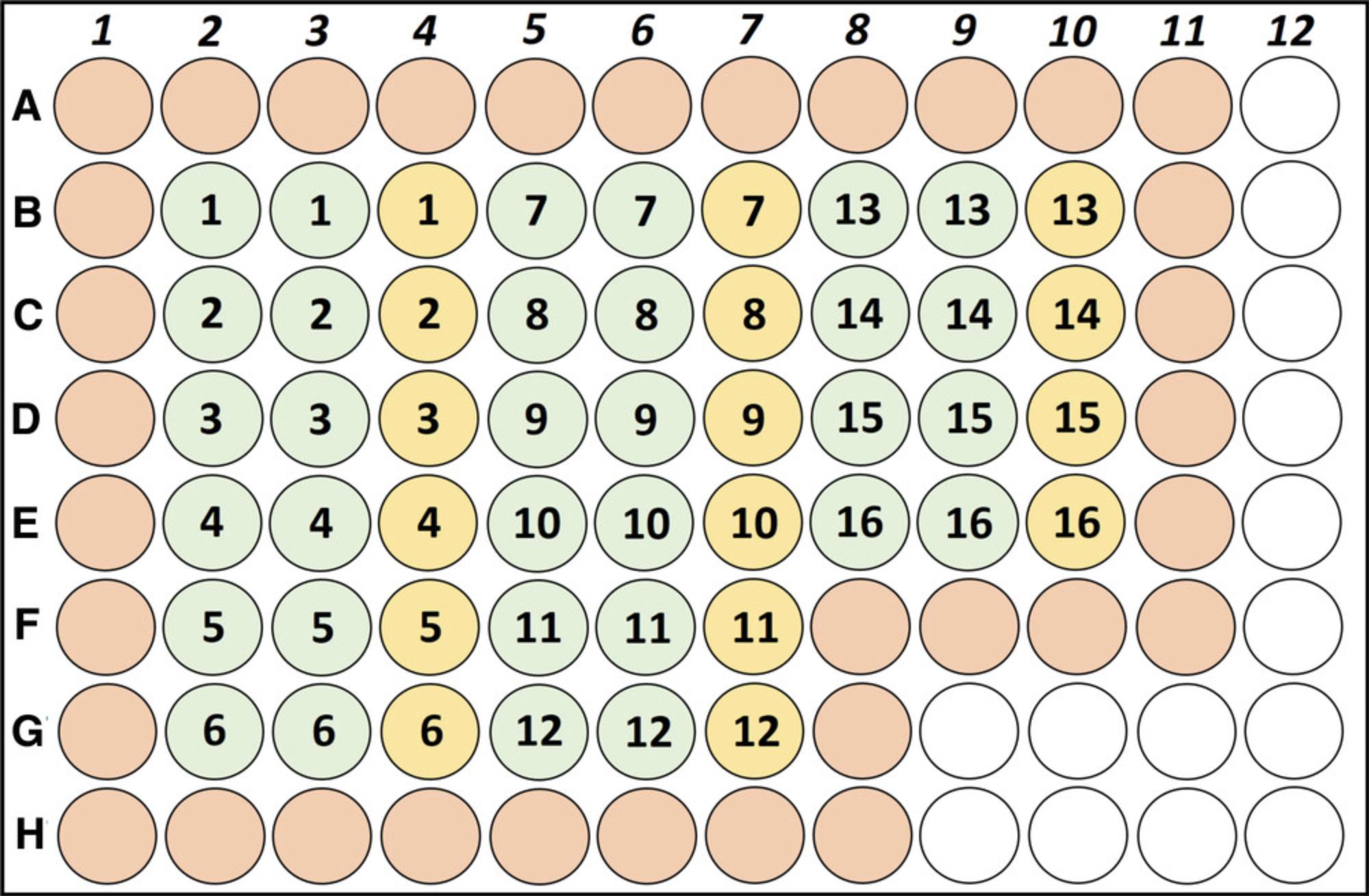
18.Prepare cold stimulation solution by mixing 20 ml cold splenocyte cultivation medium with 40 µl of 1 mg/ml anti-CD3 for a final concentration of 2 µg/ml anti-CD3.
19.Add 100 µl of the prepared cold stimulation solution (2 µg/ml anti-CD3) or cold splenocyte cultivation medium (for the unstimulated control) to the respective wells to start stimulation. Use two wells for stimulation with anti-CD3 in duplicate and the third as the unstimulated control (see Fig. 3). Add 200 µl cold cultivation medium to wells around the samples to avoid dehydration of the sample wells.
20.Place plate in a humidified 37°C, 5% CO2 incubator for 48 hr.
21.Centrifuge plate for 4 min at 450 × g , 4°C.
22.Collect 150 µl of each supernatant into 1.5-ml reaction tubes and store at −80°C until further processing.
23.When ready, use commercially available IL-2 and IFN-γ ELISA kits and a plate reader to determine amount of released IL-2 and IFN-γ, adhering closely to the manufacturer's protocol.
