Cell Lysis, Two-Step Purification, and RNase Digestion
Clémentine Delan-Forino, David Tollervey
RNA degradation
Protein–RNA interaction
RNA-binding sites
UV cross-linking
Yeast
Exosome
RNA processing
Abstract
The RNA exosome complex functions in both the accurate processing and rapid degradation of many classes of RNA in eukaryotes and Archaea. Functional and structural analyses indicate that RNA can either be threaded through the central channel of the exosome or more directly access the active sites of the ribonucleases Rrp44 and Rrp6, but in most cases, it remains unclear how many substrates follow each pathway in vivo. Here we describe the method for using an UV cross-linking technique termed CRAC to generate stringent, transcriptome-wide mapping of exosome–substrate interaction sites in vivo and at base-pair resolution.
We present a protocol for the identification of RNA interaction sites for the exosome, using UV cross-linking and analysis of c DNA (CRAC) [ 1 , 2 ]. A number of related protocols for the identification of sites of RNA–protein interaction have been reported, including HITS-CLIP, CLIP-Seq, iCLIP, eCLIP, and others [ 3 , 4 , 5 , 6 ]. These all exploit protein immunoprecipitation to isolate protein–RNA complexes. CRAC is distinguished by the inclusion of tandem affinity purification and denaturing purification, allowing greater stringency in the recovery of authentic RNA–protein interaction sites.
To allow CRAC analyses, strains are created that express a “bait” protein with a tripartite tag. This generally consists of His6, followed by a TEV-protease cleavage site, then two copies of the z-domain from Protein A (HTP). The tag is inserted at the C terminus of the endogenous gene within the chromosome. The fusion construct is the only version of the protein expressed and this is under the control of the endogenous promoter. Several alternative tags have been successfully used, including a version with N-terminal fusion to a tag consisting of 3× FLAG-PreSission protease (PP) cleavage site-His6 (FPH) [ 7 ]. This is a smaller construct and is suitable for use on proteins with structures that are incompatible with C-terminal tagging. An additional variant is the insertion of a PP site into a protein that is also HTP tagged. This allows the separation of different domains of multidomain proteins. Importantly, the intact protein is cross-linked in the living cell, with domain separation in vitro. This has been successfully applied to the exosome subunit Rrp44/Dis3 to specifically identify binding sites for the PIN endonuclease domain [ 8 ].
Briefly, during standard CRAC analyses, covalently linked protein–exosome complexes are generated in vivo by irradiation with UV-C (254 nm). This generates RNA radicals that rapidly react with proteins in direct contact with the affected nucleotide (zero length cross-linking). The cells are then lysed and complexes with the bait protein are purified using an IgG column. Protein–RNA complexes are specifically eluted by TEV cleavage of the fusion protein and cross-linked RNAs trimmed using RNase A/T1, leaving a protected “footprint” of the protein binding site on the RNA. Trimmed complexes are denatured using 6 M Guanidinium, immobilized on Ni-NTA affinity resin and washed under denaturing conditions to dissociate copurifying proteins and complexes. The subsequent enzymatic steps are all performed on-column, during which RNA 3′ and 5′ ends are prepared, labeled with 32P (to allow RNA–protein complexes to be followed during gel separation) and linkers ligated. Note, however, that alternatives to using 32P labeling have been reported (e.g., [ 6 ]). The linker-ligated, RNA–protein complexes are eluted from the Ni-NTA resin and size selected on a denaturing SDS-PAGE gel. Following elution, the bound RNA is released by degradation of the bait protein using treatment with Proteinase K. The recovered RNA fragments are identified by reverse transcription, PCR amplification and sequencing using an Illumina platform.
Relative to CLIP-related protocols, CRAC offers the advantages of stringent purification, that substantially reduces background, and on-bead linker ligation that simplifies separation of reaction constituents during successive enzymatic steps. It also avoids the necessity to generate high-affinity antibodies needed for immunoprecipitation. Potential disadvantages are that, despite their ubiquitous use in yeast studies, tagged constructs may not be fully functional. This can be partially mitigated by confirming the ability of the tagged protein to support normal cell growth and/or RNA processing, or by comparing the behavior of N- and C-terminal tagged constructs. Additionally, because linkers are ligated to the protein–RNA complex, a possible disadvantage is that UV-cross-linking of the RNA at, or near, the 5′ or 3′ end it may sterically hinder on-column (de)phosphorylation and/or linker ligation. With these caveats, CRAC has been successfully applied to >50 proteins in budding yeast, and in other systems ranging from pathogenic bacteria to viral infected mouse cells [ 7 , 9 ].
Before start
Appropriate negative controls and experimental replicates are required to determine the background signal and true positive binding sites. We routinely use the (untagged) yeast parental strain as a negative control, performing a minimum of two biological and technical replicates for each sample. It is commonly observed that technical replicates (even samples from the same culture) processed in two independent CRAC experiments show more differences than two biological replicates (independent cultures) processed together.
All steps should be performed wearing disposable gloves and materials should be free of DNase and RNase. Prior to each CRAC experiment, pipettes should be cleaned with DNAZap (ThermoFisher; AM9890) to avoid DNA contamination at the PCR step, followed by RNaseZAP (ThermoFisher; AM9890) treatment, and rinsed with deionized water. All the buffers should be prepared with deionized water and free of RNases; however, DEPC treatment is not normally essential. To minimize buffer contamination, adjust the pH by taking small aliquots for measurements. Filter-sterilize stock solutions following preparation, and store at 4 °C. Where required, add β-mercaptoethanol and protease inhibitors to the buffers shortly before use. Wash buffers should be prepared immediately before starting the CRAC experiment.
