An In Vivo Mouse Model for Chronic Inflammation–Induced Immune Suppression: A “Factory” for Myeloid-Derived Suppressor Cells (MDSCs)
Kerem Ben-Meir, Kerem Ben-Meir, Nira Twaik, Nira Twaik, Yaron Meirow, Yaron Meirow, Michal Baniyash, Michal Baniyash
Abstract
Myeloid-derived suppressor cells (MDSCs) represent a heterogeneous population of immature myeloid cells known to play a role in perpetuating a wide range of pathologies, such as chronic infections, autoimmune diseases, and cancer. MDSCs were first identified in mice by the markers CD11b+Gr1+, and later, based on their morphology, they were classified into two subsets: polymorphonuclear MDSCs, identified by the markers CD11b+Ly6G+Ly6CLow, and monocytic MDSCs, detected as being CD11b+Ly6G–Ly6CHi. MDSCs are studied as immunosuppressive cells in various diseases characterized by chronic inflammation and are associated with disease causes/triggers such as pathogens, autoantigens, and cancer. Therefore, different diseases may diversely affect MDSC metabolism, migration, and differentiation, thus influencing the generated MDSC functional features and ensuing suppressive environment. In order to study MDSCs in a pathology-free environment, we established and calibrated a highly reproducible mouse model that results in the development of chronic inflammation, which is the major cause of MDSC accumulation and immune suppression. The model presented can be used to study MDSC phenotypes, functional diversity, and plasticity. It also permits study of MDSC migration from the bone marrow to peripheral lymphatic and non-lymphatic organs and MDSC crosstalk with extrinsic factors, both in vivo and ex vivo. Furthermore, this model can serve as a platform to assess the effects of anti-MDSC modalities. © 2022 The Authors. Current Protocols published by Wiley Periodicals LLC.
Basic Protocol : Repetitive M.tb immunizations for the induction of chronic inflammation
Alternate Protocol 1 : Creating a lower grade of inflammation by changing the site of immunization
Alternate Protocol 2 : In vivo evaluation of immune status
Support Protocol 1 : Preparation of reconstituted M.tb aliquots and M.tb-IFA emulsions for each of the three injections
Support Protocol 2 : Preparation of an ovalbumin lentiviral expression vector
Support Protocol 3 : Fluorescence titering assay for the lentiviral expression vector
Support Protocol 4 : Spleen excision, tissue dissociation, and preparation of a single-cell suspension
Support Protocol 5 : Labeling of splenocytes with CFSE proliferation dye
INTRODUCTION
Chronic inflammatory conditions are characterized by prolonged stimulation of the immune system and are commonly found in association with chronic infections, autoimmune diseases, or cancer (Baniyash, 2016; Ben-meir, Twaik, & Baniyash, 2018; Bronte et al., 1998; Wang, Tan, Hou, & Dou, 2021; Yan et al., 2020). This prolonged stimulation and the inability of the immune system to clear the inflammatory reaction are accompanied by the accumulation of polymorphonuclear myeloid-derived suppressor cells (PMN-MDSCs) and monocytic MDSCs (M-MDSCs), which share common characteristics among different diseases that involve immune suppression (Ashkenazi-Preiser, Mikula, Baniyash, & Baniyash, 2021; Ben-meir et al., 2018; Gabrilovich et al., 2007; Ostrand-Rosenberg, Sinha, Chornoguz, & Ecker, 2012; Seung, Rowley, Dubey, Schreiber, & Rowley, 1995). Several murine models can be used for research on MDSC biology. The majority of these include transgenic mouse models, orthotopic tumor implantation, or chemically induced cancers. However, these models feature lower numbers of accumulating MDSCs, thus limiting the yield of cell isolation processes from the spleen, bone marrow (BM), and other inflamed tissues (e.g., tumor, colon). Moreover, MDSCs in various models may exhibit variability in their suppressive phenotype due to the pathology/chronic inflammatory cause.
In the protocols described herein, we present a pathology-free mouse model for chronic inflammation, which is associated with elevated levels of MDSCs and ensuing immune suppression, as evident in the above-described pathologies. This model is achieved by repetitive immunizations with heat-killed Mycobacterium tuberculosis (M.tb), which leads to the induction of a prolonged Th1-dependent immune response, which results in systemic chronic inflammation associated with immune suppression and vast accumulation of MDSCs. Induction of inflammation via immunization with M.tb emulsion has been in use in previously published studies, mostly in the context of adjuvant-induced arthritis (see Current Protocols articles: Fehrenbacher, Vasko, & Duarte, 2012; Marvizon, Walwyn, Minasyan, Chen, & Taylor, 2015; Wagner, Bindler, & Andriambeloson, 2008), and has not been applied for the induction of chronic inflammatory conditions and associated immune suppression.
The presented model enables the isolation of higher amounts of homogenous MDSCs and use of a lower number of mice per experiment. This model system and the associated MDSCs can serve as a platform for testing various biological pathways that can be targeted, followed by proteomic, transcriptomic, and metabolomic analyses. In addition, the isolated MDSCs could be used in adoptive transfer experiments to suppress or attenuate exacerbated immune responses, as in autoimmune diseases and organ transplants (Cai et al., 2020; Park & Kwok, 2022).
The presented pathology-free chronic inflammation lasts for 16 days and is induced by three immunizations with M.tb (Fig. 1A and 1B). The first immunization is administered subcutaneously (SC); the second intra-footpad (IFP), injected into one paw; and the third intraperitoneally (IP). The first two immunizations are composed of M.tb emulsified in incomplete Freund's adjuvant (IFA), whereas the third dose is soluble M.tb in saline, given as a booster. This immunization regimen induces a strong Th1-dependent chronic inflammatory immune response, associated with the accumulation of MDSCs featuring enhanced suppressive activity ex vivo and in vivo (Salgame, 2005).
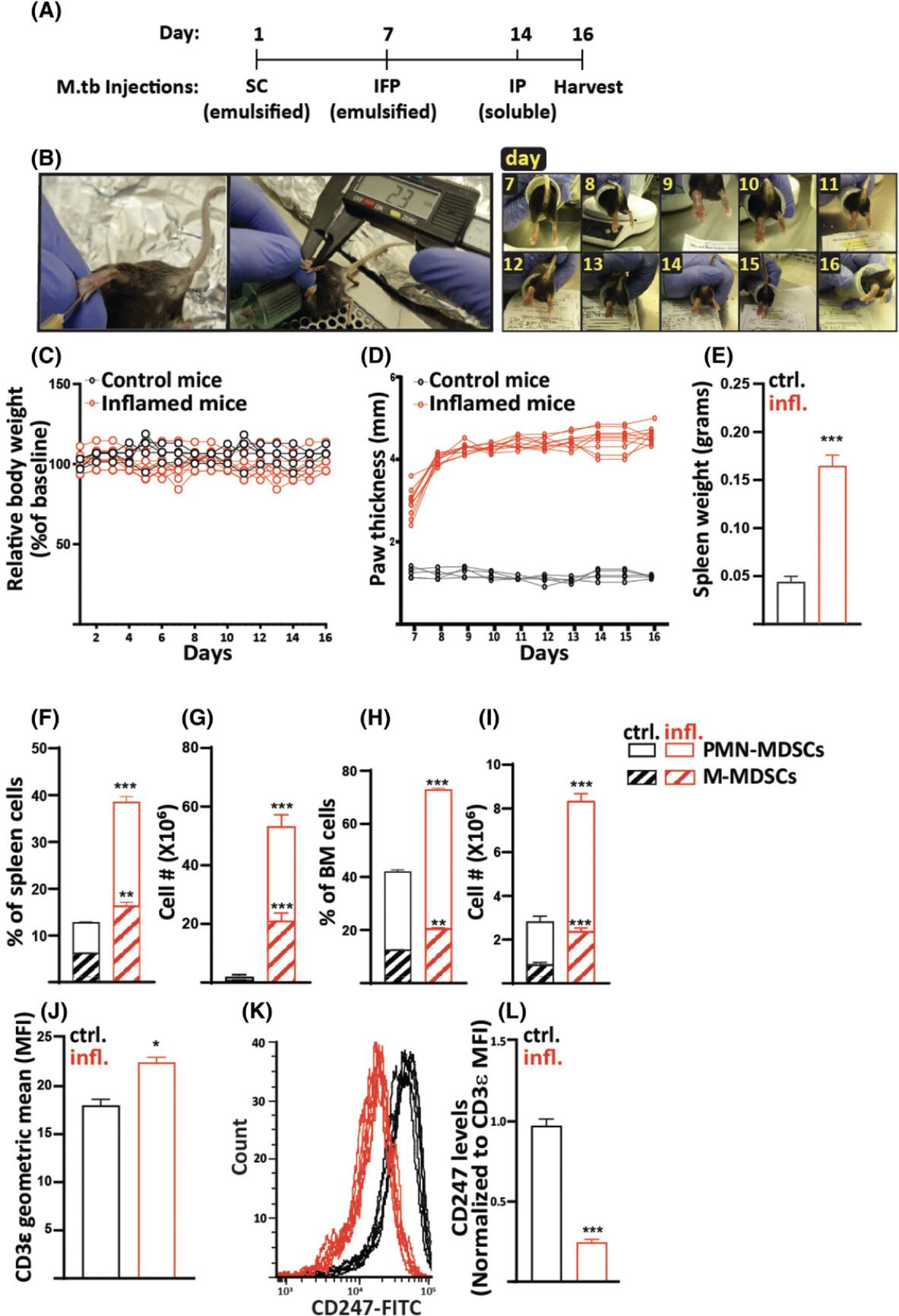
The IFP injection induces a strong immune response together with uncomfortable swelling for the animals. This emphasizes the importance of giving analgesics to the mice from the second injection onward. As non-steroidal anti-inflammatory drugs (NSAIDs) will weaken the immune response, we recommend use of opioid analgesics such as buprenorphine. Use of buprenorphine in this model has been studied by our lab and was not shown to affect the inflammation or the MDSC accumulation and suppressive phenotype (Supporting Information, Fig. 1).