Determination of stimulus-induced changes in mRNA expression
24.Transfer 500 µl of the adjusted cell suspension (5 × 106 cells/ml; see step 16) into a 1.5-ml reaction tube.
25.Add 500 µl stimulation solution (2 µg/ml anti-CD3; see step 18) to reaction tube with the 500 µl of cell suspension.
26.Place tubes in a humidified 37°C, 5% CO2 incubator for 4 hr.
27.Extract mRNA with the RNeasy Mini Kit according to the manufacturer's protocol.
28.Synthesize complementary DNA (cDNA) by reverse transcription using MultiScribe reverse transcriptase and random hexamer primers following the manufacturer's instructions.
29.Determine relative mRNA expression of the target genes by qPCR using a PCR machine and Takyon mastermix for the IL-2 (Rn00587673_m1) and IFN-γ (Rn00594078_m1) gene expression assays. Use 18s (#4319413E) as a reference gene for relative quantification.
REAGENTS AND SOLUTIONS
Cyclosporine A (CsA) stock solution, 100 mg/ml
Prepare a stock solution of CsA (100 mg/kg) by dissolving 100 mg CsA powder (LC Laboratories, cat. no. C-6000, lot no. ATC-109) in 100 µl 95% (v/v) ethanol (EtOH; for injections; B. Braun Melsungen AG, cat. no. 03837980) in a glass vial on a magnetic stirrer for 15 min. Add 900 µl Miglyol 812 (Caesar & Loretz GmbH, cat. no. 15379609) slowly to CsA/EtOH solution and keep stirring until remaining CsA is completely dissolved. Store ≤2 days at 4°C, protected from light. Before use, mix 1 ml stock solution with 19 ml of 0.9% (w/v) NaCl (for injections; Fresenius Kabi) with a syringe and an 18G cannula (18G × 1.5″, BD, cat. no. 303129) in a centrifuge tube to obtain a homogenous emulsion with a concentration of 5 mg/ml for intraperitoneal injection (see Basic Protocol 1, step 9). Prepare 1-ml syringes with the individual volume according to the rat's weight: weight × 4 = injection volume (e.g., for a 250-g rat, the injection volume is 1 ml).
Saccharin solution, 0.2%
Dissolve 10 g sodium saccharin (Sigma-Aldrich, cat. no. 109185-500g) in 1 L tap water by gently shaking in a canister before adding an additional 4 L tap water. Prepare immediately before use. Fill labeled bottles (S1 to S16) just prior to the experiment (see Basic Protocol 1, step 3). Store filled bottles at room temperature (21°C) throughout the whole experiment.
Splenocyte cultivation medium
Discard 50 ml from the original bottle of RPMI 1640 medium with GlutaMAX (Gibco, Thermo Fisher Scientific, cat. no. 72400-021). Add 50 ml fetal bovine serum (FBS; Gibco, Thermo Fisher Scientific, cat. no. 26140079) and 500 µl gentamicin (Gibco, Thermo Fisher Scientific, cat. no. 15750-037). Store ≤ 2 weeks at 4°C.
COMMENTARY
Background Information
The principle of taste-immune associative learning
The principle of taste-immune associative learning goes back to the initial observations of Ivan Petrovič Pavlov. In the 1900s, Pavlov found out that physiological, “unconditioned” responses (URs), such as a dog's salivation in response to food (the US), can be paired with a neutral stimulus, such as a metronome or bell (the CS), resulting in a CR that mimics the original physiological response (Pavlov, 1928). About 20 years later, Metalnikov & Chorine provided the first evidence of a “conditioned reflex in the immune response” in guinea pigs (Metalnikov & Chorine, 1926). In their experiments, repeated intraperitoneal injection of tapioca plant extract as the US paired with a sensory stimulus (scratch or heat) as the CS in the acquisition phase resulted in an altered peritoneal leukocyte profile when the CS was re-presented at a later point without the US (retrieval). The principle of conditioned immune responses was not followed up until 1975, when Ader & Cohen introduced the conditioned taste aversion or avoidance (CTA) paradigm to induce conditioned immunosuppression (Ader & Cohen, 1975). Presentation of saccharin (CS) prior to an injection with the immunosuppressant and cytostatic drug cyclophosphamide (US) induced attenuated consumption of this taste, accompanied by a suppressed immune response upon injection of antigenic sheep erythrocytes. Since these early observations, several studies confirmed conditioned immunosuppressive effects due to taste-immune associative learning on acute-phase reactions (Exton, Bull, King, & Husband, 1995; Janz et al., 1996), immune cell proliferation (Lysle, Cunnick, Fowler, & Rabin, 1988) and activation (Ghanta, Hiramoto, Solvason, & Spector, 1985), and antibody production (Cohen, Ader, Green, & Bovbjerg, 1979), not only in laboratory rodents but also in humans (Goebel et al., 2002), thereby setting the stage for the research field of psychoneuroimmunology.