Steps
All steps must be carried out on ice, unless stated otherwise. For troubleshooting, it is a good idea to monitor the course of the experiment by retaining samples at points during the CRAC protocol. This allows potential problems with Protein–RNA purification steps to be identified. Three aliquots per sample are taken during the purification (Subheading 3.2.2 3.2.2 “Crude Lysate” and “IgG supernatant,” Subheading 3.2.3 3.2.3 “TEV Eluate”). These can be analyzed by Western blot.
Cell Lysis
Add one volume (1mL
) of TN150 lysis buffer (+ protease inhibitors) to cell pellets and thaw rapidly.
Add 2.5 volumes of Zirconia beads to cell pellet (~ 2.5mL
, measure in 15 ml Falcon tubes). Vortex the cells 0h 1m 0s
, then put them On ice
for 0h 1m 0s
between each vortexing step. Repeat five more times.
Add three volumes of TN150 (+ protease inhibitors) (~3mL
), shake vigorously, and centrifuge the suspension in the falcon tube (4000x g,4°C
).
Transfer the supernatant (~ 4.5mL
) to three 1.5 ml tubes and spin lysate at 16000x g,4°C
in a microcentrifuge. Keep tubes On ice
throughout the process.
Keep supernatants On ice
, ready to be mixed with IgG sepharose beads in Step 10 below.
Protein A:IgG Purification
Resuspend IgG Sepharose beads by gently inverting the bottle. Pipette out 167µL
into a 15 ml tube. The bead:buffer ratio is approximately 75:25 so this equates to 125 μl of beads. Wash the beads twice with 5mL
. Collect beads between washes by pulsing in the centrifuge to 1000rpm,0h 0m 0s
(210x g,0h 0m 0s
).
Remove the supernatant after the second wash and add 125µL
per sample. Gently resuspend the beads, and aliquot 250µL
per sample into 15 ml tubes.
Take 5µL
aliquots for troubleshooting the purification, and store at -20°C
.
Mix remaining lysates with IgG Sepharose beads and rotate for a minimum of 2h 0m 0s
at 4°C
. This step can be extended to .
Collect the beads by pulsing to 1000rpm,4°C,0h 0m 0s
and remove most of the supernatant.
Take a 5µL
aliquot for troubleshooting (“IgG supernatant”) and store at -20°C
.
Wash beads twice with 10mL
and twice with 10mL
For each wash, gently agitate at 4°C
for 0h 5m 0s
.
Gently resuspend gently the beads in 600µL
and transfer to a 1.5 ml tube.
TEV Cleavage
Add 20units (U)
–30units (U)
of TEV protease to the beads and mix by inverting tube.
Incubate at 18°C
for 2h 0m 0s
with shaking (make sure beads remain in suspension).
Pass the mixture through a microcentrifuge column (SnapCap) to remove the beads. Spin column (1000rpm,0h 0m 0s
) to collect all the eluate in a 1.5 ml tube. Do not put eluate on ice.
Take a 25µL
(“TEV eluate”) and store at -20°C
.
RNase Digestion and Binding to Ni-NTA Resin
Prepare 1.5 ml microcentrifuge tubes containing 0.4grams (g)
.
Prepare 50µL
by pipetting 100µL
into tubes and wash them twice with 1mL
. Leave the resin in about 100µL
until use.
Add 1µL
(0.15 units, Agilent) to 550µL
. Incubate for 0h 5m 0s
at 37°C
.
Immediately transfer the RNase treated eluate into the prepared microcentrifuge tubes containing guanidine hydrochloride. The final volume will be around 700µL
.
Add 27µL
(300millimolar (mM)
final concentration) and 3µL
(10millimolar (mM)
final concentration) to the eluate.
Remove the remaining supernatant from the washed Ni-NTA resin. Add the eluate to the resin.
Incubate with gentle agitation at 4°C
.
Pulse the microcentrifuge tubes to collect the resin. Remove the supernatant and wash three times with 400µL
and three times with 400µL
.
Transfer the resin to a microcentrifuge column (SnapCap).
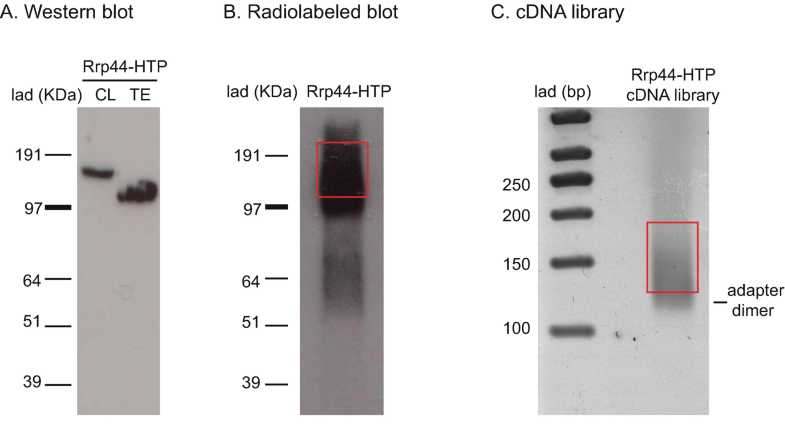