The main protocol described in this article is the Basic Protocol, for the induction of chronic inflammation and associated immune suppression by repetitive immunizations with M.tb. We also present the outcome of the developed inflammation and immune suppression by showing in vivo MDSC accumulation together with increases in additional markers of inflammation, all described in the Understanding Results section and Figure 1. In Support Protocol 1, you will find detailed instructions for the preparation of the M.tb aliquots and use of those when preparing the M.tb emulsion for injections. As the inflammation created under the conditions of the Basic Protocol is highly robust, we also describe a method to create a low-grade inflammation by alternating the site of the second M.tb injection from the mouse footpad to the mouse side flank (see Alternate Protocol 1 and the outcome described in Fig. 2). To show that the immune system of the mice in the presented model is suppressed, we demonstrate how antigen-specific T cells, which are adoptively transferred into mice that were previously immunized with the same antigen, fail to respond to the specific antigen and proliferate the immune-suppressed mice. Instructions for immunization and adoptive transfer are described in Alternate Protocol 2, preparation of the immunization vector is described in Support Protocol 2, and the method for quantifying the prepared vector is described in Support Protocol 3. Preparation of a single-cell suspension of splenocytes, which is a prerequisite for various procedures, such as cell separation, cell analysis, and cell culture, is described in Support Protocol 4. Guidelines for the labeling of splenocytes with CFSE cell proliferation dye are described in Support Protocol 5.
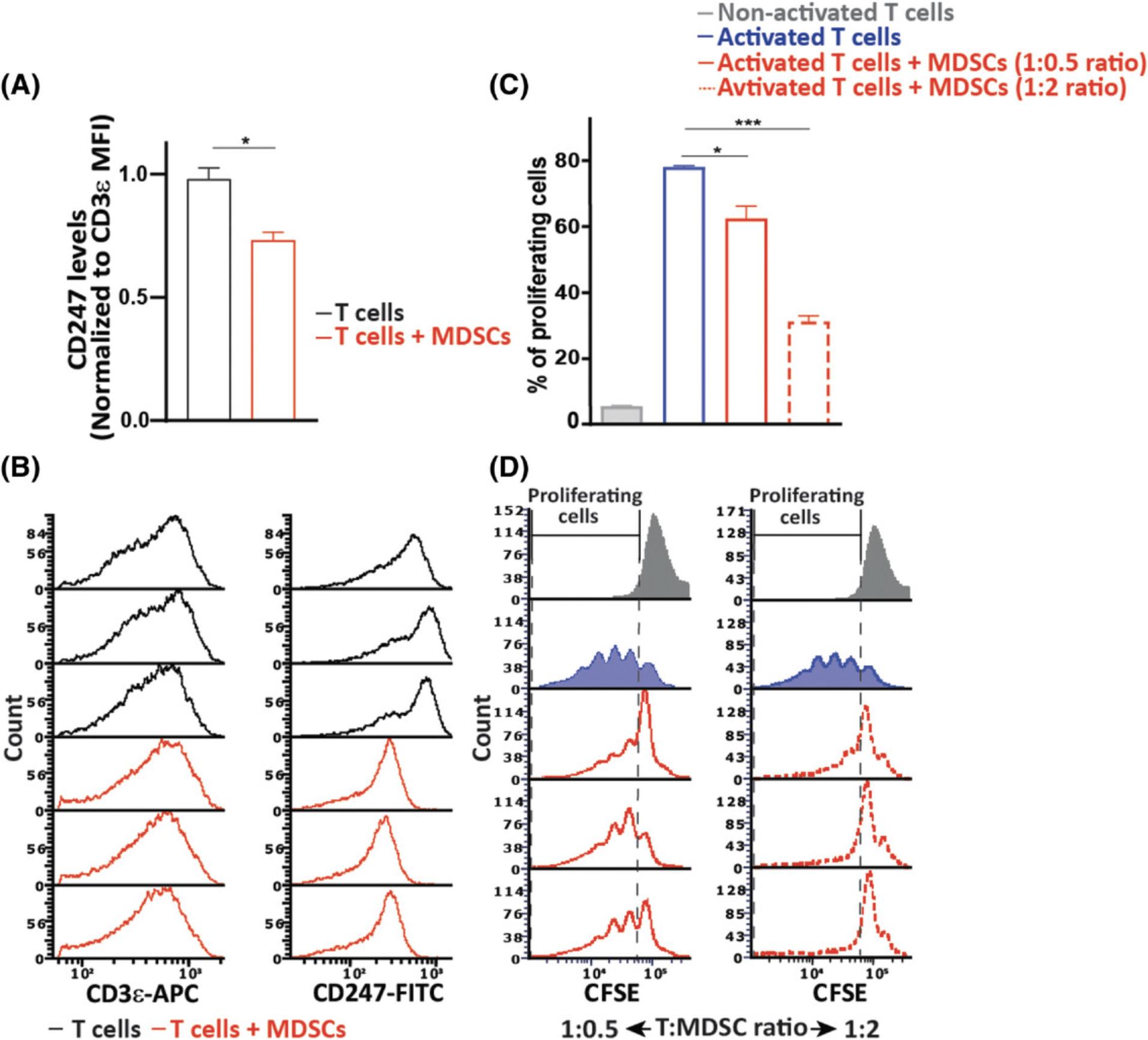
NOTE : All protocols using live animals must first be reviewed and approved by an Institutional Animal Care and Use Committee (IACUC) and must follow officially approved procedures for the care and use of laboratory animals. In particular, the method of euthanasia should be consistent with animal ethics regulations.
Basic Protocol: REPETITIVE M.tb IMMUNIZATIONS FOR THE INDUCTION OF CHRONIC INFLAMMATION
The main protocol for the induction of chronic inflammation and associated immune suppression by repetitive immunizations of mice with M.tb is presented below. Refer to Support Protocol 1 for detailed instructions for the preparation of the M.tb aliquots and use of those when preparing the M.tb emulsion for injections.
Materials
-
4 to 6 male or female, 8- to 10-week-old C57BL/6J mice (The Jackson Laboratory, cat. no. 000664)
-
1 mg/ml M.tb emulsion (see Support Protocol 1, steps 4 to 12)
-
0.3 mg/ml buprenorphine (Bupaq Multidose)
-
Phosphate-buffered saline (PBS; Dulbecco's PBS, without Ca2+ and Mg2+, Biological Industries, cat. no. 02-023-1A)
-
2 mg/ml concentrated M.tb emulsion (see Support Protocol 1, steps 13 to 15)
-
Flow vaporizer for isoflurane inhalational anesthesia (VetFlo isoflurane system), with induction chamber and rodent face-mask adaptor
-
Alcohol wipes
-
2.5-ml Luer syringes with 25-G needles (for M.tb emulsion injections)
-
Digital weighing scale (suitable for mice)
-
1-ml Luer syringes with 27-G needles (for buprenorphine injections)
-
Additional reagents and equipment for preparing 0.5 mg/ml M.tb solution (see Support Protocol 1, steps 16 to 19), for mouse euthanasia by CO2 asphyxiation or cervical dislocation, and for tissue harvesting
CAUTION : Use of an isoflurane flow vaporizer to anesthetize the mice is considered safe when compared to use of ketamine in combination with xylazine, with very short recovery time, minimal stress to the animals, and lower morbidity rates (Woodward et al., 2007). However, a high dose of isoflurane can be lethal. Use of isoflurane thus requires proper guidance.
NOTE : The first M.tb emulsion injection, between the two scapulae, should be SC. Make sure you are experienced and trained in the method of injection ahead of time.
Acclimate the animals
1.Allow 4 to 6 male or female, 8- to 10-week-old C57BL/6J mice acclimate for ≥3 days under standard lighting and temperature conditions of the animal facility, with food and water ad libitum , to eliminate the effects of stress.
Day 1: First injection
2.Place a mouse into the induction chamber of the flow vaporizer for isoflurane inhalational anesthesia and allow it to inhale isoflurane until unconscious.
3.Take mouse out of the chamber and transfer it to the rodent face-mask adaptor.
4.Sterilize site of injection, between the two scapulae, using alcohol wipes.
5.Inject 100 µl of 1 mg/ml M.tb emulsion (50 µg M.tb, prepared as in Support Protocol 1, steps 4 to 12) SC using a 2.5-ml Luer syringe with a 25-G needle and then return animal to its cage.
Day 7: Second injection
6.Prepare buprenorphine at a concentration of 0.015 mg/ml (15 µg/ml) by adding 50 µl of 0.3 mg/ml buprenorphine stock solution to 950 µl PBS.
7.Place a mouse on digital weighing scale, document animal's weight, and then inject one of the side flanks with buprenorphine 0.05 mg/kg (0.05 µg/g) per mouse SC using a 1-ml Luer syringe with a 27-G needle (i.e., for a mouse weight of 25 g, inject 80 µl, which is 1.25 µg buprenorphine). Upon completion, transfer mouse to the induction chamber and allow it to inhale isoflurane until unconscious.
8.Take mouse out of the chamber and transfer it to mask adaptor.
9.Sterilize mouse's paw at site of injection using alcohol wipes.
10.Inject 50 µl of 2 mg/ml concentrated M.tb emulsion (from Support Protocol 1, steps 13 to 15) IFP, in the mouse's paw (see picture in Fig. 1B), using a 2.5-ml Luer syringe with a 25-G needle and return animal to its cage.
11.Continue to administer buprenorphine on a daily basis by repeating steps 6 to 7, omitting the anesthesia.
Day 14: Third injection
12.Prepare 0.5 mg/ml M.tb solution (60 µl of 10 mg/ml M.tb stock in 1140 µl PBS) as in Support Protocol 1, steps 16 to 19.
13.Take a mouse out of the chamber, inject 100 µl of 0.5 mg/ml M.tb solution IP using a 1-ml syringe with a 27-G needle, and return animal to its cage.
Sacrifice on day 16
14.Euthanize mice using either CO2 asphyxiation (<20% displacement/min) or cervical dislocation.
15.Harvest tissues.
Alternate Protocol 1: CREATING A LOWER GRADE OF INFLAMMATION BY CHANGING THE SITE OF IMMUNIZATION
The mouse model presented in the Basic Protocol is ideal when a strong systemic inflammatory immune response accompanied by large numbers of highly suppressive MDSCs is desired (see Fig. 1 and 2 and Understanding Results). However, for some studies, when assessing the effects of therapeutic modalities, the robust inflammation and elevated levels of MDSCs could mask differences in the in vivo phenotypes that would otherwise be measurable. With the purpose of reducing the immune response and the severity of the associated inflammation and immune suppression, it is possible to alter the third step within this model and thereby cause a milder inflammation.