Pharmacological effects of cyclosporine A
CsA is a small-molecule drug that is frequently used to suppress a patient's immune response (Kapturczak, Meier-Kriesche, & Kaplan, 2004). Such a severe intervention might be inevitable for distinct medical reasons, including autoimmune diseases (e.g., rheumatoid arthritis, psoriasis, Crohn's disease) or allotransplantations (e.g., kidney, liver, heart, bone marrow) (White, 1982). However, use of CsA is also associated with massive side effects when long-term treatment is required (Graham, 1994; White, 1982). These include an enhanced susceptibility to infections (e.g., bacterial, viral, fungal), as well as hypertension and nephrotoxicity.
CsA exerts its immunosuppressive effects via inhibition of calcineurin in T cells (Fig. 4) (Steinbach et al., 2007). T-cell proliferation is mediated by the Ca2+-activated serine/threonine phosphatase calcineurin (CaN), which dephosphorylates the transcription factor nuclear factor of activated T cells (NFAT). In its activated form, NFAT can translocate into the nucleus to induce the transcription of Th1-associated cytokines, especially IL-2 and IFN-γ, which further promote inflammatory processes (O'Shea, Ma, & Lipsky, 2002). Therefore, inhibition of CaN by CsA results in diminished expression and release of IL-2 and IFN-γ by T cells and thereby suppresses the immune response.
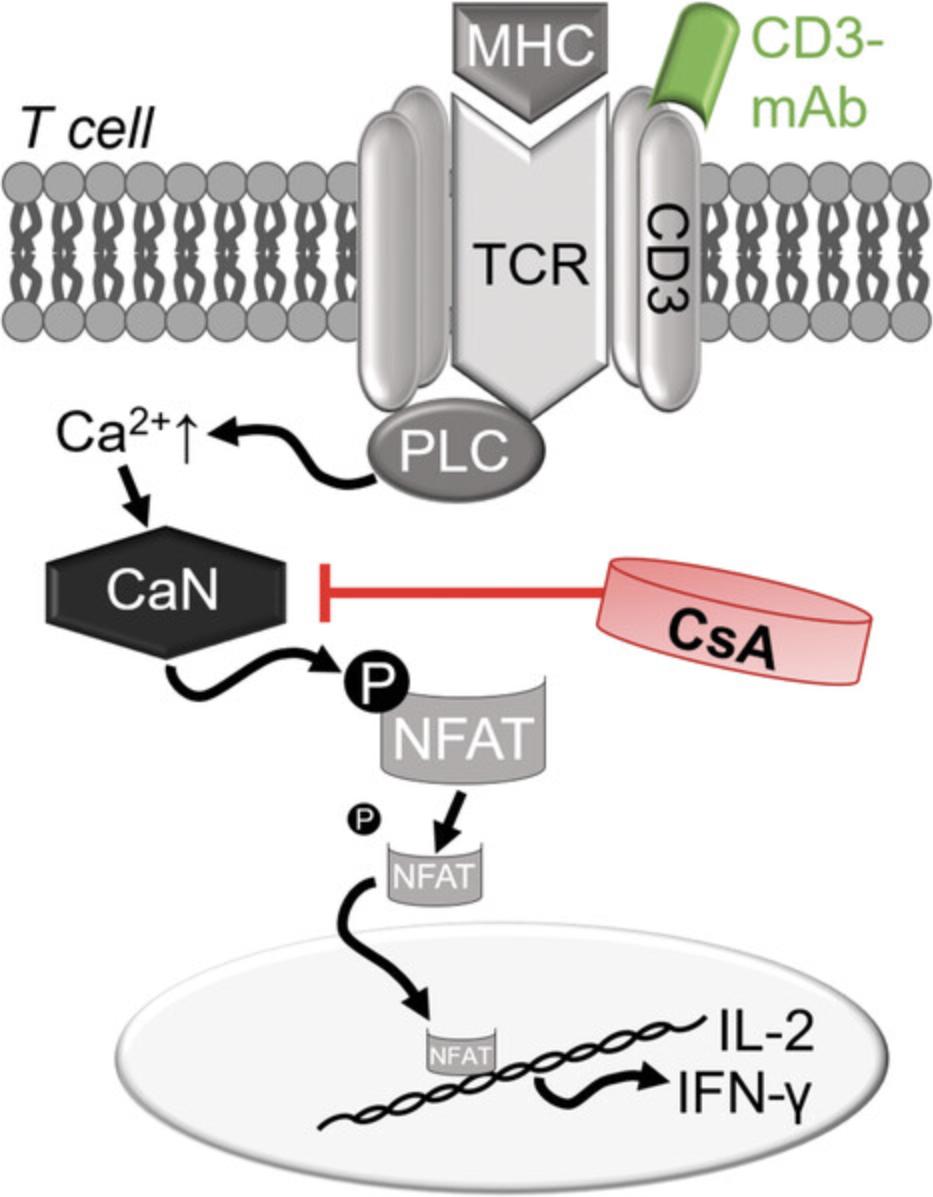
Pharmacological effects of CsA can easily be detected in secondary lymphoid organs containing a large number of lymphocytes, such as the spleen or lymph nodes (Exton, von Hörsten, Vöge, et al., 1998). Stimulation of cultured splenocytes with an anti-CD3 mAb initiates the cascade of T-cell proliferation and represents a useful ex vivo tool to study pharmacological effects of CsA, such as attenuated CD3-induced release of IL-2 or IFN-γ (Exton, von Hörsten, Vöge, et al., 1998; Hadamitzky, Bösche, et al., 2016; Hörbelt et al., 2019; Lueckemann et al., 2016; Pacheco-López et al., 2009).
Adaptations and applications of the protocols
In Basic Protocol 1, the taste of saccharin is used as a sensory stimulus (CS), and the immunosuppressive drug CsA is applied as a US. However, it is important to mention that several variables in this protocol can be adapted depending on the scientific question. Indeed, it is possible to implement other sensations as the CS, such as olfactory, tactile, auditory, or visual stimuli. However, taste seems to be particularly suitable for associative learning paradigms, which may be due to the evolutionary advantage of avoiding ingestion of potentially malignant substances linked to a specific taste (Hadamitzky et al., 2020; Pacheco-López et al., 2013). This strong association results in improved reproducibility across experiments and even allows application in disease models, such as collagen type II arthritis (Lückemann et al., 2020) or heterotopic heart transplantation (Exton, von Hörsten, Schult, et al., 1998; Hadamitzky, Bösche, et al., 2016). This applicability represents an essential step to characterize putative beneficial effects of conditioned immunosuppression in the context of specific diseases and to further translate study results into clinical research. Moreover, we have shown that the paradigm of taste-immune associative learning is not restricted to CsA as the US, but rather can be translated to other immunosuppressants, such as rapamycin (Lückemann, Unteroberdörster, Martinez Gomez, Schedlowski, & Hadamitzky, 2019).
Remaining challenges and outlook
In summary, experimental data demonstrate that conditioned pharmacological effects, obtained by means of associative learning, have been successfully implemented as controlled drug-dose reduction strategies supporting the efficacy of medical treatments. However, in order to systematically use such paradigms as a supportive treatment option to optimize pharmacological treatment effects for patients’ benefit, it is important to understand the central and peripheral mechanisms of how learned immune responses can be protected from being extinguished (Hadamitzky, Engler, & Schedlowski, 2013). It has already been demonstrated that sub-effective doses of the US administered in close temporal proximity to the CS at retrieval prevent the CRs from being extinguished (Hadamitzky, Bösche, et al., 2016). Even though still unknown, it is suggested that the presentation of such reminder cues partially replicates an encoding experience, thereby enabling the conditioned immune memories to be strengthened (Sinclair & Barense, 2019).
Despite these advances, further research in basic and clinical science is urgently required for adequate and reliable application of conditioned immunosuppression for patients in need of sufficient treatment. Moreover, it is important to investigate whether this phenomenon generalizes across various drug categories, such as analgesics or antidepressants (Hadamitzky & Schedlowski, 2022).
Critical Parameters
Basic Protocol 1 was established in male Dark Agouti rats with an average age of ∼63 days (230 to 250 g). Given that immune function changes with age, make sure not to use “old” animals (e.g., >365 days).
For the success of this procedure, it is critical to monitor drinking behavior every day, including during training. If the animals do not learn to drink twice a day, in the morning and evening sessions, for 15 min, expand the training phase to >5 days until water intake is stable for a consistent baseline.
During the association trials (acquisition), injections of CsA should be administered no later than 30 min following saccharin presentation.
Temperature, humidity, and the light/dark cycle in the animals’ vivarium need to be controlled and kept constant during the whole experiment. Keep the number of staff members as low as possible (maximum of four) and always run studies with sex-counterbalanced experimenters. Include no more than 16 animals at a time. The space in the 96-well plate used in the subsequent immunological analyses limits the sample size, given that running duplicates and unstimulated controls per animal is required for proper analyses (Basic Protocol 2 and Fig. 3).
Taste-immune associative learning studies with CsA have shown that CTA extinction most commonly occurs after 4 to 7 CS re-exposures (Exton, von Hörsten, Vöge, et al., 1998). However, observations also indicate that it is not a significant CTA, but rather re-exposure to the taste itself, that is essential for conditioned immunosuppression (Ader & Cohen, 1982; Bovbjerg, Ader, & Cohen, 1984; Bovbjerg, Kim, Siskind, & Weksler, 1987; Exton, von Hörsten, Schult, et al., 1998; Exton et al., 2002; Lueckemann et al., 2016).
It is also critical to sacrifice animals no earlier than 60 min following the last retrieval trial (e.g., day 17 in Basic Protocol 1), given that central as well as peripheral activity and distribution have been confirmed at that time point (Hadamitzky, Bösche, et al., 2016; Pacheco-López et al., 2013; von Hörsten et al., 1998).
When the spleens are harvested for the subsequent immunological analyses (Basic Protocol 2), make sure that you sterilize the forceps with ethanol between animals. Moreover, minimize the amount of visceral fat on this lymphatic organ. Adequate mechanical disruption of the whole organ, indicated by murky HBSS solution, is essential to gain enough cells for immunological analyses.