Carry out the Basic Protocol with the following change: Perform the second injection (step 10 in the Basic Protocol) SC in one of the side flanks of each mouse instead of IFP (see Fig. 3A for a timeline of the model). As in the Basic Protocol, inject 50 µl M.tb emulsion. Note that the side-flank injection does not require analgetic drug administration. Altering the site of injection from the footpad to the side flank induces lower splenic MDSC accumulation than that observed in the original model. The inflammation is accompanied by an increase in BM and spleen MDSC percentages (Fig. 3B and 3C, respectively), and although milder than the IFP model in the Basic Protocol, downregulated expression of T-cell CD247 is still observed (Fig. 3D). This suggests that by changing the site of immunization, the level of immune suppression can be lowered as compared to the robust suppression found in the original model.
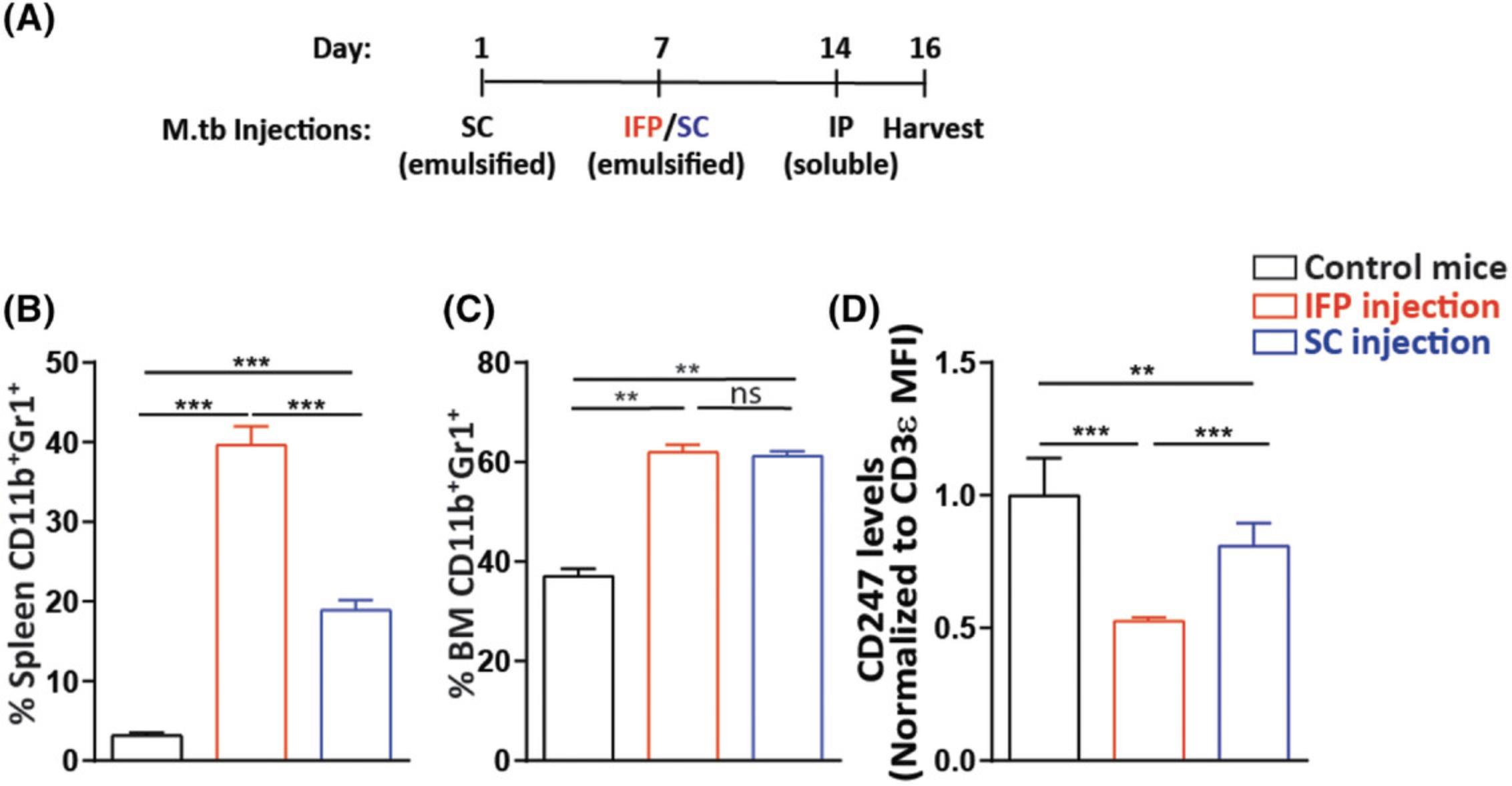
Alternate Protocol 2: IN VIVO EVALUATION OF IMMUNE STATUS
The in vivo immune-suppressive state of the mice (Basic Protocol) can be evaluated by introducing the immune system to a challenge and measuring the corresponding response. Such responses to challenges include cell-based immune reactions such as the mixed lymphocyte reaction (MLR) (Song et al., 1999), in vivo killing (Sade-Feldman et al., 2013; Vaknin et al., 2008), recovery from viral infection (Bronstein-Sitton et al., 2003), and proliferation of T cells in response to a known antigen (described herein).
Assessment of T-cell proliferation in vivo in an antigen-specific manner is based on use of transgenic CD8+OT-I+ T cells expressing the Vα2 Vβ5 chains, comprising a T-cell receptor (TCR) that recognizes ovalbumin (OVA) peptide (OVA257-264) in the context of H2Kb (MHC class I) (Hogquist et al., 1994). Immunization with a viral vector coding for OVA is performed by an intradermal injection into the mouse ear pinnae, which is a highly efficient vaccination method (Lin, Smith, & Tscharke, 2012). Such an immunization is designed to induce expression of the foreign OVA protein and in vivo presentation of the OVA peptide by infected cells. Whereas adoptive transfer of OT-I+ T cells into control-immunized recipient mice will result in these cells’ activation and proliferation, their transfer into chronically inflamed immunized mice will result in these cells’ suppression and an inability of these cells to proliferate. This will indicate that the in vivo immune-suppressive environment generated during chronic inflammation inhibits the activation and proliferation of the transplanted T cells, as shown in Figure 4.Assessment of T-cell proliferation is carried out by a) labeling splenocytes with CFSE cell proliferation dye prior to the adoptive transfer and b) tracking proliferating cells after the adoptive transfer, as indicated by decay of their fluorescence intensity. A labeled cell that divides will give rise to two daughter cells that will have roughly half of the fluorescence of the original mother cell. Thus, the meaning of a sample with a high percentage of cells exhibiting low fluorescence compared to another sample with a lower percentage of cells displaying the same fluorescence intensity is that the first sample has higher proliferation rates.
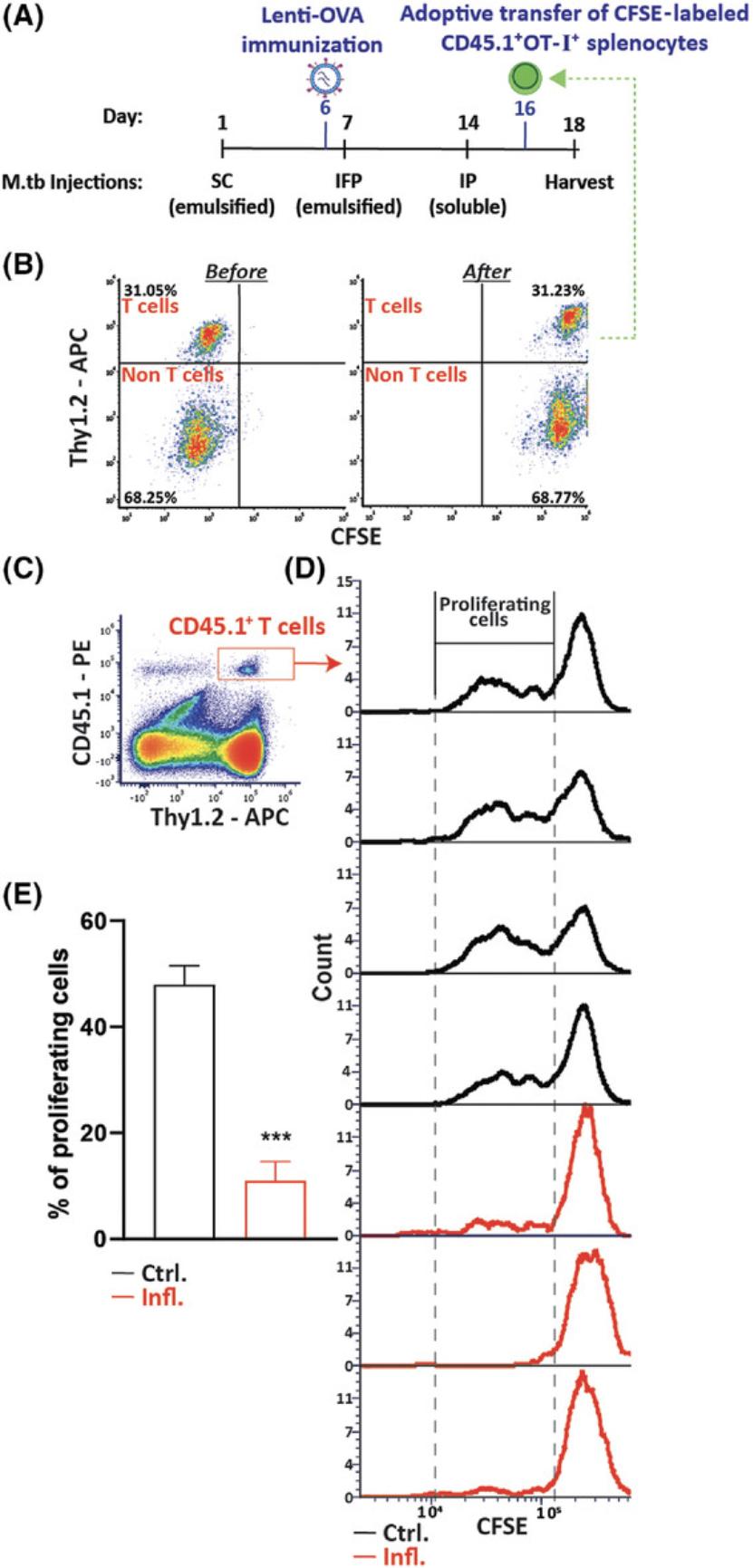
Materials
-
Recipient mice: male or female wild-type C57BL/6 mice (The Jackson Laboratory, cat. no. 000664), 8 to 10 weeks old, divided into two groups: 4 to 6 control (healthy) mice and 4 to 6 mice that are 6 days after first injection of M.tb emulsion (see Support Protocol 1, steps 4 to 12; see Fig. 4A for timeline of injections)
-
1 × 108 TU/ml diluted OVA-albumin lentivector (see Support Protocols 2 and 3)
-
T-cell donor mice: naïve CD45.1+OT-I+ male or female mice, 8 to 10 weeks old, generated by crossing OT-I strain (The Jackson Laboratory, cat. no. 003831) with CD45.1 strain (also known as Ly5.2 or Ly5b; The Jackson Laboratory, cat. no. 002014)
-
Trypan blue (0.4%; Sigma, cat. no. T8154)
-
Sterile PBS (Dulbecco's PBS, without Ca2+ and Mg2+, Biological Industries, cat. no. 02-023-1A)
-
FACS buffer (see recipe)
-
Antibodies:
- APC anti-Thy1.2 (BioLegend, cat. no. 140312)
- PE anti-CD45.1 (BioLegend, cat. no. 110707)
- Purified anti-mouse CD16/32 (Fc block; BioLegend, cat. no. 156603)
-
Flow vaporizer for isoflurane inhalational anesthesia (VetFlo isoflurane system), with induction chamber and rodent face-mask adaptor
-
Alcohol wipes
-
1-ml syringes with 29-G needles
-
Hemocytometer (Hausser Scientific Bright-Line Phase Hemocytometer, Fisher, cat. no. 02-671-6)
-
Light microscope
-
1.7-ml microcentrifuge tubes (Axygen, cat. no. 14-222-156)
-
Microcentrifuge, 4°C
-
Flow cytometer, equipped with lasers for multi-color analysis of FITC, PE, and APC fluorophores
-
Additional reagents or equipment for SC injection of M.tb emulsion (see Basic Protocol, steps 1 to 13), for spleen harvesting and preparation of a single-cell suspension from donor mouse spleens (see Support Protocol 4), and for splenocyte labeling with CFSE proliferation dye (see Support Protocol 5)
NOTE : Make sure that both the donor and the recipient mice are of the same gender and C57BL/6 background and that the host expresses CD45.2, whereas the donor OT-I expresses CD45.1.