Troubleshooting
Poor drinking behavior
During the water restriction period or the conditioning phase, some rats may display poor drinking performance. In most cases, this is attributable to a blocked buckler of the fluid bottle. Thus, make sure that fluid bottles are fully functional.
Poor taste avoidance
Conditioning may not be effective in drug-injected rats (i.e., some treated animals do not avoid consuming the saccharin). To avoid poor performance, make sure that the saccharin bottles are not stored close to the animals in the vivarium because the smell of the saccharin may influence the association with the immunological agent.
Retention interval
Basic Protocol 1 commonly uses a short-term, 2-day retention interval, i.e., the time interval between acquisition and retrieval (Hadamitzky et al., 2015; Hadamitzky, Bösche, et al., 2016; Hadamitzky, Orlowski, et al., 2016). Given that CsA has a very short half-life, residual effects are not present at retrieval after a 2-day retention interval. However, a prolonged retention interval can be applied (Hörbelt et al., 2019). Use of other immunopharmacological drugs with distinct cell signaling pathways may be applicable (Lückemann et al., 2019). However, these substances may also differ in half-life and drug wash-out phase and therefore may need a prolonged retention interval. If no information is available in the literature, determine residual effects of the desired drug before applying it as the US in the protocol. For this purpose, inject a specific dose of the desired drug on three subsequent days. Perform ex vivo immunological analyses such as ELISA, FACS, or PCR after different retention intervals (e.g., 2, 5, 7, 10 days; Basic Protocol 2). Within the experimental protocol, use the time point when no immunological effects are detectable any more as the “determined” retention interval.
Interventions
If one plans on implementing a surgical intervention (e.g., brain cannulas for EEG), keep in mind that pharmacological treatment for analgesia (such as opioids) may affect the learning and/or memory process of taste-immune conditioning. In this case, conduct surgeries some days before initiating the conditioning protocol (Basic Protocol 1).
Understanding Results
During the first acquisition trial, animals in all groups should show a marginal neophobic response toward the new taste stimulus saccharin, indicated by reduced fluid intake, which is calculated in relation to the morning water intake over the training period (approximately 10% to 20%). The second and third CS-US association trial should produce reliable avoidance effects toward the saccharin (typically with <50% of regular fluid intake) in all conditioned groups but the “VEH-G.” controls (Fig. 5A), calculated in relation to the morning water intake during training. During prolonged unreinforced re-exposure to the CS, animals learn that the CS no longer predicts the US, with the consequence that the behavior elicited by the CS extinguishes. Thus, on the third retrieval day, CTA is most commonly not as pronounced as on retrieval days 1 and 2. However, after three CS re-exposures to the taste stimulus, CTA is usually robust and obvious in conditioned animals (“CS-G.”; typically with <80% of regular fluid intake). If the CTA is rather weak, note that it is not a significant CTA but rather re-exposure to the taste of the CS itself that is essential for reliable conditioned immunosuppression (Ader & Cohen, 1982; Bovbjerg et al., 1984; Bovbjerg et al., 1987; Exton, von Hörsten, Schult, et al., 1998; Exton et al., 2002).
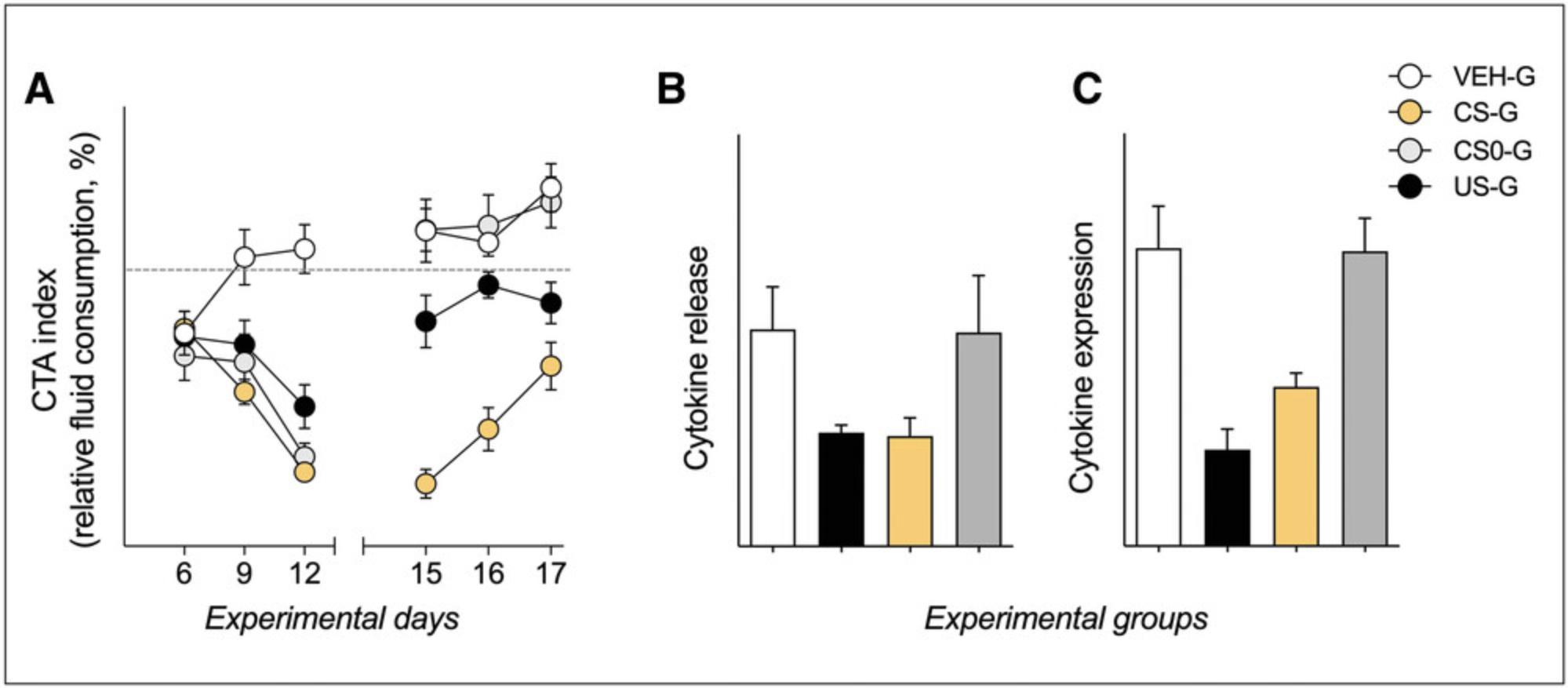
When the animals are euthanized for immunological analyses (Basic Protocol 2) 60 min following the third CS re-exposure, a behaviorally conditioned reduction in IL-2 protein and mRNA production should occur in CsA-treated animals (“US-G.”; approximately 50% to 60% in relation to controls) as well as conditioned animals (“CS-G.”; approximately 60% to 75% in relation to controls; Fig. 5B and 5C), assessed in ex vivo stimulated splenic T cells. In any case, always look at the whole dataset, comprising not only CTA but also immunological readouts (Hadamitzky, Bösche, et al., 2016).
Time Considerations
The full conditioning paradigm described in Basic Protocol 1 can be completed in 17 days (see Fig. 1 and 2). The training phase lasts from days 1 to 5, where animals learn to drink twice a day for 15 min in the morning and evening sessions. On days 6 to 12, the acquisition phase is initiated, where animals learn to associate the new unfamiliar taste of saccharin with immunological alterations induced by injections of the immunosuppressant CsA. Association trials are conducted on days 6, 9, and 12, whereas on the days in between, animals receive water twice a day for 15 min, as in the training phase. After a 2-day retention interval on days 13 and 14, the retrieval phase lasts from days 15 to 17, where only conditioned animals are re-exposed to saccharin (“CS-G.”). The most time-sensitive steps are on the acquisition days 6, 9, and 12, as well as during retrieval (days 15 to 17), because it is best to inject the animals with CsA (US) as quickly as possible following presentation of the saccharin (CS). Note that drug (CsA) preparation before acquisition and retrieval may require ∼30 min, and daily weighing of the water/saccharin bottles following the drinking sessions may require about 15 min.
The splenocyte isolation and cultivation described in Basic Protocol 2 can be completed in 3 days. Harvesting of the spleen and subsequent isolation of cells need to be conducted 60 min following the last CS re-exposure on day 1 (which is day 17 of Basic Protocol 1). Further cultivation and stimulation with anti-CD3 are performed on the same day. Extraction of mRNA is performed 4 hr after stimulation, on the same day. Supernatants of stimulated splenocytes are collected after 48 hr of stimulation and either examined by means of ELISA (cytokine release) on that same day (day 3) or stored at −80°C for later analysis.
Acknowledgments
We thank Marie Jakobs and Jan Alexander Heistermann for checking the current catalog numbers for the Materials lists.
This work was supported by a center grant of the German Research Foundation (DFG) SFB1280, TP A18, project no. 316803389.
Open access funding enabled and organized by Projekt DEAL.
Author Contributions
Stephan Leisengang : Methodology, Visualization, Writing─original draft, Writing─review and editing; Manfred Schedlowski : Funding acquisition, Project administration, Supervision, Writing─review and editing; Martin Hadamitzky : Methodology, Supervision, Visualization, Writing─original draft, Writing─review and editing; Laura Lückemann : Conceptualization, Investigation, Methodology, Visualization, Writing─original draft, Writing─review and editing.
Conflict of Interest
The authors declare no conflict of interest.
Open Research
Data Availability Statement
Data sharing is not applicable to this article as no new data were created or analyzed in this study.