NOTE : The inoculation model of infection in mouse ear pinnae is performed as instructed in detail in Lin et al. (2012). Make sure that you are experienced and trained in the method of injection ahead of time.
NOTE : Adoptive transfer of T cells is performed via the lateral tail vein as instructed in detail in Zimmerman, Hu, & Liu (2010). Make sure that you are experienced and trained in the method of injection ahead of time.
M.tb injections (days 1, 7, and 14 in Fig. 4A)
1.Carry out steps 1 to 13 of Basic Protocol, injecting M.tb emulsions as follows: day 1: 100 μg M.tb emulsion SC, day 7: 100 μg M.tb emulsion IFP, and day 14: 100 μg soluble M.tb IP.
Vaccinate recipient mice (CD45.2 mice; day 6 as in Fig. 4A)
2.Place one mouse in induction chamber of the flow vaporizer for isoflurane inhalational anesthesia and allow it to inhale isoflurane until unconscious.
3.Take mouse out of the chamber and transfer it to rodent face-mask adaptor.
4.Sterilize mouse's ear pinnae using alcohol wipes.
5.Inject 50 µl of 1 × 108 TU/ml diluted OVA-albumin lentivector (which is 5 × 106 transducing units, or TU) intradermally into the mouse ear pinnae as in Lin et al. (2012) using a 1-ml syringe with a 29-G needle.
6.Return mouse to its cage.
Adoptive transfer of OT-I splenocytes (day 16 as in Fig. 4A)
7.Prepare a single-cell suspension from T-cell donor mouse spleens as instructed in Support Protocol 4.
8.Count cells with trypan blue using a hemocytometer and light microscope and bring splenocytes to a concentration of 20 × 106 cells/ml in sterile PBS.
9.Label splenocytes with CFSE proliferation dye, as instructed in Support Protocol 5.
10.Bring cells to a concentration of 4 × 106/ml in sterile PBS.
11.Inject 100 µl intravenously (IV), into the lateral tail vein, with a 1-ml syringe with a 29-G needle, and return each animal to its cage.
Analyze proliferation of transplanted cells (day 18 as in Fig. 4A)
12.Harvest spleens of the wild-type immunized mice and prepare a single-cell suspension in FACS buffer, as in Support Protocol 4.
13.Count cells with trypan blue using a hemocytometer and bring them to a concentration of 107 cells/ml in FACS buffer.
14.Prepare antibody mixture for staining the cells by adding 4 µl APC anti-Thy1.2, 4 µl PE anti-CD45.1, and 4 µl purified anti-mouse CD16/32 to 2 ml FACS buffer.
15.Transfer 500 µl from each spleen suspension prepared in steps 12 and 13 (a volume of 500 µl from 107 cells/ml is 5 × 106 cells) to a 1.7-ml microcentrifuge tube.
16.Centrifuge samples for 10 min at 300 × g , 4°C, in a microcentrifuge.
17.Discard supernatant, add 250 µl antibody mixture from step 14 to each tube, and resuspend cells.
18.Incubate in the dark at 4°C for 30 min.
19.Centrifuge samples for 10 min at 300 × g , 4°C.
20.Discard supernatant and resuspend samples in 500 µl FACS buffer.
21.Analyze cells in a flow cytometer.
Support Protocol 1: PREPARATION OF RECONSTITUTED M.tb ALIQUOTS AND M.tb-IFA EMULSIONS FOR EACH OF THE THREE INJECTIONS
This protocol details how to prepare reconstituted M.tb aliquots to be kept in the freezer, followed by instructions for M.tb-IFA emulsion preparation for the different injections (see Basic Protocol and Alternate Protocols 1 and 2).
Materials
-
M.tb (heat-killed M.tb H37 Ra, desiccated, Difco, cat. no. 231141)
-
Sterile PBS (Dulbecco's PBS, without Ca2+ and Mg2++, Biological Industries, cat. no. 02-023-1A)
-
Chilled water
-
IFA (Difco, cat. no. 263910)
-
1.7-ml microcentrifuge tubes (Axygen, cat. no. 14-222-156)
-
Indirect sonicator (e.g., Qsonica Cup Horn sonicator)
-
Vortex
-
1- and 2.5-ml syringes
-
Female-to-female Luer adaptor, for emulsification (see notes below and Fig. 5B)
-
Laminar flow culture hood (Labconco Purifier BSC Class II or equivalent)
-
25-G needles
-
Petri dish
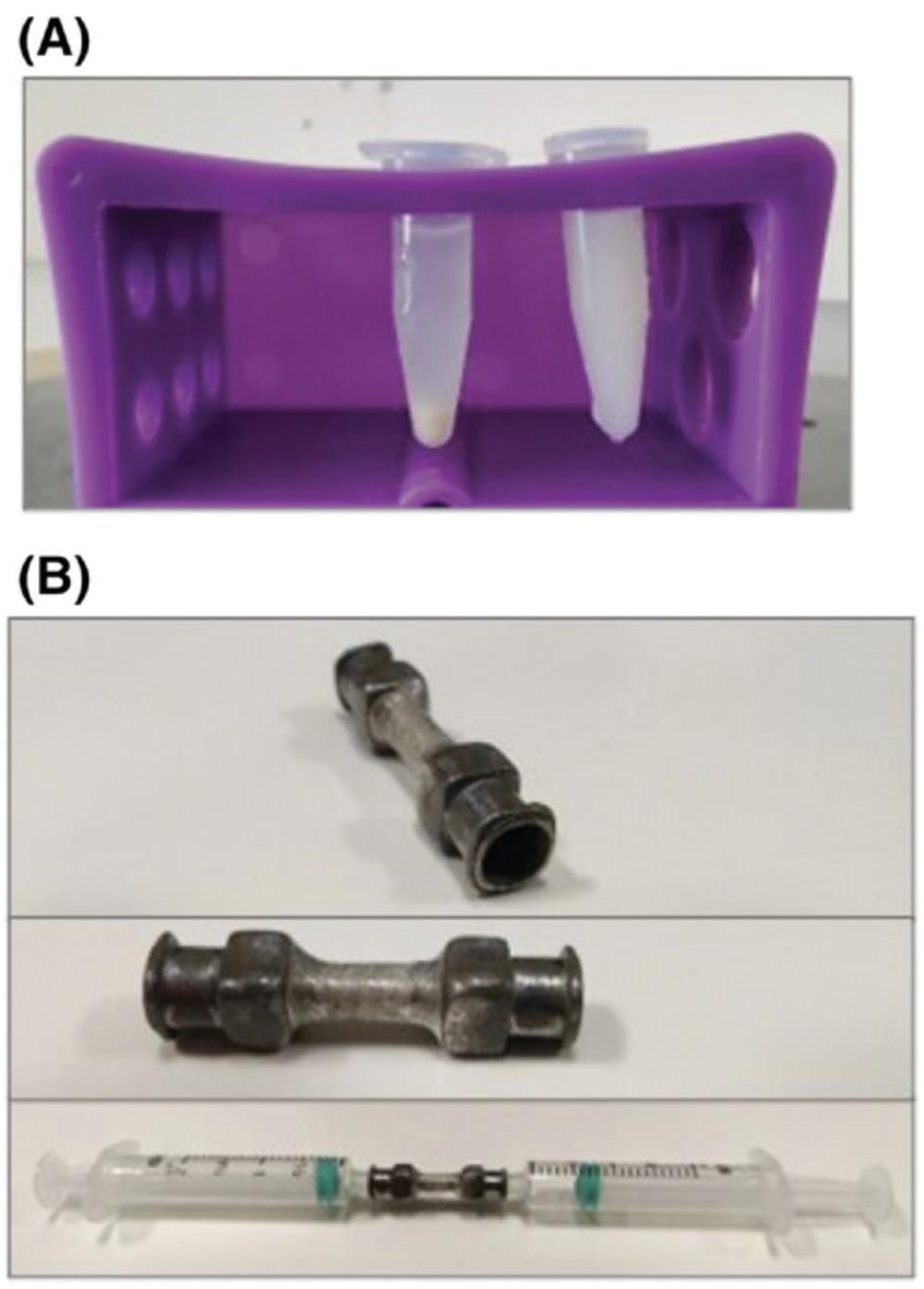
Reconstitution and sonication of M.tb
1.Reconstitute 100 mg M.tb in 10 ml sterile PBS to reach a concentration of 10 mg/ml and divide into 1.4-ml aliquots in 1.7-ml microcentrifuge tubes, resuspending the M.tb between each pipetting.
2.Sonicate each aliquot at an amplitude of 80%, in 10-s ON and 5-s OFF intervals, for 3 min (total of 4.5 min, including the OFF time) in an indirect sonicator. Repeat this cycle 10 times. Replace chilled water in the sonicator cup with new chilled water between each sonication cycle.
3.Prepare 1.7-ml microcentrifuge tubes containing 60 µl of the sonicated M.tb and keep at −20°C.
Preparation of M.tb-IFA emulsion for the first injection
4.Add 540 µl sterile PBS to a thawed aliquot from step 3.Vortex briefly.
5.Sonicate at an amplitude of 80%, in 10-s ON and 5-s OFF intervals, for 1.5 min.
6.Briefly spin down sonicated M.tb tube.
7.Aspirate the M.tb solution into a 2.5-ml syringe and discard air bubbles.
8.Aspirate an equal amount of IFA into another 2.5-ml syringe and discard air bubbles.
9.Connect M.tb solution syringe to the female-to-female Luer adaptor and gently press plunger to remove air from the adaptor. Then, connect IFA syringe to the other side.
10.Making sure that the syringes are tightly connected to the adaptor, press down one of the plungers on the work surface to transfer the liquid to the other syringe. Repeat process 10 to 15 times, alternating between the syringes.
11.Disconnect syringe containing the emulsion and connect a 25-G needle. Dispense one drop of emulsion into a petri dish containing a small amount of PBS. If the drop retains its shape, proceed to using emulsion, which is stable and ready. If the drop disseminates into the PBS, reconnect syringe to the adaptor and repeat mixing solution as in step 10.
12.Use emulsion immediately and make fresh for every use.
Preparation of M.tb-IFA emulsion for the second injection
13.Add 480 µl (not 540 µl) PBS to one thawed M.tb aliquot from step 3 for a total volume of 540 µl (not 600 µl). Vortex briefly.
14.Add the 540 µl to another 60-µl thawed M.tb aliquot to reach a 2 mg/ml concentration, for a total volume of 600 µl. Vortex briefly.
15.Sonicate and emulsify according to steps 5 to 12.
Preparation of M.tb solution for the third injection
16.Add 540 µl PBS to a thawed M.tb aliquot from step 3. Vortex briefly.
17.Sonicate at an amplitude of 80%, in 10-s ON and 5-s OFF intervals, for 1.5 min.
18.Add 600 µl PBS to the 600 µl of sonicated M.tb (to reach 0.5 mg/ml).
19.Connect a 27-G needle to a 1-ml syringe and aspirate solution into the syringe for use.
Support Protocol 2: PREPARATION OF AN OVALBUMIN LENTIVIRAL EXPRESSION VECTOR
Lentiviral vectors for Alternate Protocol 2 are produced by HEK-293T cells co-transfected with the ovalbumin vector plasmid together with the packaging and envelope plasmids. The protocol below includes instructions for working with HEK-293T under cell culture conditions and for preparing lentiviral vectors by transfection of these cells. The transfection method for plasmid delivery is performed using the Mirus Bio Transfection Reagent following the manufacturer's instructions and is described briefly here. We also recommend reading the product datasheet as well as watching the manufacturer's instruction video: https://youtu.be/c31BIB_7A2M.
The method for viral particle concentration described here is in regular use in our lab and provides a high titer together with allowing easy handling when compared to the ultracentrifugation method (Saha, Lin, & Wong, 1994).
Materials
-
Complete RPMI growth medium (see recipe)
-
HEK-293T cells (Merck, cat. no. 12022001)
-
70% (v/v) ethanol
-
Trypsin-EDTA solution (Merck, cat. no. T4049-500ML)
-
TransIT-LT1 Transfection Reagent (Mirus Bio, cat. no. MIR-2300)
-
Opti-MEM (reduced-serum medium; Gibco, cat. no. 31985062)
-
Vector plasmid: 1 mg/ml ovalbumin-GFP expression vector plasmid (Addgene, cat. no. 163524)
-
env plasmid: 1 mg/ml pCMV-VSV-G 2 (Addgene, cat. no. 8454)
-
Packaging plasmid: 1 mg/ml pCMV delta R8.2 (Addgene, cat. no. 12263)
-
Sterile 15-ml tubes
-
37°C water bath
-
Swinging-bucket centrifuge
-
10-cm tissue culture plates (Nunc)
-
Tissue culture inverted microscope
-
Sterile 1.7-ml microcentrifuge tubes (Axygen, cat. no. 14-222-156)
-
0.45-μm syringe filter (Millipore, cat. no. SLHV033R)
-
Centrifugal filter unit (Amicon, Merck, cat. no. UFC910024)
-
Microcentrifuge, 4°C
-
Sterile 0.2-ml PCR tubes (Eppendorf, cat. no. 0030124537)
NOTE : All solutions and equipment coming into contact with cells must be sterile, and proper sterile technique should be used accordingly.
NOTE : All culture incubations are performed in a 37°C, 5% CO2 incubator unless otherwise specified.
Day 1: First seeding of HEK-293T cells
1.Pre-warm two 15-ml tubes containing 10 ml complete RPMI growth medium in a 37°C water bath.
2.Take a vial of frozen HEK-293T cells from liquid nitrogen and immediately partially thaw vial in the water bath. Decontaminate vial using 70% ethanol.
3.Quickly transfer cells into the pre-warmed complete RPMI growth medium from step 1 with a sterile pipet.
4.Centrifuge 5 min at 400 × g at room temperature in a swinging-bucket centrifuge.
5.Discard cells’ supernatant and resuspend cells in the other pre-warmed 10 ml complete RPMI growth medium.
6.Transfer the 10 ml resuspended cells to a 10-cm tissue culture plate and incubate overnight in a 37°C, 5% CO2 incubator.
Day 2: Replace complete RPMI growth medium for HEK-293T cells
7.Pre-warm a 15-ml tube containing 10 ml complete RPMI growth medium in a 37°C water bath.
8.Examine HEK-293T cells from the previous day under a tissue culture inverted microscope.
9.Aspirate and discard supernatant from the plate from step 6 and add the new pre-warmed complete RPMI growth medium from step 7.
10.Return plate to the incubator for overnight incubation.
Day 3: Subculturing (or passaging) cells
11.Examine HEK-293T cells from the previous day under a tissue culture inverted microscope.
12.Pre-warm a 15-ml tube containing 0.5 ml trypsin-EDTA solution and another two tubes each containing 10 ml complete RPMI growth medium in a 37°C water bath.
13.Aspirate and discard cells’ supernatant and add the pre-warmed trypsin-EDTA. Tilt plate from side to side to enable equal distribution of the solution.
14.Incubate for 5 min in the incubator.
15.Set a 1-ml pipettor to 400 µl, tilt plate to a 60° angle, and wash cells down by aspirating the liquid from the bottom of the tilted plate and dispensing it at the top.
16.Transfer a volume of 100 µl of suspended cells into each of the tubes with pre-warmed complete RPMI growth medium from step 12.
17.Transfer the 10 ml from each of the two tubes to two new 10-cm tissue culture plates and return cells to the incubator.
18.Continue to grow cells and split them when they reach 80% to 90% confluence.
Day 5: Seeding of a HEK-293T plate for transfection
19.Take one plate of HEK-293T cells out of incubator and detach cells using trypsin-EDTA solution as in steps 12 to 15.
20.Bring cells to a concentration of 1 × 105 cells/ml in pre-warmed complete RPMI growth medium and seed 10 ml in a 10-cm tissue culture plate.
21.Mark plate so as not to confuse it with other HEK-293T plates in the incubator and incubate plate overnight.
Day 6: Transfection
22.Pre-warm complete RPMI growth medium. Four hours before transfection, replace growth medium of the cells with 10 ml pre-warmed medium.
23.Take TransIT-LT1 Transfection Reagent out of the 4°C refrigerator and allow it to reach room temperature.
24.Create the following mixture of plasmids in a sterile 1.7-ml microcentrifuge tube:
1500 µl Opti-MEM
6 µg vector plasmid (6 µl of 1 mg/ml DNA)
3 µg env plasmid (3 µl of 1 mg/ml DNA)
3 µg packaging plasmid (3 μl of 1 mg/ml DNA).
25.Add 45 µl TransIT-LT1 Transfection Reagent to the plasmid/Opti-MEM mixture and pipet gently with a 1-ml pipettor to mix completely.
26.Incubate mixture for 20 min at room temperature.
27.Gently aspirate and discard 1.5 ml growth medium from the cells from step 22.Add plasmid/TransIT-LT1 Transfection Reagent mixture prepared in steps 24 to 26 to the cultured cells in a dropwise manner.
28.Incubate plate for 48 hr.
Day 8 (48 hr post-transfection): First collection of medium containing viral particles
29.Take plate of transfected cells out of the incubator and transfer supernatant (which contains the viral particles) to a new sterile 15-ml tube. Filter through a 0.45-µm syringe filter. Store collected supernatant at 4°C until step 31.
30.Add 10 ml pre-warmed complete RPMI growth medium to the cells and return plate to the incubator for an additional 24 hr.
Day 9 (72 hr post-transfection): Second collection of medium containing viral particles and concentration to a concentrated viral stock
31.Collect growth medium from the cells and filter through a 0.45-µm syringe filter. Add it to supernatant from the previous day (see step 29).
32.Transfer virus-containing supernatant to the upper part of a centrifugal filter unit and centrifuge for 10 min at 1000 × g , 4°C, in a microcentrifuge.
33.Discard flow-through (from the bottom part of the tube) and top up upper part of the centrifugal filter unit with more supernatant.
34.Repeat steps 31 to 33 until volume of the supernatant is 100 to 250 µl.
35.Without touching the filter's membrane, aspirate the 100 to 250 µl of the concentrated supernatant that is above the filter and transfer it to a new sterile 1.7-ml microcentrifuge tube.
36.Add 250 µl PBS to top part of the filter unit and gently pipet the PBS onto the filter. Transfer PBS and any leftover liquid to the sterile tube with concentrated supernatant from step 35.
37.Mix concentrated medium with PBS well by pipetting until fully homogenized.
38.Divide homogenized solution into 50-µl aliquots in sterile 0.2-ml PCR tubes. Keep aliquots in a –80°C freezer.