Literature Cited
- Ader, R., & Cohen, N. (1975). Behaviorally conditioned immunosuppression. Psychosomatic Medicine , 37(4), 333–340. doi: 10.1097/00006842-197507000-00007
- Ader, R., & Cohen, N. (1982). Behaviorally conditioned immunosuppression and murine systemic lupus erythematosus. Science , 215(4539), 1534–1536. doi: 10.1126/science.7063864
- Bovbjerg, D., Ader, R., & Cohen, N. (1984). Acquisition and extinction of conditioned suppression of a graft-vs-host response in the rat. Journal of Immunology , 132(1), 111–113.
- Bovbjerg, D., Kim, Y. T., Siskind, G. W., & Weksler, M. E. (1987). Conditioned suppression of plaque-forming cell responses with cyclophosphamide. The role of taste aversion. Annals of the New York Academy of Sciences , 496, 588–594. doi: 10.1111/j.1749-6632.1987.tb35818.x
- Cohen, N., Ader, R., Green, N., & Bovbjerg, D. (1979). Conditioned suppression of a thymus-independent antibody response. Psychosomatic Medicine , 41(6), 487–491. doi: 10.1097/00006842-197910000-00005
- Davis, J. A. (2008). Mouse and rat anesthesia and analgesia. Current Protocols in Neuroscience , 42, A.4B.1–A.4B.21. doi: 10.1002/0471142301.nsa04bs42
- Donovan, J., & Brown, P. (1995). Parenteral injections. Current Protocols in Immunology , 14(1), 1.6.1–1.6.10. doi: 10.1002/0471142735.im0106s14
- Donovan, J., & Brown, P. (2006). Euthanasia. Current Protocols in Immunology , 73, 1.8.1–1.8.4. doi: 10.1002/0471142735.im0108s73
- Exton, M. S., Bull, D. F., King, M. G., & Husband, A. J. (1995). Modification of body temperature and sleep state using behavioral conditioning. Physiology & Behavior, 57(4), 723–729. doi: 10.1016/0031-9384(94)00314-9
- Exton, M. S., Gierse, C., Meier, B., Mosen, M., Xie, Y., Frede, S., … Schedlowskia, M. (2002). Behaviorally conditioned immunosuppression in the rat is regulated via noradrenaline and β-adrenoceptors. Journal of Neuroimmunology , 131(1-2), 21–30. doi: 10.1016/S0165-5728(02)00249-7
- Exton, M. S., von Hörsten, S., Schult, M., Vöge, J., Strubel, T., Donath, S., … Schedlowski, M. (1998). Behaviorally conditioned immunosuppression using cyclosporine A: Central nervous system reduces IL-2 production via splenic innervation. Journal of Neuroimmunology , 88(1-2), 182–191. doi: 10.1016/S0165-5728(98)00122-2
- Exton, M. S., von Hörsten, S., Vöge, J., Westermann, J., Schult, M., Nagel, E., & Schedlowski, M. (1998). Conditioned taste aversion produced by cyclosporine A: Concomitant reduction in lymphoid organ weight and splenocyte proliferation. Physiology & Behavior, 63(2), 241–247. doi: 10.1016/s0031-9384(97)00432-0
- Ghanta, V. K., Hiramoto, R. N., Solvason, H. B., & Spector, N. H. (1985). Neural and environmental influences on neoplasia and conditioning of NK activity. Journal of Immunology , 135(2), 848s–852s.
- Goebel, M. U., Trebst, A. E., Steiner, J., Xie, Y. F., Exton, M. S., Frede, S., … Schedlowski, M. (2002). Behavioral conditioning of immunosuppression is possible in humans. FASEB Journal , 16(14), 1869–1873. doi: 10.1096/fj.02-0389com
- Graham, R. M. (1994). Cyclosporine: Mechanisms of action and toxicity. Cleveland Clinic Journal of Medicine , 61(4), 308–313. doi: 10.3949/ccjm.61.4.308
- Hadamitzky, M., Bösche, K., Engler, A., Schedlowski, M., & Engler, H. (2015). Extinction of conditioned taste aversion is related to the aversion strength and associated with c-fos expression in the insular cortex. Neuroscience , 303, 34–41. doi: 10.1016/j.neuroscience.2015.06.040
- Hadamitzky, M., Engler, H., & Schedlowski, M. (2013). Learned immunosuppression: Extinction, renewal, and the challenge of reconsolidation. Journal of Neuroimmune Pharmacology , 8(1), 180–188. doi: 10.1007/s11481-012-9388-6
- Hadamitzky, M., Bösche, K., Wirth, T., Buck, B., Beetz, O., Christians, U., … Schedlowski, M. (2016). Memory-updating abrogates extinction of learned immunosuppression. Brain, Behavior, and Immunity , 52, 40–48. doi: 10.1016/j.bbi.2015.09.009
- Hadamitzky, M., Lückemann, L., Pacheco-López, G., & Schedlowski, M. (2020). Pavlovian conditioning of immunological and neuroendocrine functions. Physiological Reviews , 100(1), 357–405. doi: 10.1152/physrev.00033.2018