Support Protocol 3: FLUORESCENCE TITERING ASSAY FOR THE LENTIVIRAL EXPRESSION VECTOR
Quantification of infectious viral particles (Support Protocol 2) is performed by using the GFP fluorescent reporter gene in the vector and measuring the percentage of GFP-positive cells by flow cytometry.
Materials
-
Complete RPMI growth medium (see recipe) with and without 12 µg/ml polybrene (Merck, cat. no. TR-1003)
-
50-µl viral aliquots (see Support Protocol 2)
-
Trypsin-EDTA solution (Merck, cat. no. T4049-500ML)
-
FACS buffer (see recipe) or PBS (Dulbecco's PBS, without Ca2+ and Mg2+, Biological Industries, cat. no. 02-023-1A)
-
96-well microtiter plate (Nunc, cat. no. 168055)
-
1.7-ml microcentrifuge tubes (Axygen, cat. no. 14-222-156)
-
U-shaped 96-well plate
-
Swinging-bucket centrifuge with 96-well plate adaptor
-
Flow cytometer, equipped with lasers for analysis of GFP
-
Additional reagents and equipment for HEK-293T cell culture (see Support Protocol 2, steps 1 to 18)
NOTE : All solutions and equipment coming into contact with cells must be sterile, and proper sterile technique should be used accordingly.
NOTE : All culture incubations are performed in a 37°C, 5% CO2 incubator unless otherwise specified.
Day 1: Seeding of HEK-293T cells
1.Prepare HEK-293T cell culture as in Support Protocol 2, steps 1 to 18.
2.Prepare cells at a concentration of 0.5 × 105 cells/ml in 10 ml complete RPMI growth medium.
3.Seed cells by dispensing 200 µl into wells B2 to D8 of a 96-well microtiter plate according to the 96-well microtiter plate plan described in Table 1.
4.Incubate overnight in a 37°C, 5% CO2 incubator.
Day 2: Infection
5.Thaw two 50-µl viral aliquots from −80°C freezer.
6.Prepare 1.7-ml microcentrifuge tubes with dilutions of the virus in complete RPMI growth medium with 12 µg/ml polybrene according to Table 2.
Tube # | Volume (µl) | Volume of medium (µl) | Dilution factor |
---|---|---|---|
1 | 100 µl lentiviral stock | 400 | 1 |
2 | 100 µl from tube #1 | 900 | 1:50 |
3 | 100 µl from tube #2 | 900 | 1:500 |
4 | 100 µl from tube #3 | 900 | 1:5,000 |
5 | 100 µl from tube #4 | 900 | 1:50,000 |
6 | 100 µl from tube #5 | 900 | 1:500,000 |
7.Aspirate and discard 100 µl supernatant from each assay well of the plate from step 4.
8.Add 100 µl of each virus dilution (see step 6) to the assay wells according to the plate plan described in Table 1 (with three technical replicates).
9.Incubate cells for 48 hr.
Day 4: Harvest and analyze cells’ fluorescence using a flow cytometer
10.Gently aspirate supernatant from the assay wells.
11.Add 50 µl trypsin-EDTA solution to each well and incubate at 37°C for 5 min.
12.Support Protocol 3, Materials and Step 6: Please specify the preferred tube type.
13.Transfer the 200 µl from each assay well to a U-shaped 96-well plate.
14.Centrifuge 5 min at 400 × g in a swinging-bucket centrifuge with a 96-well plate adaptor.
15.Discard supernatant and resuspend cells in 150 µl FACS buffer.
16.Read fluorescence in a flow cytometer.
17.Calculate the mean percentage of GFP-positive cells in each of the three technical replicates of the samples.
Support Protocol 4: SPLEEN EXCISION, TISSUE DISSOCIATION, AND PREPARATION OF A SINGLE-CELL SUSPENSION
The following protocol is for the extraction of spleen cells and preparation of a single-cell suspension, a prerequisite for various procedures, such as cell separation, cell analysis, and cell culture (see Basic Protocol and Alternate Protocols 1 and 2).
Materials
-
Male or female, 8- to 10-week-old C57BL/6J mice (The Jackson Laboratory, cat. no. 000664)
-
70% (v/v) ethanol
-
PBS (Dulbecco's PBS, without Ca2+ and Mg2+, Biological Industries, cat. no. 02-023-1A)
-
Erythrocyte lysis buffer (ELB; see recipe), 4°C
-
Suitable buffer (for resuspension), 4°C
-
Gauze sponges
-
Straight surgical scissors
-
Curved surgical forceps
-
Sterile 5-ml syringe plungers
-
Culture dishes, 60 × 15 mm (Thermo Fisher, cat. no. 150288)
-
15-ml tubes
-
Swinging-bucket centrifuge, 4°C
-
70-μm mesh strainers (Fisher, cat. no. 22-363-548)
-
Additional reagents and equipment for mouse euthanasia by CO2 asphyxiation or cervical dislocation
NOTE : This protocol applies for other mouse strains as well.
NOTE : All solutions and equipment coming into contact with cells must be sterile, and proper sterile technique should be used accordingly.
1.Euthanize male or female, 8- to 10-week-old mice using either CO2 asphyxiation (<20% displacement/min) or cervical dislocation.
2.Disinfect animals’ fur using 70% ethanol and wipe excess ethanol away using gauze sponges.
3.Using straight surgical scissors and curved surgical forceps, cut skin and remove as much fat and connective tissue as possible. Then, remove spleen and gently place it in cold PBS in a culture dish.
4.Homogenize spleen using the flat top of a sterile 5-ml syringe plunger.
5.Collect cell suspension into a 15-ml tube and rinse culture dish with new cold PBS to collect remaining cells.
6.Leave behind fat and connective tissue.
7.Centrifuge 8 min at 300 × g , 4°C, in a swinging-bucket centrifuge. Discard supernatant.
8.Fully resuspend cell pellet in cold ELB and immediately centrifuge as in step 7.
9.Resuspend cell pellet in 10 ml of a cold suitable buffer and strain through a 70-µm mesh strainer into a new 15-ml tube.
Support Protocol 5: LABELING OF SPLENOCYTES WITH CFSE PROLIFERATION DYE
The protocol below describes the process of labeling fresh splenocytes with CFSE fluorescent tracker prior to adoptive transfer (Alternate Protocol 2).
Materials
-
Splenocytes in single-cell suspension (see Support Protocol 4)
-
Trypan blue (0.4%; Sigma, cat. no. T8154)
-
PBS (Dulbecco's PBS, without Ca2+ and Mg2+, Biological Industries, cat. no. 02-023-1A)
-
25 mM 5(6)-carboxyfluorescein diacetate N -succinimidyl ester (CFSE) cell proliferation dye (Thermo, cat. no. C1157), dissolved in dimethyl sulfoxide (DMSO)
-
Filtered heat-inactivated fetal bovine serum (HI-FBS; Merck-Sigma-Aldrich, cat. no. F7524)
-
Hemocytometer (Hausser Scientific Bright-Line Phase Hemocytometer, Fisher, cat. no. 02-671-6)
-
Light microscope
-
15-ml tubes
-
37°C water bath
-
Swinging-bucket centrifuge, 4°C
NOTE : All solutions and equipment coming into contact with cells must be sterile, and proper sterile technique should be used accordingly.
1.Count splenocytes in single-cell suspension using a with trypan blue using a hemocytometer and light microscope and bring them to a concentration of 20 × 106 cells/ml in PBS.
2.In a new 15-ml tube, prepare CFSE mixture by diluting CFSE cell proliferation dye in PBS to a concentration of 5 × 10-6 M (5 μM).
3.Mix cells with the CFSE solution at a 1:1 ratio (i.e., 1 ml of cells with 1 ml of 5 μM CFSE) and incubate in a 37°C water bath for 10 min. Gently shake tube every 2 min to ensure homogeneity of the cell solution.
4.Add 1 volume of filtered HI-FBS to cells and incubate at room temperature for 1 min (i.e., if 1 ml cells was mixed with 1 ml CFSE, add 2 ml HI-FBS).
5.Top up cells’ tube with PBS and centrifuge for 8 min at 300 × g , 4°C, in a swinging-bucket centrifuge. Discard supernatant.
6.Resuspend cells in 10 ml PBS and count cells with trypan blue using a hemocytometer.
REAGENTS AND SOLUTIONS
Complete RPMI growth medium
- RPMI medium (Gibco, cat. no. 11875119)
- 8% (v/v) HI-FBS (Sigma-Aldrich, cat. no. F7524)
- 2 mM L-glutamine (Biological Industries, cat. no. 03-020-1C)
- 100 IU/ml penicillin-100 µg/ml streptomycin (Merck, cat. no. P4333)
- Store ≤45 days at 4°C
Erythrocyte lysis buffer (ELB)
- 4.011 g ammonium chloride (Sigma, cat. no. 213330) (150 mM final)
- 500.6 mg potassium bicarbonate (Sigma, cat. no. 237205) (10 mM final)
- 80 µl 0.5 M EDTA, pH 8 (Biological Industries, cat. no. 01-862-1B) (80 μM final)
- 500 ml H2O
- Mix well on stir plate
- Adjust to pH 7.2 with HCl
- Sterilize and store sealed ≤1 year at room temperature or 4°C
FACS buffer
- 1 × PBS (Dulbecco's PBS, without Ca2+ and Mg2+, Biological Industries, cat. no. 02-023-1A)
- 1.5% (v/v) HI-FBS (Sigma-Aldrich, cat. no. F7524)
- 0.05% (w/v) sodium azide (Merck, cat. no. S2002)
- Store ≤1 year at 4°C
COMMENTARY
Background Information
MDSCs are a heterogeneous population of immature progenitor cells with features and functions that contribute to the development of an immune-suppressive environment and the perpetuation of various pathologies characterized by chronic inflammation. MDSCs negatively regulate immune responses by inhibiting the activity of effector cells such as natural killer (NK) and T cells and are also known to support tumor growth. Mouse pan- MDSCs are characterized by a CD11b+Gr1+ phenotype and can be subdivided into monocytic [CD11b+Ly6G-Ly6CHi (M-MDSCs)] and granulocytic or polymorphonuclear [CD11b+Ly6G+Ly6CLow (PMN-MDSC)] cells. Identification of human MDSCs is more intricate and is based on several markers (CD11b+CD33+HLA-DR-), and these cells can be further divided into monocytic (CD14+CD11b+CD33+HLA-DR-) and granulocytic (CD15+CD11b+CD33+HLA-DR-) phenotypes (Baniyash, Sade-Feldman, & Kanterman, 2014; Meirow, Kanterman, & Baniyash, 2015; Talmadge & Gabrilovich, 2013). In pathological non-cancerous and cancerous settings characterized by chronic inflammation, immunosuppressive conditions are generated due to MDSC polarization and differentiation arrest. Polarized MDSCs expand in the BM, migrate, and accumulate in the periphery and at inflammatory sites, inducing local and systemic immune suppression (Ben-meir et al., 2018). As MDSCs play a role both in supporting tumor growth and in impairing immune function, their accumulation is recognized as a major obstacle in tumor immunotherapies (Gao et al., 2021; Krishnamoorthy, Gerhardt, & Vareki, 2021). There is a tremendous need to combat chronic inflammatory conditions and associated immune suppression in order to increase the success rates of anti-cancer treatments. Thus, much attention must be allocated to study the key regulatory pathways governing MDSC suppressive biology.
Critical Parameters and Troubleshooting
To obtain reproducible results between experiments, it is important to work with mice within the same age range, not younger than 7 to 8 weeks old, and of the same gender.
The overall health of the mice is important. Mice harboring parasites, for example, may have an alternative Th2 response, which will damage the Th1 immune response against the M.tb vaccination (Moliva, Turner, & Torrelles, 2017). Watch for any abnormal signs in the mice, like skin infections and/or irritations, and monitor the general immune response when repeating experiments by keeping documentation of the spleen weight and MDSC percentages. If you suspect a parasitic infection in your mice, consult with your IACUC-authorized veterinarian about possible treatments and solutions.
Please see Table 3 for a troubleshooting guide.
Problem | Possible cause | Solution |
---|---|---|
M.tb emulsion disseminates into the PBS in Support Protocol 1, step 11 | M.tb emulsion is not stable enough |
1. Re-connect the syringe to the adaptor and repeat mixing the solution 2. Place the emulsion at 4°C for ∼15 min and repeat the mixing step |
High variability between mice in the swelling of the injected paw | 1. M.tb emulsion is not stable 2. M.tb emulsion leaked out from the paw after injection |
1. See above troubleshooting 2. When injecting into the paw in the Basic Protocol, step 10, wait for ∼10 s before withdrawing the needle from the paw |
Understanding Results
Repetitive immunizations induce MDSC accumulation featuring immunosuppressive function
To validate the outcome of this model and assess the developing inflammation, MDSC accumulation, and associated immune suppression, several measurements are used. Measuring body weight as an indication of general distress revealed no differences between the control and inflamed groups (Fig. 1C). Foot swelling as a result of inflammation was followed from the second IFP injection onward, and as expected, the paw of treated mice swelled significantly compared to that of control mice (Fig. 1D). Of note, no visible wounds formed in the swollen paws (Fig. 1B). At the end point of the experiment, spleens of inflamed mice were notable for an increased weight, to 0.189 ± 0.035 g, as compared to 0.044 ± 0.013 g in control mice (Fig. 1E). The increase in the spleen weight was associated with an elevation in MDSC levels that could be evaluated by determining either levels of total MDSCs (CD11b+Gr1+; Supporting Information, Fig. 1) or the sum of both CD11b+Ly6G+Ly6CLow (PMN-MDSCs) and CD11b+Ly6G-Ly6CHi (M-MDSCs) cells, as indicated by percentages and calculated numbers (Fig. 1F and 1G). This increase in MDSC percentages and numbers was also found in the BM (Fig. 1H and 1I).
To evaluate the generated immunosuppressive environment in vivo , levels of splenic T-cell receptor ζ chain (CD247) were evaluated. Downregulated CD247 expression with unchanged cell surface expression of CD3ε or upregulated total, surface, and intracellular CD3ε levels serves as a biomarker for chronic inflammation and associated immune suppression (Eldor et al., 2015; Ezernitchi et al., 2006; Whiteside, 2004). As shown in Figures 1J to 1L, levels of CD247 were downregulated whereas CD3ε expression was not, indicating a suppressed immune system in the inflamed mice. To further demonstrate the suppressive phenotype of MDSCs, we co-cultured naïve CD3ε+ T cells with CD11b+Gr1+ cells isolated from the spleens of inflamed mice at a 1:2 ratio. After 20 hr, the cells were fixed, permeabilized, stained for the expression of CD247 and CD3ε, and analyzed by flow cytometry. As shown in Figures 2A and 2B, T cells co-cultured with MDSCs had lower levels of CD247 compared to the control T cells, independent of the unchanged levels of CD3ε, indicating the suppressive effect mediated by MDSCs ex vivo. The ability of MDSCs to inhibit T-cell activation and proliferation is also a hallmark of the MDSC suppressive phenotype. We thus co-cultured CFSE-labeled T cells during activation with MDSCs isolated from inflamed mice at different T cell/MDSC ratios. After 72 hr, the cells were collected, and the proliferation of T cells was measured by flow cytometry (Fig. 2C and 2D). As expected, T cells co-cultured with MDSCs were significantly inhibited in their proliferation, demonstrating the high suppressive potency of MDSCs. These results reflect the in vivo suppressive function of MDSCs.
Inflamed mice are characterized by a suppressed immune system
To test whether chronic inflammation affects the host's immune status, the ability of adoptively transferred T cells to proliferate in vivo in an antigen-specific manner was measured. To this end, 6 days after the first injection, as described in Figure 4A, mice were immunized with a single intradermal dose of a lentiviral expression vector for the ovalbumin protein (OVA). The OVA peptide presented by cells in the context of the MHC class I molecules is recognized by OT-I transgenic CD8+ T cells (Hogquist et al., 1994; Sade-Feldman et al., 2013). On day 16 of the model, when the peak of the inflammatory response is evident, the mice were injected IV with CFSE-labeled CD45.1+OT-I+ splenocytes. It is important to note that both the donor and the host were of the C57BL/6 background and same gender and that the host expressed CD45.2. Four days later, the proliferative response of the adoptively transferred CD45.1+OT-I+ T cells in the spleen of the inflamed and non-inflamed immunized CD45.2 mice was analyzed by flow cytometry. As seen in Figures 3B to 3D, CD45.1+OT-I+ T cells adoptively transferred into the control group displayed a high proliferative response as compared to the negligible proliferation of T cells injected into the inflamed mice. Taken together, these results indicate that our model of repetitive immunizations with M.tb induces a chronic inflammatory and immune-suppressive environment that resembles the harmful conditions generated in the various pathologies mentioned above.
Time Considerations
Allow the animals to acclimate for ≥3 days prior to the experiment.
Basic Protocol and Alternate Protocol 1: The model requires 16 days for the inflammatory response to develop.
Alternate Protocol 2: This protocol requires 18 days.
Support Protocol 1: Preparation of reconstituted M.tb aliquots takes about 2 to 3 hr in total. Preparation of M.tb-IFA emulsion for injections from the frozen aliquots takes ∼30 min.
Support Protocol 2: For preparation of ovalbumin lentiviral expression vector, an overall time of 3 to 4 weeks should be expected.
Support Protocol 3: The fluorescence titering assay takes 2 days if the HEK-293T cells have been thawed and have undergone at least one passage.
Support Protocol 4: Spleen tissue dissociation and preparation of a single-cell suspension takes about 1 to 2 hr.
Support Protocol 5: Labeling of splenocytes with CFSE proliferation dye takes 1 hr.
Acknowledgments
The authors gratefully acknowledge the support of the Society of Research Associates of the Lautenberg Center and the Harold B. Abramson Chair in Immunology. The authors are also thankful for the grant support of the Israel Science Foundation (ISF), the Israeli Ministry of Health, the Israel Cancer Research Fund (ICRF), the Israel Ministry of Science and Technology, the Gross Foundation, the Bruce and Baila Waldholtz funds, and the Joseph and Matilda Melnick Funds as well as to Ms. Andrea Safir for generously providing editing expertise.
Author Contributions
Kerem Ben-Meir : Conceptualization, Data curation, Formal analysis, Investigation, Methodology, Validation, Writing—original draft; Nira Twaik : Data curation, Methodology; Yaron Meirow : Data curation, Methodology; Michal Baniyash : Conceptualization, Funding acquisition, Project administration, Supervision, Writing—review and editing.
Conflict of Interest
The authors declare no conflict of interest.
Open Research
Data Availability Statement
The data, tools, and supplementary material for this article (or their source) that support the protocols are available from the corresponding author upon reasonable request.
Supporting Information
Filename | Description |
---|---|
cpz1558-sup-0001-SuppMat.pdf1.4 MB | Supplementary Information |
Please note: The publisher is not responsible for the content or functionality of any supporting information supplied by the authors. Any queries (other than missing content) should be directed to the corresponding author for the article.
Literature Cited
- Ashkenazi-Preiser, H., Mikula, I., Baniyash, M., & Baniyash, M. (2021). The diverse roles of myeloid derived suppressor cells in mucosal immunity. Cellular Immunology , 365, 104361. doi: 10.1016/j.cellimm.2021.104361
- Baniyash, M. (2016). Myeloid-derived suppressor cells as intruders and targets: Clinical implications in cancer therapy. Cancer Immunology, Immunotherapy , 65, 857–867. doi: 10.1007/s00262-016-1849-y
- Baniyash, M., Sade-Feldman, M., & Kanterman, J. (2014). Chronic inflammation and cancer: Suppressing the suppressors. Cancer Immunology, Immunotherapy: CII , 63, 11–20. doi: 10.1007/s00262-013-1468-9
- Ben-meir, K., Twaik, N., & Baniyash, M. (2018). Plasticity and biological diversity of myeloid derived suppressor cells. Current Opinion in Immunology , 51, 154–161. doi: 10.1016/j.coi.2018.03.015
- Bronstein-Sitton, N., Cohen-Daniel, L., Vaknin, I., Ezernitchi, A. V., Leshem, B., Halabi, A., … Baniyash, M. (2003). Sustained exposure to bacterial antigen induces interferon-γ-dependent T cell receptor ζ down-regulation and impaired T cell function. Nature Immunology , 4, 957–964. doi: 10.1038/ni975
- Bronte, V., Wang, M., Overwijk, W. W., Surman, D. R., Pericle, F., Rosenberg, S. A., & Restifo, N. P. (1998). Apoptotic death of CD8+ T lymphocytes after immunization: Induction of a suppressive population of Mac-1+/Gr-1+ cells. Journal of Immunology , 161, 5313–5320.