- Hadamitzky, M., Orlowski, K., Schwitalla, J. C., Bösche, K., Unteroberdörster, M., Bendix, I., … Schedlowskia, M. (2016). Transient inhibition of protein synthesis in the rat insular cortex delays extinction of conditioned taste aversion with cyclosporine A. Neurobiology of Learning and Memory , 133, 129–135. doi: 10.1016/j.nlm.2016.06.008
- Hadamitzky, M., & Schedlowski, M. (2022). Harnessing associative learning paradigms to optimize drug treatment. Trends in Pharmacological Sciences , 43(6), 464–472. doi: 10.1016/j.tips.2022.03.002
- Hörbelt, T., Martínez-Gómez, E. M., Hadamitzky, M., Schedlowski, M., & Lückemann, L. (2019). Behaviorally conditioned immunosuppression with cyclosporine A forms long lasting memory trace. Behavioural Brain Research , 376, 112208. doi: 10.1016/j.bbr.2019.112208
- Janz, L. J., Green-Johnson, J., Murray, L., Vriend, C. Y., Nance, D. M., Greenberg, A. H., & Dyck, D. G. (1996). Pavlovian conditioning of LPS-induced responses: Effects on corticosterone, splenic NE, and IL-2 production. Physiology & Behavior, 59(6), 1103–1109. doi: 10.1016/0031-9384(95)02171-x
- Kapturczak, M. H., Meier-Kriesche, H. U., & Kaplan, B. (2004). Pharmacology of calcineurin antagonists. Transplantation Proceedings , 36(2 Suppl), 25S–32S. doi: 10.1016/j.transproceed.2004.01.018
- Lückemann, L., Stangl, H., Straub, R. H., Schedlowski, M., & Hadamitzky, M. (2020). Learned immunosuppressive placebo response attenuates disease progression in a rodent model of rheumatoid arthritis. Arthritis Rheumatol , 72(4), 588–597. doi: 10.1002/art.41101
- Lückemann, L., Unteroberdörster, M., Martinez Gomez, E., Schedlowski, M., & Hadamitzky, M. (2019). Behavioral conditioning of anti-proliferative and immunosuppressive properties of the mTOR inhibitor rapamycin. Brain, Behavior, and Immunity , 79, 326–331. doi: 10.1016/j.bbi.2019.04.013
- Lueckemann, L., Bösche, K., Engler, H., Schwitalla, J.-C., Hadamitzky, M., & Schedlowski, M. (2016). Pre-exposure to the unconditioned or conditioned stimulus does not affect learned immunosuppression in rats. Brain, Behavior, and Immunity , 51, 252–257. doi: 10.1016/j.bbi.2015.09.005
- Lysle, D. T., Cunnick, J. E., Fowler, H., & Rabin, B. S. (1988). Pavlovian conditioning of shock-induced supression of lymphocyte reactivity: Acquisition, extinction, and preexposure effects. Life Sciences , 42(22), 2185–2194. doi: 10.1016/0024-3205(88)90369-4
- Metalnikov, S., & Chorine, V. (1926). Rôle des réflexes conditionel dan l‘immunité. Annales de l‘Institut Pasteur , 1640.
- O'Shea, J. J., Ma, A., & Lipsky, P. (2002). Cytokines and autoimmunity. Nature Reviews Immunology , 2(1), 37–45. doi: 10.1038/nri702
- Pacheco-López, G., Doenlen, R., Krügel, U., Arnold, M., Wirth, T., Riether, C., … Schedlowski, M. (2013). Neurobehavioural activation during peripheral immunosuppression. The International Journal of Neuropsychopharmacology , 16(1), 137–149. doi: 10.1017/S1461145711001799
- Pacheco-López, G., Riether, C., Doenlen, R., Engler, H., Niemi, M.-B., Engler, A., … Schedlowski, M. (2009). Calcineurin inhibition in splenocytes induced by pavlovian conditioning. FASEB Journal , 23(4), 1161–1167. doi: 10.1096/fj.08-115683
- Pavlov, I. P. (1928). Conditioned reflexes. London: M. Lawrence.
- Sinclair, A. H., & Barense, M. D. (2019). Prediction error and memory reactivation: How incomplete reminders drive reconsolidation. Trends in Neuroscience , 42(10), 727–739. doi: 10.1016/j.tins.2019.08.007
- Steinbach, W. J., Reedy, J. L., Cramer, R. A., Perfect, J. R., & Heitman, J. (2007). Harnessing calcineurin as a novel anti-infective agent against invasive fungal infections. Nature Reviews Microbiology , 5(6), 418–430. doi: 10.1038/nrmicro1680
- von Hörsten, S., Exton, M. S., Vöge, J., Schult, M., Nagel, E., Schmidt, R. E., … Schedlowski, M. (1998). Cyclosporine A Affects Open Field Behavior in DA Rats. Pharmacology Biochemistry and Behavior , 60(1), 71–76. doi: 10.1016/S0091-3057(97)00467-X
- White, D. J. (1982). Cyclosporin A. Clinical pharmacology and therapeutic potential. Drugs , 24(4), 322–334. doi: 10.2165/00003495-198224040-00004