- Cai, S., Choi, J. Y., Borges, T. J., Zhang, H., Miao, J., Ichimura, T., … Azzi, J. R. (2020). Donor myeloid derived suppressor cells (MDSCs) prolong allogeneic cardiac graft survival through programming of recipient myeloid cells in vivo. Scientific Reports , 10, 14249. doi: 10.1038/s41598-020-71289-z
- Eldor, R., Klieger, Y., Sade-Feldman, M., Vaknin, I., Varfolomeev, I., Fuchs, C., & Baniyash, M. (2015). CD247, a novel T cell-derived diagnostic and prognostic biomarker for detecting disease progression and severity in patients with type 2 diabetes. Diabetes Care , 38, 113–118. doi: 10.2337/dc14-1544
- Ezernitchi, A. V., Vaknin, I., Cohen-Daniel, L., Levy, O., Manaster, E., Halabi, A., … Baniyash, M. (2006). TCR zeta down-regulation under chronic inflammation is mediated by myeloid suppressor cells differentially distributed between various lymphatic organs. Journal of Immunology , 177, 4763–4772. doi: 10.4049/jimmunol.177.7.4763
- Fehrenbacher, J. C., Vasko, M. R., & Duarte, D. B. (2012). Models of inflammation: Carrageenan- or complete Freund's adjuvant (CFA)-induced edema and hypersensitivity in the rat. Current Protocols in Pharmacology , 56, 5.4.1–5.4.7. doi: 10.1002/0471141755.ph0504s56
- Gabrilovich, D. I., Bronte, V., Chen, S. H., Colombo, M. P., Ochoa, A., Ostrand-Rosenberg, S., & Schreiber, H. (2007). The terminology issue for myeloid-derived suppressor cells. Cancer Research , 67, 425–425. doi: 10.1158/0008-5472.CAN-06-3037
- Gao, X., Sui, H., Zhao, S., Gao, X., Su, Y., & Qu, P. (2021). Immunotherapy targeting myeloid-derived suppressor cells (MDSCs) in tumor microenvironment. Frontiers in Immunology , 11, 3749. doi: 10.3389/fimmu.2020.585214
- Hogquist, K. A., Jameson, S. C., Heath, W. R., Howard, J. L., Bevan, M. J., & Carbone, F. R. (1994). T cell receptor antagonist peptides induce positive selection. Cell , 76, 17–27. doi: 10.1016/0092-8674(94)90169-4
- Krishnamoorthy, M., Gerhardt, L., & Vareki, S. M. (2021). Immunosuppressive effects of myeloid-derived suppressor cells in cancer and immunotherapy. Cells , 10, 1170. doi: 10.3390/cells10051170
- Lin, L. C. W., Smith, S. A., & Tscharke, D. C. (2012). An intradermal model for vaccinia virus pathogenesis in mice. Methods in Molecular Biology , 890, 147–159. doi: 10.1007/978-1-61779-876-4_9
- Louis, K. S., & Siegel, A. C. (2011). Cell viability analysis using trypan blue: Manual and automated methods. Methods in Molecular Biology , 740, 7–12. doi: 10.1007/978-1-61779-108-6_2
- Marvizon, J. C., Walwyn, W., Minasyan, A., Chen, W., & Taylor, B. K. (2015). Latent sensitization: A model for stress-sensitive chronic pain. Current Protocols in Neuroscience , 71, 9.50.1–9.50.15. doi: 10.1002/0471142301.ns0950s71
- Meirow, Y., Kanterman, J., & Baniyash, M. (2015). Paving the road to tumor development and spreading: Myeloid-derived suppressor cells are ruling the fate. Frontiers in Immunology , 6, 523. doi: 10.3389/fimmu.2015.00523
- Moliva, J. I., Turner, J., & Torrelles, J. B. (2017). Immune responses to Bacillus Calmette–Guérin vaccination: Why do they fail to protect against Mycobacterium tuberculosis? Frontiers in Immunology , 8, 407. doi: 10.3389/fimmu.2017.00407
- Ostrand-Rosenberg, S., Sinha, P., Chornoguz, O., & Ecker, C. (2012). Regulating the suppressors: Apoptosis and inflammation govern the survival of tumor-induced myeloid-derived suppressor cells (MDSC). Cancer Immunology, Immunotherapy , 61, 1319–1325. doi: 10.1007/s00262-012-1269-6
- Park, Y., & Kwok, S. K. (2022). Recent advances in cell therapeutics for systemic autoimmune diseases. Immune Network , 22(1), e10. doi: 10.4110/in.2022.22.e10
- Reuven, O., Mikula, I., Ashkenazi-Preiser, H., Twaik, N., Ben-Meir, K., Meirow, Y., Daniel, L., Kariv, G., Kurd, M., & Baniyash, M. (2022a). Phenotypic characterization and isolation of myeloid-derived suppressor cells. Current Protocols , 2, e561. doi: 10.1002/cpz1.561
- Reuven, O., Mikula, I., Ashkenazi-Preiser, H., Twaik, N., Ben-Meir, K., Meirow, Y., Daniel, L., Kariv, G., Kurd, M., & Baniyash, M. (2022b). Functional assays evaluating immunosuppression mediated by myeloid derived suppressor cells. Current Protocols , 2, e557. doi: 10.1002/cpz1.557
- Sade-Feldman, M., Kanterman, J., Ish-Shalom, E., Elnekave, M., Horwitz, E., & Baniyash, M. (2013). Tumor necrosis factor-α blocks differentiation and enhances suppressive activity of immature myeloid cells during chronic inflammation. Immunity , 38, 541–554. doi: 10.1016/j.immuni.2013.02.007
- Saha, K., Lin, Y. C., & Wong, P. K. Y. (1994). A simple method for obtaining highly viable virus from culture supernatant. Journal of Virological Methods , 46, 349–352. doi: 10.1016/0166-0934(94)90005-1
- Salgame, P. (2005). Host innate and Th1 responses and the bacterial factors that control Mycobacterium tuberculosis infection. Current Opinion in Immunology , 17, 374–380. doi: 10.1016/j.coi.2005.06.006
- Sandell, L., & Sakai, D. (2011). Mammalian cell culture. Current Protocols Essential Laboratory Techniques , 5, 4.3.1–4.3.32. doi: 10.1002/9780470089941.et0403s5
- Seung, L. P., Rowley, D. A., Dubey, P., Schreiber, H., & Rowley, J. D. (1995). Synergy between T-cell immunity and inhibition of paracrine stimulation causes tumor rejection (immune escape/polymorphonuclear cells/tumor infiltration/tumor specificity). Proceedings of the National Academy of Sciences , 92, 6254–6258. doi: 10.1073/pnas.92.14.6254
- Song, H. K., Noorchashm, H., Lieu, Y. K., Rostami, S., Greeley, S. A. S., Barker, C. F., & Naji, A. (1999). In vivo MLR: A novel method for the study of alloimmune responses. Transplantation Proceedings , 31, 834–835. doi: 10.1016/S0041-1345(98)01793-X
- Talmadge, J. E., & Gabrilovich, D. I. (2013). History of myeloid-derived suppressor cells. Nature Reviews Cancer , 13, 739–752. doi: 10.1038/nrc3581
- Vaknin, I., Blinder, L., Wang, L., Gazit, R., Shapira, E., Genina, O., … Baniyash, M. (2008). A common pathway mediated through Toll-like receptors leads to T- and natural killer–cell immunosuppression. Blood , 111, 1437–1447. doi: 10.1182/blood-2007-07-100404
- Wagner, S., Bindler, J., & Andriambeloson, E. (2008). Animal models of collagen-induced arthritis. Current Protocols in Pharmacology , 43, 5.51.1–5.51.8. doi: 10.1002/0471141755.ph0551s43
- Wang, S., Tan, Q., Hou, Y., & Dou, H. (2021). Emerging roles of myeloid-derived suppressor cells in diabetes. Frontiers in Pharmacology , 12, 3749. doi: 10.3389/fphar.2021.798320
- Whiteside, T. L. (2004). Down-regulation of ζ-chain expression in T cells: A biomarker of prognosis in cancer? Cancer Immunology, Immunotherapy , 53, 865–878. doi: 10.1007/s00262-004-0521-0
- Woodward, W. R., Choi, D., Grose, J., Malmin, B., Hurst, S., Pang, J., … Pillers, D. A. M. (2007). Isoflurane is an effective alternative to ketamine/xylazine/acepromazine as an anesthetic agent for the mouse electroretinogram. Documenta Ophthalmologica , 115, 187–201. doi: 10.1007/s10633-007-9079-4
- Yan, L., Liang, M., Yang, T., Ji, J., Jose Kumar Sreena, G. S., Hou, X., … Feng, Z. (2020). The immunoregulatory role of myeloid-derived suppressor cells in the pathogenesis of rheumatoid arthritis. Frontiers in Immunology , 11, 2216. doi: 10.3389/fimmu.2020.568362
- Zimmerman, M., Hu, X., & Liu, K. (2010). Experimental metastasis and CTL adoptive transfer immunotherapy mouse model. JoVE (Journal of Visualized Experiments) , e2077.
Citing Literature
Number of times cited according to CrossRef: 3
- Amrita Chatterjee, Monisha Jayaprakasan, Anirban Kr Chakrabarty, Naga Rajiv Lakkaniga, Bibhuti Nath Bhatt, Dipankar Banerjee, Avinash Narwaria, Chandra Kant Katiyar, Sunil Kumar Dubey, Comprehensive insights into rheumatoid arthritis: Pathophysiology, current therapies and herbal alternatives for effective disease management, Phytotherapy Research, 10.1002/ptr.8187, 38 , 6, (2764-2799), (2024).
- Or Reuven, Ivan Mikula, Hadas Ashkenazi‐Preiser, Nira Twaik, Kerem Ben‐Meir, Yaron Meirow, Leonor Daniel, Guy Kariv, Mahdi Kurd, Michal Baniyash, Phenotypic Characterization and Isolation of Myeloid‐Derived Suppressor Cells, Current Protocols, 10.1002/cpz1.561, 2 , 10, (2022).
- Or Reuven, Ivan Mikula, Hadas Ashkenazi‐Preiser, Nira Twaik, Kerem Ben‐Meir, Yaron Meirow, Leonor Daniel, Guy Kariv, Mahdi Kurd, Michal Baniyash, Functional Assays Evaluating Immunosuppression Mediated by Myeloid‐Derived Suppressor Cells, Current Protocols, 10.1002/cpz1.557, 2 , 10, (2022).